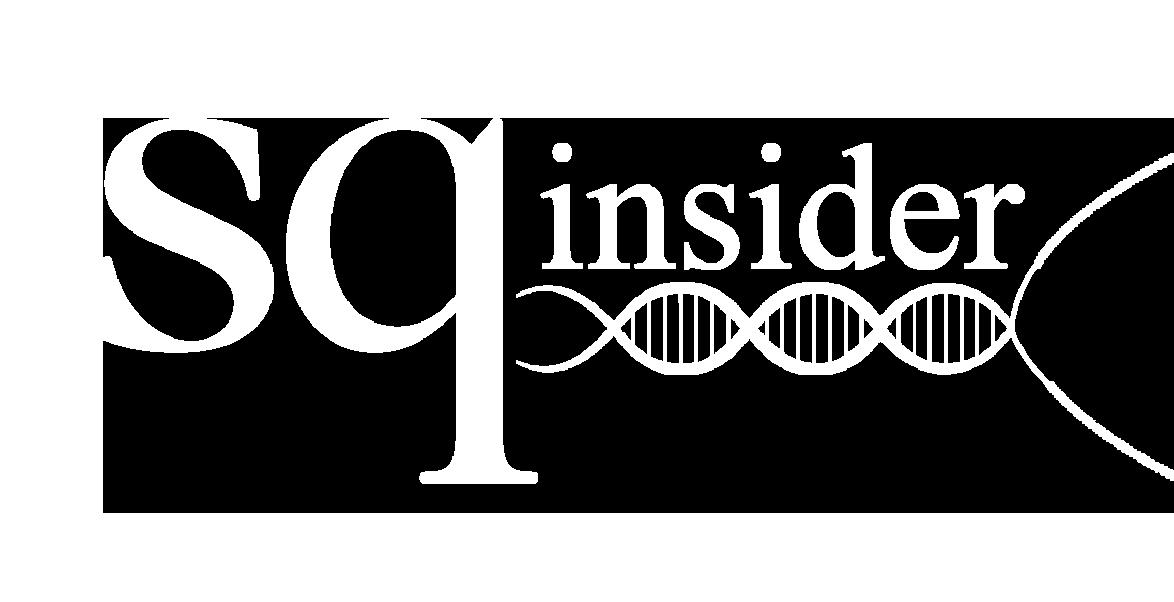
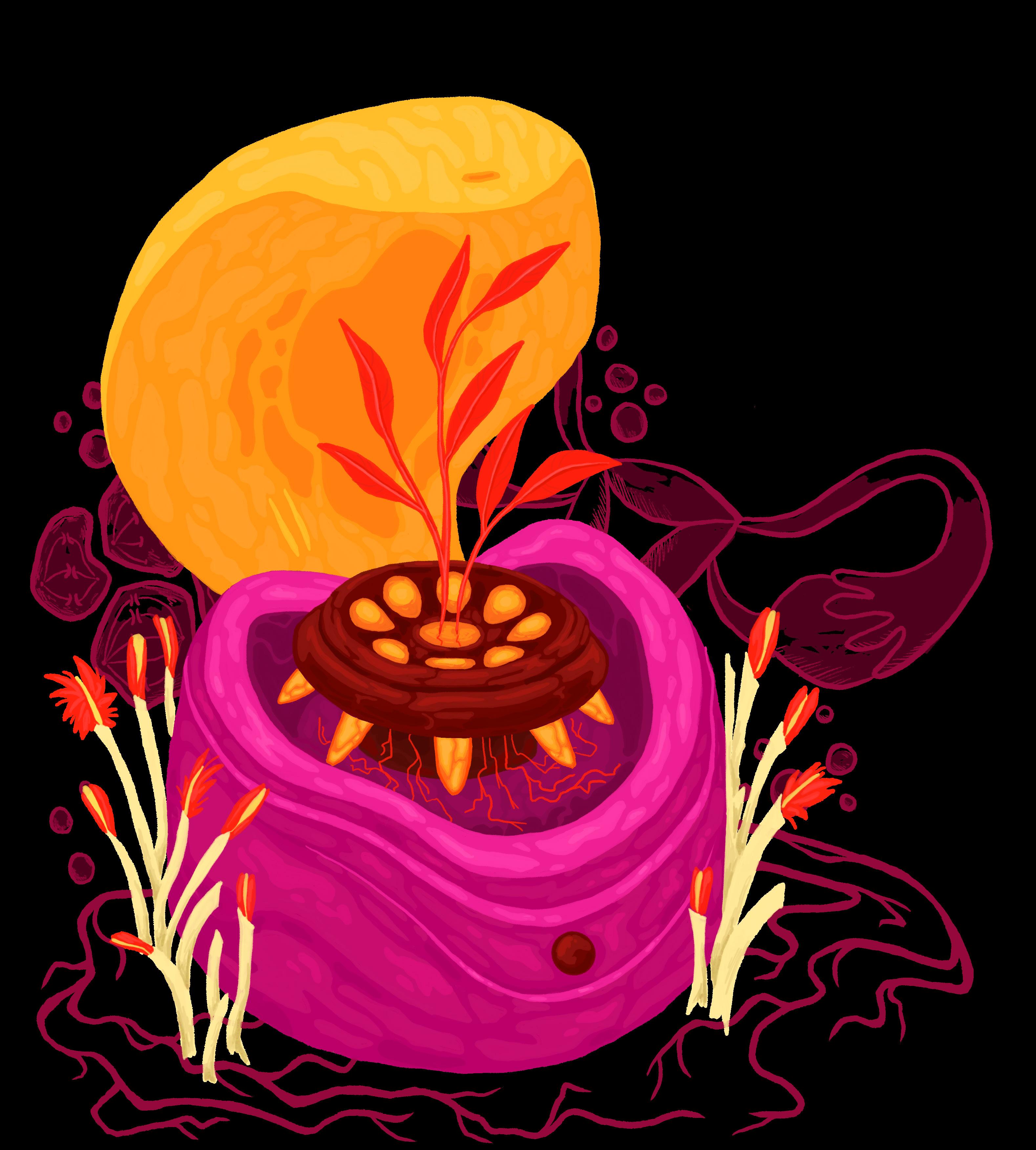
Cancer Pathways pg 2
Ovarian Cancer
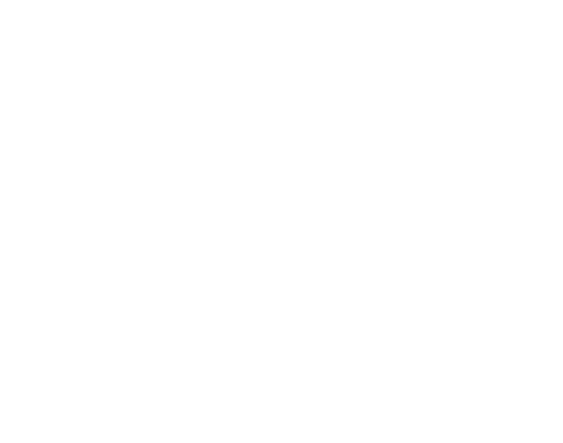
Tubeworms
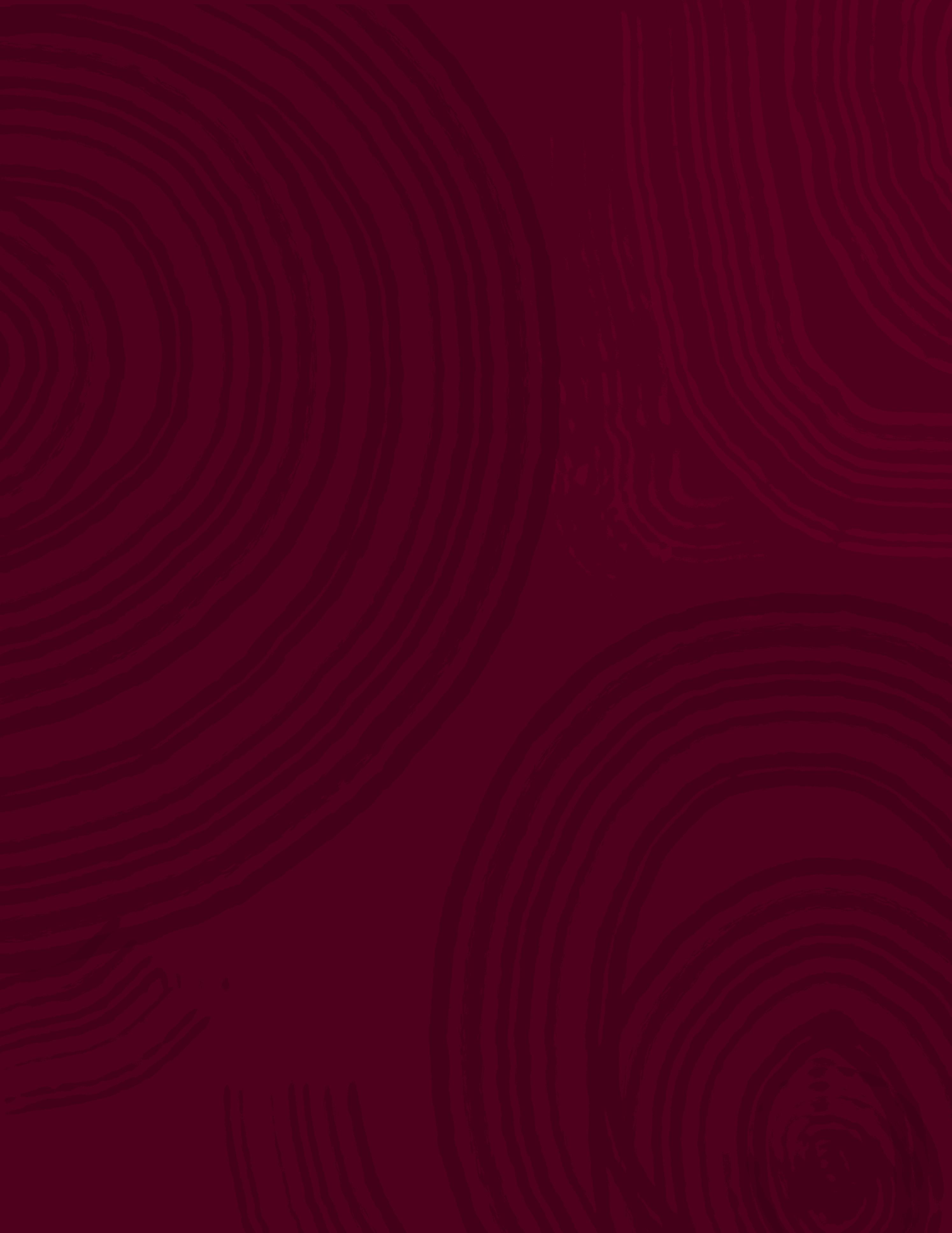
Cancer Pathways pg 2
Ovarian Cancer
Tubeworms
Sneaking and invading the surrounding tissue, hiding and taking over the body’s immune system, cancer cells are on a mission to thrive. These spy-like cells seek a constant source of oxygen and nutrients through blood vessels, or tubular structures that connect the flow of blood throughout our entire body. Blood vessels are easy-access pathways that allow cancer cells to spread and reach their ultimate goal of a total bodily invasion. But before they can accomplish this goal, cancer cells must first take advantage of the key process of angiogenesis: a cascade of steps that allows for the growth of new blood vessels flowing directly to the tumor and interconnection with the vascular system.
As I learned in Dr. Michael Burg’s fall quarter cancer biology class, the rapid division of cells begins with a build-up of mutations in genes that code for specific proteins, resulting in blockage of release pathways and causing various checkpoints and repair mechanisms to shut down. This creates an unstoppable replication process that produces more and more copies of aggressive, mutated cells, leading to tumor formation. When a tumor gains access to the circulatory system by rerouting the supply of oxygenated blood through various signals and functional molecules, it becomes malignant and prepares to spread through the bloodstream. This key step, characterized as angiogenesis, gives the cancer a way of reaching other organs to grow secondary tumors and new malignancies at distant sites (called metastasis). Without angiogenesis, the cancer wouldn’t be able to grow past a small, localized tumor.
Thus, modern cancer research focuses on effectively preventing progression to this stage. Over time, researchers focusing on angiogenesis became interested in a particular set of signaling proteins known as vas-
cular growth factors. In 1939, the first theory of vascular growth factors was proposed by researchers Ide, Baker, and Warren. Then, in 1945, Algire and Chalkley published findings in the Journal of The National Cancer Institute after experimenting with wound recovery to test whether tumor cells or normal vascular cells proliferate faster. They discovered that the mitotic rate and speed of proliferation of the tumor cells was much higher than those of normal cells, implying that the nutrients and oxygen essential for rapid cell division would need to come from the bloodstream.
In 1971, Dr. Judah Folkman discovered that tumors become dependent on their access to the vascular network. According to his paper, solid tumors are dependent on blood vessels to deliver them nutrients and oxygenated blood. His idea to block the factors responsible for the gain of blood vessels was refuted by many, but after receiving a grant for his research, he proved the existence of the protein he dubbed tumor angiogenesis factor (TAF), which is essential to the growth of tumors beyond their pre-angiogenic size of 1–2 mm. His research paved the way for more contemporary discoveries, such as that of the vascular endothelial growth factor (VEGF) protein, an essential signaling tool promoting blood vessel growth. Importantly, Folkman’s discovery of VEGF gave rise to investigations on anti-angiogenic proteins that keep the progression of cancer in check.
Having isolated the most important promoters of blood vessel growth, recent advancements in the field provide more targeted drug therapeutics that can be adjusted according to a patient’s specific diagnosis. One of the most commonly known anti-angiogenic drugs is Avastin, clinically known as bevacizumab. According to the National
Cancer Institute, Avastin can be applied to the treatment of various different cancers, making it a versatile treatment. This drug functions as an antibody that inhibits the VEGF from binding to receptors on the surface of cells, preventing VEGF signaling and the growth of vessels towards the tumor. In a tissue sample containing uninhibited VEGF, researchers observe a larger amount of blood vessels and bloody tissue. With VEGF inhibitors in place, the tissue would have the normal characteristics of vasculature, which would look more organized and significantly less bloody. As per the FDA’s treatment regulations for colorectal and ocular conditions, the applications of Avastin in modern treatment plans for many cancer types exist in the form of an injection or infusion, though other Avastin-equivalent VEGF inhibiting drugs are administered in other forms.
With any new treatment, there is always room for adjustment and modification. The adverse effects of anti-VEGF drugs are underresearched and open new sets of questions surrounding cancer treatment. Based on observations of current anti-VEGF therapies and a study on Systemic and Ocular Adverse Effects, known side effects include blood vessel leakage and the detachment of the retina, an essential component for vision. As for cancer treatment, Kamba and McDonald of the British Journal of Cancer claim that the long term consequences of suppressing signaling pathways create issues like vascular disturbances. Given the complexities of this disease, the future of treatments lies in personalized medicine, or the creation of cancer treatments more specific to patients. The field of cancer biology is ever-growing and leads us closer to mitigating the detrimental effects of cancer in each patient day by day.
A testimonial published by Johns Hopkins Medicine tells the story of a 65-year-old woman named Carol Johnson, who began feeling pain in her abdomen in September of 2009. She initially dismissed it as stress-related, but the discomfort remained for months. After surgery to remove what her doctor suspected was an ovarian cyst or diverticulitis, her surgeon informed her she actually had stage 4 ovarian cancer and if left untreated, she would only have six months left to live. Unfortunately, stories like Johnson’s are not uncommon; many undiagnosed women who raise their health concerns with their doctors are initially misdiagnosed and their ovarian cancer is caught later on, leading to poorer outcomes. Fortunately, Johnson qualified for a clinical trial and received the treatment she needed, but not all women are as lucky.
According to the American Cancer Society, ovarian cancer ranks fifth in deaths caused by cancer for women, higher than any other reproductive cancers. The CDC states that ovarian cancer is linked to the presence of mutated versions of the BRCA1 or BRCA2 genes. According to the Mayo Clinic, the symptoms might initially be invisible, but over time they may develop into abdominal bloating, weight loss, or changes in bowel movements. Ovarian cancer can be divided into epithelial cancers, which were thought to originate from the surface of the ovaries, and non-epithelial cancers.
While commonly treated with a combination of surgery and chemotherapy, ovarian cancer is so difficult to beat because it is often caught at later stages. Even after treatment, it has a high rate of recurrence. According to Mount Sinai, over 185,000 deaths are caused annually by epithelial ovarian cancer, and despite new treatments, there has been little change in mortality rates. After years of scientists and doctors attempting to create better treatments and earlier detection methods, they began focusing on previously proposed but unsupported theories that the mysterious origins of its pathology may lie beyond the ovaries, in the distant, thin fallopian tubes.
Only 11 to 12 cm long and less than 1 mm wide, the fallopian tubes (also known as uterine tubes) are a vital part of the female reproductive system. These thin tubes are a combination of muscular layers and a mucosal membrane and facilitate the movement of eggs from the ovaries, where the eggs are stored, to the uterus, where fertilized eggs implant and grow throughout pregnancy, according to the NIH. However, fallopian tubes play a much larger role in reproduction than just being a conduit. As described by Cleveland Clinic, the fallopian tubes use cilia, small hairlike structures that line the inner mucosal membrane, to propel the eggs out from the ovaries and meet the sperm. The sperm are pushed upwards by smooth muscle layers to meet at the area of the tube called the ampulla.
Despite the simple structure of the organ, anomalies, damage, or blockages in the tubes can cause a variety of issues, ranging from ectopic pregnancy (when an embryo implants outside of the uterus) to infertility. Scientists in recent decades have discovered that damages or mutations in the fallopian tube might extend into other regions, leading to ovarian cancer.
proteincoding regions of the genome and structural analyses of an individual’s tumors to verify their findings. The exomesequencing revealed mutations in the TP53 gene, a tumor suppressor gene that encodes the p53 protein known to be a driver of HGSOC. Immunohistochemistry, a technique to identify tumors by using antibodies and antigens, revealed that patients with the mutation in TP53 had no p53 identified on the surface of their ovaries, but had carcinomas and p53 signatures on the fallopian tube. The results suggested that serous tubal intraepithelial carcinomas (STICs), which are lesions on the fallopian tube, could be the origin of ovarian cancers for the majority of HGSOCs in women with these mutations, according to the National Cancer Institute. In other studies, scientists provided additional evidence that the fallopian tube is likely the origin of many HGSOCs regardless of the mutations in the BRCA1 and BRCA2 genes.
In 2017, a study from the Dana Farber Cancer Institute and Johns Hopkins Kimmels Cancer Center by Dr. Intidhar Labidi-Galy and colleagues traced the origins of high-grade serous ovarian carcinomas (HGSOC) in women with germline mutations in either the BRCA1 gene or the BRCA2 gene who also had their ovaries and fallopian tubes removed to decrease cancer risk. Scientists used whole exome-sequencing methods to sequence all the
Findings like these allow scientists and doctors to explore new avenues of beating ovarian cancer. Researchers at Penn Medicine believe that these findings could be used to identify potential preventative measures. They aim to learn how STIC lesions interact with surrounding tissue; identifying biomarkers could lead to earlier detection and more accurate diagnoses. Experts at Harvard Medical School suggest that these results provide evidence for different treatment options, such as removal of both the ovaries and fallopian tubes, or removal of the fallopian tubes alone. While removal of fallopian tubes may reduce the potential of cancer, it also has its own risks and consequences, like infertility. But, these recommendations are more attractive knowing that no current methods exist to diagnose ovarian cancer based on early screenings or symptoms, and only about 10-20% of patients are diagnosed before their tumors begin to metastasize, or spread.
Due to advancements in our understanding of the disease, deaths by ovarian cancer have been slowly declining annually. More precise research on the origins of the disease will allow for earlier detection and more targeted preventative measures to better help women manage their reproductive health.
In the eerIe depths of the east pacIfIc oceans... where sunlight cannot penetrate and pressures can crush even the sturdiest of submarines, a remarkable and enigmatic group of creatures thrive: deep-sea giant tubeworms. These animals survive the most extreme environments, colonizing the tops of hydrothermal vents and withstanding water temperatures of up to 700°F. Their existence is an impressive mystery that scientists are striving to solve.
According to the Monterey Bay Aquarium Research Institute (MBARI), giant tubeworms (scientific name Riftia pachyptila) can grow to a maximum length of 6.6 feet and are found in the deep waters of the East Pacific Rise and Galapagos Islands. Giant tubeworms live at depths of 6,200 to 11,800 feet in colonies clustered near hydrothermal vents, which can be considered underwater geysers on the ocean floor. As magma rises from the earth and cools to form new crust and volcanic mountain chains, the circulating sea water in the ocean’s crust is superheated to temperatures of up to 700°F, according to the National Oceanic and Atmospheric Association (NOAA). Due to their ability to survive in extreme conditions, giant tubeworms are aptly categorized by NOAA as extremophiles, or organisms that are able to thrive in conditions of extreme temperature, acidity, alkalinity, or chemical concentration. These giant tubeworms have developed fascinating adaptations to withstand extreme temperatures and pressures, toxic minerals, and lack of sunlight.
One such adaptation is the process by which the giant tubeworms create energy. The tubeworms, along with many other extremophiles, have evolved to convert mineral-rich hydrothermal fluid into energy through a process called chemosynthesis. As defined by NOAA, chemosynthesis is the production of food using energy released by inorganic chemical reactions. Through chemosynthesis, microbes inside the giant tubeworms generate food for the worm. This multistep process relies heavily on the tubeworms’ close physical proximity to the hydrothermal vents. First, hot water saturated with dissolved chemicals, such as hydrogen sulfide and carbon dioxide, gushes from the hydrothermal vents and enters the bodies of the tubeworms. Next, symbiotic bacteria inside of the worms absorb the hydrogen sulfide and carbon dioxide from the hydrothermal vent water as well as oxygen from the surrounding seawater. Third, the bacteria obtain energy by oxidizing hydrogen sulfide which then combines with carbon dioxide and oxygen to produce sugar, sulfur, and water, all of which the worms use as energy. The process of chemosynthesis is critical for the growth and reproduction of both the giant tubeworms and the symbiotic bacteria as it provides nutrients to both organisms.
Current researchers are interested in understanding the specifics of the unique biological processes inside tubeworms’ bodies, such as chemosynthetic symbiosis, and how these adaptations have aided the organisms in adapting to extreme environments. One study by Yale University researcher Yuanning Li in 2019 sought to explain how the tubeworms establish and maintain symbiosis with the chemosynthetic bacteria living inside of them. Li and his team of scientists used comparative genomics, transcriptomic, and proteomic analyses to explore the molecular pathways inside of the tubeworms. Findings show that the tubeworm genome lacks many of the genes linked to amino acid synthesis, suggesting that the tubeworms rely on the symbiotic bacteria for amino acids that are crucial to its survival. Thus, the bacteria inside of the tubeworms are not only responsible for creating nutrients, but also for creating amino acids that are essential for the tubeworms. Additionally, evidence of tubeworm digestive enzymes was found in trophosome tissue, referring to the middle structure of the tubeworms’ body where the symbiotic bacteria are housed. This discovery opens
the door to the possibility of a second nutritional mode in which the tubeworms digest the symbiotic bacteria to use as a supplemental source of energy. Li’s study as well as many others have indicated that the tubeworm anatomy and biological processes vary greatly from most other animals as a reflection of their extreme environment.
Aside from differences in genomics regarding amino acids, Li’s research also found differences in tubeworm genomics regarding hemoglobin, an iron-binding protein which transports oxygen in the circulatory system of many animals. For the giant tubeworms specifically, their genomic makeup includes an expanded portion of hemoglobin genes, which indicates that the hemoglobin in the tubeworms has evolved to bind oxygen and sulfide simultaneously from the environment and then transfer the molecules to the symbiotic bacteria.
From chemosynthesis to symbiotic bacteria to modified hemoglobin, deep sea giant tubeworms have developed impressive adaptations to withstand life in zones of extreme pressure, temperature, and darkness. These extreme conditions do put limitations on the extent of research; however, the environmental holdbacks do not diminish interest from modern scientists. Considerable research has gone into these organisms; however, there is much more to be discovered about these mysteries of the deep.
Editors-in-Chief: Amoolya Chandrabhatta, Emily White
Executive Editor: Sharanya Sriram
Editor-at-Large: Chiraag Kambalimath
Head Production Editor: Jacqueline Cheung
Production Ediitors: Dhathry Doppalapudi, Anasofia Vildosola
Head Illustrator: Kristiana Wong
Staff Illustrators: Christina Zhao, Leo Harris, Julia Dingman
Head Photographer: Harnoor Sidhu
Staff Photographer: Blake Riesenfeld
Head Technical Editor: Nicolas Bello