Undergraduate Research Magazine
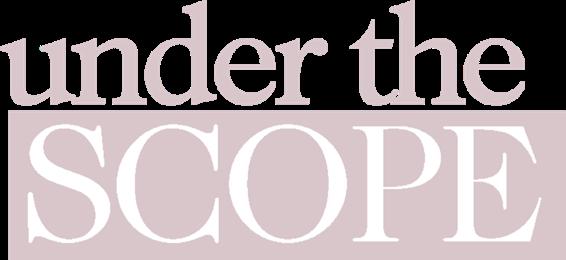
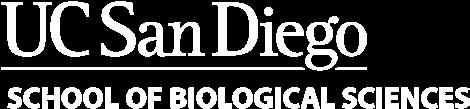
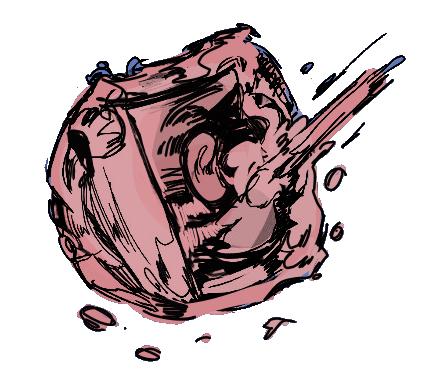
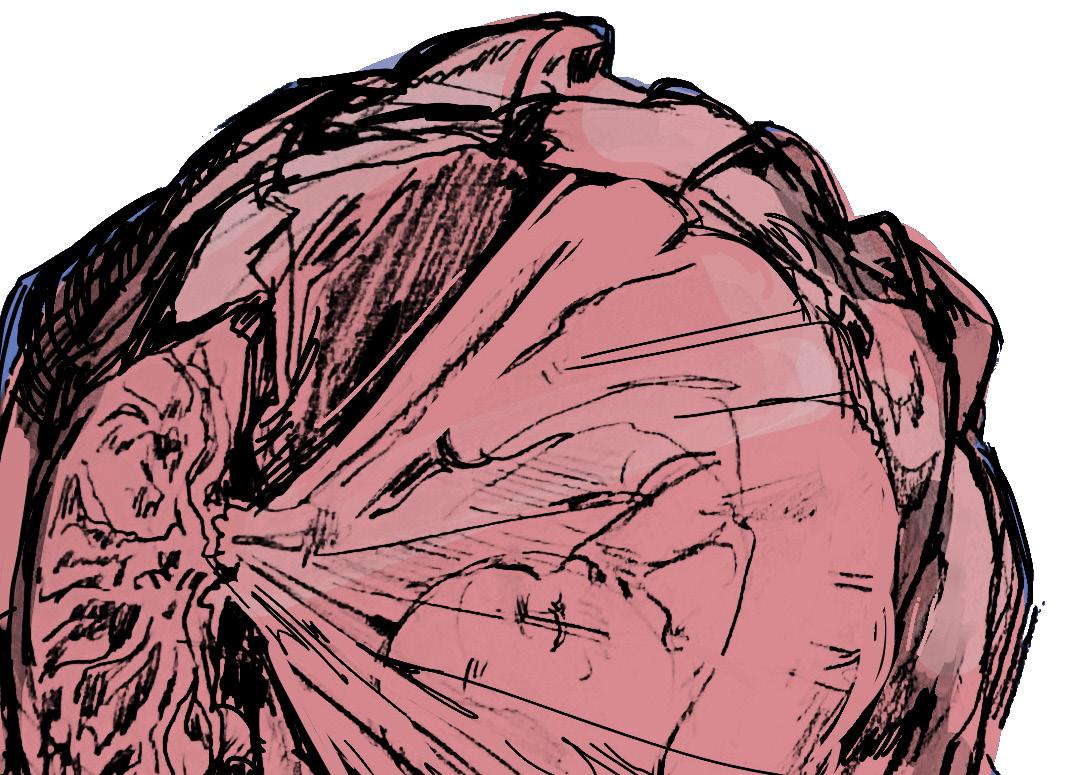
Undergraduate Research Magazine
Volume 13
2022-2023
or the past 13 years, Under the Scope has captured the essence of today’s bright minds in student research. Each article, written by a pair of staff writers, has undergone countless drafts meticulously revised by our technical editors and faculty editors before our illustration and production teams brought their words into the visual realm, creating the eye-catching spread you hold in your hands. The great care and attention given to each piece speaks to the need for accurate scientific reporting, without which the meaning of the research itself would be lost.
The features in Volume 13 outline the ups and downs of the scientific process as researchers advance long-standing methods with cutting-edge technology. Our cover story dives into new methods of monitoring factors that impact the viability of a fetus during embryonic development. As you read, you’ll learn about cutting-edge approaches in the Wilkinson Lab and Laurent Lab, which study RNA destruction in the blastocyst phase and the role of micro RNA in pregnancy-related conditions respectively. In the following articles, you’ll read about the cellular basis for autoimmunity, the influence of the external environment on biological decision-making, and how tight control of gene expression is essential to proper physiological function. Finally, you’ll learn about the promising field of algae-derived biofuel, which reminds us that some of the tools for a sustainable future lie within nature itself.
Our writing team unearthed these stories during a school year of great transition. Slowly, the time we spent on Zoom began to decline, and our meetings returned to an in-person modality. But in the final few weeks of fall quarter, we witnessed a bigger transition than we could’ve anticipated — a complete shift in what it means to be in research, following the largest
grounds. The stories told in Under the Scope have emerged from a changing landscape in science, in which the importance of graduate researchers’ well-being is finally recognized. We invite you to read about faculty perspectives on the current academic climate on page 26.
Despite the uncertainties of academia, both current and future, the ingenuity and dedication of student researchers to perfecting their craft has persisted. Similarly, what remains at the heart of Saltman Quarterly is our staff’s commitment to clear, concise, and accurate scientific communication in a time when honest scientific reporting is crucial to sorting out facts from fiction. We extend our heartfelt gratitude to all the writers, editors, illustrators, photographers, and designers whose hard work has resulted in this earnest publication.
We hope you enjoy Volume 13 of Under The Scope.
Sincerely,
Lina Lew & Sharanya SriramEditors-in-Chief
Lina Lew
Sharanya Sriram
Editor-at-Large
Nicole Adamson
Features Editor
Emily White
Production Editors
Tania Gallardo
Amber Hauw
Head Technical Editor
Nicolas Bello
Technical Editors
Gulshanbir Baidwan
Megan Hackbarth
Daniel John
Sarah Malko
Ishrak Ramzan
Irene Tsaur
Ariya Uyeno
Maya Wallace
Features Design Editor
Dhathry Doppalapudi
Head Illustrator
Kristiana Wong
Head Photographer
Bridget Spencer
Core Staff
Sevim Bianchi
Amoolya Chandrabhatta
Christina Do
Anna Hakimi
Chiraag Kambalimath
Soleil Madonia
Diana Presas-Ramos
Ava Selami
Roxana Shahmohammadi
Annika So
Syni Solanki
Megha Srivatsa
Caitlyn Truong
Head Advisors
James Cooke, Ph.D.
Assistant Teaching Professor of Neurobiology
Adriana Elaine Scott, M.A.
Student Engagement Coordinator School of Biological Sciences
Faculty Advisory Board
Elsa Cleland, Ph.D.
Douglass Forbes, Ph.D.
Sara Jackrel, Ph.D.
Ashley Juavinett, Ph.D.
Lisa McDonnell, Ph.D.
Alistair Russell, Ph.D.
Chih-Ying Su, Ph.D.
Illustrators Writers
Seher Basi
Amoha Bhale
Esha Bhattacharya
Zainab Fatima
Anshul Govindu
Kimia Habibi
Kitara Horning
Samantha Kawai
Emma Svendsen
Eventine Youngblood
Photographers
Andrea Farrell
Beatte Kim
Ava Bayley
Taryn Cornell
Lindsey Kim
Wirt Martin
Angel Williamson
Cover & Table of Contents
Illustrator
Hanh Nguyen
Photo by Andrea FarrellBY
Have you ever wondered why, on a hot summer day, you would find yourself reaching for a cool glass of freshly-squeezed orange juice rather than a mug of piping hot chocolate? Is it the weather? Is it the nostalgia of that one particular drink from your childhood? Were you influenced by your friend’s drink of choice? Several factors could have influenced your decision-making processes, including environmental stimuli, the body’s innate survival responses, learned behaviors from prior experience, and the social patterns you observe on a daily basis. At UC San Diego, the laboratories of Sreekanth Chalasani and Matthew LovettBarron seek to better understand what drives decision-making processes, and how various factors influence behaviors.
BY
At the Chalasani Lab, researchers Jessica Haley and Irene Chen study prey nematode species Caenorhabditis elegans, or roundworms, to understand how organisms pursue food and make survival-related decisions. To observe foraging strategies and mimic environments with varying degrees of food availability, the researchers place groups of C. elegans on separate plates with varying amounts of food. The worms are then left to acclimate to the specific food concentrations for a three hour training period. After this training interval, the C. elegans are transferred to a plate with lower food concentration — a “patchy” environment. Then, the researchers recorded the nematodes’ search pattern as they explored a new environment with less accessibility to food, in comparison to the training plate. According to their observations, C. elegans initially exposed to high food availability during the first training period searched a wider area of the foodless environment, while those with low food availability searched a smaller area. What is going on inside a worm’s nervous system that caused these results? The lab found that the C. elegans’ decision to explore farther for more food rather than settle for the most accessible patch is determined by dopamine, the neurotransmitter responsible for motivation. The dependence of foraging behavior on dopamine signifies that C. elegans internalize their observed patterns of food accessibility which triggers the release of
dopamine in new environments and, in turn, influences their search behaviors.
While dopamine drives reward-based decision-making in C. elegans another neurotransmitter may be involved in decisions surrounding survival instinct in more predatory species: octopamine. Pristionchus pacificus a predatory nematode, competes with the C. elegans for bacterial food, following a slightly different decision-making process. To model the strategic value of predation for the P. pacificus nematode, the Chalasani Lab used previously established neuroeconomics and foraging theory. The theory dictates that predatory foraging strategies involve exploration and an offensive strategy with the motivation being food as a reward, whereas territorial foraging strategies involve a more defensive approach. For example, the P. pacificus nematode is motivated by predation when it bites larval C. elegans However, biting an adult C. elegans, which are more difficult to kill, is considered a territorial defense of bacterial food. This difference in motivational approach is mediated by the previously mentioned octopamine (invertebrate version of norepinephrine) acting as both a hormone and neurotransmitter. The multifaceted messenger plays the key role of increasing and maintaining blood pressure and is released when the body enters a “fight-or-flight” response. Therefore, octopamine is significant in guiding the nematodes’ switches between predatory behaviors with prey and territorial behaviors in defense of bacterial food.
The Lovett-Barron Lab examines decision-making from another approach: by focusing on how sharing information through social interaction impacts behavior. To study these connections, the undergraduate researchers, John Jefferson and Shefali Gupta, worked with postdoctoral fellow Lisanne Schulze to monitor changes in brain activity from states of passiveness to alertness, hunger, and stress, using Danionella translucida, or glassfish. Glassfish are ideal subjects for this study because they exhibit social behaviors such as moving in groups, or schools, and are mostly adaptive due to visual cues. Conversely, in humans or mice, the process of sensation and behavioral modification is complicated by additional abilities of touch and smell. To analyze the neural activity of D. translucida the Lovett-Baron Lab utilizes two-photon calcium imaging to record brain activity while the transparent fish experience the illusion of moving and schooling from a virtual reality simulation. In two-photon imaging, a green fluorescent protein extracted from jellyfish is engineered to glow brighter in the presence of calcium, and thus indicate which areas of the glassfish brain are most active in reaction to stimuli. Because neurons are activated by calcium flooding the cell during action potential, the glassfish injected with the green fluorescent protein exhibit the artificial green glow when their neurons are active. By analyzing the GCaMP (a genetically encoded calcium indicator) signals through microscopic imaging, the lab maps out which areas are most active during each glassfish interaction. This information is then used to identify what genes are expressed for each behavior exhibited; live-brain volumes with activity information are compared with fixed brain-volumes using patternmatching algorithms. Because the goal of this lab is to understand how animals make decisions as a cohesive group, this method of gene comparison with simpler organisms is useful to identify how social interactions and visual decision making evolved. Through these experimental methods, the Lovett-Baron Lab monitors the neural activity of individual glassfish after exposure to certain visual cues in order to find recurrently expressed genes. The goal is to ultimately determine possible links between these
.common genes and observed behaviors, such as escaping and eating, carried out by the other glassfish. The lab hopes to find how groups of animals become more social through development, as well as determine how groups of animals are able to escape from predators and hunt prey in a more effective way than individuals alone. Through the experiments conducted thus far, they found that no glassfish took the initiative of engaging in more risky behaviors or venturing out further than the others. Instead, they found that no fish ranks above the others, with the group moving as a cohesive whole and changing direction in direct response to one another. This process is analogous to the broader patterns found in highway traffic: no individual car causes the traffic, yet the interactions between each car impedes overall movement.
The research conducted by these two labs suggests that decision-making behavior is impacted by a variety of factors, including environmental conditions, learned survival patterns, and social visual cues. Environmental inputs and visual cues are some of the sole influences on decision-making behavior of these nematodes and glassfish. Humans, while subject to the same influences, also have other senses such as sound and smell that complicate decision-making. While more research needs to be done to fully understand complex decision-making, it remains clear that our surroundings directly influence our decisions. So the next time you reach for the orange juice over the hot chocolate, consider reflecting on how your decision was influenced by the environment around you.
Overall, it is safe to say that environmental input triggers the release of various neurotransmitters that contribute to decisionmaking in invertebrate nematodes.P. pacificus nematode biting larval C. elegans, demonstrating predatory foraging behavior P. pacificus nematode, in yellow, displaying territorial and defensive foraging strategies by biting adult C. elegans
In humans or mice, the process of sensation and behavioral modification is complicated by additional abilities of touch and smell.
Imagine passing through security at an airport — how does security prevent unauthorized passengers or prohibited objects onto the flight? Officers check boarding passes at multiple points, metal detectors screen each person before entry, and upon arrival, customs screens for unwanted goods again. In other words, a multi-tiered security system creates a nearly impenetrable barrier against potential threats. Our body has a similar network of defensive measures: the immune system. One tier of this system consists of T cells, which are cells that screen potentially dangerous entities like viruses or bacteria. But what happens when the body’s security systems make a mistake and detect threats where none are present?
This results in a phenomenon known as autoimmunity (or selfimmunity), where T cells attack the wrong target: our own tissue. However, there is no reason to worry just yet as our body has a second tier of defense. This includes regulatory T cells (Treg cells) that distinguish and filter out malfunctioning T cells from properly functioning ones. By dampening the attacking instincts of T cells, Treg cells prevent an ‘overreaction.’ Unfortunately, this secondary procedure can fail, causing rogue T cells to fall through the cracks.
For the 24 million U.S. citizens affected by autoimmune conditions, this prolonged internal assault has significant health impacts. One of the most common conditions resulting from this is Rheumatoid Arthritis (RA). Treg cells play a particularly essential role in regulating RA, since they control how hyperactive, or T cell-intensive, the body’s response is. This can either be pro-inflammatory (hyperactive) or anti-inflammatory (“nonhyperactive”). In RA, defective Treg cells skew the response towards hyperactivity. As a result, the action of rogue T cells leads to inflammation, causing stiffness, discomfort, pain, and even loss of joint function.
What can be done to remedy the situation? Preventative measures have failed, so it is important to brainstorm ways to minimize harm. This is what drives researchers in the Zheng Lab, at the Salk Institute for Biological Studies, and the Ay Lab, at the La Jolla Institute for Immunology, as they look to pinpoint specific genes and molecules that contribute to the stability of Treg cells. By
essential damage control process.
The first step towards a solution is identifying the cause. The Zheng Lab research focuses on the which is critical for the development and function of Treg cells. The FOXP3 gene appears to act as an “on-off” switch, deciding when T cells or Treg cells are deployed. This introduces fascinating possibilities, as wielding power over this “master remote” would provide unprecedented control over the body’s immune response. The Zheng Lab previously demonstrated the link between FOXP3 and Treg cells. When PhD researcher Tianyun Hua removed FOXP3 from mice Treg cells using gene therapy, they observed a more hyperactive immune response in these mice. This suggested that removing FOXP3 leads to fewer or dysfunctional Treg cells, which established FOXP3’s the optimal Treg cell potency. Furthermore, the study explained how FOXP3 maintains balance within the body’s immune system by encoding proteins that determine when, where, and how many Treg cells are present at a given time.
In order to determine the actual transcription factors or coding regions associated with FOXP3
performed a genome-wide analysis in Treg into mouse
T cells, the lab individually knocked out, or removed, parts of the genome to observe the functional changes in the immune system. They discovered that the absence of a specific proteincoding section called the “ncBAF complex” reduced Treg cell activity, making the ncBAF complex a
With this information, researchers can better treat the Treg cell imbalance in RA. With a precise target to manipulate, researchers will be able to increase Treg cell functionality, which might lead to novel treatments against this widespread autoimmune disease. Overall, by identifying the source of the problem, the Zheng Lab lays the foundation for future research that works
and led to increased severity of RA symptoms in the affected mice. Mice without the gene for PTPN2 died earlier than those containing the gene, indicating that loss of function of PTPN2 in Treg cells enhances autoimmunity by causing the FOXP3 proteins to become less stable.
While the Zheng Lab studies the regulation gene, the Ay laboratory at the La Jolla Institute for Immunology focuses on the molecular FOXP3.
The Ay Lab studies the expression of proteins that contribute to the stability of Treg cells. Specifically, PhD researcher Joaquin Reyna studies PTPN2, an enzyme that resides inside of cells and contributes , therefore maintaining
To test the role of PTPN2 in Treg stability, gene in mice and treated them with compounds that induce autoimmunity, which led to destabilization of the PTPN2 protein. This significantly reduced the expression of the PTPN2 enzyme
What would happen if the mice had a non-autoimmune form of arthritis? To test this, researchers in the Ay Lab transferred human serum that lacked PTPN2 into mice with non-autoimmune arthritis, also known as osteoarthritis, which is caused by the mechanical wearing down of joint cartilage. The researchers found that the lack of PTPN2 in mice with a non-autoimmune disease did not change the disease progression! However, when they transferred this PTPN2-negative serum to mice with autoimmune arthritis, they observed a rise of pro-inflammatory T cells that caused a significant increase in the disease progression. Based on these experiments, Reyna and their team established an association between the loss of the PTPN2 intracellular enzyme and increased progression of rheumatoid arthritis. This research propels future research to focus on how PTPN2 is regulated, and how it functions in autoimmune vs. nonautoimmune forms of arthritis.
Studying autoimmune diseases like Rheumatoid Arthritis presents dozens of intricate views on how the body’s vital defenses can malfunction and cause collateral damage, creating a multitude of questions researchers still have yet to answer. However, the incredible work done in the Ay and Zheng Labs have provided hope for patients in the future. While researchers like Hua and Reyna have identified and extensively studied specific mechanisms involved in autoimmune processes, understanding the role of Treg cells in the immune system is only the first essential step towards developing autoimmune therapies that target these specific genes and pathways. For the countless people across the globe who suffer from autoimmune conditions, continued research and progress towards future treatment options hopes to improve the function and balance of the body’s personal security system.
As a result, the action of rogue T cells leads to inflammation, causing stiffness, discomfort, pain, and even loss of joint function.
No matter where we end up in life, everyone begins at the same place: as a developing embryo. We all transform from cells to the complex organisms that we are today, yet this process contains numerous potential complications for both the parent and child-to-be.
Despite advancements in healthcare, a 2020 study done by the Centers for Disease Control shows the United States has the highest maternal mortality rates compared to similarly developed countries, like New Zealand and Canada. It is clear that more attention needs to be focused on the health of the parent and child.
During pregnancy, the health of the embryo and parent are notoriously hard to monitor. Whether it be fluctuations in developmental milestones, impacted development due to environmental conditions, or inconsistent methods of monitoring parental health between pregnancies, it is clear that concrete methods of evaluating health of parent and child are needed. At UC San Diego, the Wilkinson Lab and the Laurent Lab seek novel methods of monitoring embryonic development and pregnancy complications.
Bayley WRITTEN BY ILLUSTRATED BY Kitara Horning and Emma Svendsen AvaWhile embryonic development centers the creation of life, one of its most important factors is the exact opposite: destruction. As embryonic cells replicate and differentiate rapidly during development, the cells must eliminate possible mutations before they can harm the embryo. But what mechanisms enable this kind of quality control during development, and what is the scope of their impact? The Wilkinson Lab at UC San Diego focuses on just that.
The first stage of development, the blastocyst stage, is vital to future embryo survival. Blastocysts are fertilized eggs composed of two different kinds of cells: the trophectoderm, outer cells that eventually become the placenta, and the embryoblast, inner cells which eventually become the embryo. As these two cell types develop rapidly, they rely on the cell’s ability to correctly produce a protein from a DNA sequence. Typically, cells produce proteins by first reading and transcribing a section of DNA into a complementary strand of messenger RNA (mRNA), which is read by ribosomes and translated into a protein. However, if the produced mRNA contains errors, so will the protein, leading to a range of disorders or possible non-viability of the embryo. To regulate the quality of proteins produced, cells use a process known as nonsense-mediated RNA destruction (NMD). NMD identifies and degrades incorrect mRNA through a pathway of proteins and regulatory factors, preventing malformed proteins from being produced and potentially harming development.
As an MD/PhD student researcher in the Wilkinson Lab, Dr. Jennifer Chousal explored how malfunctioning NMD pathways impact embryonic development. Using a mouse model, Dr. Chousal and her team created “knockout embryos” by removing UPF2, an integral protein of the NMD pathway, from the cell lines. After culturing these knockout cells and waiting for maturation into blastocysts, Dr. Chousal and her team performed outgrowth assays, which measure proliferation and success of cell lines, to evaluate the development of both the outer trophectoderm and the inner cell mass. The assays revealed that, while the trophectoderm remained unaffected, the inner cell mass was unable to develop. These results indicate that despite a successful implantation of the embryo into the uterus and successful placenta formation, the blastocyst would not develop further, making the
embryo non-viable. Dr. Chousal’s team found higher levels of programmed cell death upon further investigation of the knockout embryos. Additionally, they experienced difficulty deriving stem cells, suggesting that tissue development would not have been successful in this embryo as stem cells are required to specialize
As the embryo develops, cells are constantly rearranging to form the framework for a new, complex organism
To gain a better understanding of NMD’s role in embryonic development, Dr. Chousal’s team took a specific look at when NMD is most active. They measured levels of RNA degradation throughout several stages of development in healthy embryos. The embryos were observed from the blastocyst phase to the gastrulation phase, in which cells rearrange in layers to prepare for tissue and limb development. Dr. Chousal’s team found a decrease in mRNA degradation levels following embryonic implantation, but found that these levels increased during differentiation of the gastrulation phase. Known factors of the NMD pathway, such as specific proteins like UPF2, followed this trend with low levels during implantation and higher levels during gastrulation. Embryos with normal NMD pathways had both types and levels of expressed proteins that matched those of known, properlydeveloped embryos. In contrast, the knockout embryos did not reach this phase of development and thus did not have levels of RNA measured.
The work of Dr. Chousal and the Wilkinson Lab makes one point clear: the ability to properly regulate mRNA and its related proteins is essential for proper embryonic development. However, even if an embryo develops properly through these milestones, other factors can impact the viability of a pregnancy that are equally difficult to monitor. The Laurent Lab looks to tackle one of the most daunting: preeclampsia.
Preeclampsia is a condition affecting about 4% of pregnancies, causing high blood pressure, an increased risk for organ damage during pregnancy in the parent, and an increased risk of seizures after birth. Traditionally, doctors look for indicators of preeclampsia by examining blood pressure, analyzing the amount of protein in the parent’s urine, or looking for a previous history of high blood pressure. However, these external indicators are inconsistent between pregnancies, leaving researchers to question if better signals exist within the body. The Laurent Lab investigates this problem further, looking towards microRNA as a solution.
MicroRNA (miRNA), or small segments of RNA, specifically inhibits gene translation, which prevents the synthesis of certain proteins. miRNAs bind to mRNA through complementary base pairs, thereby preventing the mRNA from being processed
by ribosomes. Researchers at the Laurent Lab believe that dysregulation of miRNA could potentially predict preeclampsia.
As an undergraduate researcher, current master’s student Cassandra Wauer examined miRNA from extracellular vesicles in mouse urine samples, searching for miRNA segments that could be linked to preeclampsia. She and her team focused on these vesicles due to the critical role they play in intercellular communication, and chose to sample urine due to its ease and lack of intrusion for the subject. Wauer first prepped the urine samples to prevent breakdown of the sensitive miRNA. Following preparation, she processed the samples, performed a quality check using a bioanalyzer to ensure miRNA viability, and entered the data into a larger database. Specifically, Wauer looked for already-identified RNA sequences associated with preeclampsia, and compared previous data to her experimental results of which mice developed preeclampsia.
The results were inconsistent and did not yield what Wauer and her team were searching for. However, she currently works to change the experiment’s focus in the hopes of yielding clearer results. MicroRNA may be too sensitive to be reliable, but perhaps the same information could be gleaned from mRNA or other small RNA molecules. These more resilient molecules could expedite the experiment, as they would allow Wauer to process more than the original experiment’s mere 16 samples in a day. Despite these setbacks, this work presents a promising opportunity for the health of pregnant people, and fine-tuning the specifics may lead to impactful results.
While birth and its related processes are natural and integral to life, efforts are needed to improve the health of parent and child. From monitoring embryonic development to finding early treatment for devastating conditions like preeclampsia, these predictors could benefit people who are pregnant for the first time with no known risk factors. The work of the Wilkinson and Laurent labs is critical in addressing this issue, suggesting that the key to treating potential complications may be found in everyday cellular processes and molecules, like NMD and miRNA. As labs work to advance reproductive science, there becomes a greater opportunity to ensure successful and healthy delivery for all.
You’ve set out to bake the perfect cake. For the last hour, you’ve flipped through numerous recipes in your cookbook, with each cake recipe unique in its own way. Just as there are different recipes for cake, a similar diversity exists in your own genome. Each part of your genome serves a particular purpose, such as cellular functions or protein construction, all of which come together to form the most complex and interesting cake of all–you!
To identify all the processes that create organisms like yourself, it’s important to understand the central dogma of biology. As the basis of genetic expression, it allows the conversion of endless streams of DNA into the physiology, development, and functionality of your body’s genome, formulating the basis of evolution at the species level.
But how do these fundamental components and processes lead to the expression of genes, or sequences of DNA, that form the basic units of inheritance? In the Kadonaga Lab at UC San Diego, research associate and recent graduate Torrey Rhyne studies the DNA core promoter in eukaryotic organisms in order to better understand its role in gene expression. The core promoter is a stretch of DNA that directs the initiation of gene transcription by acting as a recognition site for binding of RNA polymerase to DNA. RNA polymerase transcribes, or converts, the DNA into messenger RNA (mRNA), providing the body with readable templates to make functional proteins that are important to the structure, function, and regulation of the body.
Within the core promoter of some genes, a short DNA sequence containing many thymine and adenine nucleotides, known as the TATA box, further guides the initiation of transcription, indicating where DNA can be read and decoded. However, the TATA box is found in less than 25% of the core promoters within the human genome, leaving many scientists puzzled as to how human cells direct transcription.
At the Kadonaga Lab, Rhyne and her colleagues generated and examined a vast database of core promoters across the human species, each containing differing sequences in the region where the TATA box is primarily located. Upon inserting the promoter sequences into HeLa cells, human cells that can be rapidly cultured and used for in-vitro experimental models, these promoters then helped drive the transcription of stretches
of DNA, called downstream core promoter regions (DPRs). Using machine learning techniques, the researchers assigned transcription scores to each DPR sequence by comparing the scores they obtained experimentally to known values stored in the computer database. These transcription scores quantify DNA transcription and thus gene expression by measuring the mRNA levels within each cell. Analyzing this data, they concluded that DPRs containing vast repetitions of guanine, a specific nucleotide base, had higher transcription scores than those without guanine nucleotides. In other words, the DPRs containing these specific guanine sequences exhibited a higher frequency of transcription, and thus a greater likelihood of gene expression to occur, compared to DPRs of differing nucleotide sequences. Likewise, RNA polymerase appeared to have a higher affinity toward these DPRs, therefore increasing the likelihood for transcription initiation to occur. With this information, the Kadonaga Lab was unable to discover universal core promoter elements. While the TATA box may function as a core promoter element in one organism, the DPR may act as a critical core promoter element in another, as commonly seen in humans.
The digit development in mammalian limbs, starting from the initial paddle formation and progressing into separated digits through the processes of controlled expression of BMP4 through MSX2 regulation.
Overall, identifying core promoter elements such as the DPR helps scientists understand the transformation of DNA to functional proteins. Applying their research to modernday issues, the Kadonaga Lab hopes that their contributions to understanding gene expression will advance theory behind the molecular mechanisms of complex genetic conditions, allowing researchers to trace its pathology back to the DNA level.
Just as a cookbook provides the basic instructions and ingredients for a cake, the central dogma of biology lays the foundation for the transformation of the information coded in DNA into the structures and functions needed to construct an organism.
The transcriptional process of reading DNA to form biological structures is as essential as understanding the recipe when baking. Like altering the amount of ingredients
that can transform the cake, changes to the regulation of transcription can impact how the DNA is read and the organism is formed. Discovering its intricacies is essential to many areas of research, including the mechanisms of mammalian development. UC San Diego’s Cooper Lab studies a specific instance of this phenomenon, where Ph.D candidate and student researcher Alex Weitzel contributed to studying the interdependent MSX2 and BMP4 genes that enable the growth and positioning of limbs and digits.
During fetal development, the body starts from scratch and differentiates cells into features such as limbs. To direct this growth the body applies particular mechanisms, one of which is the gene regulation studied by Weitzel which allows for digit construction. Initially, a “paddle” formation is created, where digits are connected by lines of cells. He found that to achieve the ideal separated digits, the BMP4 gene is expressed in a specific pattern between future digits. The BMP4 gene is responsible for apoptosis, or cell death, allowing it to remove the excess cells between digits. A second gene, MSX2, is also required to ensure this process happens correctly. MSX2 is an upstream activating sequence which enhances the transcription of surrounding genes such as BMP4. The expression of MSX2 along the same patterns between digits also increases BMP4 expression in those areas, making sure only necessary cells are removed.
The Cooper Lab recognized that the MSX2 and BMP4 mechanisms were not always part of mammalian development, since our ancestors had paddled limbs, yet their role has evolved as most mammals today have separated digits. Genetic evolution
occurs over time through accumulated changes in a species’ collective genome, like generations of bakers adding unique flairs to their recipes. The Cooper Lab identified a method to delve into this aspect of evolution: the jerboa animal model. Jerboas have radically different front and hind limbs. Their hind limbs are much larger and skinnier than the front limbs, which have standard rodent anatomy. The animal model allows researchers to compare the genetic evolutionary changes that have led the jerboa to form different front and hind limbs, while also eliminating confounding factors that arise between different species. The front limbs undergo standard limb development, as the Cooper Lab expected, but the hind limbs modify this process to better suit their unique structure. Studying the evolution of genes and their function can help us better decipher the complex processes that construct diverse structures, a prime example being the separation of digits and the formation of different limb structures. Grasping genetic evolutionary developments will help scientists understand the significance of each individual recipe within the overall cookbook of mammalian development.
The multifaceted role of genetics begins with DNA transcription, aided by core promoters, eliciting the first step in gene expression: using information from a gene to synthesize proteins or aid in cellular processes. The following interactions between different genes are essential to the body’s function, such as limb development, and evolutionary changes, as evidenced by the jerboa. Everywhere you look, genetics holds a considerable influence. Interactions between your genome and the world is what makes you, you — and that is as perfect as any slice of cake.
When the COVID-19 pandemic swept the nation, many commercial industries neared collapse, while biotechnology companies like Pfizer and Moderna capitalized to push for rapid development of their respective vaccines. From developing pharmaceuticals, vaccines, and even cleaning supplies, the biotechnology industry plays a crucial role in developing a wide range of commercial products from various biomolecules. However, as research, development, and demand for these products increase, so do worries of the industry’s environmental sustainability. As a response to these concerns, researchers and governments alike are looking to maximize production of sustainable bioproducts and biofuels with hopes of eventually replacing unsustainable sources of energy.
In a major collaboration, UC San Diego’s Shurin Lab, Los Alamos National Labs, and the United States Department of Energy look towards algae as a sustainable alternative biofuel. As one of the most utilized organisms in biotechnology, algae is cultivated and typically utilized as a source of pharmaceutical and nutraceutical compounds such as biolipids, beta carotene, and chlorophyll. These compounds can be used directly as alternative energy sources and play a significant role in the development of other bioproducts.
At UC San Diego, PhD student Ugbad Farah studies ecology and sustainability in Dr. Jonathan Shurin’s laboratory. Farah began studying moss variation in extreme environments as a master’s student at Cal State Los Angeles, where she discovered that environmental factors such as elevation, precipitation, and distance significantly altered the appearance of the same species of plant. With this experience, she proposed a question: does adaptation to environmental variations yield measurable differences in the raw production of biomolecules? Inspired by this question, Farah continued her research career in the Shurin Lab with an emphasis in biofuel production and sustainability in the field of biotechnology. She now works as a leading scientist on various algae projects including the Department of Energy collaboration.
In her primary project, Farah studies the directed evolution of algae populations. Directed evolution describes the phenomenon in which researchers guide the evolution of a population by tailoring environmental conditions over an extended period of time to understand how these conditions influence evolution. In her research, Farah studies whether evolution of algae under different conditions impacts the bioproducts it produces. To achieve this, Farah cultivated and utilized a controlled strain of algae, Nannochloropsis oceanica for 16
Algae can be cultivated under specific conditions to rapidly multiply and form colonies. These colonies can then be harvested to extract valuable biomolecules.
experimental groups, each with different treatments. This species was selected for its rapid reproduction cycle, as populations tend to double in as little as 19 hours. This rapid reproduction allows for changes in the phenotypes, or physical characteristics, of the algal populations, to be observed and compared between the 16 groups as they respond to different temperatures, carbon dioxide levels, nutrients, and lighting. After many generations, Farah plans to analyze each population to see how they change from their shared ancestor. Based on any observable and quantifiable changes, she can recognize trends in density, growth rates, fatty acid content, and size.
Farah’s research with algal evolution helps companies understand how to maximize algae for commercial use in biofuels. But once grown, how else can algae be applied for commercial purposes? The Mayfield Lab at UC San Diego’s California Center of Algae Biotechnology uses genetic engineering to explore different applications of algae in the production of nutritionally dense algae and biopolymers, or large molecules in biological systems synthesized from smaller repeating units.
As a PhD student at the Mayfield Lab, Crisandra Diaz researches how to genetically synthesize nutritionally dense
algae with higher lipid and protein content. To induce mutations within algae strains, she uses techniques called UV mutagenesis and selective algae breeding that aim to create mutations with more lipid and protein content. In UV mutagenesis, an oven exposes individual colonies of microalgae to UV light that damages the DNA and forms mutations within the algae. The algae strains proceed through sequencing to identify which strains contain the most desirable mutations for lighter lipid and fat content. After identifying the mutations, Diaz and her team perform tests involving characterization and sequencing to determine which algae strains can breed together, in hopes of optimizing the breeding of algae with higher lipid and protein content. By maximizing lipid and protein content in algae, genetically modified algae can act as a food source in low-resource environments to help combat the global hunger crisis. Algae can act as a sustainable alternative form of nutrition in low-resource environments, due to its ability to regrow in the same area of water and take up less energy and land to grow than other food sources.
In addition to research, Diaz and the Mayfield Lab also synthesize a sustainable alternative to polyurethane plastics known as algal-based bioplastics. In most commercial uses, such as insulation, adhesives, and car manufacturing, polyurethane is made from petroleum and other less sustainable sources, creating a need for developing methods from more sustainable sources such as algae.
The Mayfield Lab first derived algal polyurethane foam from compost and passaged the foam to a carbon-free media to make
the foam the only carbon source. After passaging and discovering bacterial colonies on the foam, the researchers sequenced the proteins from these bacterial colonies and tested if the bacterial enzymes break polyurethane bonds. The ability to break polyurethane bonds is an important feature for these bacterial enzymes since it allows the degradation of polyurethane plastics. To test whether the bacterial enzymes can break the bonds in polyurethane, the researchers used a fluorometric assay, where they mixed together the bacterial enzyme and the substrate with polyurethane bonds. The mixture then emitted a fluorescent light, signaling the breaking down of bonds in the substrate by a specific bacterial enzyme. The researchers repeated this process with other bacterial enzymes and other substrates with polyurethane bonds to compare the most biodegradable product. The research done on algae-based polyurethane foam is significant in improving the breakdown of algal-based polyurethane plastics. The algal-based polyurethane foam can use algal bacterial enzymes to break down further into smaller monomers. Monomers are small repeating units that can interact to form a larger molecule such as a polymer. These monomers can reform into a polymer that resembles the original foam structure,
creating a bio-recyclable loop. By breaking down into monomers and rebuilding the molecule, algal-based polyurethane plastics can be recycled and still maintain the structural integrity of the original plastic product. This method is an improvement from other plastic recycling methods, such as heating or “mashing” that weakens the recycled product to a lower structural integrity than the original product.
Algae research provides a promising new avenue for introducing environmentally-friendly materials and products to the biotechnology industry, with applications in many areas of life. Algae can be used as a sustainable form of energy through the production of algae-based biofuels. Algae can also be used in other applications such as generating nutritionally-dense food or biodegradable plastics. The varied applications of algae in different sectors of the biotechnology industry open new opportunities to improve the environmental impact of current materials, food, and energy sources, and through continued research and development, algae will play a key role in the creation of a more sustainable future.
Based on the quantities of biomolecules extracted, conditions are then modified and applied to algal populations. These populations are then cultivated and compared to see which conditions offer optimal biomolecule harvests.
What are some of the pros and cons of going into academia and research?
In Fall 2022, the UC San Diego undergraduate campus slowly returned to an in-person class modality. Students’ day-to-day schedules jumped out of Zoom and Google Calendar and into packed lecture halls and small-group discussion sections often led by graduate teaching assistants. Yet behind the scenes, negotiations between the UAW academic worker union and the University of California reached a tipping point, resulting in the largest academic worker strike in history. Protests, chants and signage surrounding fair pay for academic workers and graduate student researchers were found on nearly every corner of campus. Across each UC school, people at all levels of education were asked to consider the current well-being of researchers in academia.
With discussions disrupted and classes canceled, UC San Diego undergraduate students were left wondering: What does my future in research look like? In this interview feature, we asked three UC San Diego biology faculty to help us tackle this question.
There is an inequality that is present throughout our society across all different sectors of the economy that has been making its way into academia for a long time, and the humanities felt it first, but it’s now getting to the sciences, and that is pushing a trend that is frankly very worrying.
I think the way society claims to value those things is different from how much society is willing to pay for [research beyond the sciences]. There is an inequality that is present throughout our society across all different sectors of the economy that has been making its way into academia for a long time, and the humanities felt it first, but it’s now getting to the sciences, and that is pushing a trend that is frankly very worrying.
Any advice for prospective grad students interested in pursuing a career in teaching/research?
I always encourage people, especially between the undergraduate level and the subsequent master’s or PhD, to take a break in between and really consider what you want to do, why you’re doing it, who you want to do it with, where you want to do it, all these basic questions. Get some experience, because you need to take a breath.
What were your thoughts/takeaways from the union strike last quarter?
I felt very sad for the faculty to have students that were feeling that way, that they were feeling like they couldn’t have an honest conversation with people. At the same time,
I feel like the entire system (and I think we all feel this way now) is just not equipped to respond to that financial challenge. I think that was a quick short-term solution that sets a precedent, which it’s not clear how sustainable that’s ever going to be in the long-term, because inflation will keep happening and prices will keep going up.
As a relatively new faculty member on campus, what are your outlooks on the post-pandemic career prospects of academia?
I think that in-person interactions are just so important to being engaged with what you’re trying to learn and getting excited about it, and that experience is just not the same, even if you can conquer technology. Maybe there should be positions that center around remote learning, and those individuals should be trained and equipped to teach remotely as opposed to us that teach in-person trying to do both.
What advice would you give someone who is uncertain about entering academia?
If people are constantly telling you that’s impossible, you’re gonna get bogged down and dragged down by that. But if you set out to do something and didn’t know how hard it was, and then you end up doing it, well then great, right?
Dr. Shermin de SilvaGiven your previous graduate school and current teaching experience, what is your take on the strike and graduate student situation?
I saw a lot of change in San Diego while I was [in graduate school]. When I got here, [graduate housing] was a pretty affordable option, given our stipend. Then the price of graduate housing changed, and the housing market changed, my costs of living went up, and now my rent gets raised as much as it possibly can every year according to the law. I don’t think you can support equity in a situation where people cannot meet their costs of living. I don’t think there’s anything that, in good faith, argues against the idea that for someone to be successful, they need housing security and the ability to support themselves. By virtue of the way that academic research is funded, slow movement is built into that system. More dollars must go through an entire governmental process for more dollars that arrive at the institutions that pay students. It’s transparent to me that graduate students need to make an income that, at the very least, covers their basic costs, and the situation isn’t tenable when that’s not the case. It seems to me that the strike was basically necessary when it happened. There is a lot of change within academia right now regarding what it means to be a graduate student and a postdoc, which is being renegotiated in important ways. Ultimately, and perhaps unfortunately, it will come down to the kind of funding we can secure from the government.
The costs of IAships to the university has increased quite considerably. The current, and perhaps changing situation is that UCs are not going to increase funding to make up for what will now be a loss of IAs in classes. We could be looking at a very significant decrease in the number of IAs in our classrooms,
which strikes me as a bad thing. I think there is a general problem right now regarding what institutions like UCSD want to do with and for their students and how much funding there is, or should be, to do those things. Helping students learn to work with their peers, problem-solve, and tackle authentic questions is, to me, integral to a decent education. I understand the need for 400-person classes, but I think that the saving grace of those large classes was the possibility of small group work in sections. At this point in time, I feel like we’re all the way from undergraduates to postdocs where there’s just not enough funding to do the things that institutions are supposed to do, and that’s a detriment to everyone, especially folks who are already disincentivized from being in the sciences or who don’t feel welcome in them. I think there needs to be an agreement amongst all of us that we’re here to achieve a certain thing together, and we’re not going to accept austerity and a lack of funding and accept a degraded version of education and teaching and research because it doesn’t suit a governmental bottom line.
What would you change or would like to see in the coming years for academia?
One thing that I think is gaining some traction is an additional emphasis on mentorship and an openness to different outcomes. If you go back to the 20th century, 50-60% of people who go to grad schools are starting labs. The pipeline of academia into academia was very strong, but now that’s quite different. Now, it’s like 15-20% of people are making it from academia into academic research professor positions.
The question now is, is everyone going to accept a system where agreeing to take that step [into academia] means that young people have to live through 5-10 years of precarity? Or, is everyone going to agree that what’s happening here is valuable and put more money into the research that people love to talk about, like the cure to cancer? Somewhere along the path, people have to develop the hard-earned skills necessary to ask those research questions, know what the cutting edge of a field is like, and network with people who can develop the right ideas. That network, a lot of it, is happening at universities.
I think the honest truth is, you can do either and be fine. One thing I try to recommend is to take a couple of gap years. Not only are you going to have a couple of years of making money and getting off the treadmill and cycle of continuous midterms, but you’re going to give yourself some time to make an informed decision and be a better applicant. I would say it’s less of a question of
The question now is, is everyone going to accept a system where agreeing to take that step [into academia] means that young people have to live through 5-10 years of precarity? Or, is everyone going to agree that what’s happening here is valuable and put more money into the research that people love to talk about?
Dr. Matt Flaggbiotech vs. academia than it is about, “Do you want to take a gap year or not?”
It can also be good to be in a few lab contexts. Arriving at graduate school is 2-6 rotations where you’re going to try to make a big decision about the lab. It’s going to have a pretty serious effect for the next 5-10 years of your life, and having good antennae for what a happy lab looks like is a hugely beneficial thing to have in your pocket.
Any last motivational words for anyone planning to pursue research?
Be reasonably self-defensive. If a situation seems bad, do your best not to imagine that you’re going to be the exception to the rule. If someone, an advisor, seems bad to their people, assume they’ll be bad to you and get out of there. Be kind to yourself, and pursue a good advisor over prestigious research. We’re seemingly at a point where, regardless of the university you go to, if you can make it through a Ph.D. program, you’re a very desirable hire for biotech. When you orient yourself within the academic landscape, choose the place that seems most capable of being supportive of you and what you need. Try to resist the urge to go to the prestige university and find the prestige lab that publishes in the prestige journals. That tends to be like getting a job at a Michelin-star restaurant, these places where your badge of honor for being at a prestigious lab is supposed to make up for your horrible quality of life. It’s not worth it. There are good, fulfilling lives and careers that can come from an advisor who is like, “I care about my grad students, we publish where we publish, and you’re here to learn how to do research in a way that’s about your growth.” That’s possible if you prioritize it.
What was your journey into academia? What was most instrumental or important to you?
My journey is an unusual one. I’m an immigrant, so I have a two-part journey — the first part was as an undergrad student and PhD student back in Argentina, which was my initiation into academia. The systems are very different. The one thing that is emphasized in both places is education. In the very early stages of your training, you have to get involved with teaching and education of undergrad students. The requirement of instruction in a PhD program, for example, or even as an undergrad student — I was also a TA as an undergrad student — starts nurturing your passion for helping and teaching others to be part of this big enterprise that higher education is. And of course, there is also the research component, which I’m really passionate about as well.
I think it’s really important is to have good mentors. That’s something I always tell students here. Make sure that you choose a lab you enjoy going to, and where your mentor fulfills your expectations as a mentee. There are of course multiple mentoring styles, and multiple mentee styles, and I think it’s up to mentors and mentees to figure out whether they are a good match. It’s a bidirectional relationship. Having good mentors, mentors that support and understand you, is probably one of the most important things for career and development of passion for your work. It’s a really critical decision that sometimes people overlook.
Especially in the very early stages, anything you learn is going to be useful. Even the things you don’t like will be useful, because at the very least you know what you don’t like, and that can be as helpful as knowing what you like. You know what to look for afterwards. When you are an undergrad, or even at the PhD level, my suggestion is to look for the type of guidance or mentorship you’re going to get wherever you go, rather than the specifics of what you’re studying. Then as you move on, especially after you finish your PhD and you decide whether to continue in academia, you’ll have to start thinking more about what you really like in terms of your research topic. Some mentors will really train you well and develop you as a researcher or as an instructor, so as you move on to the later stages, you can choose more specifically the area or topic you would like to study.
I think that really reflects my journey in academia. I got the right mentorship. I was in a small lab when I was a PhD student, with lots of interactions with the professor running the lab, even daily interactions. We would work on the bench together sometimes. Especially with undergrads, I still make myself available and work with them on the bench to teach them techniques at the beginning. And I think I do it, in part, because that has been my experience as an undergrad and grad student.
Then I came to the U.S. around 20 years ago, which was very different in many ways. I changed from a small lab to a big lab that had 30 or so members,
I changed languages from Spanish to English, and I changed organisms — I did my PhD studying soil bacterium that can degrade environmental pollutants, but here I started working in plants and circadian clocks. I had not been trained in plant biology or chronobiology before I came to the U.S. for my postdoc. But in terms of the independence that I needed to work in a large lab, I had the right foundations, so I didn’t find it too challenging to transition. In the long run, it was very advantageous. If you think about how long it took me to transition, yeah, maybe it took me a couple of years to get adjusted to all these changes, but in the long run it gave me a much broader perspective. Although I didn’t work with plant pathogens in my PhD, I worked with bacteria, and in my postdoc I worked with plants specifically, and in my lab now we are using bacterial pathogens to study how the clock is influenced by bacterial pathogen infections. So in a sense, I could bring a little bit of that previous expertise into my work.
Are career prospects in research the same now compared to when you started?
I think nowadays, what I see is that in the younger generations, there’s a better understanding of the many career paths you can take when you decide to do research. Academia is certainly one path, but there are many options in industry, or even in legal matters like patents. As you develop yourself as a researcher, if you’re in the right place, maybe what you thought was an academic path might change because you might realize ‘This is not what I like.” In institutions like UC San Diego, you have many opportunities to explore those alternate paths. There are many workshops and informational events where you can get in contact with people that took Career Path A, B, C, or D, and that can spark your interest in taking a different direction.
Figuring out what you’re passionate about can be very difficult. In the long run, if you can discover that thing which you enjoy and really love, that’ll be the main driver of whatever you do. That is something that is very difficult to teach. You can nurture passion and love for research, but there is something that is innate to each person that makes you more passionate about certain things. If you can find that passion, that will really, really ease your path into whatever you want to do. I feel like I’ve figured that out.
Figuring out what you’re passionate about can be very difficult. In the long run, if you can discover that thing which you enjoy and really love, that’ll be the main driver of whatever you do. That is something that is very difficult to teach. You can nurture passion and love for research, but there is something that is innate to each person that makes you passionate about certain things.
Dr. Pruneda-Paz