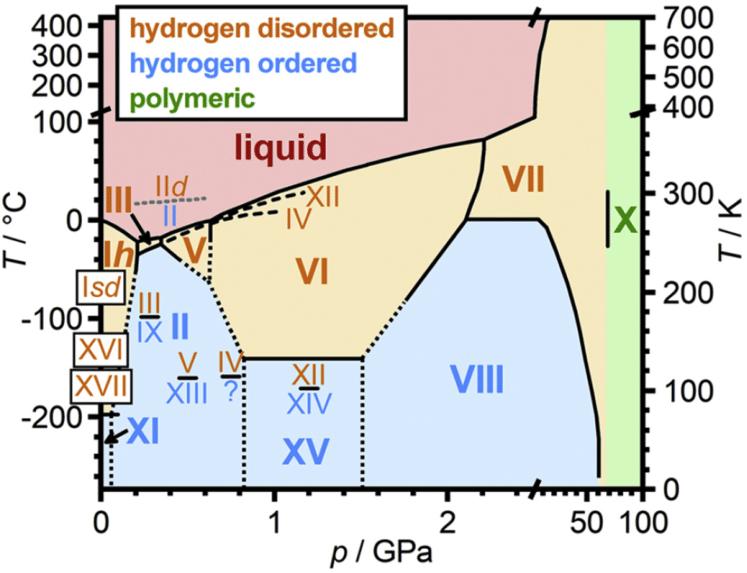
5 minute read
Ice Variants: How Pressure and Temperature Alter the Crystalline Structure of Water
CHEMISTRY
Ice Variants: How Pressure and Temperature Alter the Crystalline Structure of Water
Advertisement
Elizabeth Dean
It is a much beloved factoid that water expands upon freezing. Indeed ‘normal’ ice on earth has a volume about 9% greater than that of liquid water [1] and the secret to the low density of ice lies in its crystalline structure. Further, this crystalline structure is controlled by the pressure and temperature of the surrounding environment, which produce different types of ices. To date, research has identified and produced 19 different ice polymorphs [2]. Indeed, we can be confident that water’s bizarre freezing behaviour is responsible for the nature of our world today; if the ice that formed at earth’s pressure and temperature conditions were denser it would sink, clogging bodies of water into solid blocks of ice [1].
Crystalline structures of ice on earth
Ice I molecular structure On earth, the dominant ice polymorph which constitutes everything from arctic icebergs to the ice cubes in soft drinks is the arbitrarily named ’Ice I’ [5]. Ice I is therefore produced at atmospheric pressure and around 0 degrees Celsius. When the ice is freezing in these conditions, strangely the H20 molecules do not stop moving as whole units. Instead, intermolecular interactions lead to the oxygen atoms forming a hexagonal crystalline structure whilst the hydrogen atoms are randomly oriented [4]. This open hexagonal structure with such large gaps between the atoms is what causes ice to be lower density than water [6]. In Ice I, each oxygen atom is loosely surrounded by 4 hydrogen atoms. 2 are covalently bonded to the oxygen, and two form a hydrogen bond with the oxygen atom’s unshared electron pairs [2].
Ice variants; crystalline structure and hydrogen ordering
Under different pressure and temperature conditions, ice polymorphs will form a dazzling array of different crystalline structures. The behaviour of hydrogen atoms in ices also varies. An ice phase diagram, shown below, demonstrates the pressure and temperature rates across which different ice polymorphs exist. Indeed, Ice 1 –despite its ubiquity on earth-is only rarely found in space.
Ices are also described as being either ‘hydrogen ordered’ or ‘hydrogen disordered’, depending on the behaviour of their hydrogen atoms. Returning to Ice I, the hydrogen atoms do not participate in the crystalline structure and are randomly oriented around their oxygen. This kind of ‘disordered’ ice can deform under pressure, hence flowing glaciers of Ice I. By contrast ‘hydrogen ordered’ ices have hydrogen atoms in crystalline structures as well, with the effect that ices are more brittle [5].
An ice phase diagram for ices I-XVII
CHEMISTRY
Matching up some ice phases to their crystalline structures.
Ice II and IV = rhombohedral
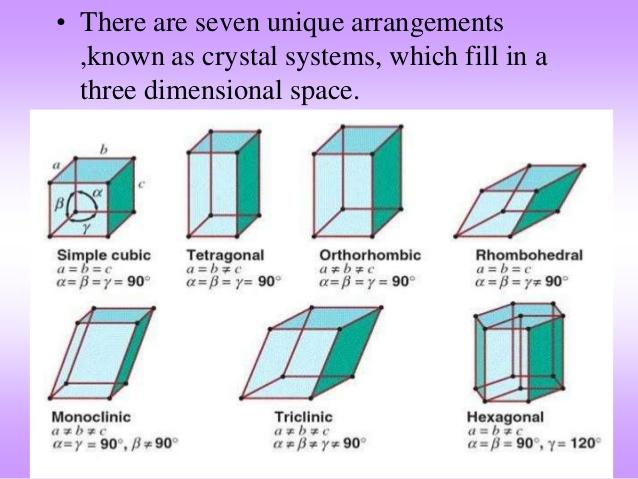
Ice III, VI, IX, XII, XV, XIC = tetragonal
Ice V, XIII = monoclinic

Ice VII and VIII =cubic
Ice IX and XIV = orthorhombic [3]
Investigating ice polymorphs and their structures
Many different ice polymorphs can be formed in the lab by either by cooling water under high pressure or by applying pressure and cooling to an earlier ice polymorph [11]. A good example is Ice VI which is a hydrogen disordered ice polymorph. Ice VI has two ordered forms –Ice XV and Ice XIX- both of which are hydrogenordered ices, each with their hydrogen atoms aligned in completely different patterns [5]. Ice XV was first produced at Innsbruck university about 10 years ago [7], more recently Ice XIX was first made in a lab at Innsbruck University 3 years ago. To achieve this, the team slowed the cooling process for Ice XV, reduced the temperature to about –200 degrees Celsius and increased the pressure to 2 gigapascals.
Now they had to describe the crystalline structure. This is done using neutron diffraction, whereby a stream of neutrons is fired at the substance. The resultant diffraction pattern allows scientists to painstakingly deduce the crystal structure of an ice polymorph [5]. The process usually requires replacing the water used in the sample with ‘heavy water’. In ‘heavy water’, the water molecules have deuterium which is an isotope of hydrogen containing an extra neutron [5][7]. However, this was impractical for the experiment since heavy water freezes too slowly. Luckily, the team found that dousing the heavy water with some light water minimised disruption to ice-freezing process and meant it was still suitable for neutron diffraction [5][7].
The fact that both Ice XV and XIX come from same ‘parent’ ice means that scientists can now observe the transition process between two ice forms in one experiment. It is hoped that this will help improve our understanding of the hydrogen bond; hydrogen bonds are imperfectly understood but key in different ice polymorph structures [7].
Superionic water or Ice XVIII and the ice giants Uranus and Neptune
As one might expect, giving the massive variations of temperature and pressure across the universe and subsequent incidence of different ice polymorphs, the study of ice polymorphs is valuable to astrophysics because it helps explain the behaviour of icy structures of celestial bodies [5][7]. For example, Ice VII, (which has a cubic crystalline structure and forms at 30,000 atm) is hypothesized to constitute the ocean floors of Europa, (the smallest of Jupiter's Galilean moons) [12]
Another variant, Ice XVIII has also received much attention due to its potential role in the icy cores of Uranus and Neptune. Uranus and Neptune have been found to have magnetic fields which differ greatly to that of earth. One hypothesis suggests that super ionic water could play a role. [8]. Ice XVIII or ‘superionic water’ is a phase 19
CHEMISTRY
that exists at very high temperatures and pressures. In it the water molecules ionise so that the oxygen atoms crystallize into a regular lattice and hydrogen atoms float freely. This means that under the effect of an electrical current the hydrogen ions (really just protons) can freely move to carry current [9].
Returning to our gas giants Uranus and Neptune. On earth, iron in the planet’s iron core flows in such a way that the negatively charged electrons create earth’s magnetic field. However gaseous Uranus and Neptune of course lack this ferromagnetic core, yet also display magnetic fields. Therefore, it is hypothesised that the flow of protons in superionic ice instead acts to create their magnetic fields [10].
Left: diagram to show oxygen ions (red) in a lattice and the diffusing protons (white)
Right: Under the influence of an electrical field, the protons/H+ ions travel towards the anode [9]
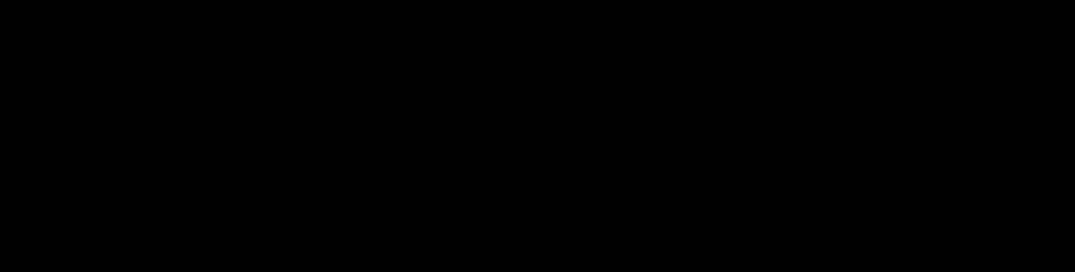
Sources: [1] https://www.sciencealert.com/water-unique-properties-tetrahedral-molecule-arrangement [2] https://www.britannica.com/science/water/Structures-of-ice. [3] https://www.lpi.usra.edu/meetings/scssi2008/pdf/9014.pdf [4]https://www.reference.com/science/happens-water-freezes-644a34973e18fc85 [5]https://www.livescience.com/exotic-ice-19-discovered.html [6] http://hyperphysics.phy-astr.gsu.edu/hbase/Chemical/waterdens.html. [7] https://www.sciencedaily.com/releases/2021/02/210218094516.htm [8] https://www.chemistryworld.com/news/glimpse-of-waters-superionic-state-may-explain-icy-giantsoddities/3008626.article#/ [9] https://www.nature.com/articles/s41586-019-1114-6 [10]https://www.apexmagnets.com/news-how-tos/superionic-water/ [11] https://www.nature.com/articles/19480 [12] https://www.sciencedaily.com/releases/2007/05/070516151053.htm