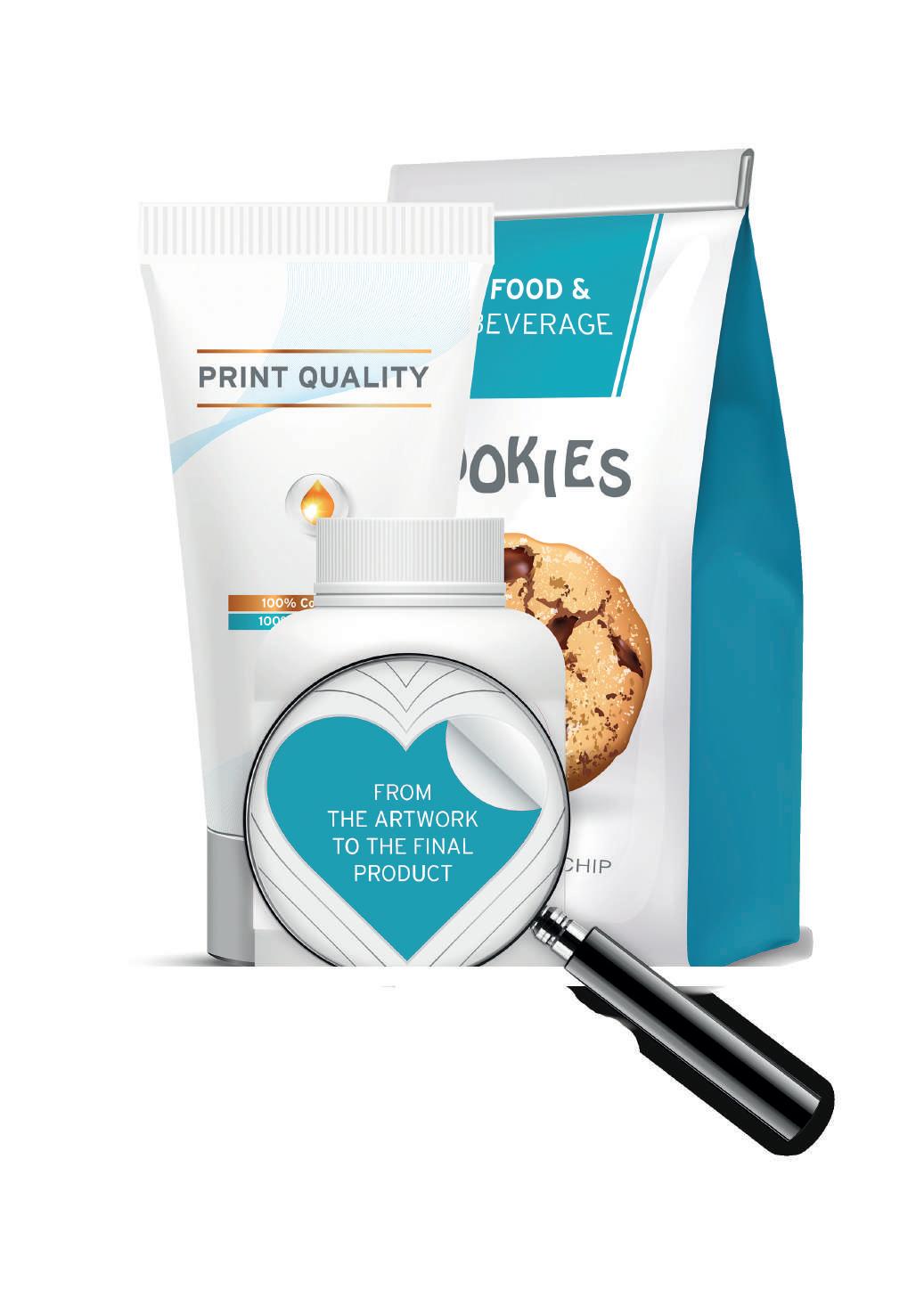
24 minute read
Advanced Capsule Development for Today’s Needs: HPMC
from IPI Autumn 2020
by Senglobal
The use of compounds (of biologic or chemical origin), as therapeutics is as old as mankind. Traditionally, the administering of such therapeutic products to the body occurred through the mouth by swallowing. As early as 1500 B.C. therapeutic products were being enrobed with a gelatin film, which can be considered as the first attempt at a gelatin capsule development.
Capsules Background Whilst the development of capsules has been relatively slow since then, more than 100 years ago the pre-manufactured empty two-piece capsule was introduced. These two-piece capsules consist of a body part that can be filled with the therapeutic agent or formulation, and a cap to close the open end to contain the product. The capsules were originally made of gelatin and quickly gained appraisal for medicinal products due to the ease of filling and administration. They also assist with taste masking from unpleasantly flavoured medicinal formulations.
Advertisement
With the emergence of life sciences in the middle of the last century, our medical, pharmacological and pharmaceutical knowledge gradually increased. Since the 1960s, several new chemical entities now reach the market every year. Along with these new drugs, pharmacokinetics and drug delivery evolved as important sciences in their own rights. Gelatin capsules have served as enabling dosage forms to bring these new approaches to the market. For example, we can view these capsules as the forerunners of many of the new drug delivery technologies, like liquid and semi-solids, multiparticulates e.g. pellets and minitablets, as well as dry powder inhalation formulations.
Defining the Desired Product Characteristic Besides the versatility of the two-piece capsule dosage form, gelatin provides some favourable material attributes such as: high mechanical robustness, fast aqueous dissolution, taste and order masking, bi-chromatic differentiation, and ease of swallowing. Based on these attributes, gelatin capsules represent a type of “golden standard” for the administration of a vast variety of drug delivery technology. However, as pharmaceutical and medical science and technologies evolve, we are facing new challenges in the provision of effective drug products. These challenges span from access to medicines in developing countries, to highly sophisticated drug products for unmet medical needs. Whilst other drug delivery technologies are being developed, the capsules still play a crucial part in delivering affordable, accessible and even specialised medicines. In order to meet current and future challenges, it is essential we work on the development of two-piece capsules with specific characteristics and/ or functionalities, while maintaining the key features of versatility and commercial viability of the gelatin capsule.
Science and technology continue, and will continue, to progress rapidly. The constant generation of new therapeutics and drug delivery technologies leads to the increasing demand for capsules with specific attributes in order to enable their development to the benefit of patients. Such specific attributes are derived from the target product profile (TPP) that includes all the important aspects that the finished
Requirements Aspects
- Dissolution
Targets
- IR - MR/SR - Enteric
Scientific/ technical - Head space humidity - Fine particle fraction DPI - Hygroscopicity - Moisture sensitivity
- Stability - Degradation - Impurities - Market access
Capsule attributes
- 2–3 min - 10–15 min - 30–45 min - pH dependent - Low moisture - Equilibrated moisture
- 25°C/60RH - 40°C/75RH
Patient
Commercial - Mechanical resistance - Handling - Packaging
- Paediatric form - Special populations - Swallowability - Palatability - Acceptability - Dose titration - Stress resistance - Piercing/shearing performance (DPI) - Head space humidity (e.g. multiparticulates) - Capsule size
- Weight/age/body surface dosing
- Differentiation
- Adherence
- Acceptability
- Religious/ preference
- Counterfeiting
- Technology accessibility
- Regulatory compliance - Variable/flexible dosing - Easy to open/ sprinkle (e.g. minitablets) - Polypharmacy - Visual impairment - Medication errors/safety - Effectiveness - Safety - Swallowability - Appearance - Taste - Order - Halal - Kosher - Vegetarian - Vegan - Falsified medicine - Substandard medicine - Standard manufacturing - Equipment/component availability - Technology transfer - Approval process - Market access - Commercialisation - Mono-chromatic - Bi-chromatic - Bi-chromatic/ imprint - Fill volume (Fixed dose combination, multiple release) - Easy to open/ sprinkle - Surface properties - Colour shade - Size - Shape
- Polymer - Capsule composition
- Bi-chromatic/ complex printing - Tagging - Machineability - Yield - Global supply chain - Global monograph compliance - Regional monograph references
- Overall manufacturing cost
- Ecological footprint - Market access - Patient access - Energy, cleaning, waste, etc. - per unit operation - per GMP space
- Formulation design - Excipient selection - Process design - Direct filling - No of unit operations Table 1: Target product profile (TPP) considerations relevant capsule and formulation selection Table 1: Target product profile (TPP) considerations relevant capsule and formulation selection
drug product needs to achieve [ICH Q8 R2 Guideline]. The major aspects that have to be addressed in the TPP depended on the specific molecule, therapeutic component or combinations thereof, the delivery form(s), the targeted patient populations, the specific markets/regions and, finally, the technology accessibility as well as overall manufacturing costs. Some of these considerations are listed in Table 1. determine the manufacturing costs [Cogdill et al. J Pharm Innov (2007) 2:38–50]. Therefore, whilst it is critical that those in capsule development meet the ever more complicated needs of customers and the pharmaceutical market, they must carefully assess and balance the complexity of formulations and processes with efficiency in manufacturing.
Formulation and Process Design By defining the TTP, the targets of the desired drug product attributes are clearly laid out, thus serving the development of detailed plans towards formulation and process development. The multifactorial nature of drug development requires a systematic approach that starts by a certain degree of prioritisation of the required and desired attributes, as well as the collection of existing or prior knowledge in the company. Developing a product which is closest to all the required and desired final drug product attributes will benefit from a non-biased, evidence-based evaluation of the different potential formulation strategies.
In order to mitigate the risk, a formal risk assessment must be performed to identify known or predicted critical material attributes or process parameters that will, or might, impact the consistent product performance, attributes or manufacturability. During development, these critical material attributes and process parameters will be evaluated by experiments and/or simulation and predictive modelling to ensure that the product and process can be kept under control within the typical material and process variabilities [Stranzinger et al. Int J Pharm: X 1: 100004 (2019); Madlmeier et al. Int J Pharm 567:118441 (2019)].
The strong focus on technology and science in formulation, in addition to process design bias towards the use of multiple excipients and process steps often tends to over-engineer pharmaceutical products, with the consequence of product and process complexity, tight controls, reduced yield and finally, higher costs. Ensuring manufacturing effectiveness and efficiency enhancements is very challenging [Friedli et al. J Pharm Innov (2010) 5:181–192], and in an increasingly competitive market, formulation and process complexity will 82 INTERNATIONAL PHARMACEUTICAL INDUSTRY Autumn 2020 Volume 12 Issue 3
Progressing Capsule Technology: HPMC Challenges in progressing capsule technology not only arise from the complexity of formulations and manufacturing costs. New challenges are emerging as a result of other trends, such as shorter development timelines (fast track, breakthrough, priority review, and/or accelerated approval), paediatric formulations, personalisation of therapies, demographic changes, decentralisation of manufacturing, as well as cost containment and sustainability. All of these aspects require advanced excipients, formulations, processes and flexible dosage forms as they will play an essential role in supporting the emerging needs and meeting the challenges.
HPMC capsules and their advancement may provide a solution to many of the issues we are facing in developing appropriate capsules for complex formulations or specialised demands. Hydroxypropyl methycellulose, also referred to as hypromellose or HPMC, is a cellulose-based semisynthetic polymer established as a pharmaceutical excipient in the early 1970s. Four different types of HPMC, used for example as film forming agent in coatings or matrix former in modified release tablets, are described in pharmaceutical monographs [USP 41, Pharm Eur 10th edition]. HPMC is also considered a crystallisation inhibitor to increase solubility and bioavailability of poorly soluble low ionised drugs containing a neutral amine group [Zarmpi et al. AAPS J 22:49 (2020)]. Additional beneficial functionalities of HPMC are the largely temperature- and humidityindependent viscoelastic properties, as well as its relative inertness against reactive chemicals [Handbook of pharmaceutical excipients. Eds: Rowe RC, Sheskey PJ, Quinn ME. pp. 326-329 (2009)].
To combine these unique HPMC material properties with the advantage of two-piece capsule technologies, intensive research and development of technology has been invested in, in order to manufacture different types of HPMC capsules. The latest addition to the portfolio of two-piece capsules is an HPMC capsule manufactured by an innovative thermogelation process. This continues to broaden the range of functionalities and applications of capsules in drug development and manufacturing. The thermogelation technology used in producing this capsule utilises the reversible sol–gel transformation of HPMC upon heating to 50°–90° C to form a pure HPMC capsule. Specific desired product characteristics defined in the TPP can now be integrated and delivered by these capsules. The functionality provided by this pure HPMC capsule might avoid the need for intensive formulation and process development programmes caused by the addition of multiple excipients and processing steps. By avoiding these intensive processes, we can reduce complex manufacturing processes, and lower risks and costs. Key Functionalities of Thermogelated HPMC Capsules Thermogelated HPMC capsules (e.g. ACGcaps H+) are characterised by a homogeneous, smooth surface structure with glass-like
120.00% % acetaminophen dissolved 20.00% 40.00% 60.00% 80.00% 100.00% 0.00%
0 5 10 15 30 45 Time Figure 1: Dissolution profiles of thermogelated HPMC capsules (ACGcaps™ H+) filled with acetaminophen in different media (USP Apparatus 2 (Paddle) with sinker at 50 rpm; ACGcaps H+ capsule size 0 transparent filled with 464 mg acetaminophen (paracetamol) granules composed of 90 % acetaminophen; 1 % mag stearate; 3 % PVP; 2 % croscarmellose sodium and 4 % lactose).
pH 1.2 - 0.1 N HCl pH 6.8 - phosphate buffer USP pH 6.8 - phosphate buffer 2nd JP pH 4.5 - acetate buffer purified water Figure 1: Dissolution profiles of thermogelated HPMC capsules (ACGcaps™ H+) filled with acetaminophen in different media (USP Apparatus 2 (Paddle) with sinker at 50 rpm; ACGcaps H+ capsule size 0 transparent filled with 464 mg acetaminophen (paracetamol) granules composed of 90 % acetaminophen; 1 % mag stearate; 3 % PVP; 2 % croscarmellose sodium and 4 % lactose).
transparency. As for any two-piece capsules, colourants can be added to retrieve coloured transparent or opaque capsules, which can further be customised by mono- and/or bichromatic printing. Different dose strength, fixed dose combination products, products with multiple release profiles can all be effectively differentiated within the brand, but also across other drug products to reduce confusion and increase drug safety in the context of polypharmacy. In addition, the glass-like transparency of the capsules serves the patient preference in inhalation therapy, to be able to verify that the powder was present before and released after use.
Using standard dissolution tests (e.g. USP type II apparatus with sinker) and acetaminophen as a model drug formulation, the capsule ruptures and releases the content within 5–10 minutes in all media (pH 1.2 – 0.1N HCl; pH 4.5 – acetate buffer; pH 6.8 – phosphate buffer).
The dissolution profiles are in accordance with the pharmacopeial requirements for immediate release oral formulations. However, for weak acid or weak base drugs with poor aqueous solubility like dabrafenib, HPMC capsules might act as a crystallisation inhibitor and increase the bioavailability [Ouellet et al. J Pharm Sci 102(9): 3100–3109 (2013)]. For such BCS class two compounds, the thermogelated HPMC capsules provide superior dissolution and bioenhancement functionality, which might also contribute to a reduced inter- and intra-subject variability.
Specialised Patient Needs Multiparticulates like pellets, minitablets or granules are increasingly being considered as the formulation of choice for special patient populations to increase palatability and swallowability, to allow individual dosing according to body weight, body surface, age or kidney function [Klingmann et al. J Pediatr 163:1728-32 (2012); Klingmann et al. J Pediatr 167:893-6 (2015)]. Modified release, e.g. enteric formulations, are the preferred oral form due to their gradual and predictable release from the stomach [Digenis et al. J Clin Pharmacol 30, 621-631 (1990); Abrahamsson et al. Int J Pharm 140, 229-235 (1996)]. Many of these formulations are designed to protect acid and moisture sensitive drugs as well as quickly dissolve in the duodenum by using hydrophilic excipients. To achieve sufficient stability of such systems, the head space humidity in a two-piece capsule needs to be within a defined range. The capsule moisture (LOD) of HPMC capsules is directly correlated with the head space humidity and is adjustable without impacting the mechanical and elastic properties of the capsule or its dissolution HPMC capsule. For example, if manufacturing of products at conditions of 22°C and 20% RH, the HPMC capsule provides the respective design space for robust product performance. In the same way, interactive powder blends or engineered particles for dry powder inhalation require specific head space humidity that can easily be adjusted with HPMC capsules without negatively impacting the performance of the capsules in the diverse inhalation devices [Geller et al. J Aerosol Med Pulmo Drug Del 24(4):175-182 (2011)].
Advanced drug delivery technologies targeting specific areas in the GI tract, e.g. colonic delivery, and emerging sensitive therapeutics, e.g. live microbiota, require oral dosage forms that do not use compression and release the therapeutic agent within a specific gastric environment quickly. Coating of filled capsules have been demonstrated to be most suited for targeted delivery to the gastrointestinal tract. Aqueous coating composition has demonstrated favourable adhesion characteristics of HPMC, and as such on HPMC capsules. By using different types of coating polymers and varying the coating thickness, the in vivo release could be targeted to different areas within the gastrointestinal tract [Cole et al. Int J Pharm 231: 83-95 (2002)]. Conclusion Pharmaceutical drug products are an essential part in healthcare delivery in developed as well as developing countries. Evolving science continues to fuel the therapeutic options for acute, chronic and life-threatening disease, as well as the relevant new drug delivery technologies to enable their therapeutic efficacy and site-specific delivery. There is a growing demand for affordable medicines from low- and middle-income countries and it is our global responsibility to produce high-quality medicines more efficiently and locally to meet these needs.
The two-piece capsule is an established dosage form with proven viability for a large variety of compounds, formulations, drug delivery technologies and applications. Because a capsule product can be manufactured with just two-unit operation (blending and filling), encapsulation has been used from early medicine development, through to commercial manufacturing, with global availability in pharmaceutical manufacturing sites. However, advances in technology and the growing complexity of customer needs have resulted in rising manufacturing costs of capsules with more specialised properties or formulations.
To deliver on these emerging requirements, the advantages of capsules can be applied to a variety of other products by developing capsules with specific properties. The HPMC capsule manufactured by a thermogelation process provides a solution to many of the challenges faced by those developing and manufacturing drugs. It is a fast-dissolving capsule that provides the opportunity to be adjusted to specific moisture or head space humidity whilst maintaining the elastic properties even under stress (e.g. piercing or shearing in a device). As such, the capsule is highly resistant across environmental temperature and moisture conditions. For products sensitive to compression, liquid or semisolids or life microbiota requiring targeted delivery in the gastrointestinal tract, the capsule can be easily coated with different coatings and coating thickness. Using standard capsule-filling equipment, at known machineability performance, the new HPMC capsule also contributes to formulation and process simplification, which are the most important factors in increasing cost efficiency in pharmaceutical manufacturing.
Sven Stegemann
Sven Stegemann, head of Global Scientific Business Development, ACG. Over the course of his 28-year career in the pharmaceutical industry, Prof. Dr. Sven Stegemann has worked as an adviser to major pharmaceutical companies on ways to improve the formulation design, development and manufacture of pharmaceutical products, including advanced drug delivery and manufacturing technologies and controls. In his academic role, he focuses his research on the rational development of patient-centric drug products and their associated manufacturing technologies, as well as education and training of students and young scientists.
Best Practices for Glass Delamination Testing Studies
Matthias Bicker, Daniel Haines, and Uwe Rothhaar
SCHOTT pharma services provides compatibility testing for drug products in glass vials1 including a delamination screening package aligned with USP <790>2, USP <1660>3 , and EP 3.2.1.4 recommendations. Over the last decade, the design of such studies was progressively improved to provide reliable data for risk assessment for drug container compatibility. The essential factor for the suitability of such investigations is the categorization of different observations with respect to their criticality and that features need to be found which are early indicators for the later occurrence of delamination (i.e. applied predictive screening test methods “should look for precursors that lead to delamination”3).
The containers to be tested and filled with drug product or placebo solution, can be drawn from real-time stability studies or stored under accelerated ageing conditions. The extent of glass corrosion and chemical attack is assessed by analyses of the inner glass surface morphology, the concentrations of extracted elements in solution, and by identification of particles and flakes. Some typical results derived with such studies have been previously published5,6 with additional information concerning glass leaching recently discussed7 .
SCHOTT pharma services uses a combination of the recommended analytical techniques. Illustrative results are summarized in the following section. Visual and Optical Inspection
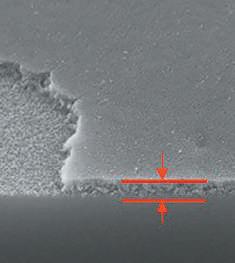
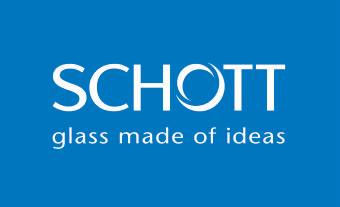
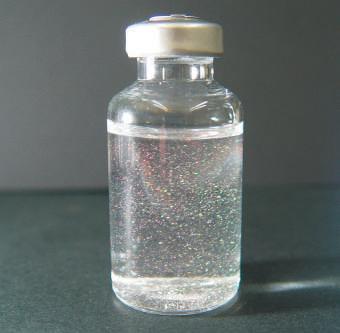
Flake-like particles by visual inspection • Used to detect particles via visual inspection by eye and camera (filled vials) and to visualize coloration and scattering (for empty and for emptied vials)
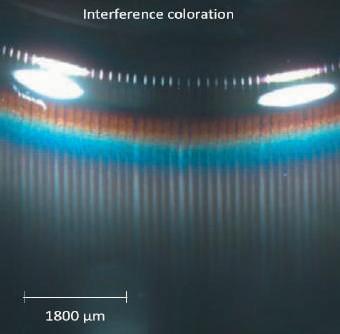
Coloration ring by stereo-microscopy • Allows for the identification of containers with high particle load and with changed surface and surface near regions to determine the worst samples of a set by stereo-microscopy
SEM Cross-section Analysis
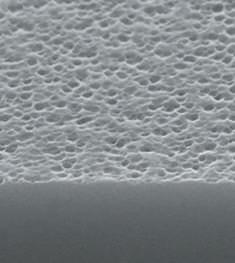
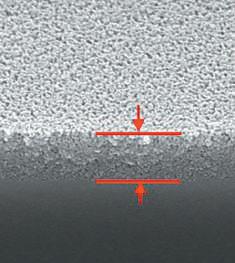
Roughening Reaction zone
200nm 100.00 K X
Delaminated area (left) and reaction zone (right) (Note: scale bar valid for all three micrographs)
• Used to determine the extent of chemical attack of inner glass surface and of surface near regions • Allows for classification between different levels of glass corrosion. Typical features are roughening, formation of reaction zones and/ or delaminated areas at the interior surface in contact with the drug product
ICP Analysis
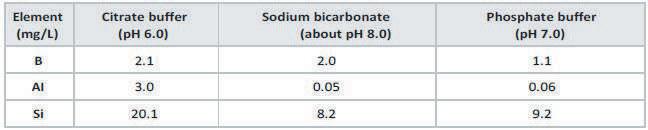
Concentration of selected leachables found after storage for 24 weeks at 40°C with different filling solutions
• Used to quantify the amounts of leached glass elements • Allows for the confirmation of the chemical mechanism of drug container interaction
SEM/EDS and Raman Microscopy
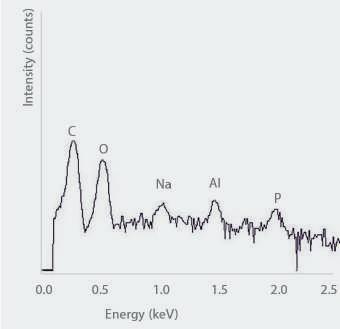
Exemplary EDS spectrum of an inorganic flake-like particle
• Used to analyse the composition of particles after filtration • Allows identification of morphology (SEM), elemental components (SEM/
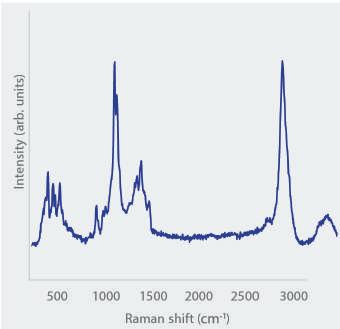
Exemplary Raman spectrum of an organic flake-like particle
EDS), and molecular structure (Raman) of isolated particles to distinguish between glass flakes and other particles
TOF-SIMS
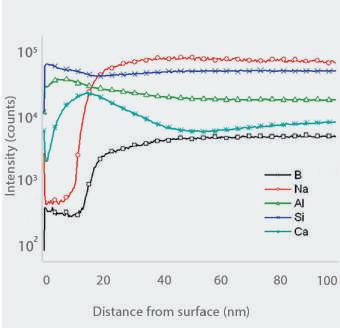
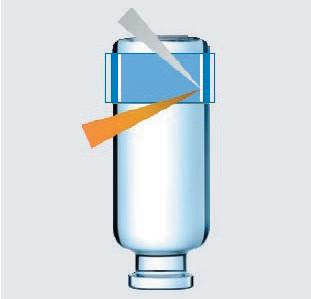
Altered elemental composition of surface near layer with B and Na depletion
• Used to characterize the elemental composition of the near surface region of container interior • Allows a better understanding of the mechanism of drug container interaction and induced glass corrosion
Exemplary Study Protocol
1. Visual inspection by eye and magnifying video camera with respect to the presence of particles or flakes (10 filled vials per time point according to Tables 1 + 2). 2. Optical inspection of emptied containers per time point: Stereomicroscopy with extended depth of focus to qualitatively determine if there are any indications for reaction zones or scattering present on the interior surface (10 vials per time point according to Tables 1 + 2). Selection of two ”worst” samples on the basis
of stereo-microscopic inspection for subsequent SEM cross-section analyses. 3. SEM (scanning electron microscopy) cross-section analyses on the interior surface of two “worst” vials for selected test conditions and selected time points as described in Tables 1 + 2; analyses of three areas: wall near bottom, middle of the vial body, and wall near shoulder. These investigations reveal the presence of a potential reaction zone. 4. ICP (inductively coupled plasma) analyses of 10 mL drug solution pooled from the vials for selected test conditions and selected time points according to Tables 1 + 2 to quantitatively determine the amount of
“glass” elements leached into solution for selected “glass” elements (e.g. Si, B,
Ca, Al) to ascertain if the amounts and ratios found are normal or if there is a pronounced chemical attack. 5. Filtration of the solution of one selected vial according to Tables 1+2 through a silver membrane (pore size approx. 0.2 μm) using a vacuum filtration unit. Subsequent SEM/
EDS and Raman analyses of found particulate matter to determine the elemental composition and morphology of the particles by SEM/
EDS and the molecular structure by match of Raman signals to library.
Optional: (If the mechanism of glass corrosion is unclear or reaction zones are observed.) 6. SIMS (secondary ion mass spectrometry) depth profiling of the interior surface to get information about the composition of the surface near region.
The techniques described above are applied at different time points under accelerated storage conditions. These conditions are defined on the basis of the drug product application and customer requirements. Exemplary study designs for predictive delamination screenings for drug products with a shelf-life of three years at 5°C (Table 1) and 25°C (Table 2) are shown on the following page.
The tables illustrate the test methods applied for one set of vials filled with drug product and stored for different time points and include the number of characterized samples.
Rationale behind the Study Protocol Storage Conditions: The definition of the accelerated storage conditions requires an estimation of an acceleration factor. In the case of a delamination or a glass leachable study, the kinetics of solid-state reactions should be considered. In such cases the impact of increasing the temperature can be described by an Arrhenius equation k = A exp(-Ea/RT) (k: rate constant, R: universal gas constant, Ea: activation energy, T: temperature, A: frequency factor).
The calculation of the acceleration factor which is the ratio k(T2)/k(T1) requires an
Method
Visual Inspection Optical Inspection Sem cross-section ICP analyses* Particle analyses by SEM/ EDS and Raman if flake-like particles are found Sims (optional)
Empty vial Control
–5 2 –After filling
0 weeks 10 10 2 Yes
1 4 weeks 10 10 2 Yes
1 8 weeks 10 10 2 Yes
1 12 weeks 10 10 2 Yes
1
1
Storage conditions/time points Storage at 40°C
*: Drug solution pooled from multiple vials
Table 1: Exemplary study design for a drug product with a shelf-life of three years at 5°C tested by using accelerated storage at 40°C
Table 2: Exemplary study design for a drug product with a shelf-life of three years at 25°C tested by using accelerated storage at 60°C
Method
Visual Inspection Optical Inspection Sem cross-section ICP analyses* Particle analyses by SEM/ EDS and Raman if flake-like particles are found Sims (optional)
Empty vial Control
–5 2 –After filling
0 weeks 10 10 2 Yes
1 6 weeks 10 10 2 Yes
1 11 weeks 10 10 2 Yes
1 16 weeks 10 10 2 Yes
1
1
Storage conditions/time points Storage at 60°C
*: Drug solution pooled from multiple vials
estimation of the unknown activation energy Ea. The lower the Ea-value the smaller the acceleration. For the dissolution of a borosilicate glass (Pyrex, buffered at pH 7) an Ea-value of 54 kJ/mol8 was published and we use this value to calculate the storage period at different temperatures. Using this approach, we obtain an acceleration factor of about 13.5 when storing a drug product at 40°C instead of 5°C For a drug product with a shelf-life of three years at 5°C the storage period at 40°C shortens to 12 weeks.
Please note that reactions with activation energies lower than 54 kJ/mol will have lower acceleration factors, while the acceleration will be higher for reactions with higher activation energy.

Number of Samples: We recommend at least 10 vials per time point and per sample set and five additional empty vials per vial container type as reference samples.
It is quite unlikely to observe a delamination effect by only applying a standard USP <790> / EP 2.9.20.2,9 test method, because of the low frequency of randomly drawn vial samples showing visible flakes and because of the low sensitivity of the method. Therefore, we are using inhouse inspection methods in addition that allow the identification of containers with small flakes load and/or changed regions to determine the worst samples of a set by stereo-microscopy.
Early indicators for delamination like "reaction zones" will be found with a much higher probability for a population of vials (compared to the probability to find the final stage of delamination). Therefore, we adjusted the number of vials to find early indicators with a high probability. If the probability to find a feature (e.g. reaction zone) for a single vial out of one time point is Y, we can calculate the probability P to find this feature at least once in a group of N vials with the equation P = 1-(1-Y)N, as shown in Table 3 below. It becomes clear that the reduction of the number of vials is significantly increasing the chance to miss a feature, which is why the aforementioned study design is recommended.
REFERENCES
1. FDA Guidance for Industry, “Container
Closure Systems for Packaging Human
Drugs and Biologics”, May 1999. 2. U.S. Pharmacopeial Convention (USP),
USP <790>, “Visible Particulates in
Injections”. 3. U.S. Pharmacopeial Convention (USP), USP <1660> Durability of Glass
Containers, “Evaluation of the Inner
Surface Durability of Glass Containers”. 4. European Union Pharmacopeia (EP),
EP 3.2.1., “Glass Containers for Pharmaceutical Use”. 5. Rothhaar, U., Klause, M., Hladik, B.
Comparative Delamination Study to
Demonstrate the Impact of Container
Quality and Nature of Buffer System, J.
Pharm. Sci. Technol. 2016, 70, pgs 560 – 567. 6. Haines, D. Scheumann, V., Rothhaar, U.
Glass Flakes: Pre-Testing Stops a Big Problem before it Even Starts, Contract
Pharma 2013, June, pgs 92 – 98. 7. Hladik, B., Buscke, F., Frost, R., Rothhaar,
U. Comparative Leachable Study for
Glass Vials to Demonstrate the Impact of Low Fill Volume, J. Pharm. Sci.
Technol. 2019, 73, pgs 345 – 355. 8. Perera, G., Doeremus, R.H., Landford,
W. J. Am. Ceram. Soc., 1991, 74, pgs 1269 – 1274. 9. European Union Pharmacopeia (EP),
EP 2.9.20., “Particulate Contamination
Visible Particles”. 10. Laboratories of SCHOTT pharma services are DIN EN ISO/IEC 17025 accredited (DAkkS) and FDA registered.
SCHOTT pharma services can access more than 40 years experience in analytical testing of pharmaceutical packaging containers. All quality relevant documents are electronically available, ensuring a hassle-free audit process.
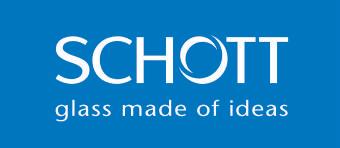
Dr. Daniel Haines
Scientific Advisor for SCHOTT pharma services, earned his doctorate in Inorganic Chemistry at the University of Chicago. He joined SCHOTT in 2001 with a focus on developing glass coatings to control drug formulation interactions with glass surfaces. Since 2010 he is responsible for SCHOTT pharma services in North America providing analytical support of packaging material for pharmaceutical companies.
Email: daniel.haines@us.schott.com
Laboratory address in Germany:
SCHOTT AG SCHOTT pharma services Hattenbergstraße 10 55122 Mainz Germany Phone: +49 (0) 6131 66 7339 pharma.services@schott.com Dr. Uwe Rothhaar
Director of SCHOTT pharma services earned his doctorate in Physics at the University of Kaiserslautern in Germany. He joined SCHOTT in 2000 and focused his activities on analytical support around glass and glass surfaces and coatings. Over the last years he is responsible for SCHOTT pharma services providing compatibility and compendial testing for pharmaceutical containers for pharmaceutical companies.
Email: uwe.rothhaar@schott.com
Laboratory address in USA:
SCHOTT North America, Inc. Attn. Dr. Dan Haines 201 South Blakely Street, #121 Dunmore, PA 18512 USA Phone: +1 570 457-7485 x 653 daniel.haines@us.schott.com Dr. Matthias Bicker
Scientific Advisor of SCHOTT pharma services earned his doctorate in Physics at the University of Goettingen in Germany. He joined SCHOTT in 2001 and was a key player in the development of new coating solutions for packaging containers, based on SCHOTT’s proprietary PICVD coating technology. Since 2005, he has continuously been working as a project manager on innovation projects for pharmaceutical packaging applications. Over the last 7 years, he has gained substantial experience in collaborating with the pharmaceutical industry in the area of characterization of primary packaging components aligned with most recent regulatory guideline recommendations and related analytical services supported by the contract laboratory SCHOTT pharma services.
Email: matthias.bicker@schott.com