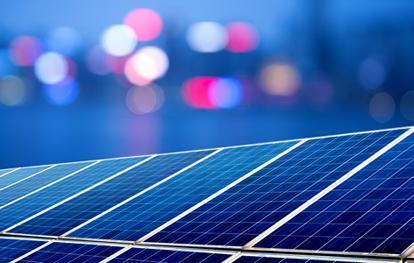
24 minute read
Integrating Sustainable Energy Solutions into Urban Design
Tamer Aly Kamel
Advertisement
Supervisors:
Prof. Dr. Yehya M. Serag, Ain Shams University Prof. Dipl.-Ing. Antje Stokman, University of Stuttgart Prof. Dipl.-Ing. (hons) Carsten Vellguth, Ain Shams University
Abstract
The residential sector is the largest consumer of energy globally and locally in Egypt. Thus, it is also the largest contributor to greenhouse gases. However, if the residential sector manages to reach sustainable energy autonomy, its contribution to greenhouse gas emissions has the potential to be neutralized. Photovoltaic (PV) panels bring clean energy directly into the built environment and, since the housing market in Egypt is dominated by the private sector, there is potential for real estate developers to not only draw out long-term and
large-scale sustainable development plans, but make financial profit from selling energy. This thesis argues for a sustainable development model which caters for private real estate developers. Taking Al-Rehab City as a case study, it examines the projected energy yield from PV panels in comparison to energy consumption. In addition, various spatial solutions are proposed to improve the overall efficiency, pushing the proposed model closer to energy autonomy. Moreover, this thesis also examines the financial aspect of such development, calculating investment costs and financial profit, while studying different investment and management scenarios. Furthermore, environmental impact is studied showing the total reduction in carbon emissions in comparison with conventional energy generation sources. Finally, this paper reflects on findings, calling for a national policy to adopt the proposed approach, further offsetting the residential sector from energy demands and carbon emissions.
Keywords: Energy Autonomy, Photovoltaic Panels, Sustainable Development, Al-Rehab City, Cairo
Introduction
As humanity ventures deeper into the Anthropocene, it is now clear that climate change is real, and humanbased activities are responsible for it. The production of electricity is the largest producer of greenhouse gas emissions while the residential sector consumes 40% of the global energy produced, making it responsible for 25% of total carbon emissions. Yet, as the problem is defined, a solution presents itself, and that is the potential to offset 25% of carbon emissions if the residential sector was supplied with clean energy. PV technology is a clean, effective, reliable and a proven alternative source of energy. PV panels are small and modular, which makes them suitable for the built environment, especially as roof mount installations. This brings the generation of clean energy into the residential sector – the root of the problem. At this point, a few questions present themselves: By capitalizing on this coupling, can the residential sector reach energy autonomy using photovoltaic panels? Which subsection of the residential sector is most tuned to rollout such scenario in a reliable manner? Can this present a financially sound investment opportunity? What is the annual reduction in carbon emissions achievable using such an approach?
The residential sector can achieve energy autonomy using roof-mounted PV panels as long as buildings are under three floors high. However, if roofs were designed for this usage, PV output can increase by 20%, pushing the autonomy limit closer to four floors. However, one cannot rely on individual decisions by tenants to invest in PV panels. Therefore, it is more reliable to target a housing project for this approach. A developer can apply this development scheme across an entire housing project which would present higher coverage figures. To test this scheme, Al-Rehab City was taken as a case study as it is considered the most successful example of private development in Cairo. After calculating energy yield from PV panels and average consumption, apartment buildings and houses can cover 60% and 130% of their demands respectively. However, when combined, and after adding more panels in public buildings, parking lots and parks, the entire project can cover 91.20% of its total demand. Yet, if the project was designed with the usage of PV panels in mind, slanting roofs and keeping buildings under four floors can optimize coverage figures to reach 134.20%. Energy generated from installed PV
panels would save 24.30 tones of Co2 from being released every year, which constitutes 77.70% of emissions from current sources. Moreover, a new dynamic energy tariff is proposed so that residents could benefit from the installed panels and provide them with an incentive to reduce their individual consumption, further reducing carbon emissions. Financing PV panels for a project like Al-Rehab City would increase total construction costs by less than 5%, while energy harvested can be sold for a potential annual profit of EGP 413 million, forming an investment scheme that pays back for itself in 5.6 years and 19.4 years of profit. Thus, the scheme offers developers a very profitable investment which presents a win-win scenario for all.
According to figures used, the residential sector can achieve energy autonomy with relative ease using PV panels, particularly if projects were planned for PV usage. If the public sector keeps this in mind, a planning policy can be initiated requiring energy autonomy for new developments. This would offset the carbon emissions share of new developments, eventually neutralizing a massive portion of emissions.
Problem Background
The emissions of greenhouse gasses (GHG) from human activities constitute the primary driving force behind climate change. Carbon emissions from burning fossil fuels constitute the largest portion of those emissions, contributing with 65% of total global GHG emissions. Moreover, looking at GHG emissions by the economic sector, the production of electricity and heating is responsible for the largest share of emissions contributing with 25% of total emissions (United States Environmental Protection Agency, 2016). Furthermore, the residential sector consumes 40% of global energy and is responsible for one-third of GHG emissions (United Nations Environment Programme, 2016). Therefore, it is obvious that the residential sector presents a critical element in both the consumption of energy, and the production of GHG. In parallel, buildings also offer a massive potential to offset an enormous amount of GHG emissions if proper sustainable management was applied. However, before venturing into exploring solutions, the local energy background must be studied. The local condition in Egypt is very similar to the global one as the residential sector consumes 43% of the total energy produced (Egyptian Electric Utility for Consumer Protection and Regulatory Agency, 2015). Additionally, it is also responsible for 45% of peak demands in Egypt (El-Khatam, 2013). On the other hand, renewable energy forms only 2% of the current installed capacity with steam and combined cycle forming the vast majority of generation sources. By 2025, the share of renewables is set to reach 20% and, thus, the public sector has launched a feed-in tariff (FiT) program to attract contributions from individuals and enterprises (Egyptian Electricity Holding Company, 2016).
That being said, a defined problem naturally presents a solution. In this case, the residential sector’s share of carbon emissions can be radically mitigated if it was supplied with clean energy. PV panels already bring energy generation inside the building envelope, where it is consumed. Capitalizing on this coupling shows a potential to reach sustainable energy autonomy. In this case, there is a potential for buildings to generate an amount of energy equivalent to their demand, which can be done individually or collectively. The results in both cases would be the almost complete removal of the residential sector from both energy generation and carbon emission concerns. Solar energy has established itself as a reliable clean energy alternative. Although there are various methods of harvesting solar energy, this thesis is strictly concerned with PV panels. PV panels convert
light from the sun into electricity by utilizing the photoelectric effect. Materials with a photoelectric property can absorb light energy and release electrons, which can be used to generate an electric current. The true potential of PV panels, however, lies in two characteristics. Firstly, the relative small size and high output of the panels, coupled with their modular structure which introduces the generation of clean energy into the built environment. Initially, an architect was mostly targeting sustainable solutions which would reduce a building’s energy consumption. However PV panels add another dimension to the architect’s toolbox as they can now integrate energy generation in the design process and thereby introduce the concept of energy autonomy. Secondly, PV panels have received a major recognition after several governments applied FiT programs. These programs created a solid financial investment, and governments have capitalized on this financial aspect to encourage contributions from both individuals and the private sector. This has served to rapidly increase the total share of renewable energy and the success of these programs is witnessed as surges in the global PV markets which occurred after governments utilized FiT policies. Such policies are also responsible for the developments of PV panels and their affordability (Cory, Couture and Kreycik, 2009).
Finding a Trajectory
Initially speaking, the problem presented can be targeted using two opposite approaches, reducing electricity consumption or substituting conventional fossil fuel generation sources with clean alternatives. Reducing electricity consumption would decrease demand, and eventually, less energy has to be provided which would reduce carbon emissions. This reduction is viable by utilizing sustainable design and efficient fixtures. Although this would promise significant results, in reality, it is very challenging as there is no collective conscious for sustainable design or efficient fixtures, and in addition, they offer limited incentives. Thus, any progress would rely on individual actions rendering this approach unreliable and unpredictable. On the other hand, substituting fossil fuel energy generation with renewable ones would prove to be more practical and realistic. Strictly regarding PV panels, there are two different approaches for this: centralized and distributed generation. Both have particular strengths and weaknesses.
Centralized generation means large power plants. These are usually built by governments which makes them easier and cheaper to construct. However, they offer limited room for contributions from other sectors and are less reliable in terms of plant failure. Moreover, power plants require transmission lines which add extra costs, power loss and health risks. Distributed generation works in the opposite way, relying on small scattered plants in the form of roof mount PV systems. Although more expensive, distributed generation is more reliable in the case of plant failure and energy losses (SMA Solar Technology AG, n.d). Additionally, distributed generation can utilize FiT programs and presents the potential to introduce the concept of energy autonomy as the energy is produced and consumed in the same location.
Under distributed generation, there are two installation methods: off-grid and grid-tied installations. An off-grid installation is completely independent from the grid and, thus, requires batteries to power it during the night. Batteries are expensive and troublesome, which renders the system more expensive and less reliable. A grid-tied system, on the other hand, would use energy harvested during morning hours, and draw energy from the grid at
night, making the system cheaper and more reliable. In addition, this system can also utilize FiT policies (Mæhlum, 2013). Therefore, a grid-tied system is the more appropriate installation method as it is more feasible, reliable and can offer financial incentives.
Typically, in this scenario, tenants pay less for electricity as they draw less energy from the grid. Although this would be attractive for tenants, it does not offer any incentives for developers, and as mentioned before, tenants are unable to deploy a large scale sustainable development scheme. An alternative usage scenario is to feed energy harvested directly into the grid capitalizing on FiT policies, or sell energy to residents for a financial return. This scenario favours developers and investors as it offers a sound investment scheme.
After studying different installation and usage scenarios, it is clear that decentralized grid-tied system while selling harvested energy is the most practical approach as it favours and targets developers and does not rely on individual altruistic actions. A developer can apply the proposed scenario with relative ease as they are the main decision-maker and, in return, can reap the financial benefits from the installed system.
Case Study
Housing projects built by the private sector offer a promising entry point for sustainable development. Al-Rehab City is one of the first housing projects built, and is arguably the most successful example of private developments in Cairo (M. Yousry, n.d.). Al-Rehab City is an integrated housing project located in New Cairo City. It spreads over a total area of ten million square meters and is expected to host 200,000 inhabitants (M. Yousry, n.d.). The project offers a mixture of middle and upper-class housing units in apartment buildings and single-family houses in addition to public buildings and other services. The construction of Al-Rehab City started in 1997 by building the first two phases from the original six. However, after the success demonstrated by unit sales and settling rates, the developer acquired an additional land to build phases seven to ten (M. Yousry, n.d.). Phases one and two roughly make 20% of the project, and are two of the most completed and inclusive phases. Thus, they are taken as the initial study zone. These two phases incorporate apartment buildings, houses, two mosques and three schools, in addition to parking lots and public parks.
For a sustainable development scheme using PV panels in a project like Al-Rehab City, the main assets for development are the roofs of residential buildings. Although covering these roofs in PV panels has the potential to cater for the majority of electricity demand, the development scheme should not stop at this point. In this context, there are an abundance of other surfaces where the scheme can be expanded upon increasing energy yield and coverage. These surfaces are public buildings, parking lots and public parks. Public buildings are represented in mosques and schools. Both of these building types offer huge volumes and footprints, and minimal energy demands, which makes them ideal for additional PV installations. Mosques are used for a few minutes, five times a day, while schools are only used during daylight hours and are closed during summer, and lighting forms the majority of electricity demand for both building types. Therefore, simple environmentally conscious architecture design can optimize daylight and ventilation, and by using LED lighting, the electricity demand of schools and mosques can be reduced to negligible figures. Thus, the calculations in the next chapters assume that schools and mosques have no electricity demand.
In addition, the project also offers vast asphalt covered streets dedicated to car parking. Covering these lots with PV panels would not only contribute to the total energy yield, but will also protect cars from the blazing summer heat and reduce the overall urban heat island effect in the neighbourhood. Moreover, public parks form 60% of the total footprint of apartment buildings, and are mostly green lawns with very few trees, shading or seats. Covering portions of these parks in PV panels on high mounting structures would further increase the total energy yield, and additionally, provide shade with the possibility of introducing seating elements in the structure itself, improving the overall usage of parks and the quality of life within the neighbourhood. Mounting structures can be scattered around parks to cover a proposed 15% of the total area in PV panels. These additional surfaces would finally constitute around one-third of the total installation surfaces, and will contribute with a similar share in total energy yield.
Applications and Calculations
Before detailing the applications of PV panels in Al-Rehab City and calculating energy yield and coverage, it is important to list the standards upon which the calculations are done. According to Mr. Hossam Al-Zayat10, the installation of a one megawatt (MW) grid-tied PV system would require ten square meters and cost EGP 13,000. Additionally, this system would produce an average of 1,750 kilowatt hours (kWh) of electricity annually. Moreover, the most optimum orientation for PV panels in Cairo is between 20° and 30° south. An average angle of 25° is used in the calculations to follow. Concerning electricity consumption, according to Mr. Abdelazeez Zahran11 (2016), the monthly average residential electricity consumption in Al-Rehab City is 6.15 kWh/v and 4.3 kWh/m2 for apartment buildings and houses respectively. However, to maintain a safety margin, the higher figure is used for both calculations. That being said, when supplying electricity to a pool of units, the total provided electricity does not mirror the total sum of consumption, but only covers a specific percentage of the projected total demand, and this is referred to as a demand factor. Demand factor for the residential sector can go as low as 60% (Electrical Notes & Articles, 2011). However according to Mr. Zahran the demand factor used to supply electricity in Al-Rehab City is 80%, and accordingly, this figure will be used to calculate electricity demands.
According to these figures, a typical apartment building in Al Rehab City – Building Type A – which is five stories high with a footprint of 355 m2, would roughly offer a monthly output of 5,180 kWh in electricity from PV panels, and a demand of 8,635 kWh. Therefore, the building would cover around 60% of its demand by retrofitting the roof with PV panels. Moving into houses, a typical single-family house – Villa Type A – which is two stories high with a footprint of 100 m2, would roughly offer an average monthly energy yield of 1,450 kWh, and a demand of 1,000 kWh, hence, an average coverage figure of 130%. These coverage rates, however, can be improved by adjusting the installation surface itself. As mentioned previously, the ideal inclination angle of PV panels in Cairo is 25° south which, when installed on a horizontal surface, casts a shadow covering around 10% of the total surface, reducing space efficiently. However, if the installation surface itself (roof) was inclined to comply with the same installation angle, then the output would increase by 20%. This is due to eliminating
10 CEO of SUNERGY, Cairo based renewable energy solutions provider 11 Head of electrical engineering sector of Al-Rehab City
shadows (+10%) and the fact than an inclined surface is longer than its horizontal projections (+10%). Construction wise, it is impractical to slant the roofs of existing buildings, which makes this solution more ideal for new constructions. The proposed inclination also offers a few environmental benefits as it increases the volume of the last floor and offers the possibility to create a wind-catcher-like effect by placing a high window on the northern wall, improving ventilation and the microclimate. Although this inclination angle is rather high and might prove to be impractical, various architectural solutions can be applied for the spatial distribution of apartments and units to make it more realistic.
Adding this 20% potential increase in output from PV panels after slanting roofs and by comparing output with demand per square meter, it is recommended to keep apartment buildings under four floors for better coverage. In this case, Building Type A would have an optimized output of 6.215 kWh and its demand would decrease to form 6,900 kWh, forming an optimized coverage figure of 90%, and 72% if kept at five floors high. In parallel, slanting the roof of Villa Type A would increase output to form 1,740 kWh, while demand would not change, forming a final optimized coverage figure of 158%.
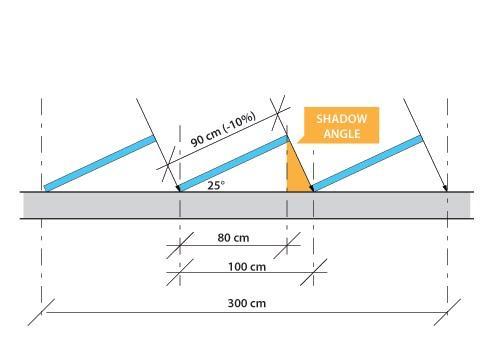
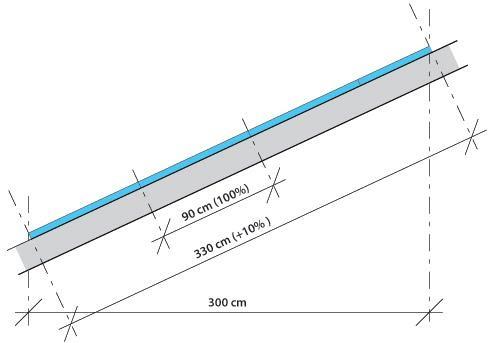
Results
After allocating installation surfaces and calculating initial coverage figures, the next step is to project the overall image for phases one and two. Starting with residential buildings, phases one and two can have a potential installation capacity of 17.2 MW for apartment buildings and 13.8 MW for houses, with an additional 4.9 MW from parking lots. Moreover, public buildings and public parks would add another 2.8 MW combined. This will form a total capacity of 39.8 MW and a potential annual energy yield of 69.7 GWh. On the other hand, the annual demand from apartment buildings and villas would form 69.3 GWh, which translates into an energy coverage rate of 100.50%. This means that covering the proposed surfaces in these phases would already achieve energy autonomy. However, if these phases were designed from scratch, applying the optimization solutions mentioned previously has the potential to increase this coverage figure to reach 139.70%. That being said, these coverage rates are dependent on the urban mixture of those specific phases. Thus, the next step is to look at the entire project.
By running the same calculation across all ten phases of Al-Rehab City, different results arise in each phase. Throughout the phases, coverage figures range from 79.9% to 140% as expected with the variation in urban mixtures. Phases with the lowest coverage rates do not include single family houses, while phase six which exhibits the highest rate is composed only of houses. The remaining inclusive phases have coverage figures ranging from 83.6% to 106.3%. In total, the ten phases of the project combined have an initial coverage figure of 91.2%. However, with optimization, this can reach 134.2%. The total installed capacity in Al-Rehab City would form 186 MW, which already forms 8% of the PV target set by the Ministry of Electricity in 2014 (Solar in Egypt: New feedin-tariff scheme to develop PV, 2015). Additionally, the project is expected to offer an annual clean energy yield of 326 GWh, which would release 31.1 tonnes in Co2 emissions annually. These emissions are equivalent to 22.3% of emissions if the same amount of
energy is generated from the currently installed local system (United States Environmental Protection Agency, 2016). Therefore, the presented approach would mitigate carbon emissions by 24.3 tonnes annually, forming 607.5 tones by the end of the system’s lifetime.
Finance: Economy is a critical component to this study, particularly given that it targets developers with a sustainable development scheme in the form of a financial investment. Using the figures mentioned in the previous chapter, the total capital needed to install PV panels across Al-Rehab City would require roughly EGP 2.3 billion, with an average of 230
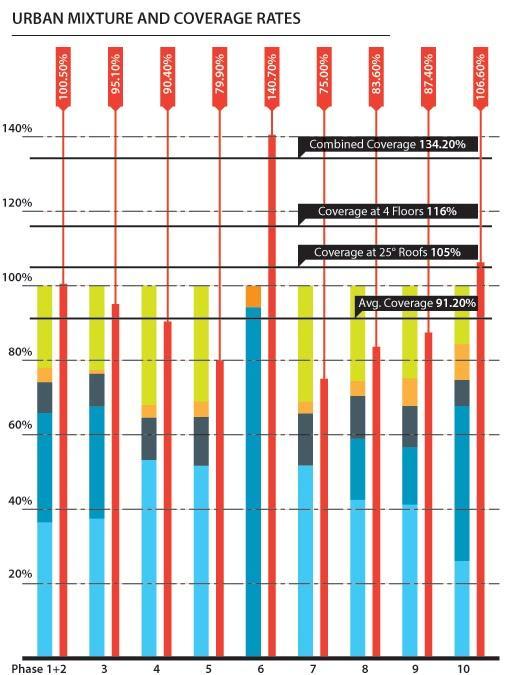
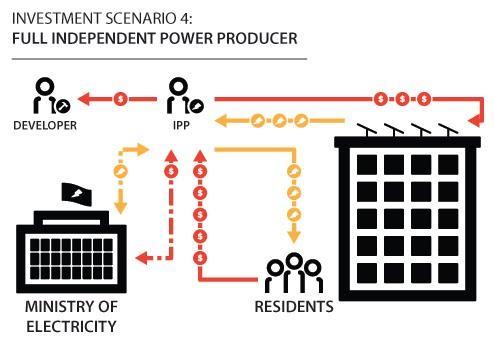
million per phase. According to the current feed-in tariff rates, the expected annual return from selling harvested energy would form EGP 413 million (Solar in Egypt: New feed-in-tariff scheme to develop PV, 2015). This means that the investment would pay back for itself in 5.6 years, leaving 19.4 years of financial profit, assuming the lifespan of the system is 25 years and taking into account negligible maintenance costs. Additionally, after comparing the capital needed with the total construction value of Al-Rehab City, the proposed scheme would only add 4.25% to the original construction costs for the developer. That being said, there are several investment scenarios for both the finance and management of harvested energy which can be applied presenting the developer with a set of options to choose from. The initial investment can be carried by the developer or by an independent power provider (IPP) who would rent installation surfaces from the developer. The harvested energy, on the other hand, can either be sold to the public sector using FiT, or sold to the residents directly. After studying the possible combination of these two scenarios, it is found that the most optimum solution is for an IPP to rent installation surfaces from the developer and sell energy to residents. This will relieve both the developer from financing and managing energy, and the public sector from the financial burden of FiT, while the IPP can adjust electricity tariff to optimize profit.
Recommendations
As seen in Chapter 6, coverage rates vary according to the urban mixture in each phase with three phases passing the 100% mark. Therefore, energy autonomy is achievable through controlling urban mixture and/or utilizing the discussed optimization solutions. Subsequently, a planning policy can be drawn out of this, controlling urban mixtures, heights and roofs, and promoting the installation of PV panels on buildings’ roofs. Phases one and two, and phase ten, have coverage figures of 100.5% and 106% respectively. Both figures barely cover the autonomy mark, which makes them ideal to analyse the specific urban mixture for each of these phases. It is important to note, however, that phases one and two have five-story-high apartment buildings, while phase ten has six story high apartment buildings. Although this mixture would change after applying the optimization solutions mentioned previously, both mixtures can act as initial guidelines to achieve energy autonomy. That being said, it is important to note that the share of apartment buildings in the mixture should ideally not pass the 20-30% range as apartment buildings are the largest consumer in this context. Using this mixture as a guideline, and with contribution from the public sector, particularly the Ministry of Housing, a new nationwide policy can be drawn out to completely neutralize the electricity demand of any new development in the residential sector. This will further reduce carbon emissions, and also help mitigate the national energy crisis. Moreover, a few urban planning guidelines can be established. Firstly, slanting roofs to optimize space efficiency would ideally favor buildings which are orientated along the cardinal directions to avoid a diagonally-slanted surface. This inclination also favors more rectangular buildings with the longer side stretching east-west. In addition, inclining roofs also favors free standing buildings, or buildings attached from the eastern and western sides to avoid stretching the inclined surface over multiple buildings unless this is solved architecturally.
To further optimize the overall performance of the presented scheme, and to introduce residents into the equation, an advanced coverage dependent electricity tariff is proposed, under the label: Coverage Cap Policy. The policy proposes to set a consumption cap that is specific to the building’s energy yield from PV panels. In
this case, the total yield would be divided by the number of tenants, and as long as the tenant consumes under their specific cap, they are charged a reduced tariff, and naturally, an expensive tariff if they consume over the cap. By adopting this policy, residents receive benefits from the installed PV system and are presented with a financial incentive to reduce their individual consumption. This policy can be applied to an individual building, a cluster or an entire housing project, eventually providing tenants with a higher collective cap.
For further findings and potential improvements, as this thesis illustrates, the potential of the private sector to apply sustainable development scenarios, by capitalizing on the same principle, the presented scheme can be stretched to introduce other sustainable solutions like waste management, recycling and urban farming. These additions would offer limitless environmental benefits while they still present the developer with financial profits.
Conclusion
It is more practical and feasible to target the private sector for the reliable large-scale application of sustainable energy solutions rather than depend on individual actions from random individuals. In this case, the private sector is represented by developers, who can easily finance and install photovoltaic panels across entire housing projects. Installing these panels would increase total construction costs by less than 5%, while, in turn, presenting developers with an investment that pays back for itself in 5.6 years, leaving almost 20 years of financial profit from selling harvested energy. Installing photovoltaic panels across a project like Al-Rehab City would initially cover 91% of electricity demand, while if this application is taken into account during the design and construction phase, simple tweaks can be done to increase this coverage figure to reach 134%. Energy harvested from the panels has the potential to mitigate carbon emissions by 77%, while the proposed Coverage Cap Policy can provide residents with attractive incentives to reduce their individual consumption, eventually improving carbon reduction figures. Photovoltaic panels bring the generation of clean energy directly into the residential sector. By capitalizing on this possible autonomy in urban planning, and as a national policy, the residential sector, which is responsible for 40% of global energy consumption and 25% of carbon emissions, can be rendered completely energy autonomous, with very minimal carbon emissions.
References
Cory, K., Couture, T. and Kreycik, C. (2009). Feed-in Tariff Policy: Design, Implementation, and RPS Policy Interactions. [online] Colorado: National Renewable Energy Laboratory. Available at: http://www.nrel.gov/docs/fy09osti/45549.pdf [Accessed 24 May 2016]. Egyptian Electric Utility for Consumer Protection and Regulatory Agency, (2015). Indicator of Electrical Energy Consumption in Economic Activities: Annual Report 2013-2014. [online] Cairo: Egyptian Electric Utility for Consumer Protection and Regulatory Agency, p.21. Available at: http://egyptera.org/Downloads/reports/ [Accessed 21 May 2016]. Electrical Notes & Articles. (2011). Demand Factor-Diversity Factor-Utilization Factor-Load Factor. [online] Available at: https://electricalnotes.wordpress.com/2011/10/31/demand-factor-diversity-factorutilization-factor-load-factor/ [Accessed 6 Jun. 2016]. Egyptian Electricity Holding Company, (2016). Annual Report 2013-2014. [online] Cairo: Egyptian Electricity Holding Company, pp.16, 28. Available at: http://www.moee.gov.eg/english_new/EEHC_Rep/REPEN2013-2014.pdf [Accessed 21 May 2016]. El-Khatam, W. (2013). Current Status and Future Prospects for the Power Market in Egypt
International Energy Agency. (2016). Renewables. [online] Available at: http://www.iea.org/topics/renewables/ [Accessed 22 Jul. 2016]. M. Yousry, A. (n.d.). The Privatization of Urban Development in Cairo: Lessons Learned from the Development Experience of Al Rehab Gated Community. Mæhlum, M. (2013). Grid-Tied, Off-Grid and Hybrid Solar Systems - Energy Informative. [online] Energy Informative. Available at: http://energyinformative.org/grid-tied-off-grid-and-hybrid-solar-systems/ [Accessed 24 May 2016]. SMA Solar Technology AG, (n.d.). Centralized or Decentralized? A (Not So) Simple Decision. [online] Niestetal: SMA Solar Technology A. Available at: https://www.sma.de/fileadmin/content/global/Solutions/Documents/Power-PlantSolutions/WP_CENTRALIZED-DECENTRALIZED_AEN130710W.PDF [Accessed 24 May 2016]. Solar in Egypt: New feed-in-tariff scheme to develop PV. (2015). [online] PV Insider. Available at: http://www.solargcc.com/wp-content/uploads/2014/12/SolarinEgypt.pdf [Accessed 24 May 2016]. United Nations Environment Programme. (2016). United Nations Environment Programme (UNEP) - SBCI. [online] Available at: http://www.unep.org/sbci/AboutSBCI/Background.asp [Accessed 21 May 2016]. United States Environmental Protection Agency. (2016). Greenhouse Gases | Climate Change | US EPA. [online] Available at: https://www3.epa.gov/climatechange/science/indicators/ghg/ [Accessed 21 May 2016]. United States Environmental Protection Agency. (2016). Global Emissions | Climate Change | US EPA. [online] Available at: https://www3.epa.gov/climatechange/ghgemissions/global.html [Accessed 21 May 2016].