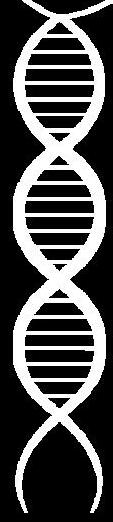
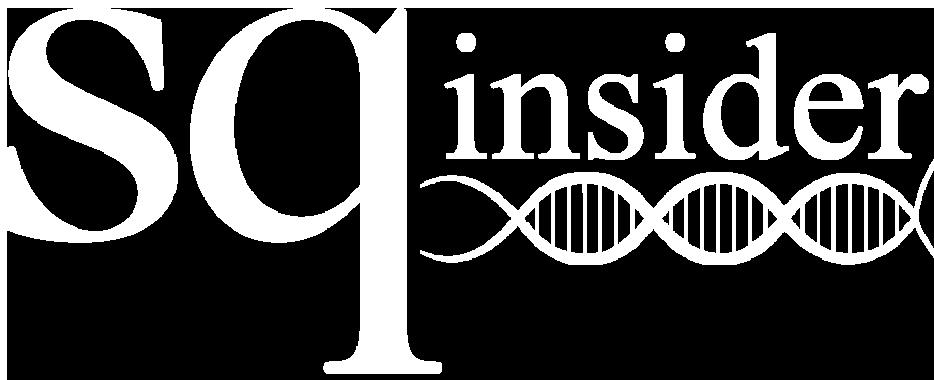
De(fying) Extinction
pg 2
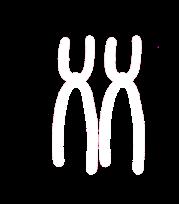
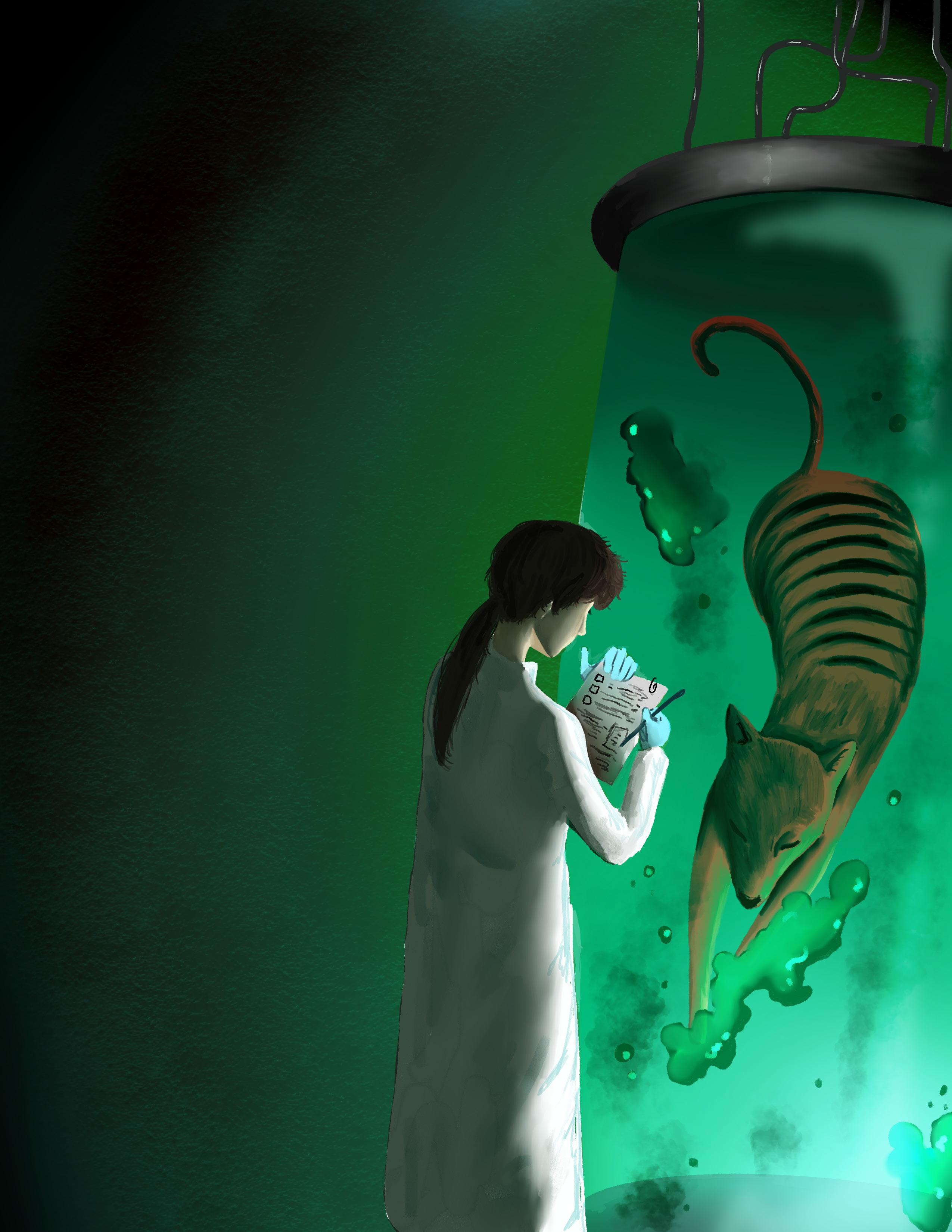
Bi-Maternal Reproduction
pg 3
High School Essay Contest
CRISPR in Medicine
pg 4
De(fying) Extinction
pg 2
Bi-Maternal Reproduction
pg 3
High School Essay Contest
CRISPR in Medicine
pg 4
written by: EMMA WONG photographed by: HARNOOR SIDHU
ecological niche.
Though no living organisms have been successfully brought back from extinction, there has been success on the molecular level. In 2022, Jacqueline Maasch and colleagues at the University of Pennsylvania used a machine learning model to identify antimicrobial peptides within the proteins of extinct Neanderthals and Denisovians, which are close relatives of humans. When these peptides were introduced into mice, they exhibited anti-infective properties, suggesting that molecular de-extinction can be used to develop new antibiotics.
The first ancient DNA (aDNA) ever sequenced came from the quagga, an extinct subspecies of the modern zebra. The Quagga Project, a non-profit organization, began using backbreeding to resurrect the species in 1987 and is one of several de-extinction efforts active today. One such effort that has attracted recent media attention is the work of Colossal Biosciences. Their website announces their first project: bringing back the wooly mammoth. But how do scientists plan to bring these ambitious projects to life?
There are three methods of de-extinction: cloning, backbreeding, and genetic engineering. The process of cloning inserts an adult somatic,
species. Close living descendants that display the desired traits of an extinct species are paired for mating. Like cloning, this method isn’t viable for many species since there must be living descendants. Additionally, successful backbreeding will result in organisms that share the phenotype, or observable traits, of the extinct species. However, they may not share the genotype of the species, casting doubt on how accurately these new organisms represent the extinct species.
The majority of modern de-extinction efforts use genetic engineering, since DNA lasts longer than cells. The first step is to sequence aDNA from an extinct species. Since aDNA is recovered in small fragments, it must be compared to a complete DNA sequence from a close living relative to ensure the fragments are in the correct order. The living relative’s DNA is known as the reference genome. Once the genome of the extinct species is assembled, scientists must determine which parts of the genome are linked to the target phenotype. Differences between the aDNA and the reference genome are a good place to start. Next, the aDNA is edited into the genome of the living relative. The invention of CRISPR-Cas9 makes this process easier than ever before. As defined by the National Human
the living Norway brown rat in 2022, around 5% of the genome was unrecoverable. These sequences were either incomplete or missing entirely. The missing sequences weren’t random—many of the genes with less than 90% completion were related to the immune response and sense of smell. Comparing the genomes of additional living organisms from the same genus—the taxonomic rank above species—as the Norway brown rat revealed that the genome recovery issues were due to evolutionary divergence. The shortness of aDNA fragments makes it difficult to map them onto the reference sequence if they are too dissimilar from one another.
Even with a completely reconstructed genome, an organism’s phenotype is often influenced by its environment. It’s impossible to know whether these gene-environment interactions are identical between the extinct species and the new offspring, though some people may overlook this issue if the organism can fill the ecological niche of the extinct species.
There’s another issue to consider: which extinct species should be prioritized for deextinction? It’s crucial to assess the impact that a resurrected species might have when reintroduced to its habitat. They might promote biodiversity, indicate the overall health of an ecosystem, or garner attention to protect that ecosystem. But they may also become invasive, bringing about predation, disease, or ecosystem changes. Furthermore, the extinct species’ ecosystem may have been transformed by climate change. There is understandably debate over whether the world is ready for newly resurrected species. But an equally important question is whether resurrected species are prepared for the world they will face.
written and Illustrated by:
LAN GAO
After all, an egg cell, just like a sperm cell, has a single copy of each chromosome pair. Theoretically, fusing two eggs would also yield a cell that contains two sets of each chromosome. If this technology were possible, then female-female couples could have children that are completely related to them biologically. Thus, the pivotal question is: Is it possible to take the chromosomes of one egg and inject its genetic material into the nucleus of another to create human offspring? The answer is yes–but only under certain conditions.
In 2004, geneticist Tomohiro Kono and his colleagues from Japan and Korea created the first viable mice born from a reconstructed egg that contained two sets of maternal DNA. To accomplish this goal, they first had to overcome several biological obstacles. The first hurdle was the difference in DNA between sperm and egg. According to Cold Spring Harbor Laboratory, some genes in eggs and sperm have differences in DNA known as epigenetic modifications which are chemical marks on the chromosomal structure that determine whether a gene is expressed or silenced. Thus, these genes are differentially silenced or expressed in sperm and egg. If two eggs fuse, then both copies of these genes could be silenced, and the fetus will have severe developmental problems. For example, according to Nature Education, Angelman Syndrome is caused by the loss of the should-beexpressed maternal copy of a gene on chromosome 15. To address this issue, researchers reconstructed the egg by transferring the nucleus of an immature egg, obtained from oneday-old mice, into a mature egg with its nucleus removed. Most of the genes in the immature egg are unmarked because the genes become epigenetically modified with chemical markers during the growth of the egg. The mature egg served as an environment for the chromosomes in the immature egg to separate without the cell growing and acquiring markers. After the chromosome separation, they put the DNA that remains in the immature egg into another mature egg with its genetic material. The unmarked genes from the immature egg replaced the function of sperm, providing the other half of the DNA and compensating for the gene expression if the copy of a gene from the mature egg is silenced.
The story did not end there: the second problem encountered by Tomohiro Kono and his colleagues was the resumption of the egg’s cell division. According to the journal Developmental Dynamics, an egg cell has not yet completed m eiosis, the division of reproductive cells, when it is released from the ovaries into one of the fallopian tubes during ovulation. Upon meeting a sperm, the egg orients and leads the sperm to bind with it. After binding, the egg
releases calcium ions (Ca2+) that resume its division. Hence, si mply combining the DNA of two eggs is not enough to generate viable offspring. Something else is needed to replace the sperm’s job of activating the egg’s division. The solution from Kono’s lab was to culture the reconstructed egg in a medium containing strontium chloride. The strontium ions (Sr2+) in the medium are similar to the calcium ions secreted naturally by the egg, so the medium can mimic the environment of fertilization and allow the researchers to artificially resume the cell division process of the egg, as stated by the National Institutes of Health. After Kono’s reconstructed eggs started to divide exponentially, it was transferred into the uteri of female mice.
Out of the 457 reconstructed eggs grown by Kono and colleagues, 371 of them were transferred to 26 female mice, of which 24 became pregnant. Although the researchers proved that certain reconstructed eggs could grow and develop in mice uteri, in the end, only 2 two pups survived with normal features. One of the bimaternal mice gave birth to several healthy pups, proving that bimaternal reproduction can generate healthy and fertile offspring. In 2018, the results of this research inspired stem cell biologist Bao-
yang Hu and his team from China to produce mice by fusing embryonic stem cells, cells cap able of becoming any type of cells, with not only eggs but also sperms. They made advancements in solving the ethical issue of getting immature eggs from newborn mice by inducing stem cells from the eggs and sperms of adult mice. They also improved the success rate of bimaternal reproduction with their best yield of 25 mice from 281 eggs transferred into uteri. This research not only brought humans more hope for the future of female-female reproduction, but it also shed light on the possibility that both females and males could reproduce without the opposite sex.
As humans delve deeper into the technology of bimaternal reproduction, we gain more insights into the intricate biological process of creating ourselves. The groundbreaking research on bimaternal and bipaternal reproduction challenges the physiological impossibilities of same-sex reproduction and aims to provide people of any gender identity with more reproductive choices. Despite this technology still facing the challenge of a relatively low success rate, the barriers can be broken by enthusiastic researchers who are willing to make the impossible possible, and maybe it could be you.
Enrique de Castro is currently a sophomore at Patrick Henry High School. He participates in extracurriculars including football, track, Model United Nations, and his school’s philosophy club. In his spare time, he likes to pet his two cats and workout with his friends. He is also a chronic procrastinator, and has still not completed his AP Computer Science assignment from two weeks ago. He is undecided about his college major, but plans on pursuing either pre-med or pre-law.
The reign of medical innovation conquers formidable beasts, completely eradicating diseases like smallpox while increasing the average lifespan tremendously. While our ability to combat medical problems has improved over the years, genetic disorders still hold a significant challenge in the field of medicine. If AP biology has taught me anything, it is that the central dogma of biology is the flow of genetic information from DNA to RNA to protein. This is why even a single point mutation in the human genome can cascade into a plethora of serious diseases like sickle cell anemia, cystic fibrosis, or hemophilia (Félix). Yet, with such a simple change, why is it so hard to cure genetic disorders? After all, DNA editing technology is already available in the medical field. For example, using restriction enzymes to insert a human insulin gene into plasmid can catalyze bacteria to produce insulin. The problem with curing genetic diseases is that it involves directly editing the human genome. Because of the central dogma, even a simple edit can have unpredictable effects in our bodily functions. It is imperative that we find safe and accurate methods to edit genomes.
Recently, the FDA approved a new type of treatment for Sickle Cell Anemia (Maron). This treatment was made possible using Clustered Regularly Interspaced Short Palindromic Repeats, or CRISPR. CRISPR is a series of repeating DNA sequences utilized in bacteria to combat viral infections. After being transcribed into RNA, it guides a restriction enzyme to the foreign viral DNA and cuts it before the virus can lyse. In 2012, researchers found a way to apply CRISPR technology to specific eukaryotic cells using the Cas9 restriction enzyme (Jackson Laboratory). The guide RNA utilizes a specific base sequence that directly pairs to the target DNA, allowing Cas9 to cut DNA at a specific sequence. After cutting the gene, the cellular repair mechanisms will replace it with a new
sequence (BioInteractive). With this new technology, it may soon be possible to safely cure genetic diseases without further damaging the genome. Additionally, CRISPR technology can combat other diseases like cancer. It can cut and disable genes in cancer-causing viruses or cancer cell RNA, or even enhance immune cells to fight cancer cells more efficiently (National Cancer Institute). The significance of this technology cannot be overstated. If we can manipulate DNA, we can unlock the full potential of living organisms.
Although the future of CRISPR is promising, it is worth noting that the treatment is not perfect. As mentioned before, even a small change in a DNA sequence can have unpredictable consequences on our bodily functions. It is imperative for the technology to be adequately tested. Additionally, possible complications could arise from the fact that CRISPR technology solely relies on the repair mechanisms of the cell. These are factors that could potentially delay the technology from achieving widespread availability for the foreseeable future.
Editors-in-Chief: Amoolya Chandrabhatta, Emily White
Executive Editor: Sharanya Sriram
Editor-at-Large: Chiraag Kambalimath
Head Technical Editor: Nicolas Bello
Head Production Editor: Jacqueline Cheung
Although there are several physical consequences to CRISPR, perhaps the most dangerous effect resonates on a societal level. CRISPR is not limited to curing diseases. In fact, individuals could use it to obtain desirable traits like intelligence or physical strength for themselves or their children. In my opinion, the defining characteristic of humanity is imperfection. Through our struggles, our spirit becomes indomitable and we gain the virtues of perseverance and discipline. If individuals are allowed to use CRISPR technology to obtain desirable traits, it could completely destroy our sense of humanity. Our diversity, our discipline, our imperfections, would be completely eliminated if we stray from using CRISPR as solely a medical treatment. Additionally, it could exacerbate inequalities around the world. According to the World Health Organization, more than 66 percent of the 120 million people affected by Sickle Cell Anemia live in African countries. Yet, the African continent is the least wealthy continent per capita. While wealthier regions could reap the physical and economic benefits of CRISPR, many developing countries would struggle to even obtain access. Therefore, the societal implications of CRISPR are twofold. It may save lives and prevent the devastation of detrimental diseases like cancer or genetic disorders, but it could also destroy our sense of humanity and widen economic barriers. As CRISPR continues to become more available, proper regulations must be set in place to prevent exploitative usage. We must be willing to ensure that CRISPR becomes a hope for a better future, rather than a disaster for the human race.