FACILITIES: AA SCHOOL OF ARCHITECTURE
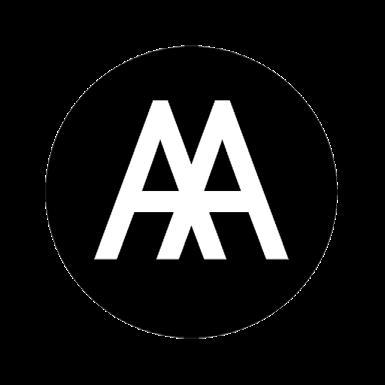
Refurbishing the City : London Building Case Studies
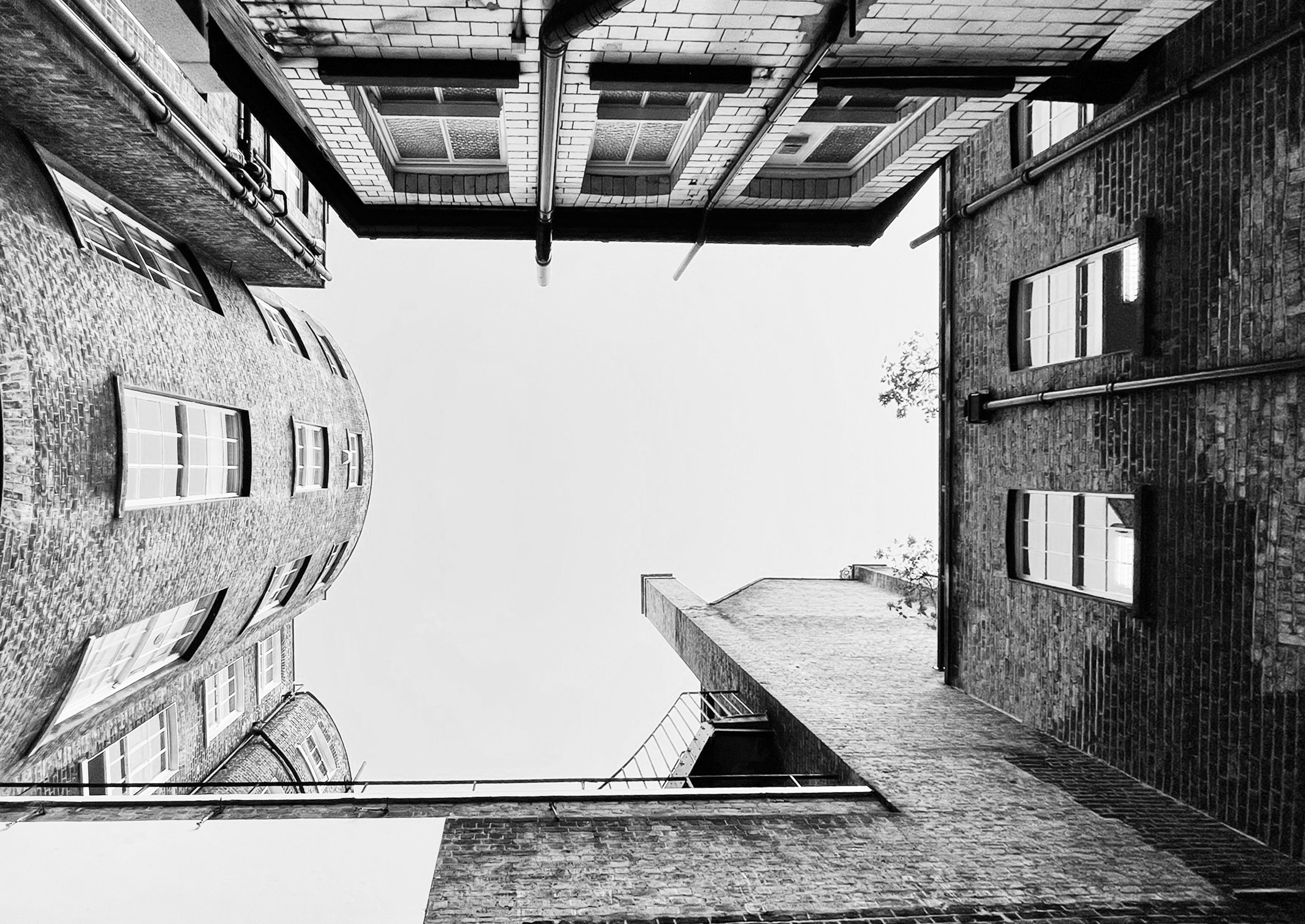
FACILITIES: AA SCHOOL OF ARCHITECTURE 11,182 words
“I certify that the contents of this document are entirely my own work and that any quotation or paraphrase from the published or unpublished work of others is duly acknowledged.”
12th January 2022
The team would like to thank everyone who contributed to the accomplishment of this project.
First of all, we would like to express our gratitude to the facilities manager: Anita Pfauntsch and her team for their invaluable support for collaboration, occupant survey and technical information that they provided us with on the project. The team would also like to express appreciation to the AA Teaching Staff, especially to Simos Yannas and Paula Cadima for the significant tutorials and the guidance on the project all these months. We are also extrmemly
grateful to the rest of the faculty and staff members of the Architectural Association School of Architecture’s Sustainable Environmental Design programme; Nick Baker, Byron Mardas, Gustavo Brunelli, Herman Calleja, Joana Gonçalves, Jorge Rodríguez and Mariam Kapsali for their valuable guidance and feedback throughout the project.
This report is the outcome of Building Studies project of the MSc - MArch Sustainable Environmental Design program, 2021-2022. The spaces studied are located inside the Architectural Association School of Architecture. The buildings of the school consist of Old Georgian and Victorian houses that have been modified over the years according to the space required by the school and its occupants. The specific site for this report was the Basement of 33 Bedford Square - The Facilities Area. This included the Facilities Office, Courtyard and Facilities Workshop.
The study consists of observations, surveys, measurements and analysis based on factors affecting the environmental performance and comfort of the spaces. Computational simulations were done to explore the correlation between the built urban form and the environmental quality and performance in and around a building.
From the initial stages of the study, some key parameters of the spaces provoked questions regarding fundamental environmental problems. These problems were carefully documented, measured and analyzed - and they became the backbone of our research methodology which concludes with design propositions for the spaces.
1.INTRODUCTION 11-13 1.1.THE BRIEF
1.2.THE METHODOLOGY
2.OVERVIEW 15-21 2.1.LOCATION................................................................................16 2.2.SITE STUDY
2.3.MATERIALITY
2.4.HISTORY....................................................................................20
3.CLIMATE ANALYSIS 23-25 3.1.THE CLIMATE CHART
3.2.WIND | SKY COVER | ILLUMINANCE
4. OUTDOOR AND INDOOR STUDIES 27-61 4.1.SHADOW ANALYSIS
4.2.CANYON SECTION
4.3.BASE CASE ........................................................................................30-39 4.3.1.Sun and Wind Study
4.3.2.Thermal Studies...............................................,......................32 4.3.3.Daylight Studies
4.4.ANALYSIS PERIODS
4.4.1.Sun and Wind Study
4.4.2.Thermal Studies
4.4.3.Daylight Studies
4.5.INFERENCES.................................................
4.5.1.Courtyard.....................................................................55 4.5.2.Office..........................................................................56 4.5.3.Workshop..........................................................................57 4.5.4.Thermal Studies: Summary.......................................................58 4.5.5.Daylight Studies: Summary.......................................................59
5.TECHNICAL STUDIES 61-77 5.1.THE METHODOLOGY
5.2.THE ELEMENTS
5.2.1.Courtyard
5.2.2.Windows
5.2.3.Thermal Mass
Fig 1.1.1: Illustrated Section through a Bedford Square Georgian Home 12
Fig 1.2.1: The Methodology Flowchart 13
Fig 2.1.1: Aerial view of the Site 16
Fig 2.2.1: Occupant, Lighting and Appliances Layout 18
Fig 2.2.2: Occupants Survey 18
Fig 2.2.3: Interior views of the ‘Office’ 18
Fig 2.2.4: The central ‘Courtyard’ 18
Fig 2.2.5: Interior views of the ‘Workshop’ 18
Fig 2.3.1: Section AA’ through the three spaces 17
Fig 2.3.1: Sectional Perspective through the Spaces 19
Fig 2.3.2: Material Composition 19
Fig 2.3.2: Plan 17
Fig 2.3.4: Sectional Elevation 21
Fig 2.4.1: Plans of a Typical Georgian Home at the Bedford Square 20
Fig 2.4.2: Sketch of the Georgian Homes at the Bedford Square 20
Fig 2.4.3: A typical Georgian Facade 21
Fig 2.4.5: Front Elevation with Interior Construction 21
Fig 3.1.1: Location of the Weather Station used for the Project: St. James Park Weather Station 24
Fig 3.1.2: Analysis of the London Climate Chart and establishing the two Analysis Periods 24
Fig 3.2.1: Wind Rose for Analysis Period-I May to Aug (left) and Analysis Period- II Nov-Feb (right) for London 25
Fig 3.2.2: Pie-chart illustrating frequency of sunny, intermediate & cloudy skies annually 25
Fig 3.2.3: Monthly maximum global illuminance & mean global illuminance for sunlight hours for London 25
Fig 4.1.1: Shading Mask 28
Fig 4.1.2: Shadow Analysis of the Site 28
Fig 4.2.1: The Canyon Section of the Courtyard showing the various sun angles 29
Fig 4.2.2: The times of the year when the sun directly reaches the Courtyard 29
Fig 4.3.1.1: Solar Radiation for the Base Case Period 30
Fig 4.3.1.2: Solar Access for the Base Case Period 30
Fig 4.3.1.3: Wind Direction for the Base Case Period 31
Fig 4.3.1.4: Wind Direction for Base Case Period in Plan for the Site 31
Fig 4.3.1.5: Wind Speed through the Site in Section 31
Fig 4.3.2.1: Dry Bulb Temperature 32
Fig 4.3.2.2: Relative Humidity 32
Fig 4.3.2.3: Comparison of Air Temperature & Relative Humidity in Section 32
Fig 4.3.2.4: Surface Temperatures in the Office 33
Fig 4.3.2.5: Surface Temperatures in the Courtyard 33
Fig 4.3.2.6: Surface Temperatures in the Workshop 33
Fig 4.3.2.7: Temperature Graph of the Office compared to the Courtyard and London Wunderground Data 34
Fig 4.3.2.8: Temperature Graph of the Workshop compared to the Courtyard and London Wunderground Data 34
Fig 4.3.2.9: Comparison of Dry Bulb Temperatures for Various Spaces 35
Fig 4.3.2.10: Heat Gain and Heat Losses in the Office (24 hour Mean) 36
Fig 4.3.2.11: Heat Gain and Heat Losses in the Workshop (24 hour Mean) 36
Fig 4.3.2.12: Temperature Graph: Office | Base Case Period | Free Running 37
Fig 4.3.2.13: Comfort Hours: Office | Base Case Period | Free Running 37
Fig 4.3.2.14: Temperature Graph: Office | Base Case Period | Existing Heating Conditions 37
Fig 4.3.2.15: Comfort Hours: Office | Base Case Period | Existing Heating Conditions 37
Fig 4.3.2.16: Temperature Graph: Workshop | Base Case Period | Free Running 38
Fig 4.3.2.17: Comfort Hours: Workshop | Base Case Period | Free Running 38
Fig 4.3.2.18: Temperature Graph: Workshop | Base Case Period | Existing Heating Conditions 38
Fig 4.3.2.19: Comfort Hours: Workshop | Base Case Period | Existing Heating Conditions 38
Fig 4.3.3.1: Spot Measurements: Passive Zone 39
Fig 4.3.3.2: Spot Measurements: Artificial Lights ON 39
Fig 4.3.3.3: Spot Measurements: Artificial Lights OFF 39
Fig 4.3.3.4: Spot Measurements: Daylight Factor 39
Fig 4.3.3.5: Daylight Simulations: Point in Time 39
Fig 4.4.1.1: Solar Radiation: Analysis Period I (May to August) 40
Fig 4.4.1.2: Solar Access: Analysis Period (May to August) 40
Fig 4.4.1.3: Solar Radiation: Analysis Period II (November to February) 40
Fig 4.4.1.4: Solar Access: Analysis Period II (November to February) 40
Fig 4.4.1.5: CFD Simulations in Plan: Analysis Period I (May to August) 41
Fig 4.4.1.6: CFD Simulations in Section: Analysis Period I (May to August) 41
Fig 4.4.1.7: CFD Simulations in Plan: Analysis Period II (November to February) 41
Fig 4.4.1.8: CFD Simulations in Section: Analysis Period II (November to February) 41
Fig 4.4.2.1: Heat Gain and Heat Losses in the Office for the Two Periods (24 hour Mean) 42
Fig 4.4.2.2: Heat Gain and Heat Losses in the Workshop for the Two Periods (24 hour Mean) 42
Fig 4.4.2.3: Temperature Graph: Office | Summer Period | Free Running 43
Fig 4.4.2.4: Comfort Hours: Office | Summer Period | Free Running 43
Fig 4.4.2.5: Temperature Graph: Office | Winter Period | Free Running 44
Fig 4.4.2.6: Comfort Hours: Office | Winter Period | Free Running 44
Fig 4.4.2.7: Temperature Graph: Office | Winter Period | Existing Heating Conditions 44
Fig 4.4.2.8: Comfort Hours: Office | Winter Period | Existing Heating Conditions 44
Fig 4.4.2.9: Energy Balance: Office | Free Running | Monthly Cumulative 45
Fig 4.4.2.10: Energy Balance: Office | Existing Heating Conditions | Monthly Cumulative 45
Fig 4.4.2.11: Temperature Graph: Workshop | Summer Period | Free Running 46
Fig 4.4.2.12: Comfort Hours: Workshop | Summer Period | Free Running 46
Fig 4.4.2.13: Temperature Graph: Workshop | Winter Period | Free Running 47
Fig 4.4.2.14: Comfort Hours: Workshop | Winter Period | Free Running 47
Fig 4.4.2.15: Temperature Graph: Workshop | Winter Period | Existing Heating Conditions 47
Fig 4.4.2.16: Comfort Hours: Workshop | Winter Period | Existing Heating Conditions 47
Fig 4.4.2.17: Energy Balance: Workshop| Free Running | Monthly Cumulative 48
Fig 4.4.2.18: Energy Balance: Workshop | Existing Heating Conditions | Monthly Cumulative 48
Fig 4.4.2.19: Temperature Graph: Courtyard | Summer Period 49
Fig 4.4.2.20: Comfort Hours: Courtyard | Summer Period 49
Fig 4.4.2.21: Temperature Graph: Courtyard | Winter Period 49
Fig 4.4.2.22: Comfort Hours: Courtyard | Winter Period 49
Fig 4.4.3.1: Sun Patch Studies 50
Fig 4.4.3.2: Point in Time Simulations | Summer Solstice & Winter Solstice | Sunny Sky Conditions 51
Fig 4.4.3.3: Point in Time Simulations | Summer Solstice & Winter Solstice | Overcast Sky Conditions 52
Fig 4.4.3.4: Point in Time Simulations | Interior Perspectives | The Two Extreme Sky Conditions 53
Fig 4.4.3.5: Spatial Daylight Analysis 54
Fig 4.4.3.6: Useful Daylight Illuminance 54
Fig 4.4.3.7: Daylight Factor 54
Fig 4.5.1.1: Solar Radiation | Summer 55
Fig 4.5.1.2: Solar Radiation | Winter 55
Fig 4.5.1.3: Sun Patch | Summer Solstice | 12 noon 55
Fig 4.5.1.4: Sun Patch | Winter Solstice | 12 noon 55
Fig 4.5.2.1: Temperature Graph: Office | Winter Period | Optimized Heating Conditions 56
Fig 4.5.2.2: Comfort Hours: Office | Winter Period | Optimized Heating Conditions 56
Fig 4.5.2.3: Energy Balance: Office | Optimized Heating Conditions | Monthly Cumulative 56
Fig 4.5.3.1: Temperature Graph: Workshop | Winter Period | Optimized Heating Conditions 57
Fig 4.5.3.2: Comfort Hours: Workshop | Winter Period | Optimized Heating Conditions 57
Fig 4.5.3.3: Energy Balance: Workshop | Optimized Heating Conditions | Monthly Cumulative 57
Fig 4.5.4.1: Thermal Studies: Summary Table 58
Fig 4.5.5.1: Daylight Studies: Summary Table 59
Fig 5.1.1: Methodology for Technical Studies 62
Fig 5.2.1.1: Graphs showing Net Temperature Increase and Comfort Percentages for Summer and Winter for the Courtyard 66 Fig 5.2.2.1: Graphs showing Net Temperature Increase and Comfort Percentages for Summer and Winter for the Office 67
Fig 5.2.2.2: Graphs showing Net Temperature Increase and Comfort Percentages for Summer and Winter for the Workshop 67
Fig 5.2.3.1: Graphs showing Net Temperature Increase and Comfort Percentages for Summer and Winter for the Office 68
Fig 5.2.3.2: Graphs showing Net Temperature Increase and Comfort Percentages for Summer and Winter for the Workshop 68
Fig 5.2.4.1: Graphs showing Net Temperature Increase and Comfort Percentages for Summer and Winter for the Office 69
Fig 5.2.4.2: Graphs showing Net Temperature Increase and Comfort Percentages for Summer and Winter for the Workshop 69 Fig 5.3.2.1: Graph showing mean OT achieved by the various Combinations | Summer Period | Office 71 Fig 5.3.2.2: Graphs showing Net Temperature Increase and Comfort Percentages for the various Combinations | Summer | Office 71
Fig 5.3.3.1: Graph showing mean OT achieved by the various Combinations | Winter Free Running | Office 72
Fig 5.3.3.2: Graphs showing Net Temperature Increase and Comfort Percentages for the various Combinations | Winter Free Running | Office 72
Fig 5.3.3.3: Graph showing mean OT achieved by the various Combinations | Winter Heated | Office 72
Fig 5.3.3.4: Graphs showing Net Temperature Increase and Comfort Percentages for the various Combinations | Winter Heated | Office 72
Fig 5.3.4.1: Graph showing Heating Loads required for various Combinations | Monthly | Office 73
Fig 5.3.5.1: Graph showing mean OT achieved by the various Combinations | Summer Period | Workshop 74
Fig 5.3.5.2: Graphs showing Net Temperature Increase and Comfort Percentages for the various Combinations | Summer | Workshop 74
Fig 5.3.6.1: Graph showing mean OT achieved by the various Combinations | Winter Free Running | Workshop 75
Fig 5.3.6.2: Graphs showing Net Temperature Increase & Comfort Percentages for the various Combinations|Winter Free Running|Workshop 75
Fig 5.3.6.3: Graph showing mean OT achieved by the various Combinations | Winter Heated | Workshop 75
Fig 5.3.6.4: Graphs showing Net Temperature Increase and Comfort Percentages for the various Combinations | Winter Heated | Workshop 75
Fig 5.3.7.1: Graph showing Heating Loads required for various Combinations | Monthly | Workshop 76
Fig 5.3.8.1: Graph showing mean OT achieved by the various Combinations | Summer Period | Courtyard 77
Fig 5.3.8.2: Graphs showing Net Temperature Increase and Comfort Percentages for the various Combinations | Summer Period | Courtyard 77
Fig 5.3.8.3: Graph showing mean OT achieved by the various Combinations | Winter Period | Courtyard 77
Fig 5.3.8.4: Graphs showing Net Temperature Increase and Comfort Percentages for the various Combinations | Winter Period | Courtyard 77
Fig 5.4.1: Comparison between Existing and Improved Case for Annual Daylight Simulations 78
“Thesunneverknewhowgreatitwas untilithitthesideofabuilding” - Louis I. Kahn
The aim for this report was to study the facilities spaces inside the AA and analyze their performance in terms of both thermal and visual comfort, with a further to propose for potential alterations, consequently improving occupant environmental comfort.
This is achieved through the combination of indoor and outdoor studies, along with environmental measurements and computational simulations. The study undertaken in this case is that of ‘Facilities’ areas, located in the basement of 33 Bedford Square which includes three different spaces: the facilities office, the workshop and a courtyard which is placed between the two other spaces. These are located inside a typical Georgian-terrace house, located in London.
One of the most intriguing parts of this study was that the spaces are located in a basement. Typically, the impression of a basement does not equate/ amount to comfort. However, these spaces include a courtyard – a semi outdoor space; which could potentially influence the indoor spaces. Thus, our research was mainly focused in trying to understand the performance of the indoor spaces with respect to this courtyard.
Multiple visits to these spaces were conducted to carry out the analysis. Outdoor and indoor spot measurements were taken over several days and in a variety of environmental conditions. Simultaneously, user comfort was also judged based on multiple interactions with the occupants. This led to the creation of a base case scenario that helped in establishing precedents. It included the characteristics of the spaces which served as the starting point for computational simulations that showed an overview of the environmental capabilities of the spaces and their relationship to its geographical surroundings. Finally, simulation tools were used to forecast the building’s performance through the course of two chosen periods of study throughout the year. At the end, these tools were used to develop and test alternative design options that could increase occupant comfort and reduce energy use.
Fig 1.1.1: Illustrated Section through a Bedford Square Georgian HomeThis report is divided into mainly five chapters. The first one includes the brief of the project, its aim, expected outcomes and the outline of the way in which the study has been conducted.
After this, an overview of the site including its location and history has been specified. This includes layout of the spaces, user comfort, materiality and construction method.
In order to establish key periods of study, in the next part, the climate of the geographical location of the site was analyzed.
The major analysis part of the report is in the outdoor and indoor studies chapter, which is subdivided into – the base case and the analysis periods. This includes analysis of the environmental comfort of the spaces through fieldwork and computational simulations.
Finally, in the fifth chapter of the study, different iterations and a technical analysis was carried out to achieve a proposed design that aims at making these spaces more comfortable for the occupants.
The spaces that are analyzed as part of this report are located inside Architectural Association School of Architecture. The AA consists of a set of Georgian and Victorian houses, located in Central London in front of Bedford square.
The spaces assigned are the facilities areas in the school which are in the Basement of 33 Bedford Square.
Latitude/Longitude : 51.52°N; 0.13°W
The three spaces studied are: Facilities Office, Workshop and Courtyard.
The facilities basement at 33 Bedford Square contains spaces for facility and duty managers that help operate the school. Out of these spaces, the office; workshop and courtyard are part of this study. The layout of the plan consists of three interconnected rectilinear volumes.
The office is towards the North East of the school, with its windows opening into an outdoor space, i.e., the courtyard. By definition, the courtyard is an open to sky space and is located between the office and workshop. This space is the largest of the three and contains a small enclosed storage shed which is not part of the study. This further connects to the workshop - which is located in the South West part of the building. The North East wall of the workshop has two windows which overlook the courtyard. The South West wall faces Morwell Street. On this wall, there are two small openings - one of which is used as a door to transfer materials into the workshop and the other is a small frosted glass window.
These spaces are partially buried into the ground - about 2.1m below the ground level. The South West wall of the workshop which faces Morwell street is semiburied, being around 1m above and 2.1m below ground level.
The courtyard has fairly narrow proportions of width : height = 1:1.6 and length : height = 1:1.
Photo Studio Office Photo Studio Foundation Bedford SquareAs shown in Fig 2.2.1 the office is used by three occupants who manage the facilities of the school. The workshop is only used by the carpenter. The layout of the two indoor spaces is crowded with storage material. After a detailed occupant survey including interviews and informal interactions - it was concluded that users are mostly comfortable in the space because of the heating and multiple adaptive opportunities that they utilise as showcased in Fig 2.2.2.
Office Workshop
No. occupants: 3 Hours/day: 9-10
No. of Luminaires: 6
Total no. of lights: 12
Total Watts: 432W
Hours / day: 10h
3 PC monitors
Total Watts: 70 24-hr Mean: 61W
1 printer
Total Watts: 600 24-hr Mean: 13W
No. of Luminaires: 4
Total no. of lights: 8
Total Watts: 288W
Hours / day: 7h
2 Saw Machines
Total Watts: 2275 24-hr Mean: 161W
Head of Estates
Space Used: > 8 years
NoiseAir QualityVentilationGlareDaylightThermal: WintersThermal: Summers
Lewis
Facilities Project Manager
Space Used: > 6 months
Maintenance Supervisor
Space Used: > 12 months
Prendergast
Maint. Operative (Carpenter)
Space Used: > 18 years
Fig 2.2.2: Occupants Survey
Adaptive Opportunity
Fig 2.2.3: Interior views of the ‘Office’ Fig 2.2.4: The central ‘Courtyard’ Fig 2.2.5: Interior views of the ‘Workshop’The construction materials used in the spaces are typical to those used for Georgian Terrace houses in London. In order to understand the environmental comfort of the space, a material study was conducted as the initial step.
As shown in Fig 2.3.2, the external walls of the office and workshop are made up of stock brick, gypsum board and plaster while also consisting of an air-gap. These walls are approximately 700 mm thick in total. The internal walls are thinner (not consisting of an air-gap) and are made of brick and plaster.
The floor in the two rooms is made of concrete, screed and wood. In the office, there is an additional layer of carpet used for insulation.
CEILING
U- value: 1.3 Wooden Planks 20mm Wooden Joists 250mm Ceiling Board 19mm
EXTERNAL WALL
U- value: 0.75 Brick 500mm Air gap 100mm Gypsum board 19mm Plaster 20mm
FLOOR: WORKSHOP
U- value: 1.87 Wood 25mm Screed 15mm Concrete 200mm
INTERNAL WALL
U- value: 1.46 Brick 250mm Plaster 20mm
FLOOR: OFFICE
U- value: 1.58 Carpet 5mm Wood 25mm Screed 15mm Concrete 200mm
SASH WINDOW
U- value: 5.6 Single glazing Clear glass 50% Operable
The period between 1714-1830 in England was characterized by Georgian architecture, which was named after the four George monarchs. Throughout this period, buildings were influenced by different architectural styles as the main references changed with the years and dogmas were rethought. Starting with the Classicism and the Palladian proportion-based, through the combination of Gothic, Greek, Roman, Chinese and Gothic.
The terrace house is one of the remarkable building typologies of the Georgian era, and Bedford Square houses, built in 1775-83 are one of the most famous examples. They were built as city homes for wealthy clients. The intention was to optimize the space and these residential developments would be intended to have as many homes as possible into the length of the street, resulting in “one uniform row of houses”.
Georgian terrace houses were typically three stories high with a narrow plan and could have a back garden. They each consisted of two rooms on each floor, one in the front and another in the back, separated by a staircase and a passage.
• Façade: The façade followed the Palladianism proportion and axial symmetry, as it is seen in the distribution of the windows, but had also a great number of details, such as the ornamental work with stones above the door entrance.
• Windows: Sash windows started to be used from the early eighteenth century, with two sliding parts, usually with six glazed panes each and were installed with a set back from the façade.
• Doors: Doors were typically located at the side of the façade and had a ‘fanlight’, a semi-circular window on the upper part to allow light to come in.
Brick was the predominant construction material after the great fire of 1666, as it was fire resistant, as well as cheaper and less complicated to fabricate. Stock bricks were the most resistant ones and were typically used on the exterior structure in Bedford Square buildings. Internally, partitions were made with a less resistant material. Floors structure from early nineteenth century was built with timber joists.
A typical basement of larger London Georgian terrace houses was meant for service activities, so they were separated from the main floors. The kitchen was placed in the back, and servants’ room in the front, along with pantry and room for household works. In addition, there was a storage space, usually for coal, that would extend under the street level.
The “area” was a transitional space between the building and the footpath, and stairs led directly to the basement from the street. It not only allowed there to be windows in the lower part, but also represented a physical separation, which contributed to privacy. As opposed to the front of these terrace houses, alterations to the rear wouldn’t harm the coherence in architecture features.
Fanlight
The St. James’ Station’s weather file was consulted for wind direction which is depicted in the wind rose (fig 3.2.1). The prevailing wind in London is predominantly from South West direction. However, after the study was conducted, it became clear that the facilities spaces experience very low wind speeds and even though the dominant direction differs for summer months - the difference is very little.
Sky conditions are typically clear, partially cloudy and overcast for almost equal periods throughout the year (fig 3.2.2).
Analysis Period I: Mean GI 31,000 lux & Max. GI 59,000 lux
Analysis Period II: Mean GI 11,000 lux & Max. GI 26,000 lux
Fig 3.2.2: Pie-chart illustrating frequency of sunny, intermediate & cloudy skies annually
Mean wind speed: 1.57m/s
Mean wind speed: 1.48 m/s
Fig 3.2.1: Wind Rose for Analysis Period-I May to Aug (left) and Analysis Period- II Nov-Feb (right) for London
Jul Mar Sept May Nov Feb Aug Apr Oct Jun Dec
Jan 0 10000 30000 5000020000 40000 60000
(Source: Ladybug Tools) (Source: Satel-Light) (Source: Satel-Light)
Fig 3.2.3: Monthly maximum global illuminance & mean global illuminance for sunlight hours for London
By using the Grasshopper (Ladybug) plug-in software in Rhino 3D, a shadow analysis was performed on the spaces of facilities at AA with respect to its surroundings. The shadow patterns in different times during the summer and winter solstice and the equinox are demonstrated. The solar exposure that is depicted in the courtyard shows that for most part of the year, there is almost a complete obstruction of the sun by the surrounding buildings. During summer, this could be a slight advantage, as it reduces the solar radiation and contributes to the thermal comfort of the indoor spaces, but this is true only for a very short period when outdoor dry-bulb temperature reaches high levels in the months of June and July. However, during the rest of the year, when solar heat-gains are highly required, this is a major disadvantage as the heights of these surrounding buildings act as a deterrent and over-obstruct the space.
It is only during a short period of time during the summer, that the solar rays and the natural light are allowed to penetrate into the spaces. But this as we can observe, is also fairly minimal.
Summer Solstice (12.00): The courtyard and windows of the office receive direct sunlight.
Summer Solstice (15.00): One of the windows of the office receives minimal direct sunlight.
0900 HRS
1500
Fig 4.1.1: Shading Mask
Shading mask :
Another simulation was carried out to check the sky exposure of the courtyard.
Fig 4.1.1 shows how minimal it is which is because of the presence of the relatively tall buildings of the school around the courtyard.
To further understand the times and areas where there is direct sunlight inside our spaces, the canyon section was studied plotting the various sun angles with respect to the context of the courtyard and its adjacent buildings. These sun angles were plotted for the winter and summer solstice and equinox for three different points in time through the day.
This confirmed the following, which were also seen through the shadow analysis:
Summer Solstice (12.00): Some direct sunlight can be observed in the courtyard and in the office.
Summer Solstice (15.00): Minimal direct sunlight entering into the office but it might receive glare due to the lower sun angle.
Other than these times, there is no direct sunlight that reaches the facilities spaces, as is shown in Fig. 4.2.2.
First Floor
Street Level
Basement Level
Second Floor Third Floor
First Floor Second Floor
Third Floor
Street Level
Basement Level
Fig 4.2.1: The Canyon Section of the Courtyard showing the various sun angles Fig 4.2.2: The times of the year when the sun directly reaches the CourtyardSolar studies through simulations were further carried out for the base case period to determine the solar radiation inside the courtyard.
The solar radiation during the specified period was minimal because exposure of the sun to this space is not significant.
Solar access simulations reiterated the findings from the shadow analysis and canyon section - due to lack of direct sunlight, there is no solar access in the facilities courtyard during the base case period. This is due to the low sun angle and the tall surrounding buildings
kWh/m2 Hours 630.00 560.00 490.00 420.00 350.00 280.00 210.00 140.00 70.00 00.00
Hours 255.00 229.50 204.00 178.50 153.00 127.50 102.00 76.50 51.00 25.50 00.00 700.00
After establishing wind direction through wind rose, simulations were conducted through Autodesk CFD (Computational Fluid Dynamics) software to observe the wind pattern in the courtyard area and its surroundings. The prevailing wind direction is southeast and the mean velocity from this direction is 1.51m/s. The plan was taken from a height of 4.5m from the street level. Although it indicates movement above buildings, the colour blue shows a low wind velocity in street level and, therefore, in the courtyard. As observed in the plan, tall buildings to the south of the facilities courtyard act as barriers, which prevent high speed wind to reach lower levels, as seen in the section. Closer to street level it is possible to assume that pressure difference can generate air movement. Although this might happen, the courtyard is still not being affected by the wind. It is possible to assume that geometry and location of courtyard (an enclosed space in the middle of the block and surrounded by tall buildings) prevents it from receiving higher speed winds.
Spot measurements were carried to measure the temperature and relative humidity of the spaces. It was observed that:
1. Temperature difference between indoor and outdoor spaces were higher than 10°C, reaching 16.1°C (Office x Morwell St).
2. There is variance in temperature between both indoor spaces, only by1°C.
3. Relative humidity outdoor-indoor variation was maximum 23.2%
4. Temperatures in indoor spaces are fairly stable due to the heating being on and do not have much variation between themselves even though they have completely different usage and number of occupants.
These temperatures were also compared to that at Bedford Square and Morwell street. (Fig 4.3.2.3) It was observed that temperature and relative humidity do not suffer significant change within the spaces because of the presence of radiators which stabilise the indoor spaces.
Date : 23rd November 2021
Time : 1000 hr- 1030 hr
Sky Condition : Overcast
Temp (London) : 3 °C -8 °C (min.- max.)
Doors : All Closed
Windows : All Closed
Occupants : N/A
Doors : Open
Windows : 2 Closed, 1 Partially Open
Occupants : All Present (3)
Appliances : Computers ON/ Printers OFF
Heating : ON
Note: The indoor spaces had the heating ON at the time of measurements.
Doors : Closed
Windows : All Closed
Occupants : Absent Appliances : OFF
Heating : ON
Humidity[%]
SED
In order to accurately understand the differences in surface temperatures across the spaces - the measurements were taken at three different heights. Further the two indoor spaces were compared with the outdoor space. The high ∆T between spaces is because of the radiators being on. Less difference in temperature with height in the courtyard was observed as compared to the other spaces. Thermal camera was also used to take a closer look.
The surface temperature rises from the ground towards the
The surfaces near the window are colder than surfaces further away. This variation in surface temperatures can be seen in the images from the ∆T between air temperature and surface temperature for the courtyard is significantly larger than ∆T of the indoor spaces.
Through the thermal camera, it can be observed that there is significant heat loss through the windows.
The surfaces near the windows facing SW are colder than the surfaces near the windows facing NE.
The variation of temperature across surfaces observed a similar trend to that in the office.
The next step in the study of the spaces using data loggers was comparing the facilities spaces with other surrounding areas including Bedford Square, the SED studio and the new yard.
SED studio: This was done to understand how the facilities office behaves in comparison to a space which is similarly shaped, has the same orientation and same construction. This was thought to be appropriate since the SED studio is located exactly three floors above the facilities office. ∆T of 1°C was observed between the two spaces. It can be concluded that this variation is due to the placement of office - which is sunken into the earth.
Bedford Square: This was done to understand the way our semi-outdoor space, the courtyard, behaves as compared to a completely outdoor space. Also, it was done to check if the surrounding bounding box has any influence on the micro climate of the courtyard. ∆T of 1°C was observed.
New Yard: This is a courtyard space located in the Basement of 38 Bedford Square (near the facilities courtyard). Even though, it varies in size - it is at the same height and has a similar bouding box. The trend observed was very similar.
300 600 900 1200 1500
0 300 600 900 1200 1500 0.0 5.0 10.0 15.0 20.0 25.0
TFacilities
3:00:00 PM 4:30:00 PM 6:00:00 PM 7:30:00 PM 9:00:00 PM 10:30:00 PM 12:00:00 AM 1:30:00 AM 3:00:00 AM 4:30:00 AM 6:00:00 AM 7:30:00 AM 9:00:00 AM 10:30:00 AM 12:00:00 PM 1:30:00 PM 3:00:00 PM 4:30:00 PM 6:00:00 PM 7:30:00 PM 9:00:00 PM 10:30:00 PM 12:00:00 AM 1:30:00 AM 3:00:00 AM 4:30:00 AM 6:00:00 AM 7:30:00 AM 9:00:00 AM 10:30:00 AM 12:00:00 PM 1:30:00 PM 3:00:00 PM 4:30:00 PM 6:00:00 PM 7:30:00 PM 9:00:00 PM 10:30:00 PM 12:00:00 AM 1:30:00 AM 3:00:00 AM 4:30:00 AM 6:00:00 AM 7:30:00 AM 9:00:00 AM 10:30:00 AM 12:00:00 PM 1:30:00 PM 3:00:00 PM 4:30:00 PM 6:00:00 PM 7:30:00 PM 9:00:00 PM 10:30:00 PM 12:00:00 AM 1:30:00 AM 3:00:00 AM 4:30:00 AM 6:00:00 AM 7:30:00 AM 9:00:00 AM 10:30:00 AM 12:00:00 PM 1:30:00 PM 3:00:00 PM 4:30:00 PM
3:00:00 PM 4:30:00 PM 6:00:00 PM 7:30:00 PM 9:00:00 PM 10:30:00 PM 12:00:00 AM 1:30:00 AM 3:00:00 AM 4:30:00 AM 6:00:00 AM 7:30:00 AM 9:00:00 AM 10:30:00 AM 12:00:00 PM 1:30:00 PM 3:00:00 PM 4:30:00 PM 6:00:00 PM 7:30:00 PM 9:00:00 PM 10:30:00 PM 12:00:00 AM 1:30:00 AM 3:00:00 AM 4:30:00 AM 6:00:00 AM 7:30:00 AM 9:00:00 AM 10:30:00 AM 12:00:00 PM 1:30:00 PM 3:00:00 PM 4:30:00 PM 6:00:00 PM 7:30:00 PM 9:00:00 PM 10:30:00 PM 12:00:00 AM 1:30:00 AM 3:00:00 AM 4:30:00 AM 6:00:00 AM 7:30:00 AM 9:00:00 AM 10:30:00 AM 12:00:00 PM 1:30:00 PM 3:00:00 PM 4:30:00 PM 6:00:00 PM 7:30:00 PM 9:00:00 PM 10:30:00 PM 12:00:00 AM 1:30:00 AM 3:00:00 AM 4:30:00 AM 6:00:00 AM 7:30:00 AM 9:00:00 AM 10:30:00 AM 12:00:00 PM 1:30:00 PM 3:00:00 PM 4:30:00 PM
Facilities
Fig 4.3.2.9: Comparison of Dry Bulb Temperatures for Various Spaces
Global Horizontal Radiation
Horizontal
As input data for this analysis, the mean outdoor temperature and was taken from St. James’ weather station, and direct solar radiation from Grasshopper simulations, while data for the internal loads was gathered through fieldwork. The results show a rise of 4.5K in the office and 3.8K in the workshop mean indoor temperature.
In the office, lighting and occupancy are the main contributing factor to heat gains, whereas infiltration and glazing conduction contribute to heat losses.
In the workshop, the heat gains come mostly from heavy appliances, and the major cause of heat loss is infiltration. Significantly, solar gains are low in both cases which reflects the low radiation seen in the solar studies earlier conducted. Window glazing is more significant in office’s heat loss since this space has more glazed area.
BASE CASE PERIOD
Mean Outdoor Temperature: 8.9°C
Mean Indoor Temperature Rise: 4.5 K
Predicted MinT: 13.4°C
LOADS (24 hour mean Watts)
LOADS (24 hour mean Watts)
-400.0 -300.0 -200.0 -100.0 0.0 100.0 200.0 300.0 400.0
WINTER
SOLAR GAIN 0.0
LIGHTS 162.0
73.8
BASE CASE PERIOD
Mean Outdoor Temperature: 8.9°C
Mean Indoor Temperature Rise: 3.8 K
Predicted MinT: 12.7°C
LOADS (24 hour mean Watts)
BASE CASE -400.0 -300.0 -200.0 -100.0 0.0 100.0 200.0 300.0 400.0 -89
BASE CASE -400.0 -300.0 -200.0 -100.0 0.0 100.0 200.0 300.0 400.0 -89
APPLIANCES 73.8
OCCUPANTS 123 INFILTRATION -134.1
OCCUPANTS 123 INFILTRATION -134.1
BASEMENT FLOOR -46.6
BASEMENT FLOOR -46.6
EXTERNAL WALLS -59.8
EXTERNAL WALLS -59.8
WINDOWS/GLAZING CONDUCTION -117.4
WINDOWS/GLAZING CONDUCTION -117.4
LOADS (24 hour mean Watts)
SOLAR GAIN 0
BASE
BASE CASE
SOLAR GAIN 0
LIGHTS 96
LIGHTS 96
APPLIANCES 161
APPLIANCES 161
OCCUPANTS 85
OCCUPANTS 85
INFILTRATION -157.6
INFILTRATION -157.6
BASEMENT FLOOR -54.8
BASEMENT FLOOR -54.8
EXTERNAL WALLS -53.8
EXTERNAL WALLS -53.8
WINDOWS/GLAZING CONDUCTION -76.2
WINDOWS/GLAZING CONDUCTION -76.2
A similar simulation was done to understand the operative temperature and thermal comfort of the user in the workshop.
Had the space been in free running mode, the user would be comfortable 37.5% of the time. This comfort percentage is much more in the workshop as compared to the office because the PMV/PPD comfort model has been used here with a met value of 1.5. This is because it wouldn’t be accurate to ignore the heat dissipation emitted by the user considering his activity as well as his metabolic rate, that interferes with his comfort sensation. The user operates heavy machinery and overall, his work requires him to be physically active during most hours when he occupies the space. In this case, the mean operative temperature is almost 8°C away from the comfort band mean.
However, when the heating schedule is adjusted to the way the space operates currently - the occupant is comfortable 100% of the time since the radiators are turned on for majority of the day. The operative temperature in this case reaches the comfort band and has a mean of 20.8°C.
Min. Indoor Temperature
Min. Indoor
Glazing Area Operable
Glazing Area Operable
Heating
Heating Set
Free Running: 24°C
Free Running:
Existing Heating: N/A
Existing Heating: N/A
Free Running: 50%
Free Running: 50%
Existing Heating: N/A
Existing Heating: N/A
Free Running: N/A
Free Running: N/A
Existing Heating: 24°C
Existing Heating: 24°C
Min. Indoor Temperature for
Free Running: 24°C
Free Running: 24
Existing Heating: N/A
Existing Heating: N/A
Free Running: 50%
Free Running:
Existing Heating: N/A
Existing Heating: N/A
Daylight spot measurements were taken to understand the illuminance inside the spaces. Most areas in the spaces were below the minimal standard illuminance for a workplace (300-500lux for office and 300-750 for the workshop according to CIBSE). This happens mostly because of lack of direct sunlight in the spaces. When the same measurements were taken with artificial lights turned ON- the illuminance levels reached the standard requirement.
Daylight factor was manually calculated to be able to fully understand the deficit of light in these spaces. It indicates a reduction in percentage as we go deeper into these spaces, away from the windows. To calculate DFthe office, workshop and the courtyard were compared to the illuminance at that point in time at Bedford Square.
To represent an accurate picture, even though the courtyard is an outdoor space, its DF was also calculated with respect to to Bedford square’s. As shown in Fig 4.3.3.1 - these measurements were also compared to the Global Illuminance to be able to establish a passive zone for the indoor spaces. The rule of thumb for passive zone : Passive Depth = 6m OR X2 room height. In this case, the spaces do not meet this rule of thumb.
Using Ladybug simulation tools, further studies for solar radiation were conducted to understand the solar radiation during the two established analysis periods in the year. As expected, minimal changes in radiation were seen in a year, specifically the winter months showed almost no radiation.
It may be noted that one of the reasons for this is the height of the bounding box. It can be seen that the facade containing the facilities office receives solar radition on its upper floors, however because of obstructions by other buildings and the low sun angle, the facilities office receives minimal radiation.
In conclusion, it can be clearly stated that these spaces do no get adequate radiation that could have potentially helped in heat gain and raising the temperature inside the indoor spaces.
Solar access in the courtyard was also simulated for the analysis periods and a similar result was observed. The only time the courtyard had some sun exposure was during the summer months for a brief amount of time.
In conclusion, it can be clearly stated that these spaces do no have adequate sunlight to ensure sufficient daylight inside the space.
Fig 4.4.1.1: Solar Radiation: Analysis Period I (May to August)
Analysis Period I : Summer Months (May to August)
Fig 4.4.1.2: Solar Access: Analysis Period (May to August)
• In Fig 4.4.1.1 , we can see that the space receives about 150 kWh/m2 radiation received on a average in the space
• As observed from Fig 4.4.1.2 - we can see that only a small part receives about 125-160 hours of direct sunlight in this period. Solar access is minimal
Fig 4.4.1.3: Solar Radiation: Analysis Period II (November to February)
Analysis Period II : Winter Months (November to February)
• There is almost no radiation received during this time of the year.
• No direct sunlight in the courtyard during this period.
Fig 4.4.1.4: Solar Access: Analysis Period II (November to Februar y)
Simulations were performed for both analysis periods and considered as inputs. The prevailing wind direction and mean velocity was taken from the St. James weather file. In summer, the wind comes mostly from southwest at a mean velocity of 1.57m/s, and in winter the wind comes at a mean velocity of 1.48m/s from the northeast.
A different pattern can be observed when comparing the wind performance in the surrounding environment of AA buildings. In conclusion, the courtyard is not affected in a significant way by the prevailing winds. Near our site, a local phenomena takes place between buildings wherein due to surface temperature and pressure difference - there is a possibility of more of an air movement closer to the street level, but still this wind does not reach the courtyard.
Analysis Period : Summer Months (May to August)
• Prevailing direction : South West
• Mean velocity : 1.57m/s
• In summer, the barrier effect of constructions to the left of the school contributes to the overall decrease in the wind speed, spe cifically at the lower height at which the courtyard is there.
Analysis Period II : Winter Months (November to February)
• Prevailing direction : North East
• Mean velocity : 1.48m/s
• In winter, winds coming from Northeast travel across the neighbouring buildings to AA and bedford square before reaching the courtyard - which reduces the barrier effect. However, the air movement in the courtyard still remains insignificant.
Fig 4.4.1.6: CFD Simulations in Section: Analysis Period (May to AugusFig 4.4.1.5: CFD Simulations in Plan: Analysis Period I (May to August) Fig 4.4.1.8: CFD Simulations in Section: Analysis Period II (Novembe 4.4.1.7: CFD Simulations in Plan: Analysis Period II (November to February)MInT
From the analysis conducted for the two periods, it is observed that the trend is similar to base case with regards to the main items that influence heat gain and loss. The Mean Indoor Temperature (MinT) rise differs from office to workshop in both periods. The augmented temperature during summer is influenced by solar gains.
Due to the orientation of the office windows facing the courtyard (southwest), it receives direct radiation. In the workshop, southwest windows are smaller in area and positioned in the street level of Morwell Street, which makes it more difficult to receive radiation. Hence, the temperature inside the workshop is not influenced by direct solar radiation.
SUMMER PERIOD
Mean Outdoor Temperature: 17.1°C
Mean Indoor Temperature Rise: 3.4 K
Predicted MinT: 20.5°C
Mean Outdoor Temperature: 7.3°C
Mean Indoor Temperature Rise: 4.5 K
Predicted MinT: 11.8°C
LOADS (24 hour mean Watts)
LOADS (kWh/m2) -600 -400 -200 0 200 400 600
Mean Outdoor Temperature: 17.1°C
Mean Indoor Temperature Rise: 2.0 K
Predicted MinT: 19.1°C
LOADS (kWh/m2)
Mean Outdoor Temperature: 7.3°C
Mean Indoor Temperature Rise: 3.8 K
Predicted MinT: 11.1°C
As for heat losses, infiltration is significant to both spaces. In summer it plays a larger role than in winter, as the windows are opened for longer periods when the space is occupied. -600.0 -400.0 -200.0 0.0 200.0 400.0 600.0
LOADS (kWh/m2) -600 -400 -200 0 200 400 600
SUMMER WINTER
SUMMER WINTER
SOLAR GAIN 117 0.0
SOLAR GAIN 117 0.0
LIGHTS 162 162.0
LIGHTS 162 162.0
APPLIANCES 74 73.8
APPLIANCES 74 73.8
OCCUPANTS 123 123
OCCUPANTS 123 123
INFILTRATION -304 -134.1
INFILTRATION -304 -134.1
BASEMENT FLOOR -36 -46.6
BASEMENT FLOOR -36 -46.6
EXTERNAL WALLS -45 -59.8
EXTERNAL WALLS -45 -59.8
WINDOWS/GLAZING CONDUCTION -89 -117.4
WINDOWS/GLAZING CONDUCTION -89 -117.4
Fig 4.4.2.1:
Gain and Heat Losses in the Office
the Two Periods (24 hour Mean)
LOADS (24 hour mean Watts) -600 -400 -200 0 200 400 600
LOADS (kWh/m2)
LOADS (kWh/m2) -600 -400 -200 0 200 400 600
SUMMER -89
SUMMER WINTER
SUMMER WINTER
SOLAR GAIN 13 0
SOLAR GAIN 117 0.0
SOLAR GAIN 117 0.0
LIGHTS 96 96
LIGHTS 162 162.0
LIGHTS 162 162.0
APPLIANCES 74 73.8
APPLIANCES 74 73.8
Natural Ventilation
OCCUPANTS 123 123
APPLIANCES 161 161 OCCUPANTS 75 85
OCCUPANTS 123 123
Min. Indoor Temperature for Natural Ventilation
INFILTRATION -248.1 -157.6
INFILTRATION -304 -134.1
INFILTRATION -304 -134.1
BASEMENT FLOOR -28.9 -54.8
BASEMENT FLOOR -36 -46.6
BASEMENT FLOOR -36 -46.6
Glazing Area Operable
EXTERNAL WALLS -28.2 -53.8
EXTERNAL WALLS -45 -59.8
EXTERNAL WALLS -45 -59.8
WINDOWS/GLAZING CONDUCTION -40.0 -76.2
WINDOWS/GLAZING CONDUCTION -89 -117.4
WINDOWS/GLAZING CONDUCTION -89 -117.4
4.4.2.2:
Mechanical Ventilation Heating Set Temperature
Gain and Heat Losses
Workshop
Two Periods
Mean)
Simulations were carried out for summer considering the building in a free running mode. It was observed that the office occupants are comfortable for 26% of occupied hours during this period, which is primarily post 1200hrs. The month of maximum comfort is July, which can be observed is due to the increase in outdoor dry bulb temperature and consequently in the operative temperature.
As shown from the simulation (fig 4.4.2.4), for 74% of the occupied hours, the occupants are relatively cold. However, this was found to not be an entirely accurate representation since the operative temperature in the office was fairly close to the comfort band, as is seen in Figure 4.4.2.3.
1000 1200
From the energy balance study there were several relevant observations. Comparing the two scenarios – without and with heating – allowed the team to understand how some elements were thermally affecting the space.
In a free-running scenario (fig 4.4.2.9), it was noticed that opaque conduction contributes as a heat gain during winter and facilitates heat dissipation in summer. This could be explained by the fact that the basement floor is exposed to the soil. Since ground temperature is usually more stable than air, it can be cooler in summers and warmer winters than outdoor temperature. In a free running situation, this is positive as the heat exchange with the ground can prevent the indoor temperature to achieve more extreme values, which could happen if it was only under the influence of outdoor air.
Figure 4.4.2.10 shows, however, that the previous behaviour is reversed, as it now adds to heat losses in the winter. Since the space is heated here, the indoor temperature is higher than soil and thus loses heat to it.
Regarding glazing conduction, it is clear how the single glazed and lack of solar radiation act facilitating heat losses in winter and meagre heat gains in summer.
Infiltration, as stated in the MinT analysis, is also a way through which the office loses heat to the outdoor environment in both periods, due to the outdoor air being cooler than the indoor. In winter, this phenomenon is further pronounced.
Solar gains are directly related to direct radiation, which only reaches the office windows during a few months in summer, therefore solar gains only primarily appear during the summer.
In a free-running scenario, the existing loads that contribute to heat gains – solar, equipments and occupants – are not sufficient to achieve comfort, especially in the winter months. Therefore, there is a large dependance on mechanical heating systems resulting in a cumulative heating load of 5,209 kWh annually.
Analysis Period II: November to February (Winter)
Analysis Period I: May to August (Summer)
Analysis Period II: November to February (Winter)
Storage Cooling
Glazing Conduction
Opaque Conduction
Natural Ventilation
Mechanical Ventilation
Infiltration
Occupants
Lighting
Fan
Solar Heating
Jan Feb Mar Apr May
Fig
Nov Dec
Analysis Period II: November to February (Winter)
Analysis Period I: May to August (Summer)
Analysis Period II: November to February (Winter)
Storage Cooling
Glazing Conduction
Opaque Conduction
Natural Ventilation
Mechanical Ventilation
Infiltration
Occupants
Lighting
Jan Feb Mar
Total Annual Heating Load:
The mean operative temperature was found to be 18.2°C for the workshop in the summer period, which is lower to that of the office (19.6°C). This may be attributed to less solar exposure and consequently, smaller swing in the operative temperature. Since the wall facing Morwell Street is partially buried, the thermal mass effect may also be causing slightly lower temperatures.
Although the operative temperature does not frequently reach the comfort band, it is seen in the hourly occupied comfort graph (Figure 4.4.2.12) that the user in this space feels comfortable in 69% of hours. As the PMV/PDD comfort model has been used for the workshop, which considers the users metabolic rate and clo value, the user’s perception of comfort can vary as a result of these two factors.
17.1°C (Mean DBT) 18.2°C (Mean OT) 24.4°C (Mean CB)
0 200 400 600 800 1000 1200 1400 1600 -5 0 5 10 15 20 25 30 35 Temp. ( C) Solar Radiation ( Wh /m 2)
JUNE JULY AUGUST 17.1°C (Mean DBT) 18.2°C (Mean OT) 24.4°C (Mean CB)
1200hrs
1200hrs
MAY Indoor OT Outdoor DBT Global Horizontal Radiation Adaptive Comfort Band (EN15251)
JUNE JULY AUGUST
0 200 400 600 800 1000 1200 1400 1600 -5 0 5 10 15 20 25 30 35 Temp. ( C) Solar Radiation ( Wh /m 2)
MAY
Indoor OT Outdoor DBT Global Horizontal Radiation Adaptive Comfort Band (EN15251)
0600hrs
0600hrs
MAY
HOURLY
JULY
Comfort Model:In the workshop, opaque conduction represents the main heat gain factor during winter months, and a heat loss in the summer period. Similar to as observed for the office, this is due to the construction mass exposed to the soil. In this case though, in addition to the floor of the workshop, the semiburied wall facing Morwell Street is also exposed to the soil, causing an even more pronounced effect than in the office.
Glazing conduction also appears as heat losses, mostly during winter.
The same trend is seen as in the office, with infiltration being a relevant aspect of total heat losses.
Solar gains in the workshop are negligible since the southwest windows are at the street level of Morwell Street, with one of them being blocked with opaque material and the other with frosted glass.
As analyzed for the office space, the existing heating conditions energy balance show opaque conduction performance reversing and infiltration still being a cooling factor. Due to a larger volume and lower operative temperature in the workshop as compared to the office, the cumulative annual heating load is also higher at 7,220 kWh.
Loads (kWh/m 2
Analysis Period II: November to February (Winter)
Analysis Period I: May to August (Summer)
Analysis Period II: November to February (Winter)
Storage Cooling
Glazing Conduction
Opaque Conduction
Natural Ventilation
Mechanical Ventilation
Infiltration
Occupants
Lighting Fan Electric Equipment
Solar Heating
Jan Feb Mar Apr May Jun Jul Aug Sep Oct Nov Dec
Loads (kWh/m 2
Analysis Period II: November to February (Winter)
Analysis Period I: May to August (Summer)
Analysis Period II: November to February (Winter)
Storage Cooling Glazing Conduction
Opaque Conduction
Natural Ventilation
Mechanical Ventilation
Infiltration
Occupants
Lighting Fan Electric Equipment
Solar Heating
Jan Feb Mar Apr May Jun Jul Aug Sep Oct Nov Dec
Total Annual Heating Load: 7,220 kWh
In the summer period in the courtyard, the comfort graphic (Figure 4.4.2.20) shows that most of the day the space is comfortable. The mornings are the coldest since it’s the period when it doesn’t receive solar radiation.
The occupied hours considered for the courtyard consolidated the occupancy hours of both indoor spaces, from 6am to 7pm. However, had it been considered from 9am onwards, when probably people would use more the space (since the carpenter is the only person using any of the facilities’ spaces early morning), then a higher percentage of comfortable hours may have been observed. Therefore, it may be concluded that the courtyard could be a pleasant space for people to experience during this period.
For the winter period, the temperature graph plotter in figure 4.4.2.21 shows that operative temperature follows the dry bulb one, but it doesn’t go as low, showing the courtyard as a more stable environment.
1900hrs
1800hrs
1900hrs
HOURLY
HOURLY
JUNE
MAY
NOVEMBER
NOVEMBER
JUNE
DECEMBER
DECEMBER
0 200 400 600 800 1000 1200 1400 1600
200 400 600 800 1000 1200 1400 1600
200 400 600 800 1000 1200 1400 1600
Sun Patch diagrams for the analysis periods show the difference between seasons and times of the day. In winter, the sun is expected to produce deep or no sun patches as the sun angle is very low . As we can observe, there is no sun patch for the winter period or even during mid season, i.e., the equinox.
Summer Solstice (12.00): There’s only a small patch of sunlight entering the office. Summer Solstice (15.00): There’s no sun entering directly but might receive glare. All other times of the year, none of the spaces receive any direct sunlight. Variation in overall global illumination can be observed between the three periods
0900 HRS
1200 HRS
1500 HRS
Next step was to conduct simulations for three different points in time on 21st June and 21st December with sky condition- sunny. It was observed that the average illuminance in December, because of the low sun angle and no direct sunlight in the courtyard, does not reach the minimum standard value (300 lux).
The space does not have adequate daylight even during the summer when the sun angle is higher. It is only at 12.00 and 15.00 that the average value reaches the standard. For the rest of the year, artificial lighting is essential.
(Source of Results: Ladybug Tools via Honeybee)
21ST JUNE
21ST DECEMBER
The same simulations were considered for overcast sky conditions for three different points in time on 21st June and 21st December. It can clearly be seen that there is no significant daylight inside the spaces at any point during the day when the sky is overcast. Illuminance levels have to be increased by altering colour of the surroundings to increase reflectance and/or by using artificial lighting.
(Source of Results: Ladybug Tools via Honeybee)
21ST JUNE
21ST DECEMBER
Fig 4.4.3.4 demonstrates the Image-based Illuminance study for the workhsop and office space. The study was conducted under sunny and overcast sky conditions for two indicative days (Summer Solstice, Winter Solstice) at 9.00, 12.00 and 15.00. They clearly demonstrate the difference in sunny sky and overcast sky - while the area around the window seems to have some adequate daylight, the further away from the window we go, the darker it gets - with little to no daylight at the end of the rooms.
The dynamic climate-based calculations Spatial Daylight Autonomy (sDA) and Useful Daylight Illuminance (UDI) help anticipate daylight performance throughout the year. They help evaluate over and under-illumination. When the minimum value of 300 lux is met, DA denotes the percentage of occupied hours per year. The DA study in figure 4.4.3.5 shows that the facilties’ spaces rarely reaches more than 300 lux.
The UDI reflects the percentage of occupied hours per year when illuminance is within the useful lux range of 100-300 lux. As a result, UDI can offer daylight levels that are linked to glare, occupant discomfort, and undesired solar gains. The percentage of time that each point of the floor area matches the UDI requirements is represented graphically by the percent values (100-300 lux). From the UDI, we see levels reaching between 100 and 300lux, but as is comfirmed by the sDA, these values are on the lower end of that spectrum. Since the standard illuminance required in an office is 300-500 lux and 300-750 in a workshop, the daylight in insufficient.
Finally, by considering the global illuminance the DF was calculated, which shows that these spaces receive very less daylight. Only the spaces near the windows reach 2% DF and most spaces - have 0% DF.
The courtyard is located between the office and the workshop, and hence directly influences the operative temperature in the indoor spaces. However, due to its narrow proportions and its relatively tall surrounding buildings, the courtyard is isolated from environmental impacts to a great extent. Although this may potentially be looked at as a positive in the sense of having a more stable or controlled environment, as is the case with its protection from high wind speeds, however owing to the climate, it leaves the courtyard wanting in terms of both solar radiation and solar access.
Due to the lack of solar radiation, solar gains in the summer period are extremely modest, and are almost negligible in the winter period. Despite this, as shown by the thermal simulations, the courtyard is a fairly comfortable space in the summer period, especially post the early morning hours. In the winters, however, the courtyard remains below the comfort threshold for the entire period. Further, due to its role as a transitional space and thermal regulator for the adjacent office and workshop, the lack of heat gains in the courtyard directly translate to poor heat gains in the indoor space, and the indoor spaces are consequently heavily dependent on mechanical heating.
The lack of solar access is compounded by the use of mostly non-reflective finishes in the courtyard. The poor daylight results due to this were verified through simulations and further through spot measurements, where it has found that the average illuminance in the courtyard was approximately 820 lux when the outdoors illuminance was close to 8,500 lux. This translates to a daylight factor of 10.3, which seeing as it acts as the “outdoors” for the adjacent spaces, is very low and hence leads to the indoor spaces of having daylight factors of around 0.2 with respect to the outdoors, and therefore a large dependence on artificial lighting.
After inferring from the simulations for both periods, the values were listed and then compared with standard values to understand the deficit which would need to be achieved in each of the spaces.
In terms of thermal performance, the temperatures were compared to the values from that of the EN15251 comfort band, which established the threshold that the Operative Temperatures would need to achieve.
Similarly, the percentage of occupied comfortable hours became a reference for the results of further changes in the spaces.
Outdoor Temperature
Summer Period (May to Aug)
17.1°C 7.3°C
Indoor Operative Temperature 19.6°C 12.6°C
Comfort Band Temperatures 21.5 to 27.4°C 18.9 to 24.7°C
Occupied Hours Comfortable (%) 26.0% 0.0%
Temperature Increase Required to Achieve Comfort 1.9 to 7.8°C 6.3 to 12.1°C
Outdoor Temperature
17.1°C 7.3°C
Indoor Operative Temperature 18.2°C 13.4°C
Comfort Band (Mean) Temperature 21.5 to 27.4°C 18.9 to 24.7°C
Occupied Hours Comfortable (%) 69% 18.0%
Temperature Increase Required to Achieve Comfort 3.3 to 9.2°C 5.5 to 11.3°C
Outdoor Temperature
17.1°C 7.3°C
Operative Temperature 19.9°C 10.3°C
Comfort Band (Mean) Temperature
21.5 to 27.4°C 18.9 to 24.7°C
Occupied Hours Comfortable (%) 43% 0%
Temperature Increase Required to Achieve Comfort 1.6 to 7.5°C 8.6 to 14.4°C
Although the primary focus for the team was on thermal performance, daylight also represents an important feature of the spaces, specifically because the activities inside the space require a relatively high level of illuminance as compared to that which is currently achieved.
As understood from daylight analysis, there is a lux deficit in both indoor spaces. Comparing the obtained values and standard ones for office and workshop activities, the required increase in the lux value was established.
Global Illuminance (From St. James Weather Station)
Summer Period (May to Aug)
31,265 lux 5,199 lux
Indoor Illuminance (Mean) 63 lux 10 lux
Indoor Daylight Factor (Based On GI) 0.2
Desired Illuminance
300 to 500 lux 300 to 500 lux
Illuminance Increase Required to Achieve Comfort 237 to 437 lux 290 to 490 lux
Global Illuminance (From St. James Weather Station)
31,265 lux 5,199 lux
Indoor Illuminance (Mean) 32 lux 5 lux
Indoor Daylight Factor (Based On GI) 0.1
Desired Illuminance
300 to 750 lux 300 to 750 lux
Illuminance Increase Required to Achieve Comfort 268 to 718 lux 295 to 745 lux
Global Illuminance (From St. James Weather Station)
Fig 4.5.5.1: Daylight Studies: Summary Table
31,265 lux 5,199 lux
Indoor Illuminance (Mean) 3,220 lux 535 lux
Indoor Daylight Factor (Based On GI) 10.3
The technical studies from a thermal performance point of view, were broken down into three steps. First, each member of the team individually selected a building element which was critical to the thermal performance of both the outdoor (courtyard) and indoor (office & workshop) spaces, and iterated various parameters pertaining to it. In Step 2, combinations of parameters for each element were chosen and iterated for by each team member. Finally, selected combinations from each element were brought together and iterated to give the best overall solution and the best realistic solution.
Case 01
Case 03 (i) (ii) (iii)
01(i), 02(iii), 03 ( )
01(ii), 02(ii), 03 ( )
Case 02
01(iii), 02(i), 03 ( )
01(i), 02(i), 03 (iii)
Combination 1 Combination 2 Combination 3 Combination 4
Case 03 (i) (ii) (iii)
01(i), 02(iii), 03 ( )
Case 01 Case 02
01(iii), 02(i), 03 ( )
01(ii), 02(ii), 03 ( )
Combination 1 Combination 2 Combination 3
01(ii), 02(ii), 03 ( ) Combination 4
Combination 1 Combination 2 Combination 3
Combination 4
Case 01
Case 02
Case 03 (i) (ii) (iii)
01(i), 02(iii), 03 ( )
01(ii), 02(ii), 03 ( )
01(iii), 02(i), 03 ( )
Combination 1 Combination 2 Combination 3
01(iii), 02(i), 03 ( ) Combination 4
Case 01
Case 02
Case 03 (i) (ii) (iii)
01(i), 02(iii), 03 ( )
01(ii), 02(ii), 03 ( )
01(iii), 02(i), 03 ( )
Combination 1 Combination 2 Combination 3
01(iii), 02(i), 03 ( ) Combination 4
To be able to select a building element each for the technical studies, the team made observations based on the thermal simulations, which showed that the major need was to increase the indoor temperature in the winter periods. In the existing scenario in the winter, there are minimal solar gains and the major heat gain can be attributed to opaque conduction (in free-running; otherwise, this would be heat loss). In terms of heat losses, the major aspects were infiltration and glazing conduction. Therefore, the team chose elements based on these factors to achieve the required increase in temperature and comfort to move forward.
The courtyard was chosen as one of the elements to iterate with as part of the technical studies since it is located at a crucial position between the facilities office and the workshop, and therefore acts as their immediate outdoor space and direct source to all environmental determinants - be it solar radiation, daylight, wind or noise.
In view of this, it was quickly realized that altering the parameters of the courtyard may potentially both maximize the comfort of the adjacent indoor spaces as well as maximize the potential of the courtyard itself as a usable outdoor space.
The thermal mass or the exposed mass is an important element as it comprises of the majority of the envelope directly exposed to exterior environment.
The aim while carrying out the iterations was to optimize thermal performance of the space by decreasing heat loss through the thermal mass.
So, to achieve this it was decided that - varying the thickness of the material; varying the thermal capacity of the wall by changing the properties of materials and overall composition; and increasing opaque conduction would be the ideal strategy forward.
As observed in the energy balances of the two spaces, office and workshop, the glazing conduction almost always contributed to a loss of heat. However, in the summer months it showed negligible to positive contribution in heat gains.
Therefore it made sense to explore the glazing properties of the two spaces like, Window to Wall Ratios and the Glass Type and understand the variation each of the properties brought to the space.
Apart from these, the option of night shutters was worth exploring as they have proved to be extremely affective in a climate like London, preventing the unnecessary loss of heat in the night through the glazing.
Opaque conduction had a significant role in the energy balance of both spaces. In a free running mode, the opaque conduction contributed to heat gains in winter and heat losses in summer. Another considerable item influencing thermal performance of spaces was infiltration, which presented a high influence in heat losses in the two periods for both spaces.
Since the goal was to achieve higher temperatures in winter, it was decided that adding thermal insulation materials to opaque elements as well as increasing air tightness of the rooms were relevant strategies to be explored, especially for winter, when there is no solar gains.
Height of Glazing:
Opening %:
Glass Type:
Double Glazed Low-E 4mm +12mm +4mm U-Value: 1.7 W/m2K SHGC: 0.65 VT: 0.74
Height of Glazing:
15m
100% Summers | 0% Winters
Opening %: Glass Type: Height of Glazing: Opening %: Glass Type:
Double Glazed Low-E 4mm +12mm +4mm U-Value: 1.7 W/m2K SHGC: 0.65 VT: 0.74
07m 100% Summers | 0% Winters
Height of Glazing:
Glass Type: 15m 25% Summers | 0% Winters
Double Glazed Low-E 4mm +12mm +4mm U-Value: 1.7 W/m2K SHGC: 0.65 VT: 0.74
Opening %:
07m
75% Summers | 0% Winters
Double Glazed Low-E 4mm +12mm +4mm U-Value: 1.7 W/m2K SHGC: 0.65 VT: 0.74
Comfort
Comfort
From the thermal simulations for the existing case for the summer period, it was observed that the operative temperature in the courtyard was fairly close to the comfort band (∆T 1.6°C). However, in the winter period, it was found that the difference between the comfort threshold and the courtyard operative temperature was ∆T 8.6°C. Therefore, the primary aim for the combinations of various parameters of the courtyard was to maximize heat gain in the winter period. It was found that a glazed roof with lowvalue and high SHGC was effective, however, this combined with other effective parameters for heat gain caused the atrium to overheat in the summer period. A combination of an atrium with a greater height (combination 1 & 2), and operability of the roof in summer proved to maximize summer comfort, however, an atrium with a lower height (combination 3 & 4) showed better results for the winter period.
Brick 300mm
Air gap
Air gap Chipboard 100mm + plaster
Air gap below wood finish in floor
Brick 300mm Air gap Chipboard 100mm + plaster
Air gap below wood finish in floor
Core Air Gap: Finish: Floor:
Brick | 0.3 m | External Wall Thermal Conductivity: 0.6 W/m-KDensity: 1845 kg/m3Specific Heat: 900 J/kg-K
Brick 300mm Air gap Chipboard 100mm + plaster
Air gap
Air gap below wood finish in floor
Brick 300mm Air gap Chipboard 100mm + plaster
Chipboard | 0.1m
Thermal Conductivity: 0.05 W/m-KDensity: 600 kg/m3
Specific Heat: 1000 J/kg-K
Air gap below wooden finish | 0.2m
Lightweight concrete 300mm Chipboard 100mm + plaster
Lightweight concrete 300mm Chipboard 100mm + plaster
Core Material:
Lightweight concrete 300mm Chipboard 100mm + plaster
Lightwt. Concrete| 0.3m|Ext. WallThermal Conductivity: 0.08 W/m-KDensity: 200 kg/m3
Air Gap:
Specific Heat: 840 J/kg-K
No air gap
Lightweight concrete 300mm Chipboard 100mm + plaster
Chipboard | 0.1m
Lightweight concrete 500mm Chipboard 100mm + plaster
Thermal Conductivity: 0.05 W/m-KDensity: 600 kg/m3Specific Heat: 1000 J/kg-K
No air gap
Lightweight concrete 500mm Chipboard 100mm + plaster
Lightweight concrete 500mm Chipboard 100mm + plaster
Lightwt. Concrete| 0.5m|Ext. WallThermal Conductivity: 0.08 W/m-KDensity: 200 kg/m3Specific Heat: 840 J/kg-K
Lightweight concrete 500mm Chipboard 100mm + plaster
Air Gap: Finish: Floor:
Finish: Floor: Core Material:
Brick 500mm Chipboard 100mm + plaster
No air gap
Brick 500mm Chipboard 100mm + plaster
Brick 500mm Chipboard 100mm + plaster
Chipboard | 0.1mThermal Conductivity: 0.05 W/m-KDensity: 600 kg/m3Specific Heat: 1000 J/kg-K
No air gap
Brick 500mm Chipboard 100mm + plaster
Core Material: Air Gap: Finish: Floor:
Brick | 0.5 m | External Wall Thermal Conductivity: 0.6 W/m-KDensity: 1845 kg/m3
Specific Heat: 900 J/kg-K
Air gap
Chipboard | 0.1m
Thermal Conductivity: 0.05 W/m-KDensity: 600 kg/m3Specific Heat: 1000 J/kg-K
No air gap
Summer Winter
Band
Existing
-15%
Summer Winter Comfort Band
Temp. Increase from Existing
Combination
-15% 10% 35% 60% 85%
Hourly (Occupied) Comfort % 0% 5%
0%
2%
Multiple different compositions for the walls were tried before settling on combinations that established trends and showed improvement.
Combination 1 is the exceptional case taken into consideration to understand how air gap in the floor behaves. This combination shows the highest increase in the variation of operaitve temperature in summer, however in winter it shows a negative result.
As expected, Combination 2 & 3 show the best results – since they consist of a lighter core material and a lighter finish. The operative temperature in winter is higher. In the workshop for combination 2, there is an increase in winter months and even though it is null in summer – the comfort percentage is slightly more. Combination 4 is the realistic one with just a slight alteration in the finish which shows better results than the existing case.
Based on the inferences from Step-1 & Step-2 of the technical studies, the team selected four combinations bringing together all elements to arrive that the best combined solution. The key considerations for selecting these combinations were to increase the temperature in the winter period to achieve comfort, while not overheating the spaces, especially the courtyard, in the summer period.
WINDOWS
Height of Glazing: Opening %: Glass Type:
07m
100% Summers 0% Winters
Double Glazed Low-E
4mm +12mm +4mm U-Value: 1.7 SHGC: 0.65 VT: 0.74
Height of Glazing: Opening %: Glass Type:
07m
100% Summers 0% Winters
Height of Glazing:
15m
THERMAL INSULATION
Core Material:
15%
Double Glazed Low E 4mm+12mm+4mm
U- Value: 1.7 SHGC: 0.65 VT: 0.75
Summer: OFF Winter Office:1400-0600 hrs
Winter Workshop: 1700-0900 hrs
Lightweight Concrete | 0.5 m
Thermal Conductivity: 0.08 W/m-K
Density: 200 kg/m3
Specific Heat: 840 J/kg-K
Double Glazed Low-E 4mm +12mm +4mm U-Value: 1.7 SHGC: 0.65 VT: 0.74
Opening %: Glass Type:
100% Summers 0% Winters
Double Glazed Low-E
4mm +12mm +4mm U-Value: 1.7 SHGC: 0.65 VT: 0.74
Height of Glazing: Opening %: Glass Type:
07m
75% Summers 0% Winters
Double Glazed Low-E
4mm +12mm +4mm U-Value: 1.7 SHGC: 0.65 VT: 0.74
WWR: Glass Type: Shutters: WWR: Glass Type: Shutters: WWR: Glass Type: Shutters:
No air gap
Chipboard | 0.1m
Thermal Conductivity: 0.05W/m-K Density: 600 kg/m3
Specific Heat: 1000 J/kg-K
No air gap
Polyurethane | 0.1m | Outer layer
Thermal Conductivity: 0.028 W/m.K Density: 30 kg/m³
Specific Heat: 1470 J/kg.K
Ideal Workshop: 0.27 ac/h Office: 0.7 ac/h
Uninsulated
15%
Double Glazed Low E 4mm+12mm+4mm U- Value: 1.7 SHGC: 0.65 VT: 0.75
35%
Double Glazed Low E 4mm+12mm+4mm U- Value: 1.7 SHGC: 0.65 VT: 0.75
Summer: OFF Winter Office:1400-0600 hrs Winter Workshop: 1700-0900 hrs
WWR: Glass Type: Shutters:
35%
Double Glazed Low E
5mm U- Value: 3.7 SHGC: 0.61 VT: 0.68
Summer: OFF Winter Office:1400-0600 hrs Winter Workshop: 1700-0900 hrs
Combination III
Brick | 0.3 m | External Wall
Thermal Conductivity: 0.6 W/m-K
Density: 1845 kg/m3
Specific Heat: 900 J/kg-K
Summer: OFF Winter Office:1400-0600 hrs Winter Workshop: 1700-0900 hrs
Core Material:
Air gap
Chipboard | 0.1m
Thermal Conductivity: 0.05 W/m-K Density: 600 kg/m3
Specific Heat: 1000 J/kg-K
Air gap below wooden finish | 0.2m
Polyurethane | 0.1m | Outer layer
Thermal Conductivity: 0.028 W/m.K Density: 30 kg/m³
Specific Heat: 1470 J/kg.K
Ideal Workshop: 0.27 ac/h Office: 0.7 ac/h
Brick | 0.5 m | External Wall
Thermal Conductivity: 0.6 W/m-K Density: 1845 kg/m3
Specific Heat: 900 J/kg-K
Brick | 0.5 m | External Wall
Thermal Conductivity: 0.6 W/m-K
Density: 1845 kg/m3
Specific Heat: 900 J/kg-K
Air gap
Chipboard | 0.1m
Air Gap: Finish: Floor: Core Material: Air Gap: Finish: Floor: Core Material: Air Gap: Finish: Floor:
Air gap
Chipboard | 0.1m
Thermal Conductivity: 0.05 W/m-K Density: 600 kg/m3
Specific Heat: 1000 J/kg-K
Combination III Combination IV Combination III Combination II Combination IV
No air gap
Polyurethane | 0.1m | Inner layer
Thermal Conductivity: 0.028 W/m.K Density: 30 kg/m³
Air Gap: Finish: Floor: Wall: Infiltration: Floor: Wall: Infiltration: Floor: Wall: Infiltration: Floor: Wall: Infiltration: Floor:
Polyurethane | 0.2m
Thermal Conductivity: 0.028 W/m.K Density: 30 kg/m³
Specific Heat: 1470 J/kg.K
Specific Heat: 1470 J/kg.K
Ideal Workshop: 0.27 ac/h Office: 0.7 ac/h
Thermal Conductivity: 0.05 W/m-K Density: 600 kg/m3
Specific Heat: 1000 J/kg-K
No air gap
Combination I Combination IV Combination IV
Polyurethane | 0.1m | Inner layer
Thermal Conductivity: 0.028 W/m.K Density: 30 kg/m³
Specific Heat: 1470 J/kg.K
Limitations: Below are the limitations in terms of elements which were unable to be incorporated in the final combinations (Step-3):
Although not incorporated in the final simulations, from Step-1 & Step-2, it may be assumed that night shutters will further increase in the operative temperature by approximately 1.5C in the winter period, which may further reduce heating loads.
Combination I Combination IV
Ideal Workshop: 0.27 ac/h Office: 0.7 ac/h
Uninsulated
Uninsulated
Combination III Combination IV Combination III Combination I Combination III
Although infiltration was unable to be optimized in the final simulations, from Step-1 & Step-2, it may be assumed that this can further reduce heat losses and consequently increase operative temperature.
As can be observed from the temperature and comfort graphs (fig 5.3.2.2), Combination-2 has the maximum rise in temperature and comfort from the base case. This can be attributed to the fact that this combination has highly insulated walls and floors which therefore reduce heat losses in the office. In the other combinations, fairly similar results to the existing scenario can be observed. This is fairly satisfactory since the mean operative temperature of the office can be observed to be quite close to the comfort threshold.
750.00 1000.00 1250.00 1500.00 1750.00
1900
1900
Summers: N/A
Winters (Free Running): N/A
Winters (Heated): 0600 2000
Min. Indoor Temperature
Ventilation
Glazing Area Operable
Shutters
Mechanical Ventilation
Heating Set Temperature (to maintain a 85% comfort level)
Fig 5.3.2.1: Graph showing mean OT achieved by the various Combinations | Summer Period | Office
Fig 5.3.2.2: Graphs showing Net Temperature Increase and Comfort Percentages for the various Combinations | Summer | Office
In the winter in a free-running scenario, it was found that Combination-2 conversely drops in temperature as compared to the base case (refer fig 5.3.3.2). This is due to the fact that its insulated walls and floors significantly reduce the heat gains within the space. In the other combinations, especially Combination-1, it was observed that the temperature increase from the base case was quite significant (upto 19%). This may be attributed majorly to the low U-Value and high SHGC glass used for both the glazed atrium roof and for the windows as well as to the low conductivity & density core material.
21.8°C (Mean CB)
Area Operable
Night Shutters
Mechanical Ventilation
Heating Set Temperature (to maintain a 85% comfort level)
7.3°C (Mean DBT)
Temp. Increase from Existing (Free Running) Temp. ( C)
0.00 5.00 10.00 15.00 20.00 25.00 30.00
Outdoor
NOVEMBER DECEMBER JANUARY FEBRUARY 0.00 250.00 500.00 750.00 1000.00 1250.00 1500.00 1750.00
Solar Radiation ( Wh /m 2) 12.6 C (Mean OT)
Fig 5.3.3.1: Graph showing mean OT achieved by the various Combinations | Winter Free Running | Office
12.6 C (Mean OT) 21.8°C (Mean CB)
25%
Temp. ( C)
-25%
Fig 5.3.3.2: Graphs showing Net Temperature Increase and Comfort Percentages for the various Combinations | Winter Free Running | Office
10.00 15.00 20.00 25.00 30.00 35.00
NOVEMBER DECEMBER JANUARY FEBRUARY 0.00 250.00 500.00 750.00 1000.00 1250.00 1500.00 1750.00 0.00
Fig 5.3.3.3: Graph showing mean OT achieved by the various Combinations | Winter Heated | Office
Solar Radiation
Fig 5.3.3.4: Graphs showing Net Temperature Increase and Comfort Percentages for the various Combinations | Winter Heated | Office
In the existing scenario since the office does not achieve comfortable temperatures, there is a high dependence on mechanical heating in the space. It is heated to a set-point temperature of 24°C from 0600-2200 hrs for eight months of the year from September to April. This leads to significant heating loads of around 5,209 kWh annually. It was found that with the proposed combinations, heating loads could be reduced by approximately 71% to around 1,500 kWh. Combination-2 has the lowest heating loads which can be attributed to its insulated walls & floors, which reduce heat loss from the heated space.
Cumulative Heating Load (kWh)
0 100 200 300 400 500 600 700 800 900
Existing Case: Optimized Existing Case: Combination 1: Combination 2: Combination 3: Combination 4: 5,209 kWh 3,565 kWh 1,558 kWh 1,501 kWh 1,647 kWh 1,601 kWh
January Febraury March April May June July August September October November December
Existing Optimized Combination-1 Combination-2 Combination-3 Combination-4
Fig 5.3.4.1: Graph showing Heating Loads required for various Combinations | Monthly | Office
For the workshop in the summers, it was found that none of the combinations have a significant increase in temperature or comfort as compared to the base case. Similar as to in the office, Combination-2, due to its insulation is marginally warmer that the other combinations. However, since this is a wood workshop space, the occupant has a high met value, which allows him to be comfortable for a large part of the summer period without any major increase in the indoor operative temperature.
(Mean
°C (Mean
Number
Lighting
Occupancy Schedule
Lighting Schedule
Equipment Schedule
Heating Schedule
1300
1300
1300
Summers: N/A
Ventilation
Min. Indoor
Glazing Area Operable
Night Shutters
Heating Set Temperature (to maintain
level
Closed from 1700 0900
Summers: N/A
Winters (Free Running): N/A
Winters (Heated) Combination I:
Winters (Heated) Combination II:
Winters (Heated) Combination III:
Winters (Heated) Combination IV:
(dispersed
Winters (Free Running): N/A
Winters (Heated): 0600 2000
Temp. C)
15.00 20.00 25.00 30.00 35.00
0.00 250.00 500.00 750.00 1000.00 1250.00 1500.00 1750.00 0.00 5.00
Fig
Graph showing mean OT achieved
In the free-running scenario, similar to as in the office, an increase in operative temperature and comfort can be observed in Combination-1 in the workshop due to the low U-Value and high SHGC glass used for both the glazed atrium roof and for the windows as well as to the low conductivity & density core material. Combination-2 has a significant reduction of 23% in operative temperature and in comfort since its insulated walls and floors reduce heat gains. This reduction is more pronounced in the workshop than in the office due to the larger amount of exposed surface area of the workshop to the soil.
°C (Mean CB)
13.4°C (Mean OT)
7.3°C (Mean DBT)
Temp. (
C)
Summers:
Summers:
Summers:
Summers:
Winters (Free
Winters (Heated) Combination I:
Winters (Heated) Combination II:
Winters (Heated) Combination III:
Winters (Heated) Combination IV:
30.00
Wh /m
500.00
1000.00 1250.00 1500.00 1750.00 0.00 5.00 10.00 15.00 20.00
NOVEMBER DECEMBER JANUARY FEBRUARY
Fig 5.3.6.1: Graph showing mean OT achieved by the various Combinations | Winter Free Running | Workshop
Solar Radiation
0.00
Fig 5.3.6.2: Graphs showing Net Temperature Increase and Comfort Percentages for the various Combinations | Winter Free Running | Workshop
°C (Mean CB)
13.4°C (Mean OT)
Temp. (
C)
500.00 750.00 1000.00 1250.00 1500.00 1750.00 0.00 5.00 10.00 15.00 20.00 25.00 30.00 35.00 7.3 C (Mean DBT)
NOVEMBER DECEMBER JANUARY FEBRUARY 0.00
Fig 5.3.6.3: Graph showing mean OT achieved by the various Combinations | Winter Heated | Workshop
Solar Radiation
Temp. Increase from
Fig 5.3.6.4: Graphs showing Net Temperature Increase and Comfort Percentages for the various Combinations | Winter Heated | Workshop
In the existing scenario since the workshop does not achieve a comfortable operative temperature in the winter, there is a high dependence on mechanical heating in the space. It is heated to a set-point temperature of 24°C from 0600-2200 hrs for eight months of the year from September to April, which results in to significant heating loads of around 7,220 kWh annually. It was found that with the proposed combinations, heating loads could be reduced by approximately 78% to around 1,554 kWh. Combination-1 has the lowest heating loads.
Cumulative Heating Load (kWh)
0 200 400 600 800 1000 1200
Existing Case: Optimized Existing Case: Combination 1: Combination 2: Combination 3: Combination 4: 7,220 kWh 3,676 kWh 1,554 kWh 2,434 kWh 1,872 kWh 1,895 kWh
78% reduction in annual heating loads
January Febraury March April May June July August September October November December
Existing Optimized Combination-1 Combination-2 Combination-3 Combination-4
Fig 5.3.7.1: Graph showing Heating Loads required for various Combinations | Monthly | Workshop
In the summers, the existing courtyard space (without a glazed roof) is fairly comfortable, especially after the early morning hours, and can be proposed to be used as pleasant outdoor space for the facilities team. Therefore, each of the combinations have been done considering either 75% or 100% of the atrium roof opened, so as to not overheat this space and hence they achieve similar comfort to the existing scenario.
In the winters, however, a significant impact of the enclosed atrium can be observed. As was seen in the free-running winter scenario for the indoor spaces, Combination-1 had the maximum increase in operative temperature from the existing scenario due to the low U-Value and high SHGC glass used for both the glazed atrium roof and for the windows as well as to the low conductivity & density core material. It was observed (refer fig 5.3.8.4) that Combination-1 has the highest increase in temperature for the outdoor space as well. This reiterates the important relationship between the indoor and outdoor spaces, and the role of the courtyard as a thermal regular.
°C (Mean CB)
(Mean OT)
C (Mean DBT)
Temp. ( C)
500.00 750.00 1000.00 1250.00 1500.00 1750.00 0.00 5.00 10.00 15.00 20.00 25.00 30.00 35.00
0.00
Wh /m 2)
Solar Radiation
MAY
Fig 5.3.8.1: Graph showing mean OT achieved by the various Combinations | Summer Period | Courtyard
Summer
Fig 5.3.8.2: Graphs showing Net Temperature Increase and Comfort Percentages for the various Combinations | Summer Period | Courtyard
500.00 750.00 1000.00 1250.00 1500.00 1750.00 0.00 5.00 10.00 15.00 20.00 25.00 30.00 35.00 7.3 C (Mean DBT) 10.3°C (Mean OT)
°C (Mean CB)
from
Temp. ( C)
NOVEMBER
Fig 5.3.8.3: Graph showing mean OT achieved by the various Combinations | Winter Period | Courtyard
Radiation
Temp.
Fig 5.3.8.4: Graphs showing Net Temperature Increase and Comfort Percentages for the various Combinations | Winter Period | Courtyard
Some strategies were considered to improve the extremely poor daylighting conditions in the two spaces. First and foremost the option of changing the reflectivity of the materials was considered. This did show significant improvements in the existing conditions for daylight as seen in Fig: 5.4.1. The Spatial Daylight Autonomy showed an increase of about 30% in the two spaces. However it remained closer to the windows most of the times. More so over, the addition of furniture and materials could reduce the improved conditions. Apart from this, the lighting loads could be reduced by using more efficient lighting fixtures. Sensors have also proved to be extremely beneficial which can moderate the lux values of the fixtures by sensing the overall lux value in a space.
Changing Material Reflectivity could show significant changes in the overall daylight in a space
Using efficient Lighting Fixtures could greatly reduce the lighting loads for a space
Light sensors can ensure the reduction in lighting loads by moderating lux levels for light fixtures based on daylight received
Task Lighting can also contribute to reducing lighting demands by catering to localized needs rather than lighting the entire room.
Comparison between Existing and Improved Case for Annual Daylight SimulationsIn this study the team set out to investigate the performance of a basement from an environmental perspective and how the indoor (workshop and office) and outdoor (courtyard) spaces can bring comfort to the users.
Data loggers and spot measurement results on air temperature, illuminance and wind were later confirmed by base case simulations. It was concluded that the courtyard is slightly milder than Bedford Square in terms of temperature (by ~1°C), but follows a similar trend to the outdoor space. Both indoor rooms perform poorly in winter due to the lack of solar radiation, however during the summer, temperatures are fairly close to the comfort band.
From simulations, some key findings can be highlighted. Solar radiation is minimal almost throughout the entire year, making passive solar heating unfeasible for both office and workshop. Ventilation rate is low in the courtyard environment.
As for daylight, standard UDI and DA values for office and workshop activities are not satisfied due to the basement’s H/W ratio and low reflectance finishing materials of the courtyard walls.
Thermal performance studies concluded that infiltration and glazing conduction are the main causes of heat loss during winter in both indoor spaces. Opaque conduction is a theoretical heat gain in a free-running scenario, however in reality since the space is heated during this period, the performance of opaque conduction reverses, which indicates an interesting influence of soil temperature to surfaces exposed to it.
It was understood that thermal comfort of the three spaces can be reached with minor changes during summer: The
courtyard already offers pleasant conditions from 09.00 onwards. In the workshop, the considered met and clo values of the carpenter allows him to be comfortable comparatively easily. Based on occupant surveys, adaptive opportunities alone for office users (open windows, use of blinds and adjusting layers in clothing) helps make them comfortable during the summer months.
However, for winter the spaces are largely dependent on mechanical heating to achieve comfort. From this, the next strategy was to prioritise heating conditions. Another step was to compare free running performance of indoor spaces with the current heated scenario to investigate how to bring down heating loads. At first; heating schedules were adjusted, temperature set point was changed and comfortable hours threshold was altered. This helped bring down heating loads in the office and workshop by 32% and 49%, respectively.
As a step forward, making changes in the courtyard, windows, thermal mass, and thermal insulation allowed the group to identify what factors could help bring down the heating load even further. Some major takeaways from iterating with these elements were as follows:
Maximum swing can be seen when atrium height is altered. When increased, comfort hours in summer also rise and in winter they go down. This could be attributed to augmented solar exposure of the glazing structure in summer, therefore the atrium heats up and contributes to trapping the heat. Yet, as solar exposure is minimal in winter, the higher the glazing is positioned, the bigger the courtyard’s air volume, thus, more cool air is trapped in the space.
Glazing conduction contributed to significant heat loss, hence iterating the properties and U-value of the glass
displayed changes in the final result. Increasing the U-value means more comfortable hours in summer and the opposite in winter.
Adding insulation and an air gap to floor layers leads to a significant decrease in temperature with heating turned off. In this scenario, the percentage of comfort hours in summer rises and greatly reduces in winter. However, simulating these parameters combined with heating resulted in the best option for reducing heating loads.
Finally, increasing the percentage of workshop wall area in direct contact with the soil drastically increases comfort in winter and slightly reduces it in summer for free running mode. This helps prove the reason opaque conduction was a source of heat gain in the energy balance in tehe free-running scenario.
These analysis led to the following combinations for final conclusions:
Office : A combination with insulation and air cavity added to the floor is the best solution to reduce heating loads by 71%.
Workshop : A combination with minimum atrium height, low density materials and insulated walls is the best solution to cut down heating loads by 78%.
Lastly, options for improving daylight were explored. All office, workshop and courtyard’s surfaces finishing materials were turned white in the simulation - to maximise reflectance. This showed an increase of Spatial Daylight autonomy by 30%. However, the improvement was mainly concentrated near the windows. It can be concluded that alternative light bulbs (fluorescent to LED) need to be added as another solution because changing reflectance alone cannot help save much energy.
The first four months of this course have been both exhilarating and exciting. With various theoretical subjects combined with practical and computer based tools, this Building Studies project provided us with the ideal platform to explore both, hand in hand. Starting from analyzing the space, to interviews and surveys, to carrying out spot measurements, and finally to iterating using computer based tools, the project kept us engaged with new learnings and challenges at every step.
Our space being in the basement gave us the opportunity to understand the thermal impact of a semi buried space and at the same time understand the effect of a courtyard which acted like any other overground space. The major drawback however was the ratios of the canyon section of the courtyard causing minimal heat and light to reach our spaces and thereby worsening the situation. On one hand even though the space felt unappealing and disadvantageous but on the other hand it gave us the perfect starting point to dig even deeper.
Upon carrying out on-site analyses and then using it as a method of calibration made us realize the importance of the ‘base case’ and how it acts as an instrumental step for all the subsequent processes to be near accurate. Results and findings for factors like opaque conduction, ground exposure, infiltrations, glazing conduction, affect of the micro-climate of the courtyard were fascinating to learn and even more satisfying to be able to analyze it quantitatively.
Carrying out the technical studies post the analyses helped us apply our theoretical understandings to a great extent. Observing trends and patterns and actually being able to predict results after the numerous studies and simulation exercises felt extremely elating and fulfilling. Finally, I would just like to add that apart from learning about environmental design, this project helped me develop my soft skills extensively with a lovely group of cohorts who have been nothing but enthusiastic all this while!
-SURABHI AGARWALTerm 1 was an exponential learning curve for me when it came to exploring different aspects of environmental design. Not only did this semester and particularly the team project, enhance my learning about optimizing thermal and visual comfort, but I also gained a whole new perspective on design. While I had always paid close attention to bio climatic design and vernacular techniques that can help enhance the user experience; working on an existing project – allowed me to explore the technical parameters and analyze them in minute detail.
The spaces assigned to us – at first seemed to be unconventional, spaces that are rather left untouched in a building. However, the more we investigated- the more questions opened up. A cold basement – with little to no direct sunlight had first looked like a bleak space, it was now an opportunity to explore widely. It was interesting to strategize and aim the analysis in a way that it concentrates on the deficits. Unique features of our spaces that made the study compelling were: the courtyard’s microclimate, opaque conduction through the ground which regulated indoor temperatures, understanding sensitivity of the user and adjusting solutions accordingly etc.
We found that while large scale interventions like converting the courtyard into a conservatory can make a big impact, even something as small as changing the thickness/ color of the finish and/or its density can make a difference.
Finally, I think that the analysis of the facilities helped us understand that user comfort and reduction in energy usage can be possible even in such basements and subtle changes made to the existing space can also create change. Overall, after understanding all parameters, their inter relationship and successfully arriving at a design proposal that can majorly change the user comfort- I feel confident and excited to apply my learnings from this project to my future designs.
To study a building during months to which we have access, that we are able to explore, to presence its performance and how it can respond to climate has definitely made the investigation more intriguing. Limitations of thermal comfort models as EN was an interesting learning. As it does not consider the metabolic rate of the users in the space, the comfort results can show a non-realistic situation. This was noticed when comparing thermal simulations to what was reported by the carpenter in interviews. Realising from experience that parameters can generate inaccurate information added to my critical thinking.
Although users adopt adaptive opportunities to feel more comfortable, they can also make the mistake of overusing some systems, as happened with the heating in both spaces. Through calculations, we noticed that the schedule of the heating system could be optimized to reduce the amount of energy spent with heating. I realized from this the importance of post occupancy evaluation and how consultants can help improve performance of an existing building. In addition to this, communication with the users is essential, so they can know how to maintain the indicated conditions.
The technical studies part was particularly satisfying as I could further investigate thermal performance of building envelope. Through theory readings, I could understand the role of envelope’s exposed area, thermal insulations of elements and ventilation in buildings’ heat loss and simulations allowed me to have a more quantitative perspective.
Being from a tropical climate country, it was interesting to see how exposed mass to the soil can also contribute to increase indoor temperature in winter because of the stability of ground temperature. This was used by vernacular Brazilian architecture as a strategy to cool houses, and seeing it perform in a cold climate was definitely enriching.
As someone with a keen interest in both architectural sustainability and historical architectural precedents, the process of studying spaces within the Architectural Association as part of our Term-1 project provided an intriguing opportunity. However, this study came with its set of challenges - with our allocated space of the facilities office, workshop and courtyard being located in the basement and hence being largely unexposed to the outdoor environment and further with the school’s building being a more than 200-year-old Georgian construction, which was originally built as a home.
I was pleased with the way the study progressed from our initial visits to the space, to taking spot measurements and interacting with the occupants, to further using data loggers to get more precise temperature readings; all while simultaneously using the different simulation tools of Ladybug, Honeybee, Open Studio, Energy Plus, Autodesk CFD and soft computations to question and verify our fieldwork results.
These studies allowed us to observe, analyse and eventually understand the space’s performance- related variables. Although this was a complex space, I feel we were successfully able to narrow down certain key elements as part of our technical studies which helped us understand the role of the courtyard as a thermal regular, the impact of the space being in a basement and being exposed to the soil, the role of the building envelope and various construction & finish materials in the heat loss/ gain relationship and the consequent energy demands to achieve comfort within the indoor spaces.
The understanding of these elements, their relationships with one another and their impact on the overall comfort of the space allowed us to propose new strategies which could potentially reduce the annual heating loads by almost 75%.
-MARINA LIMA VECCHIOExcel MInT Spreadsheet
Autodesk CFD
Ladybug / Honeybee
Open Studio & Energy Plus
Tools
Spot Measurement Tools : Dry Bulb Temperature | Relative Humidity | Illuminance
Data Loggers: Dry Bulb Temperature | Relative Humidity
Thermal Camera
Satel Lite: http://www.satel-light.com/ Meteonorm: https://meteonorm.com/en/ EPW Maps: https://www.ladybug.tools/epwmap/ https://www.bdp.com/en/projects/a-e/bedford-square-london/ https://www.architectsjournal.co.uk/archive/wright-wrights-aa-revamp-revealed https://historicengland.org.uk/images-books/publications/conserving-georgian-victorian-terraced-housing/ https://www.degruyter.com/document/doi/10.1515/9780691213781-toc/html http://www.jamesstevenscurl.com/georgian-architecture-4 http://www.uwe.ac.uk
http://www.18thc-cities.paris-sorbonne.fr/Bedford-estates-in-London.html?lang=en#2 https://passivehouseplus.co.uk/magazine/upgrade/historic-london-house-gets-near-passive-transformation https://www.wunderground.com/
Givoni, B. (1998) Climate Considerations in Building and Urban Design. Van Nostrand Reinhold, the USA
Yannas,S. (1993) Solar Energy and Housing Design. Architectural Association, UK (2019) Environmental Design, CIBSE Guide A. The Chartered Institution of Building Services Engineers, UK
Analysis was carried out to understand the slight differences generated by various weather stations for the same city of London.
Dry Bulb Temperature (
Jan Feb Mar Apr May Jun Jul Aug Sep Oct Nov Dec Bedford Sq 5.1 5.3 7.6 9.5 13.1 16.1 18.5 18.3 15.4 11.5 8.1 6.2 Holborn 5.8 5.8
9.5 12.9 16.1 18.5 18.7 15.8 11.8 8.6 6.8 St.James 6.8 6 8 11 14.1 17 18.9 18.2 16.4 12.6 9.1 7 Gatwick 5.7 4.5 6.7 9.1 12.7 17 17.1 16.7 15.3 11.9 7.7 5.6
C) Annual 11.2 11.5 12.1 10.8
To understand the temperature differences that have taken place over the years and the projected temperatures
Dry Bulb Temperature (
10 15
Jan Feb Mar Apr May Jun Jul Aug Sep Oct Nov Dec
2000 - 2010 6.8 6.9 8.3 11.2 14.4 17.6 19.1 19.7 14.7 11.7 8.9 6.8 2020 5.7 5.4 7.9 9.7 13.1 17.2 18.8 18.5 14.4 10.5 7.9 7.3
2100 7.7 7.8 9.8 11.5 15.1 18.8 21.7 22.6 16.7 12.6 10.5 8.6 0
C) Annual 12.2 11.4 13.6
For Summer Period | Office
For Winter Period | Office