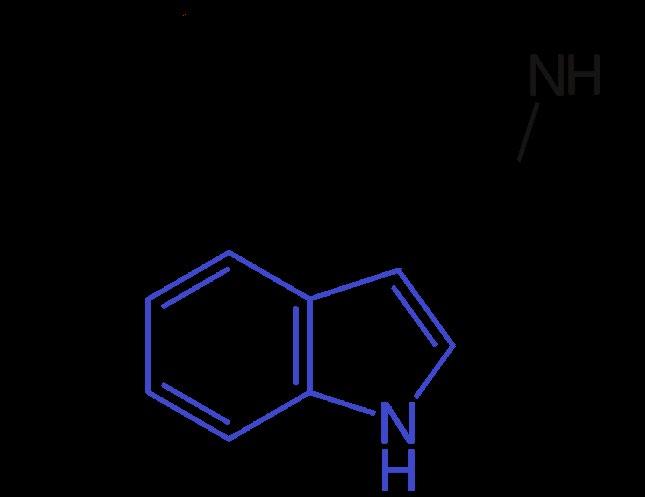
42 minute read
Psychedelic Psychotherapy – How GMOs and Magic Mushrooms Will Solve the Mental Health Crisis
Zachary Barnett
ABSTRACT: Psilocybin and its metabolic derivative, psilocin, are psychoactive molecules that occur naturally in certain mushroom species. Recent research has shown psilocybin to be an effective means of treating several mental illnesses such as anxiety, depression, OCD, and addiction. However, current legislation and the specific environmental requirements for the cultivation of psilocybin-containing mushrooms prevents the mass use of this biopharmaceutical. Fortunately, through the application of bioprocess engineering, both Escherichia coli and Saccharomyces cerevisiae can be genetically modified to produce psilocybin in vivo, which addresses the environmental and financial limitations seen in mushrooms that inhibit mass production. This thesis paper will provide an argument for the cultivation of genetically modified organisms for the mass production of psilocybin for use as an innovative antidepressant based upon reduced production costs, a high degree of chemical safety, and effective treatment rates of various disorders.
Advertisement
Introduction
The United States is undergoing a mental health crisis. Approximately 40 million adults are affected by mental health disorders each year. Anxiety disorders are the most common mental illness in the U.S and impact roughly one-fifth of the population [1]. Mental health has become a sensationalized issue in modern media, with terms like ‘anxiety,’ ‘depression,’ ‘OCD,’ and ‘addiction’ occupying a large degree of space in our headlines. Two studies published by the American Psychiatric Association involving thousands of
children and college students show that “…anxiety has increased substantially since the 1950s”. Anxiety “…has increased so much that typical schoolchildren during the 1980's reported more anxiety than child psychiatric patients did during the 1950s” [2]. Even with multiple antidepressants being available on the market, there seems to be a continuing decline in mental health, with the same researchers suggesting that “…cases of depression will continue to increase in the coming decades, as anxiety tends to predispose people to depression”. As anxiety disorders continue to sink their roots into our society, researchers have turned to an unlikely organism for reprieve: psychoactive mushrooms. Despite their illegality both in the United States and around the world, psychoactive mushrooms that contain the prodrug psilocybin have been shown to have a phenomenally beneficial impact on mental health. This chemical has exhibited a substantially therapeutic effect on depression, anxiety, OCD, addiction, and an increase in general quality of life [3]. However, these fungi are not without issue. Several factors prevent psilocybin-containing mushrooms from revolutionizing how we treat mental health. From their illegality to difficulties with cultivation, these mushrooms themselves seem unlikely to become the next innovative drug production mechanism. Despite these factors, scientists have determined a method to genetically engineer microorganisms to produce the primary psychoactive prodrug, psilocybin, found in magic mushrooms. This approach has been projected to be cheaper and more efficient than other existing options. Thus, the psilocybin produced via genetically modified organisms provides an alternative to organic synthesis, is more effective than conventional antidepressants, and more economical than mushroom cultivation.
Psilocybin
Structure
Psilocybin (4-phosphoryloxy-N, N-dimethyltryptamine) is the primary substituted tryptamine observed in various mushroom species and is unfamiliar to most Americans. All tryptamines possess a similar molecular structure, containing an indole ring joined to an amino group (NH2) via an ethyl side chain (Fig. 1). The term “substituted” refers to the replacement of any of the hydrogen atoms normally attached to the indole ring, sidechain, and/or amino group. Differences in pharmacodynamics occur via the substitution of these functional groups. In psilocybin, the R4 carbon is substituted with a phosphate group, and RN1 and RN2 are substituted with methyl groups (Fig. 2).
Figure 1. Molecular structure of tryptamine.
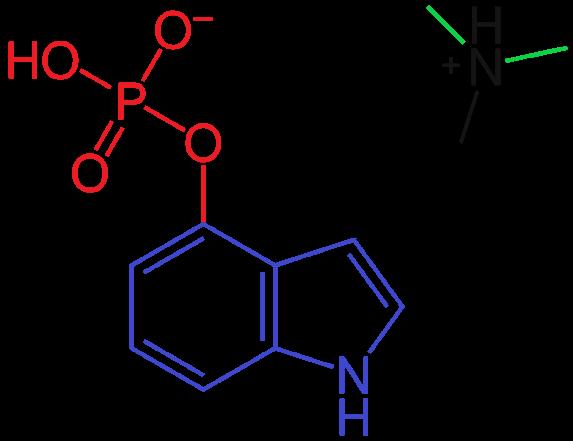
Figure 2. Molecular structure of psilocybin.
Note: The indole ring is shown in blue, ethyl side chain in black, phosphate group in red, and methyl groups in green. Both compounds possess the same backbone (indole ring and ethyl chain connected to an amino group). However, only psilocybin has an additional phosphate group and two methyl groups attached to the amino group. Varied effects occur entirely based on these minute differences.
Psilocybin is a relatively durable molecule, with one study finding “… even after 30 years of storage, detectable quantities of psilocybin were still found [in mushroom samples]” [4]. The longevity of psilocybin is due in part to the phosphate group seen on the R4 carbon. The phosphate group increases the molecule’s stability relative to its metabolites by protecting the molecule from oxidative degradation [5]. Exposure “… to temperature[s] above 70°C leads to psilocybin dephosphorylation, particularly if the extraction is carried out at acidic pH conditions” [6]. Administered orally and metabolized by the human body, psilocybin is rapidly dephosphorylated in the stomach’s acidic environment or through enzymatic processes involving alkaline phosphatases and various nonspecific esterases [7] [8].
Role in Nature
Psilocybin is found on every continent, excluding Antarctica, and across more than twelve mushroom genera, with the compound being found most widely in the species Psilocybe [9]. The exact evolutionary reasoning behind the presence of the compound in these mushrooms is unknown. However, some studies suggest psilocybin acts as a deterrent to insects that might damage the fleshy body of the mushroom. It is believed that psilocybin metabolism in insects yields a neurotransmitter analog that seems to alter the behavior of mycophagous and wood‐eating invertebrates [10]. This same neurotransmitter action is present in humans and is the source of the psychotherapeutic effects of psilocybin ingestion.
Metabolism in Humans
Dephosphorylation of psilocybin yields psilocin as a primary metabolite, with numerous secondary metabolites (Fig 3). Psilocin persists in the body for several hours before the chemical is eliminated via the kidneys through O-glucuronylation and via an enzymatically catalyzed reaction involving monoamine oxidase isozyme A (MAO-A). MAO-A is an enzyme that normally oxidatively deaminates serotonin but is non-selective and therefore acts upon other biogenic and neuroactive amines, such as psilocin [11].

Figure 3. Metabolism of psilocybin. [7]
Note: The structure of tryptamine is indicated in blue, the renal excretion path is in green, and enzymatic metabolism via monoamine oxidases is shown in red.
Following enzymatic metabolism, the primary psychoactive metabolite, psilocin, begins its journey to the brain. Psilocin crosses the blood-brain barrier more readily than psilocybin, where it begins to interact with various systems within the body [5]. Therefore, even though psilocybin is the primary indole alkaloid found in ‘magic mushrooms,’ psilocybin actually acts as a prodrug requiring metabolism to become pharmacologically active. Thus “…whenever a reference is made to the in vivo effects of psilocybin, it is
psilocin that is responsible for [said] effects” [7].
Psilocin
Structure & Activity as a Serotonin Analog:
Psilocin is structurally analogous to 5-hydroxytryptamine, a prominent endogenous neurotransmitter, more commonly known as serotonin (Fig. 4 and Fig. 5). This structural similarity allows psilocin to bind to serotonin (5-HT) receptors in the central nervous system with high affinity. Even though psilocin administration’s physiologic and psychotic effects result from its agonistic activity toward receptors located within the central nervous system, there is no guarantee that equal concentrations of psilocin would produce equal treatment intensity in two different patients. A recent study found one area of the brain to be an accurate predictor of psilocybin experience [12]. Known as the rostral anterior cingulate, increases in the thickness of this region between patients correlated with an increase in scores measuring the experience of unity, spiritual experience, blissful state, and insightfulness. It is hypothesized that due to the density of 5-HT2A receptors in this region of the brain, increased thickness corresponds to increased 5-HT2A receptors, which are activated upon the binding of psilocin.
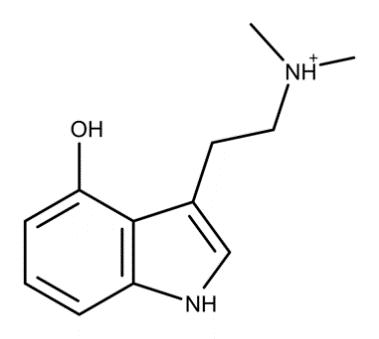
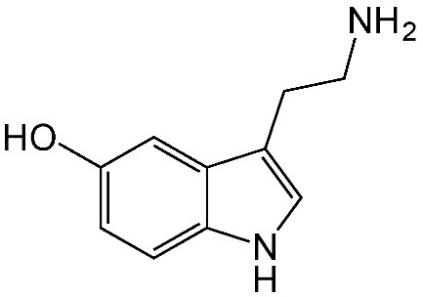
Figure 4. Molecular structure of psilocin Figure 5. Molecular structure of serotonin (5hydroxytryptamine)
Note the remarkable similarity between these two chemicals. One is endogenous, occurring naturally in the human body (right), whereas the other (left) is not. A single carbon atom shift (R4 in psilocin vs. R5 in serotonin) of the hydroxyl group (-OH) and the addition of two methyl groups result in profound changes in functionality.
While psilocin acts primarily as a 5-HT1 and 5-HT2 agonist in the brain, it should be noted that research suggests psilocin partially binds to multiple receptors, with dopamine receptors being just one of those involved [8]. Agonists are chemicals that bind to a given receptor, activating it to produce a biological response [13]. The activity of psilocin in receptors outside of the 5-HT group is supported by the discovery that under the effects of psilocin, “…the frontal cortex [is] metabolically two to three times more stimulated than the occipital cortex, despite…equal 5-HT2 densities” which is “[indicative of] regulatory mechanisms and/or other
neurotransmitter systems [being] implicated” [14]. This suggests that psilocin is far more complex than a simple serotonin analog, and therefore is of far greater pharmacological significance in terms of research and pharmaceutical potential.
Accompanying Compounds and the Entourage Effect:
It should be noted that while psilocybin comprises most of the indole alkaloid content of psychoactive mushrooms, there are numerous other compounds present that are predicted to play a role in the overall therapeutic effect of the fungus. The collective effect of these low-concentration substituted tryptamines and various chemicals can be referred to as the “Entourage Effect” and is a topic of interest for researchers given a lack of knowledge surrounding the phenomenon [15]. The presence of other molecules is lost when pure psilocybin is extracted and used for treatment, leading to a need for the full chemical profile of psilocybincontaining mushrooms to be mapped. This would allow producers to create a pharmaceutical that reaps the comprehensive benefits of this natural source. One such class of molecules present in psilocybin-containing mushrooms is β‐carboline monoamine oxidase inhibitors (MAOIs). “As potent inhibitors of MAOs, MAOIs are neuroactive compounds that interfere with [psilocin] degradation” (see ‘red pathway’ in Fig. 3). Based on the findings of several German researchers (the Hoffmeister group), it is believed that “…MAO inhibitors generally increase the pharmacological effects of such bioactive amines” [11]. More importantly, their study found that the β‐carboline profile of various mushroom species was both “…quantitatively and qualitatively inhomogeneous among species….” This leads to the basis for an argument that different species of mushrooms do indeed produce different effects and are more than the sum of their primary psychoactive compounds. The ability of at least five Psilocybe species to produce β‐carbolines was deemed “remarkable” in the light of synergistic pharmacology (i.e. the “Entourage Effect).
Efficacy of Psilocybin Treatment
Because of its effect upon the serotonergic and related systems, psilocin has been examined from a medicinal point of view as a biopharmaceutical, and results are promising. Psilocin has exhibited potential in treating anxiety and depression, OCD, and addiction to alcohol and cigarettes. The modulatory nature of psilocin upon numerous 5HT receptors responsible for precisely these behaviors explains why this serotonin analog exhibits effectiveness at treating a diverse array of mental health disorders (See Table 1, Appendix).
Anxiety Disorders:
Of particular interest is psilocybin’s ability to act as an antidepressant to treat anxiety disorders. Millions of Americans are affected by anxiety and depression every day. Cancer patients represent a subset of that population, one that experiences particularly acute symptoms of anxiety and depression. Psilocybin has proven especially effective at guaranteeing a higher quality of life for late-stage cancer patients.
One randomized, double-blind, pseudo-placebo controlled study (n=17) by Johns Hopkins University reported that psilocybin administration “…produced large decreases in clinician and self-rated measures of depressed mood and anxiety, along with increases in quality of life, life meaning, and optimism, and decreases in death anxiety. At a 6month follow-up, these changes were sustained with 80% of participants continuing to show clinically significant decreases in depressed mood and anxiety”. All participants attributed their positive changes in mood to the psilocybin treatment [16]. A second double-blind, placebocontrolled pilot study (n=12) also analyzed the effects of psilocybin. The study found “…no clinically significant adverse events…a significant reduction in anxiety….and improvement of mood that reached significance at 6 months…” [17]. A third randomized, doubleblind, placebo-controlled study published by the New York University of Medicine (n=29) further confirms the conclusions that psilocybin produces sustained benefits in reducing anxiety and depression in cancer patients. The study found “…psilocybin produced immediate, substantial, and sustained improvements in anxiety and depression that led to decreases in cancer-related demoralization and hopelessness, improved spiritual wellbeing, and increased quality of life. At the 6.5-month follow up, psilocybin was associated with enduring…antidepressant effects” [18].
Obsessive Compulsive Disorder (OCD):
In addition to the millions of Americans with anxiety disorders, approximately 8.25 million have OCD [19]. “OCD is a chronic and debilitating condition with a lifetime prevalence of 2% to 3%, which makes it the fourth most common psychiatric diagnosis” [20]. Interestingly, not only does psilocybin-treatment display significant effects upon reducing anxiety and depression, but it has also been examined as a potential treatment for OCD. A double-blind study (n=9) investigating the safety, tolerability, and clinical effects of psilocybin administration concluded that “In a controlled clinical environment, psilocybin was safely used in subjects with OCD and was associated with acute reductions in core OCD symptoms in several subjects” [20]. Certain forms of OCD are known to exhibit an inefficient response to current treatments, and thus psilocybin could act as a form of a breakthrough therapy.
Tobacco and Alcohol Addiction:
Due to the agonistic activity of psilocybin upon 5-HT receptors and their connection to addiction, psilocybin treatment has shown promise in treating common forms of substance use disorders such as tobacco and alcohol addiction. An estimated 5 million deaths per year are caused by tobacco use, and those numbers are projected to rise to more than 8 million per year by 2030, according to the WHO [21]. In an open-label pilot study (n=15) by Johns Hopkins University, otherwise healthy nicotine-dependent smokers who had a mean of six previous lifetime quit attempts were subjected to psilocybin therapy with the goal of completely stopping
tobacco use. At a 6-month follow-up, 80% of participants showed seven-day point prevalence abstinence. Eleven of these 12 self-reported quitting smoking and demonstrated biologically verified smoking abstinence throughout the following ten weeks of treatment [21]. Alcohol addiction is equally as debilitating, affecting roughly 12% of the American population. “In a singlegroup, proof-of-concept study (n=10), volunteers with DSM-IV alcohol dependence received... [psilocybin treatment] …Participants’ responses to psilocybin were qualitatively similar to those described in other populations, although some of the participants had a relatively mild response to the doses used. Drinking did not decrease significantly in the first four weeks of treatment (when participants had not yet received psilocybin) but decreased significantly following psilocybin administration.” What’s more, these gains were maintained during a 36week follow-up. Based upon these results, a larger double-blind trial is currently underway [22].
Psilocybin Compared to Current Antidepressants
Based on the volume of research and the efficiency exhibited therein, it can be argued that psilocybin is phenomenally effective at treating anxiety, depression, OCD, and assorted addictions. Psilocybin treatment could prove to be more effective than certain currently available antidepressants and smoking cessation methods. This effect is made more drastic upon the realization that when it comes to prescribing antidepressants, “It’s a best guess out of dozens on the market” [23]. When taking an antidepressant, it requires time to take effect, sometimes on the order of months before symptom relief can be experienced. Furthermore, there is no guarantee that a given antidepressant will produce the desired effect after that duration of time. Even then, antidepressants can produce a variety of undesirable side effects. This means that even if a given antidepressant successfully works to relieve a patient’s symptoms, of which there is no guarantee, it is not certain that there will not beside effects that might force the patient to abandon the antidepressant (even if it works only to start the entire cycle over again). When compared to psilocybin, the differences are astonishing. The effects of psilocybin treatment are immediate and enduring, sometimes lasting for several months at a time following a single round of treatment. Furthermore, psilocybin exhibits little to no serious side effects. Of all the trials that have been conducted up to this point (approximately 2000 subjects having received psilocybin under controlled conditions by 2005), the only theorized at-risk populations are those such as individuals with hypertension, tachycardia, or personality predisposition. It should be noted that the “…prevalence of prolonged psychiatric symptoms after serotonergic hallucinogens in thousands of healthy subjects and psychiatric patients was 0.08%-0.09% and 0.18%, respectively. Attempts to commit suicide occurred in 0.12% of psychiatric patients with 0.04% succeeding” [8]. According to numerous studies, psilocybin has very low toxicity and is not neurotoxic. The ratio between the LD50 (lethal dosage in 50% of a given population) and the ED50 (effective dosage in 50% of a given population),
which serves as an accurate indicator of safety, is 641 for psilocybin (in comparison to 9637 for vitamin A, 4816 for LSD, 199 for aspirin, and 21 for nicotine) [8]. Using the LD50 of rats and mice (LD50 = 280 and 285mg (about the weight of ten grains of rice)/kg respectively) which has been documented to be lower than humans, it is predicted that a 60-kg (~132 lb.) person would need to ingest up to 1.7kg (~3.7 lb.) of fresh mushrooms, or consume “…approximately 19g of the pure drug…” to overdose [7] [8]. Finally, it has been stated that the risk of addiction to hallucinogens, including psilocybin, is very low. In one study, monkeys failed to seek psilocybin as a reward when offered. When human use is observed, psilocybin fails to cause craving or withdrawal, and it does not directly affect the dopaminergic pathway, thereby avoiding the reward system [7] [8]. Even more, due to differences in patient response to psilocybin treatment based upon 5-HT receptor density in the rostral anterior cingulate region, there is a potential for the implementation of patient brain mapping to determine patientdependent dosing. Coupled with the previously discussed entourage effect of various psychoactive alkaloids also present in mushrooms, this would result in the potential for a customtailored, individual-centric approach, yielding targeted symptom relief. The researcher even went as far as to say, “Biomarkers prospectively predicting effective treatment options could assist in individualized treatment planning, reducing time spent on ineffective treatments, shortening patient suffering, and reducing the overall cost burden of depression” [12]. This statement alone represents an almost complete 180° compared to the currently employed treatment method. This revolutionary approach was up until recently, still a pipe dream for psychiatrists who relied upon the best guess to address these often-lifethreatening illnesses. All of these factors have psilocybin poised to be one of the best candidates to revolutionize the subset of the multibillion-dollar pharmaceutical industry pertaining to mental health.
History & Legality of Psilocybin in Society
Psilocybin originally enjoyed legal status during the 1960s after first being identified and isolated by renowned Swiss chemist Albert Hoffman in 1959. For a while, psilocybin was even sold as a licensed pharmaceutical by Sandoz under the name Indocybin (Fig. 6).
Figure 6. Indocybin as marketed by Sandoz
Note: Sandoz is a subsidiary of the pharmaceutical giant Novartis. This should serve as evidence of the previously perceived utility of psilocybin in the eyes of the pharmaceutical industry, which has disappeared following its regulation.
Picture Source: [24]
Psilocybin was originally banned in 1970 by the Comprehensive Drug Abuse Prevention and Control Act, which was passed as a part of the War on Drugs by the United States Government [25]. While psilocybin for entheogenic purposes was allowed by select Native tribes, the general population began to view mushrooms and the chemicals they contained negatively due to misinformation and a lack of exposure. Today, the Substance Abuse and Mental Health Services Administration reported that the knowledge and use of mushrooms are “not at all common” [26]. Therefore, despite the benefits of psilocybin, many Americans remain in the dark regarding its benefits due to current legislation. Despite groundbreaking research that suggests psilocybin possesses obvious therapeutic applications and low addiction potential, it remains classified as a Schedule I substance. Under this classification, psilocybin has no currently accepted medicinal use and a high potential for abuse. This designation is in stark contrast to the current studies available to the public. Thankfully, psilocybin research and anecdotal evidence from prominent experts has brought about a shift in public opinion that has resulted in substantial legislative changes. This legislative shift is akin to the shift seen regarding cannabis, another Schedule I substance. Just as Colorado pioneered cannabis decriminalization and legalization, so too did Denver, CO, become the first city to vote to decriminalize psilocybin-containing mushrooms in May of 2019 [27]. While far from legal, decriminalization is a step in the right direction if psilocybin will ever play a role in treating the millions of Americans affected by anxiety disorders, OCD, addiction, and other mental disorders whose interactions with psilocybin have yet to be studied.
Despite legislation saying otherwise, the FDA has begun to recognize psilocybin for the potential breakthrough compound that it is. A mental health care company by the name of COMPASS Pathways has entered phase 2b dose-ranging trials with 216 patients, earning the FDA’s "Breakthrough Therapy" designation for its program of psilocybin therapy for treatmentresistant depression [28]. COMPASS has even taken the initiative and patented the chemical production process of two specific polymorphs of psilocybin [29]. While it might seem as though COMPASS is laying claim to the projected capital of psilocybin in its entirety, they have only taken the crumbs of a much larger prize. These two different crystalline structures represent a subset of other potential polymorphs and fail to consider the polymorphs of other substituted tryptamines that have yet to be patented. At most, their patent is a guarantee that a small portion of a rapidly emerging multi-billion-dollar industry will be theirs. Instead of stifling innovation, the COMPASS Pathways patent motivates potential pioneers and sparks conversation. Experts in the field believe that psychedelic research will significantly increase within the next five to ten years. A team of Dutch researchers even go as far as to suggest that the recreational use of psychedelics in the United States has remained as high as 20% since the 1960s and therefore shows that psychedelics are alive and well in the Western world despite prohibitionist legislation. They, too, cite marijuana legalization as an indicator of a shift in society’s perception of these mind-altering substances [30]. This further strengthens the case for the eventual acceptance of psilocybin as a pharmaceutical and displays the international recognition it is garnering. Therefore, it would seem as though legality serves as the only reason behind psilocybin’s absence from the pharmacological industry, but this is not the case. Obtaining a means of mass-producing psilocybin remains elusive even outside of regards to the molecule’s legal status as a schedule I substance. As it stands, psilocybin can be synthesized, harvested via mushroom cultivation, or produced in vivo by genetically modified organisms.
Current Routes to Psilocybin Production
Chemical Synthesis (Synthetic)
As it stands, psilocybin is provided for research solely through chemical synthesis, absent of any living organism. However, synthetic psilocybin can cost scientists as much as $7,000 to $10,000 USD per gram [31]. These high prices not only stem from illegality and a lack of market competition but from difficulties in the chemical synthesis itself. Despite being first identified and isolated by Albert Hoffman in the 1960s, chemical psilocybin synthesis yields have only moderately improved over this time. Hoffman achieved final yields of 20%, but the difficulty of the last step requiring the phosphorylation of psilocin to psilocybin challenged Hoffman and modern chemists alike. This is only exaggerated by the difficulty of the stereospecific hydroxylation of the indole aromatic ring, which has been called “one of the most challenging fields in modern
chemical synthesis” [32]. The highest yields of psilocybin from chemical synthesis were reported by COMPASS pathways, with overall yields of 75%. Hence the subsequent patent application. However, this route requires expensive precursors, meaning the high production costs might limit its impact.
Mushroom Cultivation (Biosynthesis)
Mushroom cultivation is an incredibly inefficient means of providing an entire branch of the pharmaceutical industry with a chemical in high demand. Mushrooms are the fungus’ fruiting body, with most of the biomass being found within the given substrate in the form of thin hair-like strands called hyphae that make up a network known as mycelium. The sole purpose of the fruiting body is to produce and disperse spores. Therefore. they possess only trace concentrations (0.2% to 1% dry weight) of psilocybin. making the mushrooms themselves an inefficient method of delivery [8]. This is especially true when considering domestic and international GMPs regarding the sale of a product that is uniform in concentration [8]. Because of mushroom’s nature, once all the available nutrients have been depleted, the fruiting body emerges to spread spores and decays shortly, thereafter, resulting in a brief lifespan and harvest period. Furthermore, mushrooms tend to fare well in moist environments rich in nutrients. Unfortunately, these same conditions are ripe for the proliferation of other decomposer organisms such as mold and bacteria. Without proper sterile procedures, mushroom cultivators are at risk of also farming pathogens such as cobweb mold, black mold, and assorted bacteria. These contaminants either cause various ailments upon consumption, reduce crop yield, or excrete toxic compounds. However, it is these same destructive microorganisms that are proving to be psilocybin’s savior.
Biotechnological Production of Psilocybin
Up until July of 2018, there was little information on recombinantmicroorganism routes to psilocybin production. However, this was changed with the release of a landmark summative paper by the Hoffmeister group [33]. In two studies previously conducted by the same group, psilocybin was first enzymatically synthesized in a four-enzyme, one-pot reaction. Based upon their data, 20% of the supplied 4-hydroxyindole precursor was converted into psilocybin. In a second study, the team pressed further and genetically modified the Aspergillus nidulans mold to biosynthesize enzymes from a single transcription as a polycistron. Polycistrons contain the codons for more than one gene and therefore express more than one protein. This mRNA molecule was subsequently translated into discrete enzymes despite the genetic code being present on a single mRNA molecule. After growth in small-volume shake flasks and lacking further optimization, psilocybin was reported in minor concentrations of 0.1 g/L. Following in the footsteps of the Hoffmeister group, two equally groundbreaking studies emerged in early 2020. The first, from Miami University, was titled “In vivo production of psilocybin in E. coli”
[34]. Utilizing bioprocess engineering methods, E. coli was genetically modified to produce psilocybin in fedbatch bioreactor conditions in concentrations of ~11601160 mg (about the weight of a small paper clip) f psilocybin from 4-hydroxyindole precursor. The second study came up shortly thereafter from the Technical University of Denmark. It was titled “Metabolic Engineering of Saccharomyces cerevisiae for the de novo production of psilocybin and related tryptamine derivatives” [32]. Their study resulted in a strain of yeast capable of producing 627 ± 140 mg (about the weight of five grains of rice)/L of psilocybin and 580 ± 276 mg (about the weight of ten grains of rice)/L of psilocin. The results of both studies lay the groundwork for the biotechnological production of psilocybin in controlled environments for use as a novel pharmaceutical and even provide a starting point for the biotechnological production of similarly constructed tryptamine derivates that may also hold therapeutic relevance.
In vivo production in E. coli
The work completed by the Hoffmeister group was cited as being monumentally influential in the genetic modification of E. coli to produce psilocybin. Utilizing the gene sequences identified by the Hoffmeister group in the Psilocybe cubensis (P. cubensis) mushroom, the team from Miami University was able to encode the requisite enzymes for the biosynthesis pathway from 4hydroxyindole precursor in E. coli. The authors chose E. coli for the preexisting surplus of metabolic knowledge and genetic tools available to assist in the organism’s genetic engineering. The production of psilocybin was optimized via genetic testing, followed by the optimization of fermentation conditions. Their work has resulted in the highest psilocybin titer reported to date from a genetically modified host. To biosynthesize psilocybin in E. coli, a total of four enzymes are required. The first is an enzyme known as TrpAB and occurs naturally in E. coli. The following three enzymes are xenogeneic, originating from the genome of P. cubensis. They are denoted as PsiD, PsiK, and PsiM (see Fig. 7). The three foreign enzymes were expressed in E. coli via the T7-lac inducible promoter system. Upon absorbing the vector (plasmid), E. coli is genetically modified, such that the addition of isopropyl β-d-1thiogalactopyranoside (IPTG) induces the expression of the T7-lac operon. IPTG is similar in structure to lactose, the chemical that induces lac operon expression in unmodified E. coli. However, unlike lactose, IPTG cannot be broken down by the bacteria, which leads to constant gene expression. Therefore, induction with IPTG causes the expression of the genes immediately following the lac operon. In this case, these genes are related to the expression of PsiD, Psi M, and PsiK.
TrpAB is an E. coli native tryptophan synthase that catalyzes the final two steps of tryptophan biosynthesis in plants, fungi, and bacteria [35]. In unmodified E. coli, TrpAB cleaves 1-(indole-3-yl)glycerol phosphate in the first half of the reaction via the α-subunit (the “A” in TrpAB) to release indole. This indole is subsequently used by the β-subunit (the “B” in TrpAB) to condense indole into tryptophan [33]. However, given
that 4-hydroxyindole (see 1 in Fig. 7) is supplied, only the final step of the biosynthesis is required, allowing for just TrpB to be used. TrpB, therefore, performs the conversion of 4hydroxyindole into 4-hydroxy-Ltryptophan (see 2 in Fig. 7). This synthesis is completed via the affixation of serine (C3H7NO3) to the R2 position of 4-hydroxyindole. PsiD is a decarboxylase, an enzyme that acts to remove carboxyl groups. PsiD normally catalyzes the decarboxylation of L-tryptophan into tryptamine in mushrooms [33]. However, in E. coli, PsiD’s nonselective action allows it to readily convert 4-hydroxy-L-tryptophan to 4hydroxytryptamine (see 3 in Fig. 7). PsiK is a kinase, an enzyme that catalyzes the transfer of phosphate groups from donor molecules. In the E. coli pathway, PsiK catalyzes that phospho-transfer step, dephosphorylating adenosine triphosphate (ATP) into adenosine diphosphate (ADP). The removal of this phosphate group allows for the conversion of 4-hydroxytryptamine into norbaeocystin (see 4 in Fig. 7). PsiM is a methyltransferase, an enzyme that moves methyl groups to a given location within a molecule. In the E. coli pathway, PsiM first converts norbaeocystin into baeocystin (see 5 in Fig. 7) and is utilized again to finally convert baeocystin into psilocybin (see 6 in Fig. 7). The repetition of the methyltransferase step makes sense when considering the presence of the two methyl groups found in psilocybin’s structure (Fig. 2). It should be noted that the “…specificity of PsiM is remarkable,” as PsiM seems to require a 4-O-phosphoryloxy group (read: phosphate group in the R4 position) for proper substrate recognition [33]. This has been further supported in subsequent studies [32].
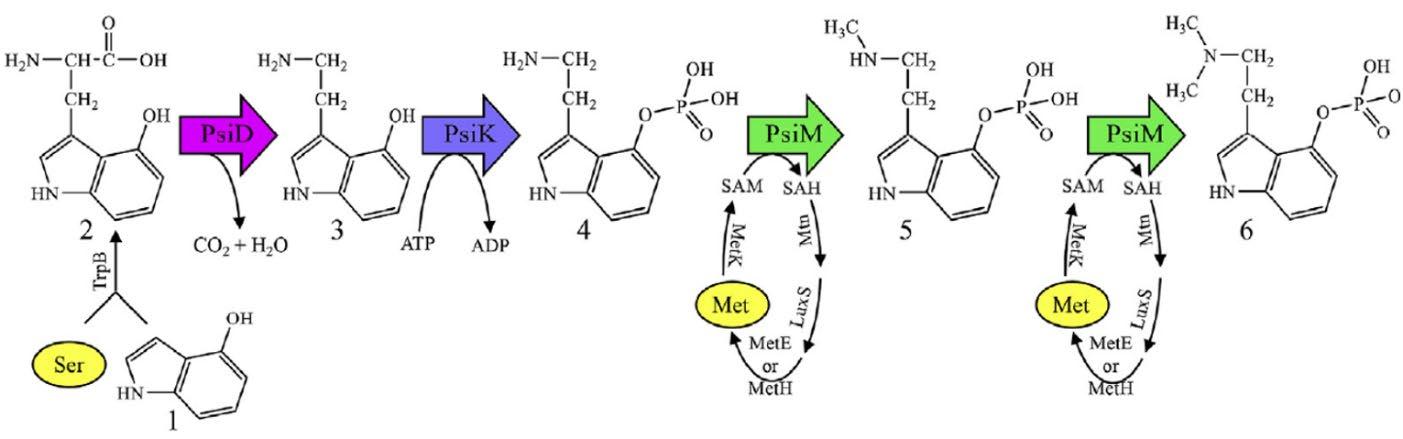
Fig 7. Biosynthetic pathway to psilocybin in E. coli recombinant host
Note: This pathway consists of three heterologous enzymes, PsiD, PsiK, PsiM, with amino acid supplements highlighted in yellow in the form of serine and methionine. [34]
A defined 27-member copy number library was created consisting of the three P. cubensis enzymes (Psi D, Psi K, and Psi M). Each enzyme was applied to a low-copy number (pACM4-SDM2x), medium-copy
number (pCDM4-SDM2x), and highcopy number (pETM6-SDM2x) plasmids. By modifying the copy number, enzymes are expressed in low, medium, or high amounts corresponding to their low, medium, or high designation. At the conclusion of this phase of the optimization study, minor improvements over the “all high” copy number construct (2.19 ± 0.02 mg/L) of 4.0 ± 0.2 mg/L were observed (Fig. 8). One might argue that the high expression of each enzyme may increase the metabolic burden upon the organism by diverting necessary resources away from the production of psilocybin and towards the manufacture of enzymes. This would contribute to the increased efficiency seen in a medium, high, and low expression compared to an allhigh expression.
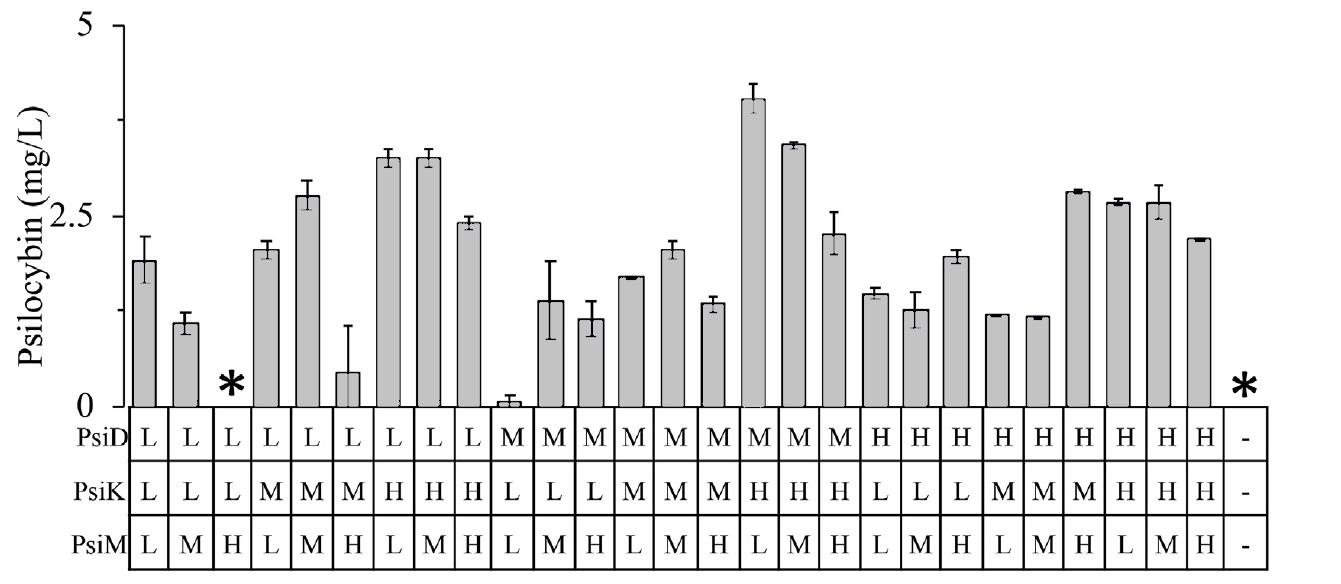
Fig 8. Bar graph of the defined copy number library psilocybin titers
Note: Asterisks (*) denote the absence of detected psilocybin.
A pseudooperon library was then created, consisting of 125 members. This pseudooperon library had different mutant T7 promoters in front of each of the three enzymes encoding for PsiD, PsiK, and PsiM. A single terminator is present at the end of the operon. By using this configuration, researchers were able to cultivate an extremely variable transcriptional landscape. This method allowed for each T7 promoter to transcribe a unique mRNA molecule capable of encoding for the translation of one, two, or all three of the enzymes. After screening a total of 231 random colonies, most variants demonstrated little (30%) to no production (65%) of psilocybin production. A small population (5%) of mutants exhibited massive increases in production relative to the defined copy number library screening (Fig. 9).
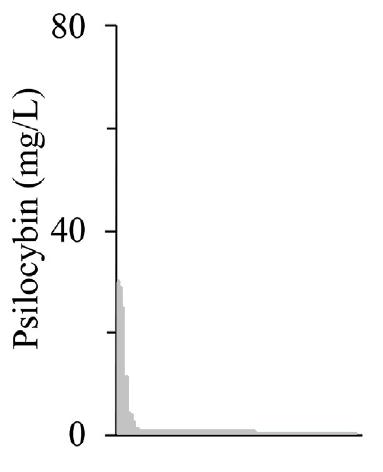
Fig 9. Pseudooperon library screening
Note: When compared to Fig. 8, a select few variants were able to synthesize psilocybin at approximately more than 5x that which was previously observed.
Finally, a three-gene pathway was expressed from a single high-copy plasmid under the control of a single T7 promoter and terminator, where each gene had an identical ribosomal binding site. The T7 promoter sequences were randomized to one of five mutant promoters. This resulted in a library with five potential promoter combinations. Significant enhancement of library performance was seen relative to the pseudo-operon library (Fig. 10). After screening, the top ten variants were re-cloned and transformed to eliminate the chance of plasmid or strain mutations and confirm that psilocybin production was indeed intentional. Variants #13 and #15 were deemed false positives due to a significant reduction in psilocybin production; however, variant #16 was selected for further optimization based upon its yield (Fig. 11). The top mutants from the basic operon screen display a 17-fold improvement in titer over those in the defined copy number library study.
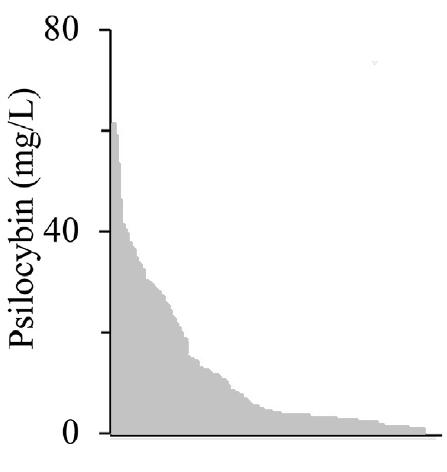

Fig 10. Basic operon library screening and reported psilocybin titers Fig 11. Additional screening of top mutants and reported psilocybin titers
Note: The highest titers (red) were reported for pPsilo16 (#16) as shown.
To further maximize psilocybin yield, fermentation conditions were optimized as well. Subsequent tests analyzed the impact of induction point, base media selection, carbon source identity, inducer concentration, and production temperature. Since these variables can all independently affect cellular growth and/or final product titers, each of them was evaluated according to a range relative to standard E. coli growth conditions. The time at which the T7 promoter system in E. coli is induced has been shown to have a significant impact on cell growth. However, based upon an evaluation of induction point sensitivity for pPsilo16, there seemed to be low sensitivity to induction point with a preference of induction at 3-4h after inoculation. The cellular growth rate was also examined in accordance with base media selection and carbon source identity. Based on data collected, pPsilo16 seemed to be very sensitive to these two variables. “When production was attempted in…undefined media such as LB, a dark-colored insoluble product was observed along with low psilocybin production. Similarly, low production was also observed when grown on glycerol; however, no colored products were observed. pPsilo16 demonstrated moderate sensitivity to IPTG concentration, with higher final concentrations of 0.5- and 1.0-mM outperforming 0.1 mM…” [34]. Production temperatures of 30, 37, 40, and 42 °C were evaluated for their effect upon psilocybin production. All tests were initiated at 37 °C, and a significant preference was seen for maintaining an isothermal fermentation temperature of 37 °C. Furthermore, varying concentrations of supplemental reactants were provided in low,
medium, and high concentrations. The amino acids required for biosynthesis (serine and methionine) were tested at 0, 1, and 5 g/L. Supplementation of methionine showed a significant increase in psilocybin production and ultimately high supplementation of amino acids (5 g/L) were deemed optimal. The 4-hydroxyindole precursor was provided at 150, 350, and 500 mg (about half the weight of a small paper clip)/L. At high concentrations of 4-hydroxyindole, cells demonstrated a noticeable growth decline, which was attributed to presumed cellular toxicity. For the final scale-up study that used all the optimization data collected, a bioreactor was filled with Andrew’s Magic Media (AMM), supplemented with 150 mg (about the weight of five grains of rice)/L 4hydroxyindole, 5 g/L of serine, and 5 g/L of methionine. Temperature was maintained at 37°C and pH was held at 6.5 via the automatic and periodic addition of 10 M NaOH. Dissolved oxygen was held at 20% saturation through impeller agitation that varied between 250 and 1000 rpm. The bioreactor was induced with 1 mM IPTG 4h post-inoculation. After the starting 20 g/L of glucose was metabolized, separate feed streams of 500 g (about twice the weight of a can of soup)/L glucose and 90 g/L (NH4)2HPO4 were supplied. At 12h post-inoculation, 4-hydroxyindole precursor was added according to the observed buildup of the 4hydroxytryptophan pathway intermediate. This study highlights the power of genetic and fermentation optimization in tandem to rapidly clarify the key parameters required to successfully scale-up recombinant E. coli. This study resulted in the production of approximately 1.16 g/L of psilocybin, which represents a cumulative 528-fold improvement over the original unoptimized construct. Despite the attractiveness of these yields, 4-hydroxyindole remains an expensive precursor costing upwards of 288 USD/g [32]. This makes it difficult to compete with the price of chemical synthesis that utilizes the same precursor. Researchers from the Technical University of Denmark recently added their voice to the debate, further reducing the cost of biosynthesis by using S. cerevisiae to circumvent 4-hydroxyindole supplementation.
In vivo production in S. cerevisiae
Despite the increase in production seen in E. coli, S. cerevisiae may arguably be a better candidate for the mass production of psilocybin. S. cerevisiae, more commonly known as yeast, is a eukaryotic organism. It, therefore, possesses a nucleus, whereas the prokaryotic E. coli does not. This difference allows for a minute change: the addition of the PsiH enzyme to the biosynthetic equation. PsiH is a P450 monooxygenase, an enzyme that selectively hydroxylates (applies a hydroxyl group, i.e. an -OH) the R4 position of tryptamine to produce 4hydroxytryptamine. This enzyme requires a compatible P450 reductase enzyme as a redox partner in order to supply the PsiH enzyme with electrons [33]. E. coli, being prokaryotic, is unable to express the family of cytochrome P450 enzymes, hence the absence of PsiH in the previously mentioned biosynthesis. Therefore, “although E. coli can produce serine
and indole naturally, it lacks the ability to express the P450 hydroxylase…” and is unable to avoid 4-hydroxyindole supplementation [34]. S. cerevisiae does not share this problem, and therefore its use further reduces the cost of psilocybin biosynthesis. To begin, fermentation was carried out in a small-scale study at 30°C and pH was maintained at 5.0 via the addition of a 12% NH4OH solution. Upon observing an increase in O2 production and a decrease in CO2 production (indicating consumption of glucose), the fed-batch portion of the experiment was begun. The initial feed rate was 0.45 g/h and was exponentially increased by 0.02 h-1 to accommodate exponential yeast growth. The feed media was defined, consisting of “…30 g/L KH2PO4, 6.3 g/L MgSO4·7H2O, 50 g/L (NH4)2SO4, 0.35 g/L Na2SO4, 500 g (about twice the weight of a can of soup)/L glucose, 1 mL/L antifoam (Antifoam 204) and a 10-fold higher vitamin and trace element concentration”. Researchers discovered that higher psilocybin titers were observed when using Catharanthus roseus (C. roseus) tryptophan decarboxylase (as opposed to P. cubensis decarboxylase, i.e. PsiD). Original testing also showed a large accumulation of tryptamine, “…indicating a significant limitation in the conversion…[to] 4hydroxytryptamine. This is associated with PsiH, the enzyme that hydroxylates tryptamine. “While the detection of psilocybin indicated that the native S. cerevisiae P450 reductase enzyme could carry out this reduction…. [accumulation] suggested a sub-optimal interaction between…” the P450 reductase and PsiH. A substitute P. cubensis reductase was identified using genomic search techniques that resulted in the identification of a single proteincoding sequence with 42.2% homology to the original amino acid sequence. The expression of this P. cubensis reductase produced a significant increase in psilocybin titer. Not only did this exhibit the first functional expression of a P450 reductase from P. cubensis, but it also demonstrated how the expression of an adequate P450 reductase/monooxygenase pair was imperative to maximizing yields. This optimal S. cerevisiae strain (ST9482) was subjected to fed-batch bioreactor cultivation, which allowed for greater control over variables such as pH, oxygen supply, aeration, and glucose concentration, which resulted in even greater psilocybin titers. “The controlled fed-batch fermentation of ST9482 led to the production of 627 ± 140 mg (about the weight of five grains of rice)/L of psilocybin and 580 ± 276 mg (about the weight of ten grains of rice)/L of psilocin”. Interestingly, the controlled feeding of glucose at low rates prevented significant accumulation of ethanol, the byproduct of yeast fermentation that ultimately kills the microorganism in high concentrations.
Biotechnological Summary
With the impeding approval of psilocybin as a breakthrough pharmaceutical, the stable manufacture and supply of this chemical must be considered. “Psilocybin preparations for pharmaceutical and research use currently rely on chemical synthesis via a difficult and expensive process…,” leaving much to be wanted in terms of a reliable supply that will equal demand. The stereoselective introduction of hydroxyl groups has
been described as “one of the most challenging fields in modern chemical synthesis” [32]. The biotechnological route to psilocybin avoids this difficulty through the beauty of previously evolved genes, the tried and true iterative genetic modification of organisms over millennia.
Conclusion
A stigma-laden view of psilocybin-containing mushrooms as street drugs that threaten communities has gripped the nation. The legal scheduling of psilocybin and its metabolic derivatives as Schedule I controlled substances with no medicinal value has grossly impeded psilocybin research and stifled entrepreneurial innovation. Current legislation has wrongly relegated psilocybin to the role of a controlled substance despite trials showing lasting impacts upon anxiety, depression, and various forms of addiction. Low toxicity, high LD50/ED50, and low addiction potential further strengthen the portfolio of traits the substituted tryptamine possesses. Psilocybin has a high degree of utility as a novel antidepressant, which is well-timed given the current mental health crisis in the United States. Until recently, primary sources of psilocybin originated from inorganic synthesis or collection of psilocybin-rich mushrooms. However, illegality, specific cultivation requirements, and difficult organic synthesis make mass production of the chemical difficult. Thankfully, biotechnological methods allow for the genetic engineering of E. coli and S. cerevisiae, workhorses of the biotechnological industry. Commonly occurring E. coli bacteria were manipulated to produce psilocybin at high concentrations, the first example of this molecule being produced by a prokaryotic organism. Despite massively progressing the path to commercial production, the biosynthesis of psilocybin in E. coli utilizes many of the same reagents as chemical synthesis. Standing on the shoulders of giants, further research has shown the potential of S. cerevisiae to yield comparably high concentrations of psilocybin while avoiding more expensive precursors used via the more efficient E. coli method. These studies verify the proof-of-concept for industrial-scale production, a key step if one hopes to see the revival of pharmaceuticals such as IndocybinTM that were arguably foregone far too early. As many experts and their writings suggest, psilocybin as a novel antidepressant can be expected to mushroom into a multibillion-dollar industry in under a decade, bringing with it a revolution in how society views psychotropic substances.
Appendix:
Table 1. List of psilocin-binding receptors and associated functions that are modulated by activation [36]
Receptor Function
5-HT2B • Anxiety • Appetite • Cardiovascular Function • GI Motility • Sleep • Vasoconstriction
5-HT1D
D1 * [37] • Anxiety • Auto receptor • Locomotion • Vasoconstriction
• Development and maintenance of addiction
5-HT1E
5-HT5A
5-HT7
5-HT6 • Auto receptor • Locomotion • Sleep
• Anxiety • Auto receptor • Memory • Mood • Respiration • Sleep • Thermoregulation • Vasoconstriction
• Anxiety • Cognition • Learning • Memory
D3* [38]
5-HT2C
5-HT1B
5-HT2A • Mood
• Antidepressant
• Addiction • Anxiety • Appetite • GI Motility • Heteroreceptor for norepinephrine and dopamine • Locomotion • Mood • Penile Erection • Sexual Behavior • Sleep • Thermoregulation • Vasoconstriction
• Addiction • Aggression • Anxiety • Autoreceptor Learning • Locomotion • Memory • Mood • Penile Erection • Sexual Behavior • Vasoconstriction
• Addiction • Anxiety • Appetite • Cognition • Imagination • Learning • Memory • Mood • Perception
*receptors are associated with dopaminergic system • Sexual Behavior • Sleep • Thermoregulation • Vasoconstriction
References
[1] "NIMH » Mental Illness," National Institute of Mental Health, [Online]. Available: https://www.nimh.nih.gov/health/statistics/mental-illness.shtml. [Accessed 16 January 2020]. Source [2] "Studies Show Normal Children Today Report More Anxiety than Child Psychiatric Patients in the 1950's," [Online]. Available: https://www.apa.org/news/press/releases/2000/12/anxiety. [Accessed 30 January 2020]. [3] F. L. O. J. A. S. C. J. R. A. W. Z. J. E. C. H. Rafael G. dos Santos, "Antidepressive, anxiolytic, and antiaddictive effects of ayahuasca, psilocybin, and lysergic acid diethylamide (LSD): a systematic review of clinical trials published in the last 25 years," Therapeutic Advances in Psychopharmacology, vol. 6, no. 3, pp. 193-213, 2016. [4] A. a. R. K. Christiansen, "Analysis of indole alkaloids in Norwegian Psilocybe semilanceata using high-performance liquid chromatography and mass spectrometry," Journal of Chromatography A, no. 244(2), pp. 357-364, 1982. [5] L. J. W. A. Horita, "The Enzymic Dephosphorylation and Oxidation of Psilocybin and Psilocin by Mammalian Tissue Homogenates," Biochemical Pharmacology, vol. 7, pp. 47-54, 1961. [6] K. Stebelska, "Fungal Hallucinogens Psilocin, Ibotenic Acid, and Muscimol," Therapeutic Drug Monitoring, vol. 35, no. 4, pp. 420-442, 2013. [7] R. J. Dinis-Oliveira, "Metabolism of psilocybin and psilocin: clinical and forensic toxicological relevance," Drug Metabolism Reviews, pp. 84-91, 2017. [8] T. P. J. H. Filip Tyls, "Psilocybin - Summary of knowledge and new perspectives," European Neuropsychopharmacology, no. 24, pp. 342-356, 2014. [9] "Psilocybin mushroom," Wikipedia, 9 March 2020. [Online]. Available: https://en.wikipedia.org/wiki/Psilocybin_mushroom. [Accessed 6 April 2020]. [10] V. V. E. G.-T. H. K. P. M. a. J. S. H. Reynolds, "Horizontal gene cluster transfer increased hallucinogenic mushroom diversity," Evolution Letters, vol. 2, no. 2, pp. 88-101, 2018. [11] S. D. J. F. F. B. F. T. A. K. F. M. C. H. D. H. Felix Blei, "Simultaneous Production of Psilocybin and a Cocktail of β-Carboline Monoamine Oxidase Inhibitors in "Magic" Mushrooms," Chemistry: A European Journal, vol. 26, no. 3, pp. 729734, 2020. [12] K. H. P. B. B. B. C. R. F. X. V. Candace R. Lewis, "Rostral Anterior Cingulate Thickness Predicts the Emotional Psilocybin Experience," Biomedicines, vol. 2, no. 8, 2020. [13] "Agonist," The Free Dictionary, [Online]. Available: https://medicaldictionary.thefreedictionary.com/agonist. [Accessed 9 April 2020].
[14] K. L. L. C. S. P. M. O. S. J. A. F. X. Vollenweider, "Positron Emission Tomography and Fluorodeoxyglucose Studies of Metabolic Hyperfrontality and Psychopathology in the Psilocybin Model of Psychosis," Neuropsychopharmacology, vol. 16, no. 5, pp. 357-372, 1997. [15] "Entourage Effect," Wikipedia, [Online]. Available: https://en.wikipedia.org/wiki/Entourage_effect. [Accessed 19 March 2020]. [16] M. W. J. M. A. C. A. U. W. A. R. B. D. R. M. P. C. M. A. K. Roland R Griffiths, "Psilocybin produces substantial and sustained decreases in depression and anxiety in patients with life-threatening cancer: A randomized double-blind trial," Journal of Psychopharmacology, vol. 30, no. 12, pp. 1181-1197, 2016. [17] M. Charles S. Grob, M. Alicia L. Danforth, M. Gurpreet S. Chopra and R. B. M. Marycie Hagerty, "Pilot Study of Psilocybin Treatment for Anxiety in Patients With Advanced-Stage Cancer," Arch Gen Psychiatry, vol. 68, no. 1, pp. 71-78, 2010. [18] A. B. J. G. G. A.-L. T. M. B. C. S. E. M. A. B. K. K. J. B. Z. S. P. C. a. B. L. S. Stephen Ross, "Rapid and sustained symptom reduction following psilocybin treatment for anxiety and depression in patients with life-threatening cancer: a randomized controlled trial," Journal of Psychopharmacology, vol. 30, no. 12, pp. 1165-1180, 2016. [19] "Facts about Obsessive Compulsive Disorder," BeyondOCD, [Online]. Available: https://beyondocd.org/ocd-facts. [Accessed 19 February 2020]. [20] C. B. W. E. K. T. P. L. D. Francisco A. Moreno, "Safety, Tolerability, and Efficacy of Psilocybin in 9 Patients With Obsessive-Compulsive Disorder," Journal of Clinical Psychiatry, vol. 67, no. 11, pp. 1735-1740, 2006. [21] A. G.-R. M. P. C. R. R. G. Matthew W Johnson, "Pilot study of the 5-HT2AR agonist psilocybin in the treatment of tobacco addiction," Journal of Psychopharmacology, vol. 28, no. 11, pp. 983-992, 2014. [22] M. W. J. Michael P. Bogenschutz, "Classic hallucinogens in the treatment of addictions," Progress in Neuro-Psychopharmacology & Biological Psychiatry, vol. 64, pp. 250-258, 2016. [23] "First Top 10 List for Antidepressants," ABC, [Online]. Available: https://abcnews.go.com/Health/MindMoodNews/story?id=67523 17&page=1. [Accessed 29 February 2020]. [24] "Sandoz Indocybin (psilocybin)," [Online]. Available: http://herbmuseum.ca/content/sandoz-indocybin-psilocybin. [Accessed 18 March 2020]. [25] "Comprehensive Drug Abuse Prevention and Control Act of 1970," Wikipedia, [Online]. Available: https://en.wikipedia.org/wiki/Comprehensive_Drug_Abuse_Prev ention_and_Control_Act_of_1970. [Accessed 18 March 2020]. [26] "Psilocybin Mushroom Fact Sheet," Drug Policy Alliance, 2017. [Online]. Available: https://www.drugpolicy.org/sites/default/files/Psilocybin_Mushr ooms_Fact_Sheet.pdf. [Accessed 19 February 2020].
[27] "Denver Votes to Decriminalize Magic Mushrooms," [Online]. Available: https://www.citylab.com/life/2019/05/psilocybin-mushroomshallucinogens-medical-use-denver-ballot/587680/. [Accessed 18 March 2020]. [28] "COMPASS Pathways Granted Patent Covering Use of Its Psilocybin Formulation in Addressing Treatment-resistant Depression," PR Newswire, [Online]. Available: https://www.prnewswire.com/news-releases/compasspathways-granted-patent-covering-use-of-its-psilocybin-formulation-inaddressing-treatment-resistant-depression-300985534.html. [Accessed 19 March 2020]. [29] C. B. J. S. N. G. M. H. K. P. Derek John Londesbrough, "Preparation of psilocybin, different polymorphic forms, intermediates, formulations and their use". United States of America Patent 2019/0119310, 9 10 2017. [30] A. F. A. S. M. M. V. J. R. H. Bas T.H. de Veen, "Psilocybin for treating substance use disorders?," Expert Review of Neurotherapeutics, vol. 17, no. 2, pp. 203212, 2017. [31] "Cost of Psilocybin," [Online]. Available: https://psilocybintechnology.com/cost-of-psilocybin/. [Accessed 11 July 2020]. [32] P. T. N. M. K. P. R. M. K. I. B. N. Milne, "Metabolic engineering of Saccharomyces cerevisiae for the de novo production of psilocybin and related tryptamine derivatives," Metabolic Engineering, vol. 60, pp. 25-36, 2019. [33] C. L. J. W. F. B. D. H. Janis Fricke, "Production Options for Psilocybin: Making of the Magic," Chemistry: A European Journal, vol. 25, no. 4, pp. 897-903, 2019. [34] N. A. K. Z. W. J. D. B. C. S. M. A. L. E. T. A. R. J. A. J. Alexandra M. Adams, "In vivo production of psilocybin in E. coli," Metabolic Engineering, vol. 56, pp. 111-119, 2019. [35] K. M. e. al., "Conservation of the structure and function of bacterial tryptophan synthases," International Union of Crystallography Journal, vol. 6, no. 4, pp. 649-664, 2019. [36] "5-HT receptor," Wikipedia, [Online]. Available: https://en.wikipedia.org/wiki/5-HT_receptor. [Accessed 13 February 2020]. [37] "Dopamine receptor D1," Wikipedia, [Online]. Available: https://en.wikipedia.org/wiki/Dopamine_receptor_D1. [Accessed 13 February 2020]. [38] "Dopamine receptor D3," Wikipedia, [Online]. Available: https://en.wikipedia.org/wiki/Dopamine_receptor_D3. [Accessed 13 February 2020].