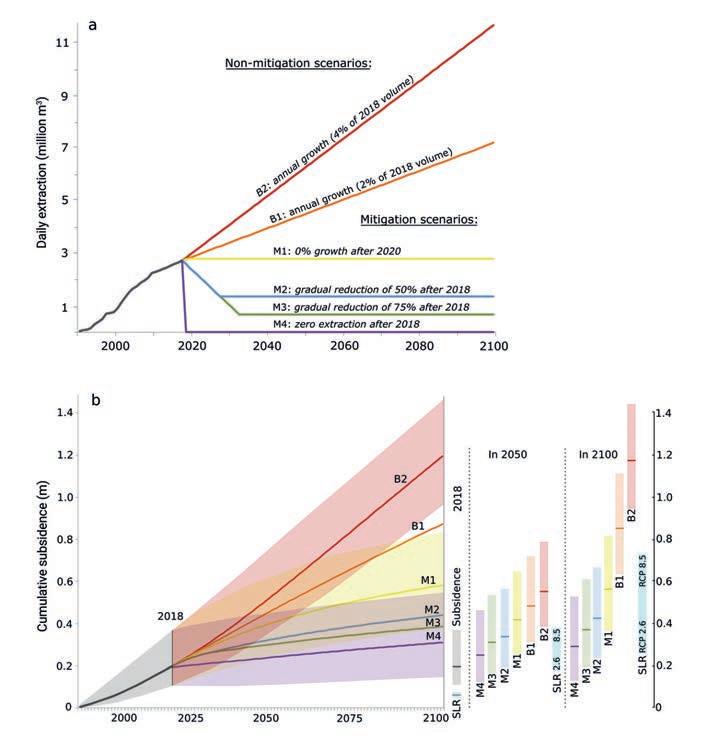
11 minute read
4. The delta’s future
Riverbed level incision (~15 cm/year) [ Binh et al., 2018; Eslami et al., 2019b; Vasilopoulos et al., 2020 ] is responsible for the increased salt intrusion length in the delta, while the anthropogenically-altered hydrological regime [ Chapter 7 ] caused by upstream hydropower operations is responsible for the frequently observed longer periods of salinity. Sediment starvation due to upstream entrapment of over 350 tributary and 14 mainstream dams, and large amounts of sand mining [ Figure 9.10 ] in Viet Nam, Cambodia, and Laos (several times larger than the total fluvial sediment supply [ Bravard et al., 2013; Brunier et al., 2014; Eslami et al., 2019b; Jordan et al., 2019 ] have resulted in the observed riverbed level incisions that drive salinity further inland [ Eslami et al., 2021a ]. Furthermore, historically, Lake Tonle Sap provided freshwater to the delta until late January. But with the observed peak water level decline in the Mekong River, Lake Tonle Sap does not store water to its capacity, and often drains by mid-late December, resulting in the maximum dry season salinity increase taking place earlier than before, and the delta experiencing a longer period of salinity.
4.1 Elevation loss
The delta is losing elevation in relation to sea level due to the combination of climate change-induced sea level rise [ Chapter 1 ], subsidence resulting from both natural and anthropogenic processes, and reduced sediment deposition. As the delta has an extremely low elevation above the sea, relative sea level rise poses a major and urgent threat to the delta. Projections of elevation are important to assess future flood exposure, saline water intrusion and potential permanent inundation as parts of the delta fall below sea level. Although subsidence is related to a large number of drivers and processes, only large-scale regional processes need to be considered, since local processes — such as sinking of individual objects or buildings — do no contribute to general delta elevation loss. As described above, the two main subsidence processes are natural shallow compaction and extraction-induced aquifer-system compaction, with the latter being the dominant driver of present-day subsidence.
The effect of potential future groundwater extraction and consequent subsidence was modelled using the aforementioned 3D model, following several mitigation and non-mitigation scenarios of groundwater extraction during the XXIst century [ Figure 9.11 ]. The amount of future extraction-induced subsidence will strongly depend on the amount of future groundwater extraction, with much lower subsidence rates associated with stronger reductions in extraction. It also became clear that in the coming decades, almost regardless of the level of mitigation, extraction-induced subsidence will outpace global sea level rise in the VMD. However, in the second half of the century, the mitigation scenarios show equal or lower rates of subsidence in comparison to gradually increasing sea level rise. For the VMD, this means that directing efforts on mitigating subsidence by reducing groundwater extractions is the most efficient way to reduce relative sea level rise in the near future.
[ Figure 9.11 ] Groundwater extraction scenarios and induced subsidence
(a) Groundwater extraction pathways for the XXIst century for different (non-)mitigation scenarios modelled in the VMD [ Minderhoud et al., 2020 ]. (b) Evolution of cumulative delta-average subsidence following the extraction pathways. Solid lines show the best-estimate model results, and the colours cover the range between the least and most conservative model. Time slices for 2050 and 2100 show the comparison of extraction-induced subsidence and sea level rise following IPCC SROCC scenarios [ Minderhoud et al., 2020 ].
In a naturally functioning delta, subsidence is counterbalanced by sediment deposition on the delta surface. However, there are no delta-wide data or estimates of present or future sediment deposition in the VMD. Therefore, it is not possible to create spatially explicit calculations including exact quantifications for sedimentation. Although unlikely in the present situation, especially considering the strong decline in fluvial sediment reaching the delta, it can be assumed that natural compaction is being compensated by sediment deposition. Following this assumption, delta-wide future elevation was projected following projections of sea level rise and extractioninduced subsidence [ Figure 9.12 ].
[ Figure 9.12 ] Projections of future elevation
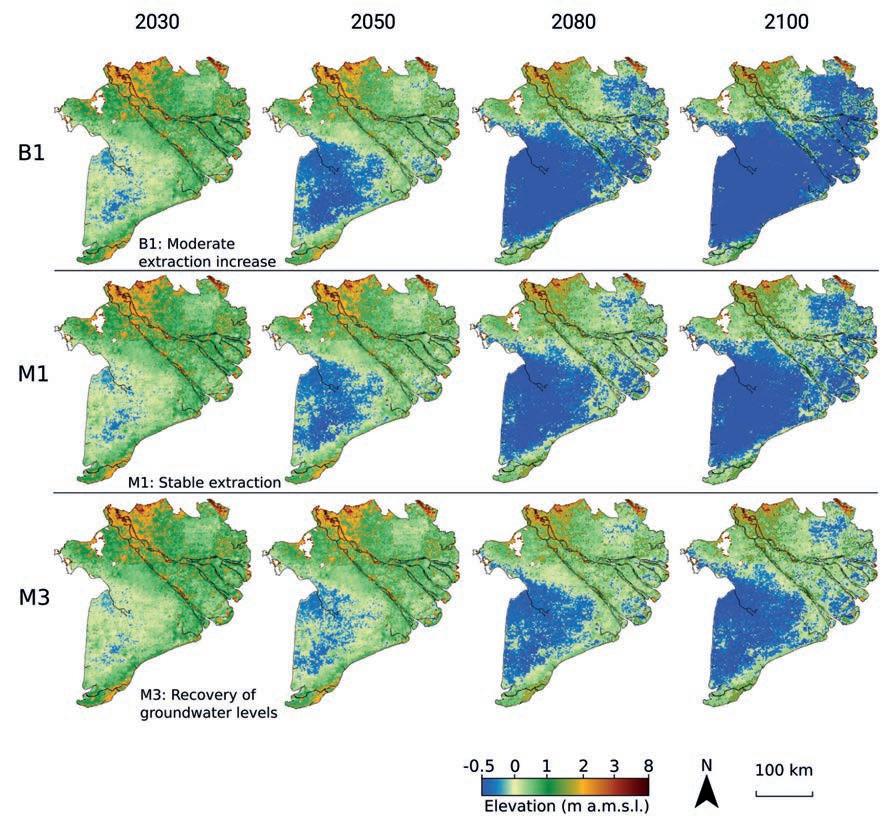
Projections of future elevation with extraction-induced subsidence following three extraction scenarios and global sea level rise according to the mid-range (RCP4.5) projection. Elevation is in metres above mean sea level based on the TopoDEM [ Minderhoud et al. 2019a, 2019b ]. Additional elevation loss by subsidence because of natural compaction is assumed to be counterbalanced by elevation gain through deposition of new sediments.
4.2 Salinity in the delta
This section presents the results of recent projections of future salt intrusion in the delta, carried out within the framework of the GEMMES Viet Nam and the Rise and Fall projects [ Eslami et al., 2021b ], using a stateof-the-art numerical model [ Eslami et al., 2019a, 2021a ] of the VMD (Delft3D-FM). This project combined the results of the first delta-wide 3D surface-water model of the VMD, stretching from Kratie to 70 km offshore, and the first hydrogeological model of the VMD [ Minderhoud et al., 2017 ] (Section 2.3.), to examine the effect of both climate change-related and anthropogenic drivers of change in isolation and in combination.
Climate change drivers of salt intrusion
Global climate change can impact salt intrusions in the VMD through seal-level rise and variations in fluvial discharge to the delta. The rates of sea level rise are set to increase in the coming decades [ Chapter 1 ], with potentially substantial implications for salt intrusion in the VMD (and other deltas). On the other hand, due to anomalies in evaporation and precipitation, river discharge is generally expected to increase in the first half of the century [ Guerra- Chanis et al., 2019; Hoang et al., 2019; Lauri et al., 2012; Hoang et al., 2016; Thompson et al., 2013 ], which could potentially reduce salt intrusion. Therefore, it is the combined effect of increased freshwater supply and sea level rise that determines the role of climatic drivers on salt intrusion in the delta. Figure 9.13a shows projected sea level rise [ MoNRE, 2016 ] along the VMD coast under RCP4.5 and RCP8.5 climate scenarios. These projections are relatively similar, with an increase of 23 cm and 25 cm respectively in 2050. However, considering the upper tail behaviour and uncertainties in sea level projections, an additional extreme scenario of a 60 cm rise by 2050 is also considered [ Bamber et al., 2019 ]. Figure 9.13b shows that dry season cumulative upstream discharge is projected to increase until 2050 (including the effects of hydropower development) under both RCP4.5 and RCP8.5 scenarios. These trends were obtained by the regional Integrated Catchment Model (INCA) [ Wade et al., 2002; Whitehead et al., 1998, 2019 ] (forced by the Global Circulation Model GFDL-CM) and validated by comparison with five different global climate models simulated by PCRGLOBWB [ Sutanudjaja et al., 2018 ] used in the Aquaduct Flood Project [ Sutanudjaja et al., 2018; Ward et al., 2020 ], (see Eslami et al. (2021b) for further details). This study considers the year 2015 (non-drought) as the baseline, and linearly increases discharge towards 2050 following these trend lines to provide a non-exaggerated projection of salt intrusion in the delta. Given the observed hydrological regime shift of the Mekong River, it is realistic to assume that Lake Tonle Sap has zero contribution to the VMD freshwater supply during the peak of the dry season from January to April (see Chapter 7).
Anthropogenic drivers of salt intrusion
The anthropogenic drivers are categorized under spatially-varied extraction-induced land subsidence [ Minderhoud et al., 2017 ], and riverbed level changes due to sediment starvation [ Bravard et al., 2013; Eslami et al., 2019b ]. Two different scenarios are considered for each driver:
uFigure 9.13c shows two opposing extreme and favourable scenarios for groundwater extraction [ Minderhoud et al., 2020 ], leading to projected land subsidence due to
[ Figure 9.13 ] Scenarios for drivers of saline water intrusion
Scenarios of salt intrusion drivers [ Eslami et al., 2021b ], climate change-driven sea level rise (a) and discharge variation (b); scenarios of groundwater extraction (c) and sediment loss from the riverbeds (d).
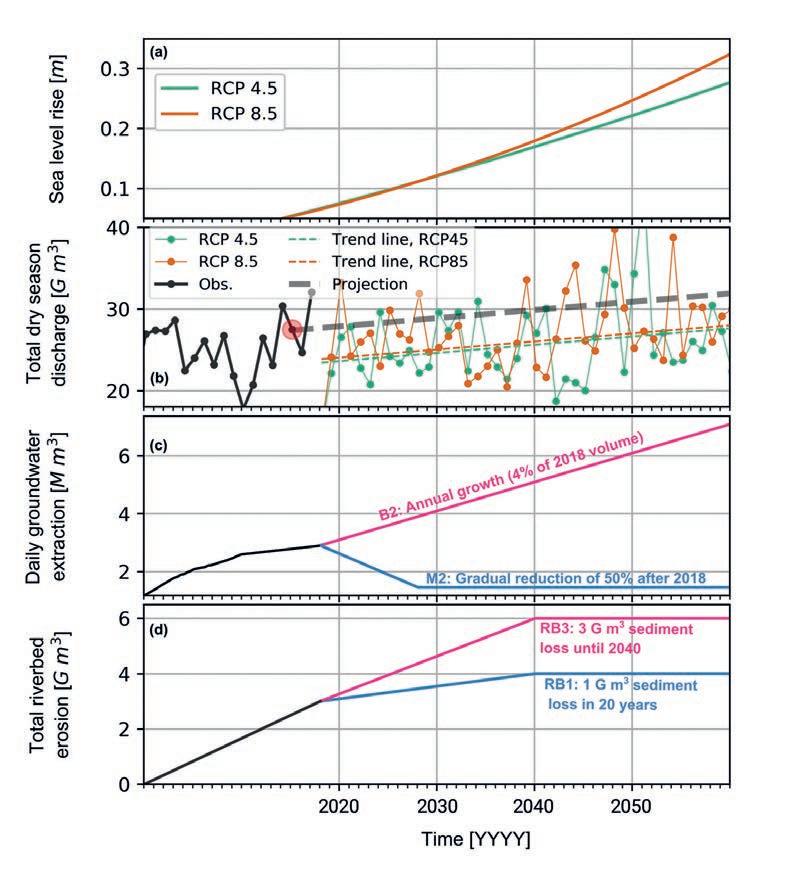
aquifer-system compaction. Given the provisions incorporated in Resolution 120 of the Vietnamese government, we may expect reduction in groundwater extraction. The M2 scenario reflects 5% annual reduction to 50% total extraction volume by 2050. B2 is considered as the extreme scenario (business as usual) with a 4% annual increase in extraction rates following the trends witnessed over the past 25 years [ Minderhoud et al., 2020 ] (see Section 4.1). The subsidence rates are translated into local bed level changes
of canals and rivers, assuming a constant coastline.
uThe average 2–3 m riverbed level incisions [ Eslami et al., 2019b; Vasilopoulos et al., 2020 ] over the past two decades (Section 3.2) are equivalent to losing ~2–3 109 m3 (150–200 M m3/year) due to sediment starvation. Considering the 1 109 m3 demand for sand through to 2040 [ SIWRP, 2015 ] and the continuous erosion caused by upstream suspended sediment trapping, two scenarios for riverbed changes through to 2040 are considered, assuming that erosion ceases beyond 2040 due to rising awareness and lack of erodible material [ Figure 9.13d ]. RB3 assumes erosion rates identical to the past 20 years (business-as-usual) and RB1 assumes significantly lower (one third) erosion over the next two decades.
Projections of salt intrusion
The isolated and combined effects of climate change and other drivers are presented in Figure 9.14. They describe a wide range of possibilities for the spatial distribution of the dry season median (P50) salinity increase across the delta. Spatial variation of net salinity increase, relative to the base case scenario (year 2015), shows how a present-day normal year could change over the next three decades. Climate change alone [ Figure 9.14a-f ] can increase salinity by 2–2.5 ppt by 2040 in all coastal provinces and add ~6% additional areas affected by salinity. However, within the estuarine networks, the effect of absolute sea level rise is balanced by upstream discharge increase. Additional land subsidence [ Figure 9.14g-l ], especially under extreme scenarios, similar to the effect of climate change, mainly increases salt intrusion in the coastal provinces by an additional average 0.5–1 PPT by the year 2040 and adds 2–6% additional salinity-affected areas with limited impact on salinity dynamics in the estuarine channels. However, even moderate scenarios of riverbed level changes dramatically increase salinity in the estuarine channels (an additional 5–30 km) and consequently increase areas affected by salt intrusion within the delta. Riverbed level changes, depending on sediment supply and sand mining practices (within and beyond the delta), can increase the total areas affected by salinity by an additional 5–25% by 2050 (an additional 150–500 103 ha of land). Given the uncertainties in various drivers of change, we may expect a range of possibilities from ~5 to ~40% increase (+100 to 700 103 ha) in areas affected by saline water intrusion by 2050.
Within the VMD provinces, Ca Mau Peninsula is most vulnerable to sea level rise and land subsidence, while provinces such as Bac Lieu, Soc Trang and Kien Giang are also highly impacted. On the other hand, the estuarine system is most sensitive to riverbed level changes. Therefore, in keeping with observed trends over the past two decades [ Eslami et al., 2019b ], we expect stronger response in the Tien estuarine system compared to Hau River in the all-drivers scenarios, mainly due to the fact that we expect little to no change in the lower Hau (downstream of Can Tho) estuarine channel depths [ Allison et al., 2017 ]. This means that even provinces further inland, such as Vinh Long and Dong Thap (currently with no experience of salt intrusion), will potentially have limited/no direct access to fresh river water during the dry season. Note that, under all-driver scenarios, in an extreme drought event similar to 2016 or 2019, saline water can creep up to ~150 km inland (see Figure 9.2 and Eslami et al., 2021b for further detail). Sensitivity analysis shows that these projections can vary significantly de-
[ Figure 9.14 ] Scenarios of increase in saline water intrusion under climatic and anthropogenic drivers
Spatial distribution of P50 (average) salinity increase and the extent of salt intrusion (2 PPT abolute salinity contour line) under RCP4.5 (uneven rows) and RCP8.5 (even rows) CC scenarios for CC scenario only (a-f); combined effect of CC and land subsidence (g-l); combined effect of CC, land subsidence and bed level incision (m-r); the green areas define the existing freshwater zones (mainly by sluice gates); the red percentage per panel is the increase in salinity-affected surface area relative to the present-day conditions, and the red number in thoudand hectares [K ha] per panel shows increase in area affected by salt intrusion uder that scenario.
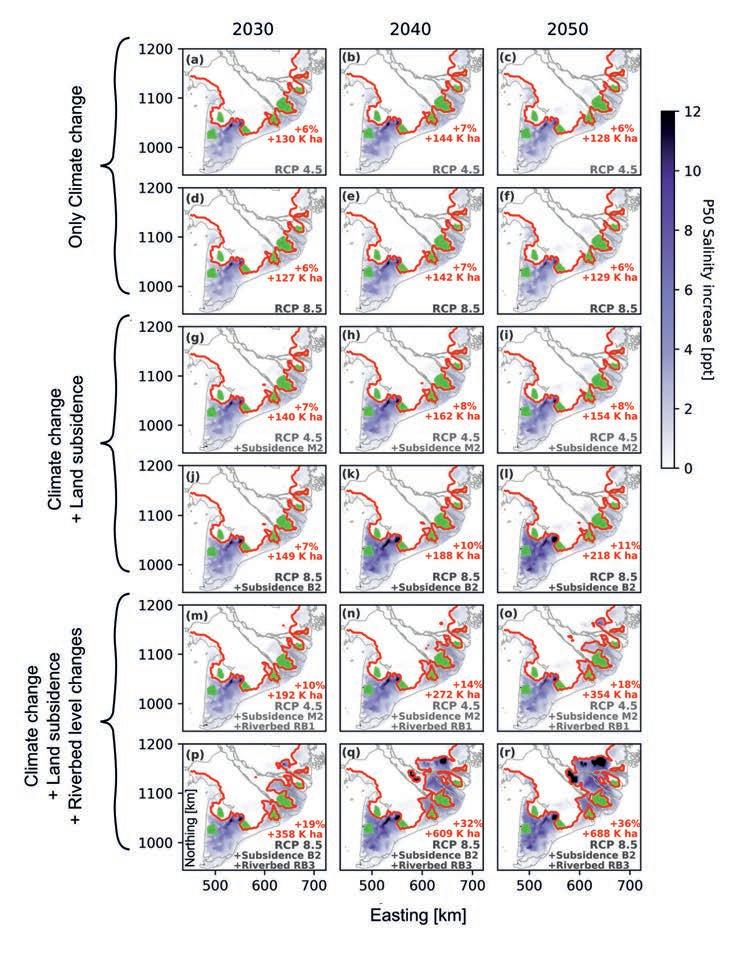
[ Figure 9.15 ] Projections of increase in areas affected by saline water intrusion
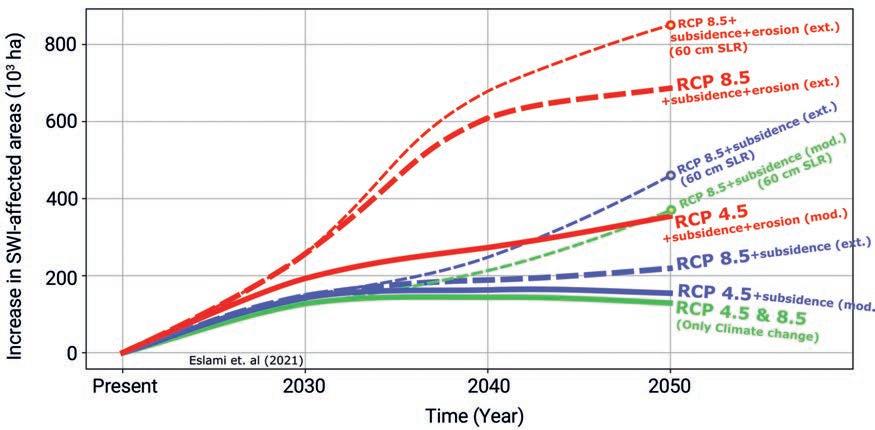
Projections of increase in SWI-affected area (2 PPT contour line in spatial P50 salinity projection maps) within the VMD (10) under various combinations of scenarios of climate change projections (RCP4.5 and 8.5), moderate (mod./M2) and extreme (ext./B2) land subsidence, and moderate (mod./RB1) and extreme (ext./RB3) riverbed erosion, including the case of extreme SLR in the year 2050.
pending on environmental or policy changes [ Eslami et al., 2021b ]. For example, if the Hau Riverbed levels — downstream of Can Tho — incise (which we do not expect), this will have massive repercussions for the Can Tho province. Furthermore, extreme sea level rise (+60 cm) in response to accelerated Antarctic ice-sheet melting [ Bamber et al., 2019 ] may significantly worsen salt intrusion within and outside the estuarine channels, with an additional 9–13% to the total areas affected by salinity, with dramatic implications for land-use and adaptation strategy, even if the coastlines were maintained.
Despite considering a range of sensitivity analyses, the undisputed overall conclusion is that riverbed level changes pose the greatest threat in increasing salt intrusion in the next 2–3 decades. Figure 9.14 summarizes the expected temporal change in areas affected by salinity under different scenarios, including the scenario considering the effects of extreme sea level rise. Under climate change alone, the areas affected by salinity will decline between 2040 and 2050. However, this will not be the case if sea level rise accelerates beyond RCP8.5 projections, and this can also not be extrapolated beyond 2050, as the climate projections — especially upstream discharge — diverge beyond 2060. Therefore, there may be a larger number of environmen-