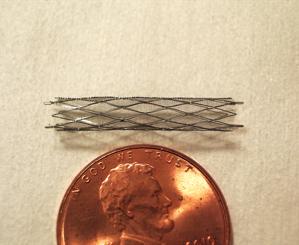
3 minute read
Youngjae Chun, PhD
Associate Professor
Department of Bioengineering (Secondary)
1041 Benedum Hall | 3700 O’Hara Street | Pittsburgh, PA 15261 P: 412-624-1193 C: 310-310-0622
yjchun@pitt.edu www.pitt.edu/~yjchun/home.html
Some of the most challenging studies for medical devices today are in the interdisciplinary research on advanced material processing, biology/chemistry of interfaces, physics for design/ manufacturing (i.e., microfabrication), in-vitro/in-vivo tests, and clinical trials. Our group’s primary research focus is on designing, manufacturing, and testing of medical devices to treat vascular diseases (e.g., cerebral and aortic aneurysms, peripheral arterial disease, coronary artery diseases and heart valves disease, etc.) using smart artificial materials through minimally invasive surgery. Our research lab is collaborating with a wide range of researchers focused on developing a device for important or sustainment of human life. Besides a fundamental knowledge and technology in design/manufacturing, we have a profound interest in biology, chemistry, and medicine because these complex interrelated topics represent the key challenges facing most medical devices used in the vascular system. In addition, we are expanding our research to implantable microsystems (e.g., sensors and actuators) for diagnose and treatment of vascular diseases, as well as more on circulatory diseases (i.e., cardiac, pulmonary, or renal) in the human body. Our goal of this research is to diagnose and help in the treatment of specific circulatory diseases concurrently using micro-scale devices implanted in the conduit of body. Our ultimate goal is to provide innovative minimally invasive surgical solutions based on new developments in science and engineering.
A Microstent for Cerebral Aneurysm Occlusion
The current gold standard to treat cerebral aneurysms is endovascular therapy using platinum coils to fill the aneurysm sac. While coils are beneficial, they are only useful for aneurysms with “necks” narrow enough to hold coils in place. To address this issue, we developed a novel approach, namely “Thin Film Nitinol Covered Microstent,” to occlude the neck of the cerebral aneurysm using a micropatterned hyper-elastic ultra-thin film nitinol (i.e., thickness <6μm). This work includes developing thin film nitinol materials with specific properties, designing hyper-elastic (i.e., >700% strain without mechanical failure) micro patterns using finite element modeling, developing innovative microfabrication techniques (i.e., a novel lift-off method) to create robust micro features, and conducting in-vitro/in-vivo testings. During this work, we had to become knowledgeable on several fields including physics for device mechanism, chemistry for blood and device interaction, and biology for thrombogenic behavior. In one example, we successfully mounted a device we developed into a 3Fr (i.e., inner diameter = 0.69mm) micro delivery catheter, deployed in 3~5mm diameter arteries in vivo swine models overcoming many engineering challenges. More importantly, results showed 4 week patency in the arteries without thrombogenic complications and undesirable excessive neointimal hyperplasia, something that was previously not thought possible in small diameter vessels. In addition, we have found that one of the dominant concerns of thin film nitinol material (or for that matter any vascular device) is thrombosis when a device is used in small vessels (e.g., <4mm diameter). For many artificial materials thrombosis is directly related to hydrophilic and negatively charged surfaces on the materials. We have successfully demonstrated a new surface that is superhydrophilic and negatively charged, a feature that prevents thrombosis from occurring in small diameter vessels.
Smart Prosthesis using Nitinol and Other Metallic Biomaterials
In addition to vascular devices and associated studies, our group focuses on prosthesis. One novel material for prosthetic devices is Nitinol. This material is a biocompatible, equiatomic alloy exhibiting shape memory properties. It has many desirable attributes ideal for body replacement. The thermally induced phase transformation from martensite to austenite allows it to be deployed using the natural heat generated by the body, exhibiting superelastic behavior. The unique properties of nitinol material such as superelasticity, thermally induced mechanical property control, and lightweight enables the prosthesis to be simple, light, and easy to control. Another novel material is Magnesium, which is a metallic biodegradable material that is ideally able to compromise its mechanical integrity and degradation during the tissue or bone remodeling period. Several magnesium alloys showed a prolonged degradation time during implantation as well as enhanced ductility for medical devices. The unique advantages of biodegradable magnesium provide a promising candidate material for prosthetic devices that are possibly totally resorbed in the healing process. Our group is also studying on other metallic biomaterials and manufacturing processes.