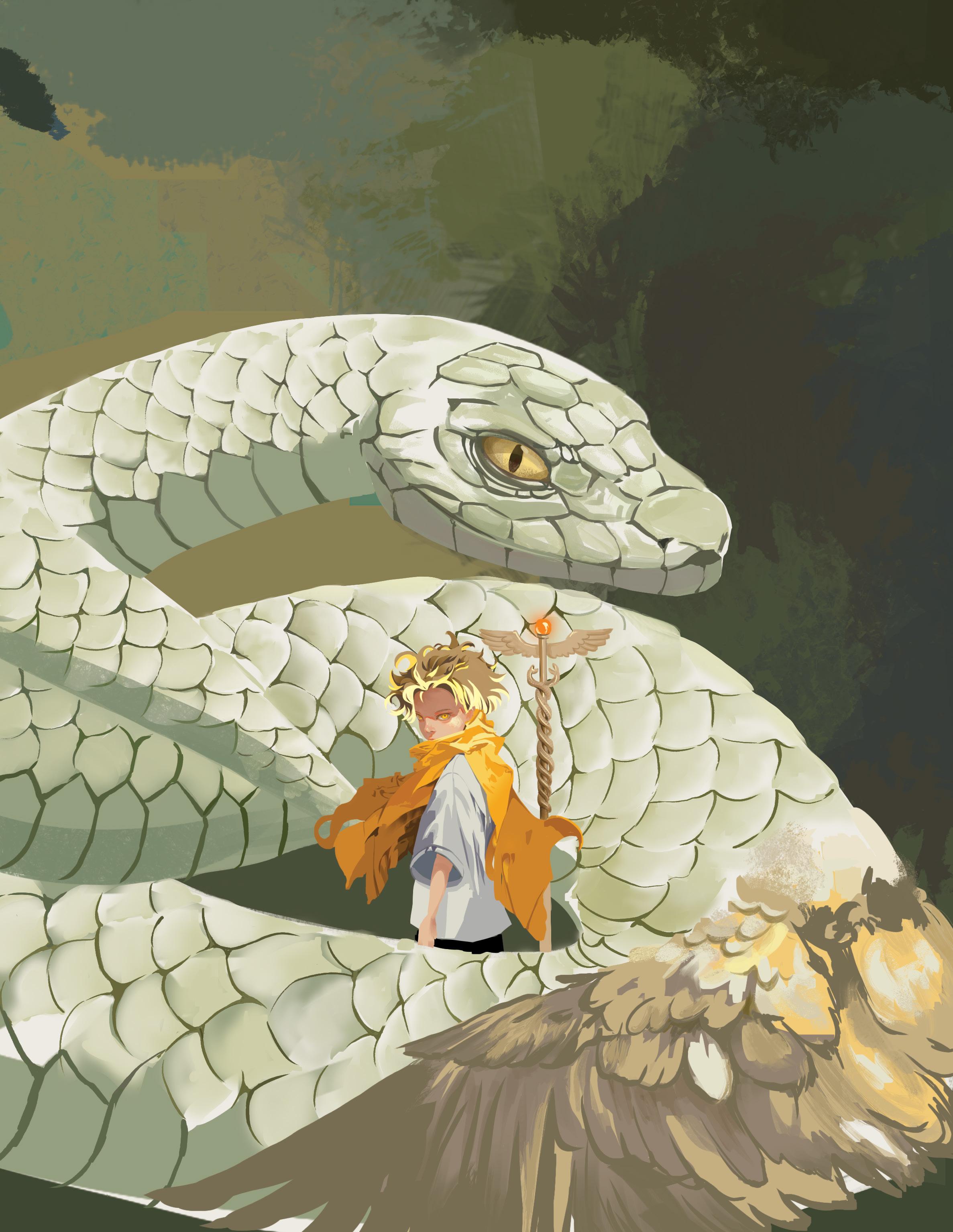
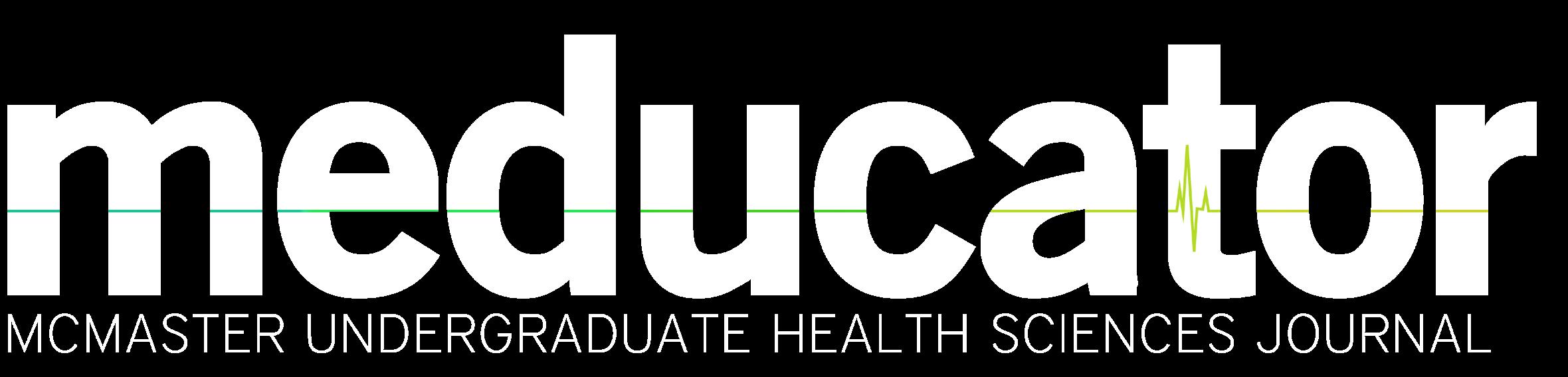
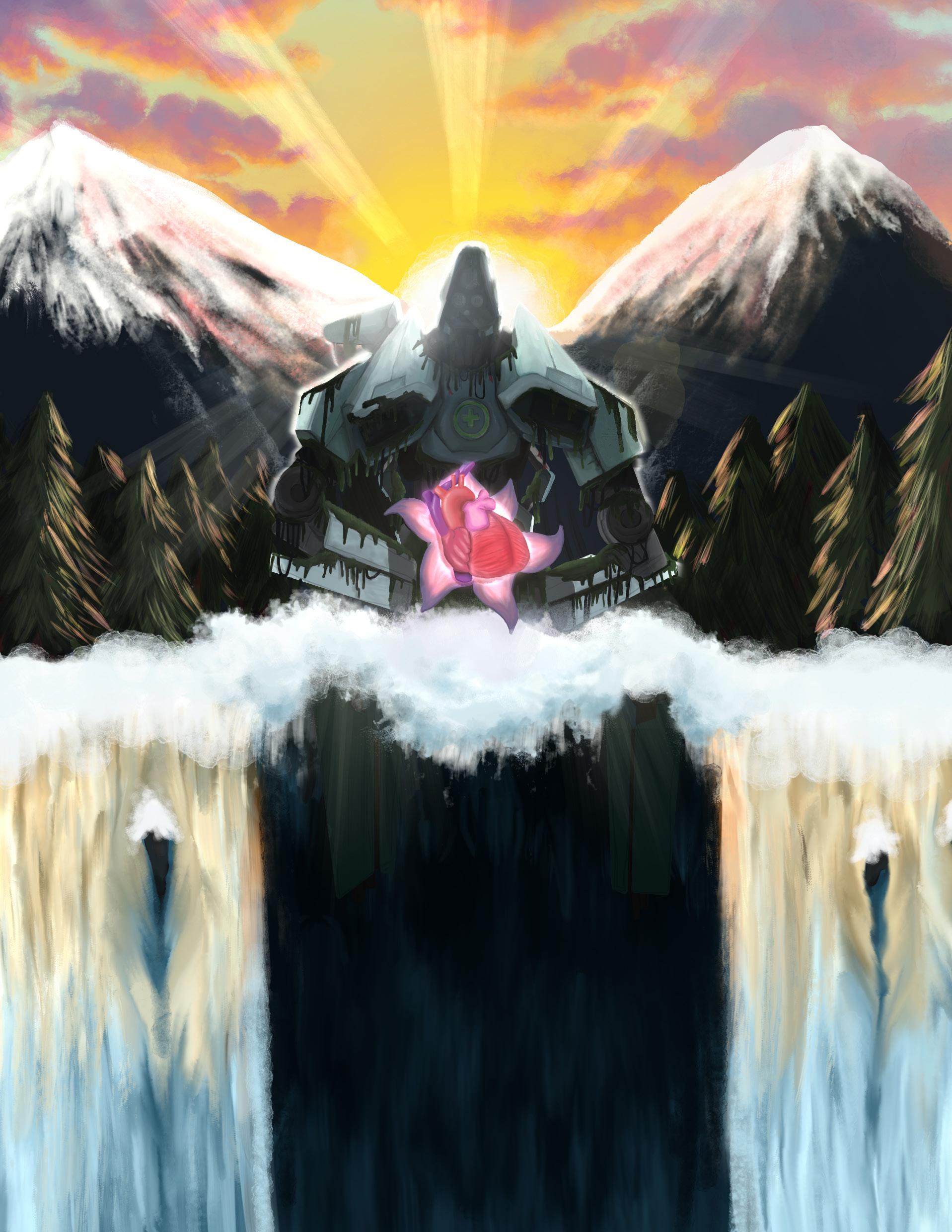
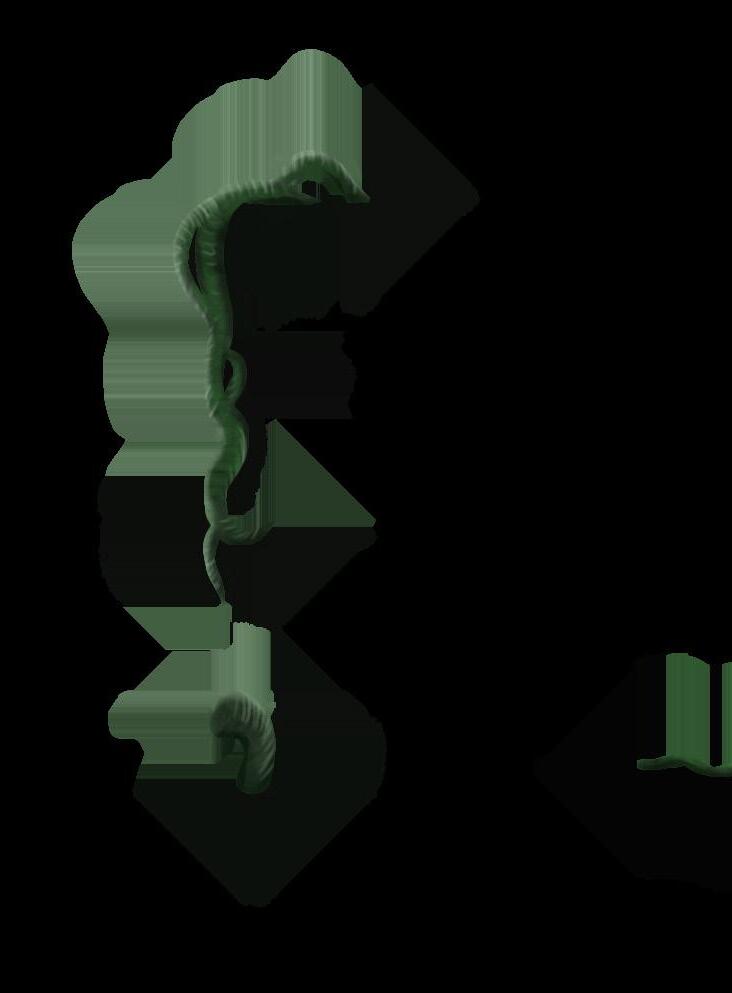
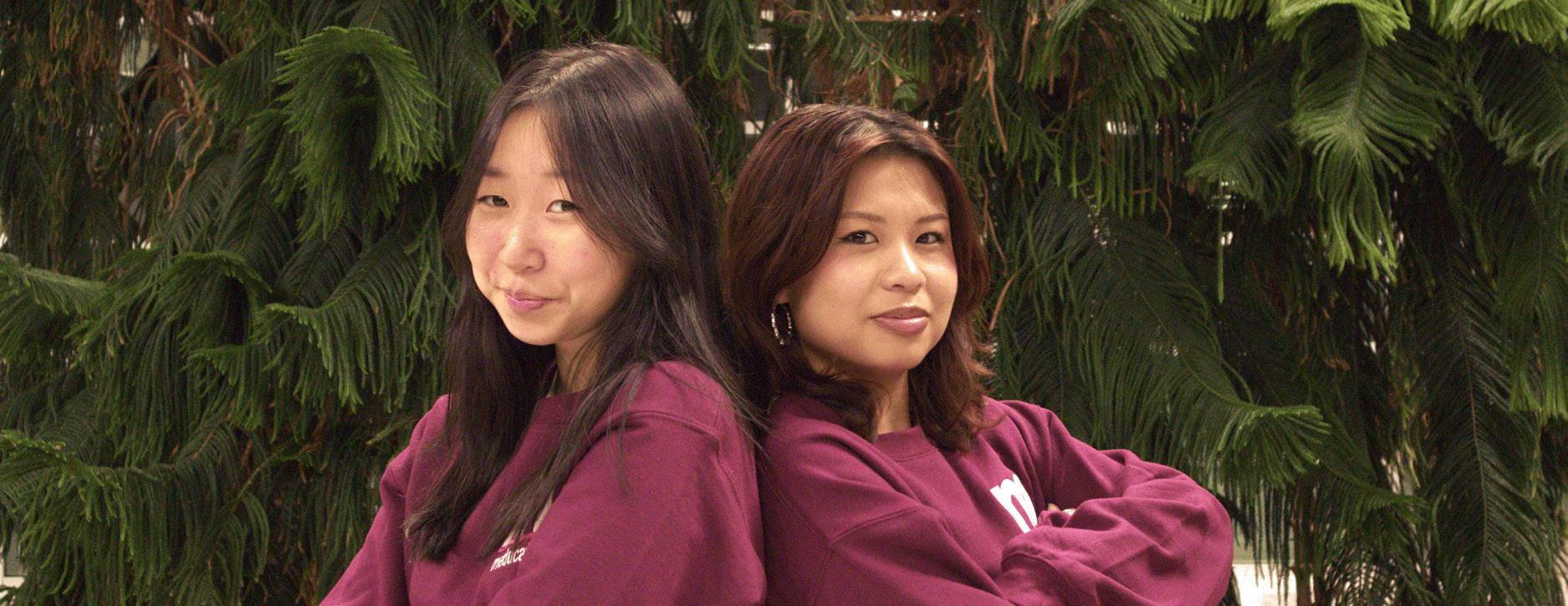
As we welcome a new issue of The Meducator, we reflect on the ebb and flow of change both within our journal and the world at large. Nature—the theme of Issue 46—mirrors this sentiment, ever progressing and renewing. The healthcare field likewise evolves relentlessly and sustainably, driven by advancements that challenge how we think and function. As seasons change, medicine moves in similar cycles: grounding us, reinventing itself, and restoring harmony. Issue 46’s cover, illustrated by Elaine Wang, encapsulates this connection, inspired by the snake associated with Asclepios, the ancient Greek God of medicine. A snake’s ability to shed its skin has rendered it a powerful symbol of the life and renewal that characterizes healthcare.
Opening Issue 46, editors Atta Yazdy, Emily Wang, and Kathy He transcend country borders to describe novel healthcare innovations, while Shehraz Riar and colleagues explore indicators of immune cell-based inflammatory ratios in acute coronary syndrome. Cynthia Duan, Nirujah Sutharsan, and Ruhani Khattra delve into N. fowleri, the brain-eating amoeba, while Prem Patel and Ciara Reid critically review pharmacologic interventions in neonatal seizures, and Hirday Josan and Miran Master discuss early detection in congenital heart defects.
Medicine continuously evolves by challenging existing ideas to nurture innovation. Our team meets with Dr. Ray Truant, Professor of Biochemistry and Biomedical Sciences, to discuss his research on Huntington’s disease. Highlighting a critical biotechnology in cardiovascular disease, Aditya Misra, Devlyn Sun, and Grace Hur review mitochondrial transplantation. From Hippocrates to the modern-era, Matthew Olejarz explores the progression of tuberculosis treatment. In a perspective shift to the future, Evan Zhao, Joel Abraham, and Zahrah Talawala look to the stars as they discuss advancements in aerospace medicine.
Finally, The Meducator takes pride in bridging research with social determinants of health. Stepping outside the walls of McMaster University, authors Aarani Selvaganesh, Angela Hong, and Jia Lu describe the implications of the Hamilton steel industry on air pollution and community health. In a stunning work illustrated by Jessica Palfy, we glimpse into the frustrations of seeking treatment for irregularities for hormonal and menstrual health.
We are endlessly grateful for our 100 talented and dedicated staff, executive members, faculty reviewers, and the McMaster community for their continued efforts throughout the publication cycle. To our executive team, Aarani, Cindy, David, Dominic, Elaine, Eric, Henin, Natalie, Nish, Olivia, Raymond, Ria, Ryan, Serena, Veronica, and Zahra—thank you for your incredible leadership and steadfast faith in us. We would like to extend a special thanks to our sponsors, Dr. Marnix E. Heersink, KRP LLP Chartered Professional Accountants, and the Honours Health Sciences Program, without whom Issue 46 would not be possible. Lastly, we offer our heartfelt appreciation to you, our reader, for the continued support of our publication.
AUDREY DONG
Bachelor of Health Sciences (Honours), Class of 2026
&
FLORENCE DENG
Bachelor of Health Sciences (Honours), Class of 2026
AUTHORS: KATHY HE1, EMILY WANG2, & ATTA YAZDY3
1 Bachelor of Health Sciences (Honours Biochemistry), Class of 2027, McMaster University
2 Bachelor of Health Sciences (Honours), Class of 2026, McMaster University
3 Bachelor of Kinesiology (Honours), Class of 2026, McMaster University
ARTISTS: AMEYA GUPTA4 & PARISA
MISHAL HOSSAIN5
4 Bachelor of Computer Science, Class of 2028, McMaster University
5 Bachelor of Health Sciences (Honours), Class of 2025, McMaster University
Muscarinic modulators show promise in schizophrenia treatment
UNITED STATES OF AMERICA | September 2024
KarXT is a new drug for treating schizophrenia and has recently received approval from the Food and Drug Administration.1,2 This oral medication is the first drug in over 70 years to present a novel mechanism of action.1,2 Unlike conventional dopamine therapies, which often induce movement issues, restlessness, and weight gain, KarXT acts on the M1 and M4 muscarinic receptors in the brain to regulate cognition, manage emotional responses, and improve intellectual function.1, 3 This gives rise to a new generation of innovative drugs for psychiatric disorders.
Closed-loop deep brain stimulation used to treat neurological conditions
BRAZIL | October 2024
Deep-brain stimulation (DBS) is a surgical procedure that involves delivering impulses to strategic points in the brain through an implanted neurostimulator to treat neurological conditions.4,5 Researchers from the University of São Paulo developed a closed-loop DBS system with low-cost commercial components.5,6 While conventional DBS requires stimulation settings to be adjusted every 3 to 12 months after implantation, closed-loop DBS can automatically adjust to brain biomarkers, allowing for adaptable and personalized care.5,6 Future research involves in vivo animal testing.6
Skeletons from Egyptian scribes provide insight into common skeletal disorders
EGYPT | June 2024
Archaeological evidence from the skeletons of ancient Egyptian scribes provides insight into skeletal changes that can arise from occupational risk factors.7 Researchers measured skeletal markers for conditions, such as intervertebral disc disease and osteoarthritis, from remains at the Abusir Necropolis.7 A statistically significant difference was observed between the scribal and reference groups.7 Analysis revealed that extended periods of cross-legged sitting or kneeling, along with tasks related to writing, caused overexertion of the jaw, neck, and shoulder.7 This archaeological evidence on the long-term effects of poor working posture is especially relevant as modern society continues to learn the importance of occupational health and safety.
Researchers at Uppsala University have discovered a new class of antibiotics that are effective against multiple bacteria, including Escherichia coli.8 The antibiotics target the LpxH protein which synthesizes a protective layer that protects bacteria from immune cells, allowing them to remain in its host environment for a prolonged period.9 Several harmful bacteria rely on LpxH, including E. coli, which urgently require novel treatments according to the World Health Organization.8 The antibiotics were successful in treating multiple bloodstream infections in preclinical mouse models.9 These preclinical results provide the foundational knowledge necessary to test these antibiotics in humans.
stem cell transplantation
A 25-year-old woman with type 1 diabetes started producing her own insulin less than three months after transplantation with autologous induced pluripotent stem cells (iPSCs).10 Researchers from Peking University developed the stem cell therapy, involving pluripotent stem cells derived from somatic cells.10 iPSCs are conventionally generated through transcription factors that reprogram cells to express genes associated with pluripotent stem cells.11 However, the iPSCs in this clinical trial were generated by abiotic chemical compounds with low molecular weights, decreasing the risk of mutation and offering more control in reprogramming somatic cells.10
In late 2023, two malaria vaccines, R21 and RTS, were prequalified for purchase by the World Health Organization following clinical trials.12 Malaria is more prevalent in children in sub-saharan Africa and in adults in Asia.12 Researchers in Bangkok tested the R21 vaccine in an adult population, as previous trials only supported its efficacy and safety in children. R21 was determined to be safe and effective in adults, with or without antimalarial drugs.12 The vaccine is another contribution towards globally reducing the risk of malaria.
Researchers at the University of Sydney have revolutionized genetic engineering with their novel discovery of SeekRNA—a new gene-editing tool with improved precision and versatility.13 Derived from RNA bacterial insertion sequences (IS), 1111 and 1100, SeekRNA uses a programmable RNA strand to self-identify target positions for insertion.14 IS1111 and IS1100 have a unique class of transposases that exhibit autonomous cutting and precise transposition abilities.14 Researchers took this principle of IS and demonstrated that by creating a match between both the sequences in SeekRNA and the gene of interest, a new target can be identified to be mobilized and inserted.14 Unlike CRISPR, SeekRNA only requires one transposase protein to cleave and insert and has shown successful results in bacterial gene editing.14 However, further in vitro research is necessary to advance SeekRNA technology.
doi: 10.35493/medu.46.04
AUTHOR: SHEHRAZ RIAR3, DAYAN AMER
3 Bachelor of Health Science (Honours), Class of 2028, McMaster University
4 Bachelor of Science (Honours), Class of 2028, University of Toronto
5 Doctor of Medicine, Class of 2027, University of Toronto
Acute coronary syndrome (ACS) is an umbrella term for a range of cardiovascular conditions characterized by reduced blood flow to the heart. For millions, ACS poses a constant threat of cardiac failure and is especially life-threatening in rural and low-income areas where access to timely diagnosis and intervention is limited. The risk of a recurrent major adverse cardiovascular event (MACE) adds to the concern. Recent studies have begun investigating the role of immune cells in the initiation and progression of atherosclerosis in response to a MACE. This review explores the practicality of using immune cell-based inflammatory ratios, including the lymphocyte-to-monocyte ratio (LMR), neutrophilto-lymphocyte ratio (NLR), and Systemic Inflammation Response Index (SIRI) as prognostic indicators of MACEs in ACS patients. We searched Ovid MEDLINE and PubMed for English peer-reviewed studies. Our findings suggested that leukocyte testing could be an accurate and cost-effective alternative to conventional Troponin I testing. However, some barriers remain, such as limited research on ACS among young and chronically ill patients and insufficient healthcare spending in rural areas. This poses a challenge for the adoption of inflammatory ratios as a prognostic indicator of MACE.
The significance of acute coronary syndrome (ACS) cannot be understated. It represents one of the leading causes of death in Canada with 21,000 ACS-related deaths in 2009 alone.1 Between 2008 and 2009, the direct costs of ACS were approximately $3.4 billion.1 It should be noted that the costs of ACS go beyond deaths as the condition affects both length and quality of life.
Due to a lack of focus on proactive measures, the rehospitalization of 1 in 15 ACS patients within six months of a major adverse cardiac event (MACE) is unsurprising.1 To some extent, the limited longterm effectiveness of MACE treatment can be attributed to the reactive nature of its detection. Clinically, MACEs are identified in emergency settings through a blood test that detects the presence of troponin I (TrI), one of three proteins that form the troponin complex.2 Because TrI is released only after cardiomyocyte death, its detection serves as a marker for myocardial damage.2 To counter this reactive latency, urban hospitals have begun using point of care (PoC) blood tests, reducing testing times by 75% compared to central laboratory (CL) testing.3 Not only do PoC tests improve physician efficiency at identifying the type of MACE, but by circumventing a CL test, they also reduce the total cost of healthcare before intervention.2 After diagnosis, the relative risk of a MACE is typically assessed by calculating a History, Electrocardiogram, Age, Risk factors, and Troponin (HEART) score or Global Registry of Acute Coronary Events (GRACE) score.4-7 Despite these significant advancements for ACS patients, rural populations are rarely addressed in terms of performing timely
This critical review was conducted following the Preferred Reporting Items for Systematic Reviews and Meta-Analyses (PRISMA) search statement. An online literature search was conducted by accessing Ovid MEDLINE and PubMed databases. Keyword searches for both databases are as follows:
OVID MEDLINE SEARCH
1. exp Acute Coronary Syndrome/ (21 417)
2. exp Angina, Unstable/ (11 423)
3. exp Myocardial Infarction/ (199 736)
4. 1 OR 2 OR 3 (222 026)
5. Lymphocyte to Monocyte Ratio*.mp. (1 728)
6. Lymphocyte-to-Monocyte Ratio*.mp. (1 728)
7. Neutrophil to Lymphocyte Ratio*.mp. (14 381)
8. Neutrophil-to-Lymphocyte Ratio*.mp. (14 381)
9. Systemic Inflammation Response Index*.mp. (399)
10. Inflammatory Ratio*.mp. (70)
11. SIRI.mp. (970)
12. LMR.mp. (2 081)
13. NLR.mp. (28 753)
14. Neutrophil to Lymphocyte Ratio*.mp. (14 381)
15. NLR.mp. (28 753)
16. 5 OR 6 OR 7 OR 8 OR 9 OR 10 OR 11 OR 12 OR 13 OR 14 OR 15 (34 698)
17. Cardiovascular Events*.mp. (51 727)
18. Major Adverse Cardiovascular Events*.mp. (9 061)
19. MACE.mp. (13 749)
20. Cardiovascular Mortality*.mp. (18 747)
21. 17 OR 18 OR 19 OR 20 (75 588)
22. 4 and 16 and 21 (85)
(("Acute Coronary Syndrome"[Mesh]) OR "Angina, Unstable"[Mesh] OR "Myocardial Infarction"[Mesh]) AND (("Lymphocyte to Monocyte Ratio"[Title/Abstract] OR "Lymphocyte-to-Monocyte Ratio"[Title/Abstract] OR "Neutrophil to Lymphocyte Ratio"[Title/Abstract] OR "Neutrophil-to-Lymphocyte Ratio"[Title/Abstract] OR "Systemic Inflammation Response Index"[Title/Abstract] OR "Inflammatory Ratio"[Title/Abstract] OR SIRI[Title/ Abstract] OR LMR[Title/Abstract] OR NLR[Title/Abstract] OR "Neutrophil to Lymphocyte Ratio"[Title/Abstract] OR NLR[Title/Abstract]) AND ("Cardiovascular Events"[Title/ Abstract] OR "Major Adverse Cardiovascular Events"[Title/ Abstract] OR MACE[Title/Abstract] OR "Cardiovascular Mortality"[Title/Abstract]))
We restricted our search to journal articles published between August 2004 and August 2024. The studies were required to discuss findings related to hospitalization due to MACE, cardiovascular mortality, myocardial infarction, or general mortality related to a relevant ACS condition. English peerreviewed retrospective and prospective studies were included, while studies involving other interventions or focusing on indicators other than LMR, NLR, and SIRI were excluded. The search terms resulted in 156 sources. After deduplication and two rounds of screening, we included 39 sources (Figure 1).
The three inflammatory cells that are the foundation of this critical review are lymphocytes, monocytes, and neutrophils.4-7,10 During or shortly before a MACE, neutrophil and monocyte counts increase in the bloodstream.7 Neutrophils are leukocytes that typically play a mediating role in inflammation, as seen in ACS. When an ACS patient has or is about to have a MACE, the release of pro-inflammatory cytokines results in neutrophil activation. Neutrophils then localize near plaques via chemokines and release reactive oxygen species and 11-13
Together, the cell counts taken from a CBC of each cell type are used to create a leukocyte ratio.14,24 The LMR, NLR, and SIRI can then be used to determine the likelihood of a MACE using their respective formulas:
For predicting MACEs, LMR, NLR, and SIRI values, thresholds can be chosen by using Youden’s index, which is a statistic that combines sensitivity and specificity into a single value.13 These ratios have shown statistically significant clinical relevance in predicting MACEs in ACS patients. If the values cross their thresholds (low for LMR, high for NLR and SIRI), it indicates a high risk of cardiac inflammation and potential MACE, allowing medical professionals to take appropriate action promptly.
In a meta-analysis including five studies with 4,343 patients, it was suggested that a lower LMR is associated with short and long-term MACEs in ACS patients.25 In a meta-analysis including five studies with 4,343 patients, it was suggested that a lower LMR is associated with short-term (hazard ratio [HR] = 3.44; confidence interval [CI] = 1.46-8.14) and long-term (HR = 1.70; CI = 1.36-2.13) MACEs in ACS patients.25 In another meta-analysis of 14 studies with 10,245 patients, high NLR was found to be a predictor of hospitalizations in STEMI patients (relative risk [RR] = 2.49; CI = 1.47-4.23).28 Finally, in a retrospective analysis investigating SIRI values in 1,724 ACS patients, higher values were associated with worse disease outcomes.29
Ultimately, this leads to the dysregulation of the vascular endothelium where inflammation is occurring. 14 After that, signalling molecules released from the site of inflammation during a MACE mobilize monocytes which secrete lytic enzymes, such as matrix metalloproteinases, to rupture plaque.15 Monocytes can also propagate thrombus production, leading to coagulation cascades.16,17 Finally, lymphocytes tend to decrease around the time of a MACE in a phenomenon known as Circulating lymphocytes move the site of inflammation, which appears as low lymphocyte count in complete blood 7,11,19-23
While there is no doubt that modern intervention methods for MACE in ACS patients play a foundational role in emergency treatment, there is much improvement needed in accessibility and efficiency for this branch of healthcare. For example, the GRACE score aims to predict ACS outcomes by considering age, heart rate, systolic blood pressure, creatinine levels, cardiac arrest on admission, ST segment deviation, abnormal cardiac enzymes, and Killip class.4,24,30,31 However, it is less accurate than inflammatory ratios, such as the NLR, because inflammatory ratios make use of immunologic biomarkers, a feature that the GRACE score does not consider.32 Thus, studies have shown that inflammatory ratios are powerful independent predictors of MACEs and even more effective when used alongside established predictors like GRACE.31,33,34 Another critical tool is the HEART score, in which physicians assign patients a score from zero to two in various cardiovascular disease determinants (e.g., electrocardiogram, age, risk factors), and then use the sum to assess their risk. 35 Despite its comprehensive approach, the HEART score has limited applicability in rural settings due to its dependence on TrI testing.35 On the other hand, calculating inflammatory ratios from CBCs is an effective and relatively inexpensive addition to the established risk assessment system and provides crucial prognostic information about a patient's condition.33,36
1: PRISMA diagram of conducted searches.
Figure 1: PRISMA-S flowchart of searches accessing MEDLINE and PubMed (31 August 2024)
Inflammatory ratios can be used alongside other readings in a CBC such as platelets to categorize whether a condition is recurring, urgent, or nonurgent.37 Furthermore, inflammatory ratios can increase the understanding of patients’ immune profiles and enable more personalized therapeutic approaches, such as anti-inflammatory drugs or immunomodulatory therapies. This level of specificity in treatment would not be available with current risk assessment tools, such as GRACE and HEART.38 This method of personalized care is gaining popularity in other medical sectors and there is potential for it to be used in ACS cases as well.
testing cannot be equitably realized by all.38,41,60,61 The effectiveness of PoC testing is also limited by delays in rural prehospital transportation.62
The application of inflammatory ratios for the treatment of ACS conditions in rural areas cannot be overlooked. We found that the two major issues rural areas have with modern ACS treatment are the prehospital transportation delay and financial costs associated with TrI testing.40-42 TrI requires highly specific blood testing while leukocyte ratios can be derived from a routine CBC, which are quicker and more cost-effective. In addition, CBCs as predictors of MACEs in ACS patients are a significantly more costeffective and accessible alternative to TrI blood testing.
Although proactive leukocyte testing is promising, it has significant limitations in applicability and research scope. Many studies that were reviewed excluded patients with chronic inflammatory conditions, autoimmune diseases, cancers, and other chronic illnesses.26,28-30,45-55 Given the uncertainty among larger patient populations, the practicality of developing leukocyte testing infrastructure in rural and inaccessible populations cannot be entirely assessed. Additionally, many studies contained mean population ages within the older adult range (>60 years) as they represent 30-40% of all hospitalized ACS patients, further introducing bias to the conclusions of these articles. Although articles with younger populations are sparse, a systematic review and meta-analysis of five studies suggests that LMR is a poor predictor of MACEs in young ACS
It is important to address the largely neglected rural ACS demographic to make inflammatory ratios a reality in clinical settings. Urban areas tend to have more established medical centres and adequate funding relative to rural areas. Given the lack of infrastructure in rural areas and large distances from urban hospitals, there is a demand for a MACE predictor that has quicker response time and does not require niche health professionals. Potential avenues for innovation include making non-invasive alternatives to the CBC. One such example is a sublingual biosensor that uses antigens present in saliva to detect inflammatory cells and their concentrations.63 Innovations in the accessibility of blood testing technologies can lessen the gap of quality treatment for ACS between urban and rural communities.
In an era of medicine where researchers are at the cusp of finding new quality-of-life innovations, the use of inflammatory ratios in triaging ACS patients based on MACE risk shows to be a promising development. From its improvement on the current risk assessment framework of GRACE, HEART, and TrI testing, to its relatively inexpensive and simple process, this risk-assessment method for ACS patients has the potential for widespread adoption in urban and rural areas. However, there is a significant literature gap in leukocyte ratio prediction of MACEs among young ACS patients and those with comorbid conditions, which is a major barrier to this practice becoming part of the standard of care.
Thus, conducting further research into the accuracy and practicality of inflammatory ratios as predictors on a broader range of patient populations could greatly improve emergency MACE triaging approaches in ACS patients, decreasing MACE mortality rates in rural areas.
The application of leukocyte testing appears limited beyond its 23-26
The accessibility challenges of leukocyte testing extend beyond age group. For example, 20-30% of the adult population report a fear of needles, which are required for CL and PoC testing in ACS patients. The invasive nature of these tests can cause high stress in these patients, due to being a necessary Fundamentally, leukocyte testing remains a traditional blood test and retains associated disadvantages. With low and middle-income nations across the globe restricting their PoC testing to urban hospitals due to regulatory, financial, and infrastructural limitations, the large-scale benefits of PoC
REVIEWED
BY:
DR. PASCAL DJIADEU (PhD)
Dr. Pascal Djiadeu is an epidemiologist and bio-statistician working with the Public Health Agency of Canada (PHAC). He is an Assistant Professor at McMaster University in the Department of Health Research Methods Evidence and Impact.
EDITED BY: DAVID GOU & FIRDOSE KHAN
AUDIT • FISCALITÉ • SERVICES-CONSEILS
We have your prescription for success
Nous avons une « ordonnance » pour le succès !
The financial decisions you make today will shape your practice tomorrow. Trust the experienced advisors of Baker Tilly for tailored, future-focused solutions.
Expert financial advice — that’s a relief!
Les décisions financières que vous prenez aujourd’hui décideront l’avenir de votre pratique. Vous pouvez faire confiance aux conseillers expérimentés de Baker Tilly pour vous fournir des solutions sur mesure tournées vers le futur.
Des conseils financiers éclairés — quel soulagement !
For more information, please contact the advisors at Baker Tilly:
Locations | Emplacements
Ottawa: 301 Moodie Drive, Suite 400
Ottawa ON, K2H 9C4
T: 613.820.8010
Pour plus de renseignements, veuillez communiquer avec les conseillers de Baker Tilly :
Gatineau :
290, boulevard St. Joseph, bureau 105 Gatineau (Québec) J8Y 3Y3
Tél. : 819 770-0009
doi: 10.35493/medu.46.10
AUTHORS:
CYNTHIA DUAN1, RUHANI KHATTRA2 & NIRUJAH SUTHARSAN3
1Bachelor of Health Sciences (Honours), Class of 2027, McMaster University
2Bachelor of Health Sciences (Honours), Class of 2026, McMaster University
3Bachelor of Health Sciences (Honours), Class of 2028, McMaster University
ARTIST: IRIS QIAN3
Naegleriafowleri, commonly referred to as the “brain-eating amoeba,” is an amphizoic amoeba that exists as both a freefloating organism and a parasite.1,2 Infection by N. fowleri is deadly in humans, exhibiting a fatality rate of 97%.3 As a free-living amoeba, N. fowleri can transition between three phenotypes depending on its environment: trophozoite, flagellate, and cyst. Under nutrient-rich conditions where N. fowleri can proliferate, it assumes a trophozoite form, which is reproductively active and infectious to humans. During this stage, it can cause primary amebic meningoencephalitis (PAM), a rapidly fatal infection of the central nervous system (CNS). Conversely, under nutrient-poor conditions, N. fowleri can temporarily transform into its flagellate form. Though this form is unfavourable for growth, it facilitates long-distance travel to search for nutrients.2,4 Finally, under environmental stress, such as the absence of water and lower temperatures, N fowleri switches into its metabolically inactive cyst form, which can withstand temperatures from 0°C to 65°C.4
While N. fowleri is ubiquitous, it is most commonly found in freshwater contaminated by soil, such as freshwater lakes, hot springs, poorly chlorinated pools, and thermally-polluted water bodies. However, it is generally unable to survive in saline water. First discovered in Australia, N fowleri infections have also been found in New Zealand, Europe, Africa, Asia, Latin America, and the United States.2 Infection is deadly but rare in humans, with only 452 cases documented from 1965 to 2018.5 These cases occurred in 39 countries, with the greatest incidence in the United States (35%), followed by Pakistan (23%) and Mexico
PATHOGENESIS & PATHOPHYSIOLOGY
Trophozoite N. fowleri can move rapidly through bodies of contaminated water.6 Infection is acquired through infected tap water used to rinse the nose and the inhalation of infected airborne particles, such as dust.6 Trophozoites enter through the nasopharyngeal mucosa and secrete cysteine proteases, which degrade the host mucus, allowing the
the trophozoite to penetrate the nasal epithelium.5 They proceed along olfactory nerves to infiltrate the brain via the cribriform plate, a component of the ethmoid bone at the base of the skull.5
N. fowleri is particularly dangerous, with higher levels of adhesion than non-pathogenic amoeba species. Evidence suggests that the attachment of the amoeba to components of the extracellular matrix, the network of proteins providing structure for cells, can activate proteins that facilitate the entrance of N. fowleri into the CNS.5
The pathogenic mechanisms of N. fowleri infection consist of phagocytosis, secretion of proteases, phospholipases, and pore-forming proteins (PFPs). N. fowleri utilise structures resembling suction cups known as amoebastomes to consume neurons through contact-dependent phagocytosis, leading to the progressive destruction of brain tissue.7 The amoeba also prompts the release of PFPs, membrane-bound proteins that degrade the stability of the host cell membrane, causing cell death.9 Additionally, N. fowleri can cross the blood-brain barrier (BBB) by destroying the tight-junction proteins (TJPs) between host endothelial cells. This mechanism involves secreting cysteine protease that degrade TJPs and modify the cell’s cytoskeleton, breaking down the BBB.8 The amoeba also uses phospholipase enzymes to destroy neuronal myelin by degrading phosphatidylcholine, a major structural component of the myelin sheath.
The presence of trophozoites prompts macrophages and other immune cells to release cytotoxic molecules, resulting in necrosis of olfactory tissues.5,9 Further, N. fowleri has evolved to evade immune mechanisms and has become resistant to destruction by cytolytic immune processes.9 Microglia, the neuronal immune cells, activate immune signalling pathways in response to the amoeba for the secretion of cytokines. However, the proteases and signalling molecules secreted by N. fowleri can cause microglial lysis.9 As amoebal growth within the host progresses, a positive feedback loop of leukocytes and pro-inflammatory cytokines is initiated, resulting in uncontrollable CNS inflammation.9 This inflammation prevents the CNS from controlling the infection, leading to fatal haemorrhage and edema.9
Symptoms of PAM include headaches, fever, nausea, and stiff neck. As brain tissue damage becomes extensive, these symptoms progress rapidly, with patients
losing bodily control and experiencing extreme light sensitivity, hallucinations, and coma.7 PAM is challenging to diagnose due to the similar clinical presentation of PAM and bacterial meningitis, lack of pathogenic signs during early physical exams and imaging, and similarity of bacterial meningitis to PAM in CSF analyses.2 CSF analyses for both bacterial meningitis and PAM typically show an elevated protein count, low to average glucose concentrations, and polymorphonuclear cells.2 This can cause misdiagnosis, which can be detrimental given the rapid progression of infection. Not only does death occur seven to ten days post-infection if not adequately treated, but symptoms of PAM can take one to seven days to appear post-infection.11 As such, early diagnosis is critical in managing and treating PAM.
For physicians to detect and diagnose PAM, a thorough patient history must be compiled, identifying recent freshwater exposures and any presentation of CNS symptoms. For a definitive diagnosis, amoebae must be detected in a CSF analysis.2 Other techniques include antigen detection by immunohistochemical staining, polymerase chain reaction, and cultures using CSF or tissue samples.2 Magnetic resonance imaging (MRI) and computed tomography (CT) scans can also be done, but findings may be unremarkable during the critical early stages of infection. In later-stage infection, diffuse haemorrhages or infarctions in the brain’s grey matter may appear in an MRI, while cerebral edema and the destruction of cisterns, enlarged pockets filled with CSF fluid, may be found in CT scans.2,4,12
Due to the rarity, rapid action, and high mortality rate of N. fowleri infections, there are no clinical trials to assess the safety and efficacy of treatment regimens.5,13 The standard of clinical care has been constructed by previous case reports and in vitro studies.5,13 Still, researchers concur that practicing prevention to circumvent treatment entirely is ideal. According to the US Center for Disease Control, individuals should protect themselves by wearing a nose clip while entering fresh water, keeping one’s head above water in hot springs, avoiding digging in shallow water, and using distilled or pre-boiled tap water to rinse nasal passages.2
the site of infection are usually hindered by the BBB.14 One study proposed nanoparticle-based drug delivery using metal carriers, whose non-reactivity and biocompatibility allow them to cross the BBB more efficiently.15,16 Silver nanoparticle-conjugated antiamoebic drugs had enhanced drug potency and reduced N. fowleri-mediated host cytotoxicity.15,16 Further, some studies have shown potential for developing an N. fowleri vaccine. One study identified an antigenic molecule, the Nfa1 protein, which reacted strongly with infected blood sera. Researchers immunised mice with recombinant Nfa1, who exhibited a 100% survival rate post-infection.14 These results were consistent with other studies vaccinating mice with recombinant Nfa1.14 While currently unavailable and awaiting validation, vaccination represents a promising strategy to manage N. fowleri infection.
While yearly infections in the United States have remained stable, recent epidemiological spikes in several tropical regions have caused concern in nations such as India, Australia, and notably Pakistan, where N. fowleri killed 7 individuals.17 This outbreak has compounded the country’s existing healthcare burden, necessitating the government to take proactive public health measures.17 Concerningly, one study suggests that the strain of N. fowleri present in Pakistan may have evolved saline resistance, increasing its survival range.18
Sporadic cases of N. fowleri infection are reported yearly in hot and humid areas.19 Given the amoeba’s thermophilic nature, these regions may be more prone to infections due to their year-round warm climate and contaminated water sources.15,17,19
The most commonly used treatment for N. fowleri is amphotericin B (AmB), an antifungal and antiprotozoal agent that has been shown to kill amoebas by inducing programmed cell death.5,14 However, AmB is limited by its toxicity, causing renal damage, anaemia, fever, vomiting, and chills, among other signs and symptoms.5,14 In other cases, AmB combined with therapeutics like fluconazole, azithromycin, and rifampin has successfully eliminated early-stage infection.5,13,14 However, many of these drugs do not effectively penetrate the BBB, requiring high concentrations that may exacerbate adverse side effects.5
Miltefosin, another compound typically used to treat breast cancer, was used in conjunction with AmB to successfully treat two cases of N. fowleri infection with full neurological recovery.5,13,14 While these results are limited by a small sample size, miltefosine is nonetheless a promising drug.13,14
Drug delivery is vital in treating amoebic CNS infections, as effective drug concentrations reaching
While infection by N. fowleri is rare, scientists are also concerned that climate change could result in more people coming into contact with contaminated water sources.19 As the fatality rate for N. fowleri infection remains constant, there is an imminent public health need to formulate evidence-based policies to prevent infection and provide effective, safe, and accessible treatments.14,17
REVIEWED BY: DR. CHERYL MAIN (MD)
Dr. Cheryl Main is an Associate Professor with and Chair of the Department of Pathology and Molecular Medicine at McMaster University. She is Chair of the Specialty Committee for Infectious Diseases with the Royal College of Physicians and Surgeons of Canada. Her research primarily focuses on infectious disease, laboratory safety, and quality assurance.
EDITED BY: DAVID GOU, FIRDOSE KHAN & MATTHEW OLEJARZ
What advice would you give to anyone who is interested in studying similar fields as future researchers in neuroscience
I think you have to go with what you find interesting and what can stoke your passion. Nobody does this as a job; I never refer to my position as a job. A job is work that you may or may not like to do. I’ve never worked a day in my life—my lab is always filled with
It goes by several names: phthisis, The White Death, the Silent Killer, and The Robber of Youth.1 Since its discovery in 1883, tuberculosis (TB) has been responsible for over one billion deaths worldwide, more than the black plague, smallpox, influenza, and HIV/AIDS combined. Two billion people globally harbor latent Mycobacterium tuberculosis (M. tb) while at least ten million more are actively infected.2 The significance of TB is undeniable as it vividly illustrates the complex interplay between the disease fighting for survival and its human hosts striving for eradication.
The earliest markers of TB date back 15,000 years ago to East Africa.3
Over the next thousands of years, TB spread to multiple ancient civilizations, including Egypt, China, the Americas, and Greece.4 DNA analysis from remnant tissues of Egyptian and Peruvian mummies highlights the prominence of TB in ancient populations.5,6 In Ancient Greece, Hippocrates discovered much of the early information available regarding TB. He coined the term phthisis and was especially knowledgeable about the pathogenesis and symptomatology of the disease.⁷
Theories on the etiology of TB emerged during the Middle Ages. Scrofula, a form of TB causing inflammation of the cervical lymph nodes, attracted widespread attention. It earned the name “King’s Evil” as it was believed only a “royal touch” from a monarch could cure the sickness. This belief persisted until the 19th century in France, when surgical removal of the swollen lymph nodes became standard practice. Surgery necessitated precise incisions to avoid damaging vessels and nerves surrounding the neck.4
Screening of TB was widely implemented during the 20th century using skin tests. These tests were first developed using latent forms of M. tb. They were later replaced by purified protein derivatives, which identified TB infections with high accuracy. TB vaccine development started after World War I, with the first successful use of the vaccine in 1921.9,11 Vaccines and antibiotics proved effective in slowing the transmission and mortality of TB, reaching patients globally. Medications, such as para-aminosalicylic acid and streptomycin, were released as antibiotic treatment options.12
TB incidence peaked during the 19th century, causing high mortality in Western Europe. One-third of the working population in England died from TB between 1838-1839.⁸ Pallor resulting from TB-induced anemia became widely known as “The White Death”.1 19th century researchers made insights into TB’s pathogenesis, with new hygienic methods being established for patients.9 “Sanatarias” – facilities meant to isolate infected patients from local communities – became the first line of treatment for patients during this time. These offered surveillance, access to fresh air and food, and surgical treatment.10
The management of TB is still ongoing, with drug resistant TB strains causing health problems in urban settings. Inequities in healthcare access have also resulted in disproportionate infection rates in China, India, many African countries, and various Indigenous communities.13,14 Within the last decade, the WHO released a programme titled the End TB Strategy, aiming to reduce TB incidence by 80%, and achieve a 90% reduction in deaths by 2030.15 Even after centuries, healthcare systems are continuously working to control and treat the disease.
AUTHOR: MATTHEW OLEJARZ1
1 Bachelor of Health Sciences (Honours), Class of 2026, McMaster University
doi: 10.35493/medu.46.16
AUTHORS: CIARA REID1 & PREM PATEL1
1 Bachelor of Health Sciences (Honours), Class of 2027, McMaster University
ARTISTS:
TRAM NGUYEN2 & CAMELA TEMACINI3
2 Bachelor of Arts (Honours Media Arts), Class of 2026, McMaster University
3 Bachelor of Health Sciences (Honours Biochemistry), Class of 2027, McMaster University
ABSTRACT
This review will compare four drugs commonly used in the treatment of neonatal seizure. We examined midazolam, levetiracetam, lidocaine, and phenobarbital, highlighting their efficacies and adverse effects. We analyzed and criticized the treatment plan proposed in the study conducted by Falsaperla et al., and ranked the drugs according to the efficacy and adverse effects identified in our literature review. Based on these findings, we placed midazolam as a possible low-dose first-line treatment, levetiracetam as a possible secondline, and lidocaine or phenobarbital as possible third-lines depending on if therapeutic hypothermia is used in tandem.
Neonates are highly susceptible to developing seizures due to their immature brain and lower neurotransmitter threshold, with the primary cause being the presence of many excitatory receptors and few inhibitory receptors. Seizures in neonates are common, occurring in around 1.5-3 out of 1,000 live births, with a mortality of up to 20%. treatments for neonatal seizures are alarmingly underdeveloped and under contention. A study by Falsaperla et al. analyzed four major drugs used to treat neonatal seizures. Here, we critique their findings and reexamine midazolam, levetiracetam, lidocaine, and phenobarbital as four viable drugs for treatment.
Midazolam is commonly used as an antiepileptic drug (AED) for neonatal seizures by primarily stimulating inhibitory GABA receptors to reduce neuronal firing, leading to muscle relaxation.3 Often taken intravenously, midazolam acts rapidly, with peak blood concentrations being reached minutes after injection. The drug is only effective for about one to two hours. Although highly effective, there are many concerns about its widespread clinical use.3 Midazolam is primarily cleared in the liver by the metabolic enzymes CYP3A4
and CYP3A5.
between neonates, which presents a challenge to determining a standardized dosing procedure. doses of midazolam may pose a significant risk for neonates who lack appropriate levels of metabolic enzymes. unpredictable responses across neonates, even with standardized dosing. Considering the variance in metabolic capacity between neonates and adverse effects of overdose, midazolam may be preferred as an AED in low concentrations.
Lidocaine works by blocking neuronal sodium channels and inhibiting glutamate release, thereby reducing neuronal firing, resulting in a relaxing effect.
reducing neonatal seizures. Lidocaine is metabolized in the liver by CYP3A4, with metabolic rates being quicker in larger term neonates. Like midazolam, metabolism is a concern due to low and varied expression of CYP3A4. Moreover, lidocaine metabolism is temperature-sensitive. Therapeutic hypothermia (TH), a form of neonatal care which involves dropping core temperatures to 33-34°C, slows lidocaine clearance by 21.8% through decreased organ perfusion and reduced enzymatic metabolism.
From this, we reason that lidocaine is best used as an AED for large term neonates who are not undergoing extensive TH or concurrently taking phenytoin.
Approved by the U.S. Food and Drug Administration in 2012, levetiracetam (LEV), an anticonvulsant drug, has shown clinical promise in the treatment of some epileptic conditions.10,11 LEV is shown to decrease presynaptic neurotransmitter release by binding to synaptic vesicles.2 Despite its neuroprotective properties, LEV has only been used in recent years to treat neonates. By decreasing the expression of nitric oxide synthase and increasing the expression of the cysteine/glutamate exchanger in the hippocampus, this molecule can diminish damage caused by seizure-induced oxidative stress.11 Additionally, LEV has a favourable pharmacokinetic profile, wide safety margins, as well as fast absorption after oral intake, although it can be administered intravenously as well. Moreover, it proves safe in liver failure, is primarily eliminated renally, and has no established severe drug interactions partially due to minimal protein binding.2,10,12 The safety of LEV was confirmed by in vivo studies conducted on animal models, which indicated no neurotoxic side effects with therapeutic doses and were successful in prognosis improvement.11 Furthermore, this molecule presents an anti-apoptotic effect, as evidenced by the reduced cellular apoptotic processes in the hippocampus and cerebral cortex of treated patients.12 In 50% of cases, side effects of lethargy and difficulty feeding were observed but resolved after adjustments of LEV doses.11 The safety that LEV offers makes it viable for use in cases when lidocaine is not advisable, which include smaller term neonates and those undergoing extensive TH.
Phenobarbital, as per the recommendation of the World Health
Organization, is used as the first-line treatment for neonatal Phenobarbital exists in both intravenous and oral forms, has a long half-life, and acts by reducing the sensitivity of GABAα receptors.2 However, it has an efficacy of less than 50% and can lead to undesirable side effects. These include increased neuronal apoptosis, irritability, sedation, hypotension, respiratory depression, and hepatotoxicity.2,12,13 Neuronal apoptosis was evident in patients who received doses above 40 mg/kg. In addition, those with hypoxic-ischemic encephalopathy reported seeing neuroprotective effects when treated with phenobarbital.2 Seizures were regulated in only 43% of the treated neonates; however, when 76 asphyxiated newborns were treated with phenobarbital, efficacy was reported in 65% of cases.2 The efficacy of drugs that act on GABA receptors, including phenobarbital, depend on certain inhibitory mechanisms which may be underdeveloped in the immature brain.2 Mice models showed a low expression of GABA receptors and enzymes involved in GABA synthesis at birth. Increased concentrations were seen during the first weeks of life, indicating that growth was directly proportional to gestational age.2 Thus, the poor efficacy of phenobarbital may be due to the unique profile of the immature brain.
Neonatal seizures are of great concern, and the consensus on an optimal treatment plan is still under criticism. The literature presented in this article outlines the different pharmacological drugs and secondary research on drug efficacy. This includes a review comparing pharmacological treatments to create a hierarchy of options for neonatal seizures.13 According to the review, phenobarbital is best used as a first-line option, followed by levetiracetam, phenytoin, and lidocaine as viable second-line options. Midazolam was considered as only a third-line option. However, based on the evidence collected, we have analyzed the advantages and adverse effects associated with this hierarchy, considering factors including efficacy, duration, metabolism, cellular mechanism, and risks. Midazolam could possibly be used as a lowdose first-line treatment because of its high effectiveness as an AED. Midazolam works quickly and has a short effective window, making it especially useful for acute seizures. While Falsaperla et al. criticized midazolam for potentially triggering respiratory depression and hypotension, it may be worth revisiting when respiratory support is available for the neonate.11,13 Midazolam could be used in lower doses to limit the risks of poor metabolism in some neonates while serving as a rapid and highly effective anti-epileptic. Further studies examining the variation in neonatal expression of CYP3A4 and CYP3A5 could improve dosing precision.
LEV shows promise in alleviating seizures and minimizing harmful long-term repercussions that often caused from other pharmacological treatments. LEV was found to reduce neurological
damage caused by oxidative stress, a common consequence of seizures.10 Furthermore, neuroprotective properties are seen in the hippocampus and cerebral cortex of patients taking LEV.11 Due to its ability to both alleviate present epileptic symptoms and protect the neonate long-term, LEV is best used earlier in treatment and may be effective as a second-line AED for neonates. In cases where minor side effects were observed, adjustments in LEV dosing provided a sufficient outcome.10 As evidence suggests that LEV can be an asset in treatment of neonates suffering from seizures, further research to solidify the clinical application of LEV is encouraged.
For potential third-line options, either lidocaine or phenobarbital may be utilized. When TH is absent or used lightly, lidocaine is advisable due to its temperature-sensitive metabolism. Lidocaine has higher efficacy compared to phenobarbital at 71% and 66%, respectively, and poses fewer serious adverse effects. However, with lidocaine and midazolam both being cleared in the liver by CYP3A4 and CYP3A5, further studies should investigate concerns regarding variability in the expression of these enzymes. Lidocaine has also been criticized for its potential to cause cardiac arrhythmia, hypotension, and seizures at high doses. Lidocaine has been used for decades as a safe anesthetic for post-neonatal patients; however, further research examining its safety in neonates can improve potential treatments.
Despite phenobarbital’s common usage as a first-line treatment for neonatal seizures, its low efficacy and potential for undesirable side effects suggests a possible use as a third-line treatment in cases where lidocaine cannot be used due to the concurrent use of TH. The efficacy of phenobarbital, which never exceeds 66%, may be attributed to its dependence on inhibitory mechanisms underdeveloped in the immature brain.1 The lower expressions of GABA receptors and enzymes involved in GABA synthesis maintains concentration of phenobarbital within the first weeks of life, indicating that the efficacy of phenobarbital is directly proportional to gestational age.13 The observed side effects of phenobarbital ranged from irritability to neuronal apoptosis, which may lead to long-term neurological damage.2,13 Thus, a study recommends that phenobarbital, as a first-line treatment, should be administered at 20 mg/kg with an additional 20 mg/kg if seizures continue.13 Approaching a threshold of 40 mg/kg presents neuronal apoptosis and may not be the initial course of action when other effective avenues exist. Despite these precautions, it would be ideal to delay the implementation of phenobarbital until later in the neonate’s life to optimize the availability of the necessary inhibitory mechanisms.
Neonatal seizures are common, with high mortality rates.1 In this review, we examined four possible pharmacological treatments that could be used to treat neonatal seizures: midazolam, levetiracetam, lidocaine, and phenobarbital as first-line, second-line, and third-line options. Further research on the efficacy and risks of these drugs could improve our understanding and treatment plans for neonatal seizures.
REVIEWED BY: DR. IPSITA GOSWAMI (MD, MSc) & DR. SAMIRA SAMIEE-ZAFARGHANDY (MD)
Dr. Ipsita Goswami is an Assistant Professor of Pediatrics in the Faculty of Health Sciences at McMaster University. She is an Associate Program Director for the NPM Fellowship Program in the Department of Pediatrics at McMaster Children’s Hospital. Her research investigates imaging techniques in neonatology and neurology.
Dr. Samira Samiee-Zafarghandy is an Associate Professor of Pediatrics in the Faculty of Health Sciences at McMaster University. She is also a neonatologist at McMaster Children’s Hospital. Her research investigates drugs and clinical trials in neonates.
EDITED BY: PARTH ARORA & EVAN SUN
doi: 10.35493/medu.46.20
AUTHORS:
ANGELA HONG1, JIA LIU2 & AARANI SELVAGANESH3
1 Bachelor of Health Sciences (Honours), Class of 2026, McMaster University
2 Bachelor of Health Sciences (Honours), Class of 2027, McMaster University
3 Bachelor of Arts & Science (Honours), Class of 2027, McMaster University
ARTIST:
AYMEN SAEED4
4 Bachelor of Science (Honours), Class of 2026, McMaster University continues to emit more pollutants than the provincial standards.9
Hamilton, often referred to as Steeltown, is recognized as one of Canada’s leading industrial cities.1 By the end of the Second World War, Hamilton produced nearly half of Canada’s steel.2 Steel is made from iron ore, which is mined and processed using oxygen or electric arc furnaces.3 However, these processes release pollutants, such as dioxins and furans, which can induce liver problems and certain cancers.4 The health issues associated with the byproducts of steel milling underscore the importance of sustainable practices that decrease the amount of emissions released through the manufacturing process in Hamilton.
The two predominant steel milling companies in Hamilton are ArcelorMittal Dofasco and Stelco.2 With Hamilton being a major steel producer, there are high concentrations of pollutants found in the air.5 According to Hamilton’s most recent Clean Air Progress Report in 2021, the amount of sulfur dioxide (SO₂), a byproduct of extracting steel from iron ore, increased from 2016 to 2021 by approximately 2 parts per billion (ppb).6 To keep air pollution under control, the Ontario Ministry of the Environment, Conservation, and Parks, created the Ambient Air Quality Criteria (AAQC) used to assess air quality in communities and set standards for the safest concentrations of pollutants to protect people’s health and the environment. For example, the standard for SO₂ is 4 ppb annually, with industry sites emitting approximately 8 ppb as of 2021.7 According to the most recent Hamilton Clean Air Progress Report in 2021, the annual concentration of Inhalable Particulate (PM10) for 24 hours has consistently exceeded the provincial AAQC of 50 μg/m3 for the past 5 years.8 PM10 is produced from reactions involving SO2 and nitric oxide (NOx), which are emitted from industrial sites, such as steel milling sites.8 However, several steel companies have received exemptions from the provincial government, permitting them to exceed the air quality standard by raising the amount of pollutants they are able to release.9 For example, ArcelorMittal Dofasco was granted an exemption that permits the release of an annual average of benzene that is roughly 22 times greater than the general air standard of 0.45 μg/m³.10 This exemption has been in place since May 18, 2016 and has expired as of June 30, 2023.10 Despite the exemption expiring, the company
Concerns regarding the public health impacts of Hamilton’s air quality continue to persist despite efforts to improve air pollution in the region in the last 20 years.11 The 2011 Air Quality Health Assessment Study estimates that air pollutants, including nitrogen dioxide (NO₂), ground-level ozone (O₃), PM₁₀, fine respirable particulate matter (PM₂.₅), SO₂, and carbon monoxide (CO) are associated with 186 premature deaths, 395 respiratory hospital admissions, and 322 cardiovascular hospital admissions annually in Hamilton.12
Notably, NO2 released during fuel combustion in industry and transportation contributes to 36% of these hospital admissions and health impacts, while ground-level O3 from sources, such as coal-fired factories and vehicles, contributes 23%.12 Particulate matter also contributes to 32% of these hospitalizations and is mainly composed of emissions from transportation activity and industrial fugitive dust.12 Furthermore, when scaled to the size of the local Hamilton population, the Ontario Medical Association estimated the costs associated with treating air pollution-related illness to be $1 billion.12
Adverse health effects associated with particulate pollution from the Hamilton industrial sector is not a new issue. In 1984, Pengelly et al. conducted a cohort study assessing the respiratory health of 3,553 children aged seven to ten years living in different quadrants of the Hamilton region corresponding with varying levels of air pollution, represented by total suspended particulate (TSP) measurements.13 Children living in the industrial core experienced the highest prevalence of respiratory hospital admission in infancy, reinforced with confounders such as the highest prevalence of parental cough, chest illness in siblings, low income, smoking in the home, and crowding in the house.13 The industrial core area is also associated with the highest ambient pollution levels, with an average annual TSP measurement of 93 μg/m3, much greater than the current 60 μg/m³ annual TSP standard set by the Ontario AAQC.13,14 The upper city areas generally had a lower prevalence of these covariables and respiratory hospitalizations, corresponding
with lower levels of TSP air pollution.13 Particulate matter, including PM₂ ₅ and PM₁₀, may be inhaled and deposited throughout the airways, inducing pulmonary inflammation and oxidative stress through reactive oxygen species (ROS) generation.15,16 ROS damage cells and tissues due to the susceptibility of structural proteins, enzymes, and DNA to oxidation, which is especially problematic in airway epithelium as it is the first line of defense against inhaled oxidants.
mechanisms and ROS generation are increased in the lung, along with the activation of antioxidant genes for cellular protection. However, when exposed to heavy metals from air pollution, the oxidative stress mechanisms generate free radicals, reduce antioxidant levels, impair membrane proteins, and alter DNA structure, contributing to inflammation and respiratory disease.
A re-analysis of the Hamilton Children’s Cohort using a spatial analytic approach demonstrated stronger associations with children’s respiratory health and air pollution, strengthening the original findings. correlation between chest wheezing and TSP air pollution or number of smokers in the home; however, the association was weak (OR: 1.01, CI: 1.00-1.01).
(OR: 1.56, CI: 1.35-1.80) was found between particulate air pollution and coughing in children under the age of two with exposure to maternal smoking. was positively associated with the prevalence of respiratory symptoms, researchers found that the pulmonary function of children exposed to higher levels of TSP pollution was better. Thus, the relationship between air pollution and respiratory health may be masked by the measurement of total particulates without accounting for particle size.
Following the Hamilton Children’s Cohort into adulthood through another prospective cohort study, Barakat-Haddad et al. investigated whether childhood exposure to air pollutants, such as particulate matter and SO health outcomes. in adulthood is associated with exposure to SO median level of 11.7 ppb from 1983-1984. posit that gaseous pollution triggers pulmonary oxidative injury and systemic inflammatory events, leading to oxidative stress associated with onset or progression of inflammatory disorders.
In 2019, the City of Hamilton declared a climate change emergency, which prompted the development of the Community Energy and Emissions Plan, ReCharge Hamilton, through collaboration with multidisciplinary groups comprised of stakeholder committees, experts, and the general public. plan aims to meet Hamilton’s future energy needs by improving energy efficiency, reducing greenhouse gas emissions, and fostering sustainable, community-supported energy solutions. ReCharge Hamilton seeks to achieve net-zero greenhouse gas emissions by 2050, while simultaneously enhancing the energy system’s resilience and promoting economic prosperity for all.
In 2016, Hamilton’s industrial sector was the largest source of emissions, accounting for 64% of total emissions. plan targets a 50% reduction in greenhouse gas emissions from steel mills by 2035, with a goal of achieving net-zero emissions by 2050.
The short-term objectives, set to be accomplished within five years, include:
1. Establishment of the Industrial Energy Efficiency and Decarbonization Working Group. This group is
In early 2022, a $1.8 billion project led by ArcelorMittal Dofasco aimed to transition its Hamilton facility from coal-based steel production to electric arc furnace processes, marking a significant
to support this transformation, accelerating the province’s transition to carbon-neutral manufacturing through the anticipated completion of this project in 2028.27,28 Despite these ambitious targets, the necessary upgrades at Dofasco’s Hamilton facility have
29 The company missed key milestones in 2023 and 2024, including demolishing a coal plant to make space for a direct reduced iron plant and obtaining approval for the construction of This delay raises concerns about the project’s progress as promised by company executives.30
In light of these challenges, we call upon the City of Hamilton to enforce stringent regulations to hold steelmakers like Dofasco accountable. Specifically, we recommend enhancing public transparency and enforcing more rigorous air quality By mandating real-time emissions reporting, communities can better understand the immediate effects of industrial activities and force steelmaking industries to prioritize pollution reduction efforts to maintain a positive public image in their commitment to sustainability. Furthermore, data transparency allows for the identification of pollution trends that may require targeted policy actions, creating a dynamic regulatory framework capable of constantly evolving and adapting to environmental changes. Implementation of stricter air quality standards such as eliminating the possibility for site-specific emission exemptions, can lead to improved quality of life for vulnerable populations and a cleaner environment to promote biodiversity by mitigating ecosystem degradation. By enhancing public transparency and implementing firm air quality standards, Hamilton can promote healthier communities and environments, driving industrial innovation towards a more sustainable future.
Dr. Michael Wilson is the Scientific Director of the McMaster Health Forum and an Assistant Professor in the Department of Health Evidence and Impact at McMaster University. His research focuses on using evidence, citizen values, and stakeholder insights to strengthen health and social systems. He leads a rapid response program delivering timely evidence syntheses to address urgent issues.
AUTHORS:
JOEL ABRAHAM2, ZAHRAH TALAWALA3 & EVAN ZHAO2
2 Bachelor of Health Sciences (Honours), Class of 2028, McMaster University
3 Bachelor of Health Sciences (Honours), Class of 2025, McMaster University
Humanity stands on the brink of a new era in exploration, poised to extend its reach beyond Earth's orbit to destinations like Mars and beyond. While the allure of space captures the imagination, the harsh realities of long-duration space travel—microgravity, cosmic radiation, and psychological isolation—pose significant challenges to astronaut health.1 This review aims to research relevant astronaut health challenges and current interventions available to address them.
Astronaut health risk factors in space can be categorized into exogenous and endogenous processes.2 Exogenous processes are external factors that arise outside of the body of the astronaut. Examples include exposure to microgravity (the condition in which very little gravity is felt in space) and cosmic radiation.2,3 Endogenous risk factors relate to disruptions of the internal processes of the body, including mental health concerns and disruption of circadian rhythms.2,4
Exposure to microgravity leads to musculoskeletal deconditioning, the loss of bone density and muscle volume due to reduced gravitational load.5 In microgravity, human bone tissue begins to reshape.6 Over time, an astronaut’s weight-bearing bones could lose approximately 1% of their density due to decreased mechanical loading, a process known as bone atrophy.8 Due to the lack of ultraviolet light on the International Space Station (ISS), vitamin D deficiencies also compromise bone health.9 A study conducted by Gabel et al. imaged the tibia and radius of 17 astronauts before and after spaceflight.10 The researchers found that 9 astronauts did not fully recover tibial bone density even after returning to Earth for 12 months.10 This irreversible damage can lead to increased fracture risk and early-onset osteoporosis.10 Additionally, an astronaut’s muscles gradually weaken due to the reduced effort required for movement in microgravity, compromising performance and causing discomfort. On short duration missions, the European Space Agency has observed the loss of 10-20% of postural muscles such as hamstrings, quadriceps, and glutes.11 Postural muscle atrophy is being investigated as a cause for lower back pain, a common astronaut complaint. 11
Microgravity induces changes in the operation of the cardiovascular (CV) system, which has evolved based on a 1 g environment (gravitational field on Earth).12 This leads to cardiac deconditioning, characterised by a reduction in CV efficiency through a number of clinical markers.12 One such complication, post-spaceflight orthostatic intolerance (PSOI), stresses the circulatory system, leading to an inability to maintain blood pressure and cerebral perfusion.12 Upon returning to Earth, about 67% of astronauts are unable to stay in an upright position for more than ten minutes.13 Recent studies exploring cardiac remodelling (changes to the shape, size, composition, and function of the heart) revealed that the left ventricular mass of astronauts decreased by 9.1% compared to preflight sizes.14 Additionally, carotid arterial stiffness after 6 months of spaceflight was also observed, equivalent to changes observed in patients after 10-20 years of aging.15 One accepted mechanism for this change includes a cephalad fluid distribution induced by microgravity environments. There is a significant alteration in the 1g gravity-based distribution of fluid within the head and body compartments in addition to loss of hydrostatic pressure gradients which drive fluid movement in the body. These lead to increased arterial pressures and resistance, which leave notable reductions in systolic and diastolic arterial strain even after recovery on Earth. There is a notable gap in the literature on the potential risks of irreversible structural changes in long deep space missions, noting the need for long-term vascular remodelling studies.
The brain is especially vulnerable in space, as intracranial fluid fails to restrict movement of the brain, resulting in increased malleability. This leads to decreases in grey matter volume, white matter structural integrity, and hippocampus size.17 While the consequences of these changes are not thoroughly researched, some cognitive impairments in astronauts have been observed. Astronauts have been shown to have impaired motor and cognitive skills after spaceflight.17 Neuro-ocular conditions include spaceflight-associated neuro-ocular syndrome (SANS), a complex condition influenced by microgravity. The absence of gravitational forces leads to increased intracranial pressure, potentially causing various forms of visual impairment, retinal folds, and compromised visual capabilities.18 Intracranial swelling may also lead to headaches, intracranial hypertension, and pulsatile tinnitus (a rhythmic ringing in the ears).18
The space radiation environment involves charged particles with a wide range of energies which can be destructive to biological tissue. The radiation exposure in one ISS mission is equivalent to 53 computed tomography (CT) scans.XX Galactic cosmic rays are the main source of the biological effects of space radiation. They are a source of highly ionising radiation, which can leave large amounts of energy in human cells and tissues, causing cellular damage.20 Solar particle events involve the release of charged particles during short solar flares and long coronal ejections from the sun. Lastly, the Earth’s trapped radiation belt houses high energy protons and electrons, which have minimal damaging effects due to Earth’s magnetic field. As astronauts engage in
deeper missions, solar particle events and galactic cosmic rays can lead to more significant and irreversible tissue damage.
Radiation contributes to DNA mutations, promoting the uncontrolled growth and proliferation of tumours, making it a complete carcinogen.21 DNA damage from radiation consists of strand breaks, gene mutations, and chromosome aberrations.22 Cells in the gastrointestinal tract are especially susceptible to radiation-induced tumours due to their high cell turnover rate.23 Kumar et al. observed that energetic heavy ions found in deep space increased colon cancer risks by decreasing epithelial cell migration, which maintains nutrient absorption and barrier function of the intestinal lumen.23 Further, space radiation can increase the oxidative stress response, a progenitor of carcinogenesis, and trigger DNA damage and mitochondrial dysfunction.23 Silveira et al. observed that 8-oxo-guanosine, a biomarker of oxidative stress and cancer risk, was elevated in astronauts.24 The National Aeronautic Space Administration (NASA) landmark twin study between astronaut Scott Kelly and Earth-residing Mark Kelly was a longitudinal exploration of the physiological, molecular and cognitive changes due to space flight.25 The study highlighted rapid telomere shortening and DNA damage from long space missions and increased frequency of chromosomal structural variants, all conducive of altered gene expression and cancer development.22 Currently, radioprotectant dietary supplements are a frontier in research efforts. Aksenov et al.’s supplement was able to reduce rates of tumorigenesis by 30%, carcinomas by 67%, and metastasis by 100% in murine models.36, 42
The particles released from cosmic radiation are high in energy relative to the ionising radiation used in medical imaging and cancer treatment, and are thus understudied in the context of neurodegenerative changes.26 Mouse models irradiated with high energy particles had altered neurogenesis due to reductions in neural precursor cell populations in the hippocampus, leading to behavioural changes, loss of memory, and accelerated neural aging.27 Additionally, radiation triggered microglial activation which induced cognitive impairment, similar to the mechanism observed in Alzheimer’s disease. This suggests that radiation alters synaptic protein expression and pruning, inhibiting the plasticity needed for brain development and efficiency.28 High energy particles also contribute to neuroinflammation due to direct damage and brain injury. Neuroinflammation, the mechanism by which injured nervous tissue is repaired, activates astrocytes and microglial cells and upregulates mitochondrial dysfunction and oxidative stress.28 Activated oxidative species needed for immune waste clearance during tissue healing are also highly reactive and can destroy functional brain tissue.
Astronaut mental health is an additional factor to consider. Prolonged distance from loved ones and mental strain from space travel can cause stressful working relationships and decrease motivation. These concerns are further exacerbated by circadian rhythm disturbance. Significant workloads during missions may force astronauts to be awake at times when they would normally be asleep.29 Misaligned circadian rhythm is linked to lower sleep duration and quality, which can result in decreased performance and exacerbate existing challenges to astronaut mental well-being.29
Interventions to safeguard astronaut health are present across pre-flight training, in-flight missions, and rehabilitation upon return to Earth. While astronaut crew selection and training processes may vary across space agencies, NASA provides the most accessible documentation and is thus the focus of pre-flight screening processes for this review.
Protecting astronauts from microgravity-induced challenges requires improving physical fitness beyond the threshold where performance in space would be impaired. Pre-training screening assesses how well a candidate can adapt to strength training and identifies potential issues that could hinder performance in space. Once selected for training, strength development is focused on postural muscles through leg presses and knee extensions.30 Astronauts must be able to deadlift their bodyweight and bench press 0.7 times their body weight to ensure they have the strength to accomplish extravehicular activities, such as space walks.30 Training for astronauts continues to improve with the research of new interventions.
To promote bone health, astronauts are given vitamin D supplementation prior to space missions and are imaged three times a year for bone mineral density.30, 34 In space, exercise plays a pivotal role in mitigating muscle and bone atrophy. Astronauts follow strict exercise regimens for approximately two hours per day, which includes using running on a treadmills and stationary biking.30 Nutrition is essential to maintaining caloric density, micronutrients, and macronutrients to support bone and muscle health.30 Countermeasures upon return to Earth include antiresorptive agents that suppress bone deterioration; however, adverse long-term effects of these drugs include femoral fractures and osteonecrosis (bone tissue death) in the jaw.31, 32 Recent research includes the Rodent Research-19 study, which tested myostatin inhibitors—drugs used to prevent muscle loss—in mice during spaceflight.7
Aerobic strength is also required for combating CV health related issues related to microgravity as well performing astronaut duties. NASA uses maximal oxygen uptake (VO2 max) to measure aerobic health. An average VO2 max is 35-40 mL/(min•kg) for men and 27-31 mL/(min•kg) for women without training.33 All astronauts must have a maintained VO2 max of at least 32.9 mL/ (min•kg), with a recommended range of 38.7-43 mL/(min•kg).30
The most immediate concern upon an astronaut’s return is PSOI caused by adaptations to microgravity. The 1998 Neurolab Mission investigated the mechanisms behind PSOI and collected data on fluid loss, muscular atrophy, changes in function and structure of sensory otolith (ear) organs, and impaired baroreceptor and betaadrenergic receptors.35 PCOI countermeasures include wearing antigravity suits with liquid cooling networks upon reentry to maintain blood pressure and heart rate and rehabilitation with fluid resuscitation once landed.35 The majority of preventions for fluid shifts in the body cover brain and ocular health. The intensity of astronaut training is intentionally neuroprotective, contributing to positive brain plasticity.17 Increased exposure to microgravity in controlled settings, such as neutral buoyancy labs, and social and intellectual engagement, meditation, and
exercise help maintain resistance.17 Post-mission, astronauts likely also experience joy, comfort, and relief from reuniting with family and friends.17 These factors may influence recovery of brain changes due to spaceflight. Physical activity helps astronauts prevent SANS by counteracting fluid shift toward the head and reducing intracranial pressure.18 Dietary adjustments, hydration management, and nutritional supplements support astronauts' ocular health and intracranial pressure.18 In the case of SANS causing changes to visual acuity, pharmacological interventions including diuretics to regulate fluid balance and antiinflammatory drugs may be used.18 Astronauts may be assigned less demanding tasks to prevent symptoms from worsening.18
There are two main approaches to radiation protection:
field surrounding a spacecraft to deflect high energy particles.36 Research investigating the counteraction of cosmic radiationinduced neurodegeneration is key in mitigating the effects of radiation. Amitriptyline was used to stop neurogenesis inhibition by reducing the expression of apoptosis-promoting amides and cellular stress responses.43 Targeting reactive oxygen species is also intriguing. Selenium supplements have antioxidant properties and are shown to reduce oxidative stress responses, though it has been only investigated thoroughly in earth studies and needs further research in astronauts to gain full treatment approval.43
Before becoming astronauts, candidates are screened for a number of psychological traits. This involves self-report personality questionnaires, formal psychiatric interviews, situational judgement tests, and counterproductive work
In training, astronauts practice in simulated environments to overcome mental challenges. Arctic and Antarctic expeditions, mountain climbing, and underwater habitats are designed to expose astronauts to isolation, a lack
However, even with training and screening, many astronauts still suffer from mental health issues. 60% of astronauts report having asthenia, a condition similar
Therefore, the requirement of post-mission behavioural counselling allows astronauts to be emotionally supported and ensures their
53 More research seeking to improve astronaut mental health is underway.
As agencies prepare for longer exploratory missions to Mars and beyond, astronauts will face prolonged exposure to microgravity and radiation.38 Research on astronaut mental health, cognitive impairment, and microgravity-induced bone and muscle atrophy during spaceflight is essential to ensure future missions are safe. Practical solutions for exogenous and endogenous risk factors in space must be developed to ensure astronaut health is protected.
REVIEWED
BY:
DR. JOAN SAARY (MD, PhD)
Dr. Joan Saary is a physician in occupational medicine with a specialization in aerospace medicine. She is an Associate Professor in the University of Toronto's Department of Medicine and a consultant for the Royal Canadian Air Force, the Canadian Forces Environmental Medicine Establishment, and the Canadian Space Agency.
EDITED BY: ANGELA HONG, JIA LU & AARANI SELVAGANESH
JESSICA PALFY Bachelor of Health Sciences (Honours), Class of 2028, McMaster University
Your blood test shows nothing concerning...
What if something is really, —really, wrong this time?
...but these should help with your symptoms
“What if something is really wrong this time?” — A common feeling regarding irregularities in menstrual and hormonal health, and sadly, one that is too often left unheard. Hormonal birth control is a revolutionary intervention. For some individuals, it is an excellent way to prevent pregnancy or manage menstrual pain. However, when people seek medical attention for irregular symptoms, birth control is often prescribed as a way to regulate periods, disregarding underlying causes and delaying diagnosis. This piece aims to bring light to the frustrations menstruating people feel when seeking treatment. Instead of addressing physiological causes, their issues are covered up by birth control. While still an important and effective medication, birth control should not be the default; healthcare should believe patients’ voices.
OPTIMIZING EARLY DETECTION: A REVIEW OF PRENTAL DIAGNOSIS IN CONGENITAL
doi: 10.35493/medu.46.30
AUTHORS: HIRDAY JOSAN1 & MIRAN MASTER2
1 Bachelor of Science (Honours), Class of 2027, McMaster University
2 Bachelor of Health Sciences (Honours Biochemistry), Class of 2027, McMaster University
ARTISTS: YUEWEN GAO3 & SOPHIE LI4
3 Bachelor of Health Sciences (Honours), Class of 2028, McMaster University
4 Bachelor of Science (Honours), Class of 2026, McMaster University
Congenital heart defects (CHDs) are structural cardiovascular malformations that disrupt blood flow and oxygen levels, significantly contributing to neonatal and postnatal mortality worldwide.1 Current prenatal screening methods reliant on routine ultrasound screening exhibit variability in referral rates to echocardiographic examinations based on examiner expertise. Noninvasive echocardiography itself faces notable limitations, including examiner variability, which can impact the accuracy and interpretation of scans.2 Thus, the ineffectiveness of current screening approaches highlights the importance of diagnostic methods that can provide greater accuracy and consistency. This review critically examines the future of CHD diagnostics and the shift from examiner-dependent techniques towards more reproducible, standardized, and quantitative methods, such as artifical intelligence-enhanced imaging acquisition and analysis, along with omics technologies.3-5 Additionally, this review explores the challenges of CHD diagnoses in rural and remote areas, where limited access to specialized care and equipment exacerbates diagnostic inaccuracies.
Congenital heart defects (CHDs) are the most prevalent birth defects in the world, affecting nearly 1% of all live births and representing the leading cause of birth defect-related neonatal mortality.9,10 CHD diagnoses are typically classified into one of two categories based on pathophysiology and the affected heart structure: cyanotic (CCHD) or acyanotic (ACHD). CCHD results in low oxygen levels in the blood, whereas ACHD causes oxygenrich blood to be pumped abnormally throughout the body. The symptoms of CHDs vary based on the type of defect, with some exhibiting minimal or no symptoms. The most prevalent symptoms for CCHDs include cyanosis—bluish discoloration of the skin, lips or nails. Meanwhile, heart murmurs are typically the most frequent symptom associated with ACHDs.1 Although the etiology of CHDs are unknown, CHD can be predicted using the multifactorial inheritance hypothesis, which posits that both genetic and environmental factors contribute to CHD development.9 Specifically, a predisposed fetus with an environmental trigger such as maternal smoking, drinking, or illness during embryonic cardiac development can lead to CHDs.1
An early prenatal diagnosis of CHD is crucial for risk assessment and informed decision-making during pregnancy.11 Current research has predominantly focused on advancements in ultrasound instrumentation and extended fetal echocardiographic examination to improve diagnostic capabilities. Studies have shown that the timely identification of CHD allows for optimal prenatal management, including delivery planning
at specialized centers equipped to handle complex cases.2,11 Early preparation for potential interventions can significantly improve survival rates and reduce morbidity associated with critical CHD.12 Additionally, prenatal diagnoses provide families with crucial information about the health of the fetus, allowing them to make informed decisions about care options and potential interventions during the course of pregnancy.13
Despite advancements in prenatal screening, many potentially life-threatening CHDs remain undetected during midgestation anomaly surveys, such as subtle anatomical distortions, atrial septal defects, and ventricular septal defects.14 While it is neither feasible nor beneficial to diagnose and address all CHDs prenatally, emerging diagnostic techniques highlighted in this review offer valuable insights that allow for close fetal monitoring. These methods help healthcare providers identify conditions needing attention as pregnancy progresses, improving patient outcomes through early detection and intervention.2,14
The initial step in diagnosing any abnormalities during the prenatal period occurs in the second trimester during obstetric ultrasound examinations.15 This ultrasound evaluates fetal cardiac health using a four-chamber view. A 2013 update to these routine screening guidelines included the examination of critical ventricular outflow tracts, allowing the identification of additional forms of CHD as illustrated in Figure 1.15 Pregnancies at high risk for cardiac disease, either due to maternal or fetal risk factors, are referred for detailed fetal echocardiography for further evaluation. The interpretative nature of the maternal ultrasound is a pressing issue, as its diagnostic accuracy heavily depends on the examiner's expertise and available equipment. These variables on the obstetrical side lead to high variability in detection and referral rates, as over one-third of CHD cases occur in pregnancies not classified as high-risk. Thus, it is essential to reassess referral guidelines for fetal echocardiography to ensure anomalies are detected during routine exams.2
FIGURE 1: Comparison between diastole four-chamber view and outflow tract of the left ventricle. The image, sourced from Chaubal et al. in their paper “Fetal Echocardiography”, illustrates key heart structures: VI: left ventricle, VD: right ventricle, VM: mitral valve, VT: tricuspid valve, AI: left atrium, AD: right atrium, AO: descending aorta; columna: spine, TSVI: left ventricular outflow tract. The fourchamber view assesses the heart structure: the symmetry of the heart chambers, the size, and the position within the chest. The ventricular outflow tract evaluates the outflow tracts of the ventricles focusing on the path the blood exits the heart. Common anomalies that are detected include the tetralogy of Fallot and truncus arteriosus.2,16
Fetal echocardiography is the most widely employed non-invasive
late first trimester, with optimal timing between 18-22 weeks of gestation. An echocardiography provides a comprehensive view of dynamic fetal cardiac physiology, allowing care providers to identify CHDs before birth and subsequent interventions. It operates using a transducer, a small camera placed on the mother’s abdomen, to generate ultrasonic sound waves to image the fetus. These waves rebound off organs like the heart, allowing physicians to visualize fetal development and assess blood flow.15
Though fetal echocardiography is considered the gold standard for diagnosing CHDs, it is not without limitations. Firstly, echocardiography-mediated screening is resourcelimited and only offered to high-risk mothers or when a CHD anomaly is identified in the fetus.17 The inconsistency present in human-based examination suggests the need for quantifiable and objective diagnostic practices.
A review of current CHD studies revealed that prenatal detection rates for certain CHDs are as low as 10%, illustrating the need for enhanced training for practitioners and more advanced diagnostic technology.2 While integrating the four-chamber view and outflow tracts into routine obstetric screening has improved the identification of CHDs, overall sensitivity remains low, especially for complex cardiac anomalies that are not easily detectable by traditional imaging.17 Unfortunately, there have been minimal studies examining the referral rates to a pediatric center for more specialized care of CHDs.
Furthermore, the diagnosis of major CHDs varies immensely between geographical regions, with rural and underserved regions experiencing lower rates of CHD detection.8 For instance, a 2011 study conducted in Norway reported a prenatal detection rate of 24% for CHDs, with most cases diagnosed postnatally instead.8 Similarly, a recent retrospective study in Alberta identified fetuses and infants diagnosed with major CHD from 2008 to 2018 and found that living in rural areas is linked to missed prenatal diagnoses of life-threatening CHDs.18 The observed discrepancies in detection rates are often attributed to variability in physician experience. The delays in timely diagnosis and management are attributed to the long distances expectant mothers must travel to access specialized care centers.19 Nevertheless, socioeconomic status and healthcare access further exacerbate these barriers, leading to disparities in prenatal care outcomes.18,19
Current techniques for initial examinations have limitations in obtaining accurate data and images. Obstetricians must be experienced in precise maneuvers for imaging and analysis of initial ultrasounds, as the variable fetal position makes fetal echocardiography challenging and can lead to inaccurate imaging.2,20 Nevertheless, artificial intelligence (AI) offers several potential improvements over traditional diagnostic techniques, bridging the skill gap in prenatal cardiology.20 With sonography sensitivity varying between 15% and 39%, AI systems can enable less experienced operators to obtain diagnostic accuracy comparable to experts.21 One AI system builds two-dimensional reconstructions from the datasets generated by automated four-dimensional (4D) ultrasound scanning. The 4D ultrasound with spatiotemporal
image correlation (STIC) allows for the acquisition of a volumetric dataset of the fetal heart where different cardiac planes can be extracted in any orientation.21 Manual navigation through volume datasets is time consuming and operator skill dependent. However, fetal intelligent navigation echocardiography (FINE) can be used as a reliable method to automate the navigation of the STIC volume data sets to produce nine standard fetal echocardiographies. FINE has resulted in a 98% sensitivity and a 93% specificity for the detection of CHD.21
The potential of AI in the field of prenatal cardiology can also work to bridge the skill gap in underserved rural communities, where access to specialized care and skilled sonographers may be limited. Recent studies indicate that AI-powered tools can analyze medical images and clinical records with a level of accuracy comparable to that of experienced clinicians.22 For instance, AI systems can assist in accurate assessments by automating image quality control and standardizing measurements.4 Moreover, AI-based telemedicine could significantly improve the quality of prenatal care in rural areas. Telemedicine presents the possibility to eliminate the need for extensive travel as a barrier for patients. Healthcare providers in remote areas could consult experts on complex cases using personalized AI scans.6 Research also indicates that telemedicine screening programs, where fetal ultrasounds are analyzed by expert personnel remotely, can be a cost-effective strategy for improving prenatal diagnosis rates.7 However, the implementation of AI in CHD diagnosis still has its limitations and challenges. The lack of transparency in the AI decision-making process raises concerns over interpretation as understanding decision-making behind a diagnosis is critical for making informed decisions that ensure patient safety.22 Additionally, ethical and legal issues related to privacy and discrimination must also be addressed with clear guidelines before clinical implementation.22
Lastly, recent advances in omics technologies, including genomic, transcriptomic, and proteomic analyses, also show promise in diagnosing prenatal CHD. Techniques such as RNA sequencing and microarray analysis can detect fetal biomarkers in maternal circulation, offering a non-invasive and potentially more accurate approach to diagnosing CHD at an earlier stage than a routine ultrasound.2,23 Emerging research suggests that omics technologies could eventually supplement or even replace traditional imaging as routine prenatal diagnostic tests. For instance, transcriptomics involving global mRNA expression provides a snapshot of fetal development at any stage of the pregnancy, which could greatly increase specificity in CHD diagnosis.23 Fetal mRNA biomarkers—PRKACB, SAV1,
STK4, and TNXB2—exhibit aberrant expressions that are associated with cardiac morphogenesis, extracellular matrix and valvular tissue biosynthesis, contractile activity, and congestive heart failure.23 However, omics-based techniques are not yet ready for widespread clinical adoption in the foreseeable future due to ongoing technological and financial constraints.23 Several barriers persist, including RNA instability due to its susceptibility to degradation, posing challenges as the quality of mRNA is critical for analysis. Additionally, the quantity of fetal mRNAs released into the maternal plasma varies across genes and individuals.23 Overcoming current technical and logistical challenges for omics technologies will be crucial for its implementation in the future.
Incorporating AI systems, omics technologies, and advancements in telemedicine into existing diagnostic procedures can significantly enhance prenatal CHD diagnoses. Current diagnostic practices, primarily reliant on routine ultrasounds and fetal echocardiography can result in missed referrals for detailed echocardiography, and some CHDs may remain undetectable in utero.2,17 Expanding these technologies to rural and underserved areas is essential, as these regions often lack access to specialized care.8 AI-based telemedicine can bridge this gap both in and out of clinics, allowing remote specialists to provide high-quality care regardless of geographic location.6,22 Taking a holistic and multidisciplinary approach—combining echocardiograms with AI-driven pattern recognition and genomic analysis—can lead to higher diagnostic accuracy and improved patient outcomes.
Further research is still needed to identify reliable biomarkers and develop a standardized AI-driven system to ensure consistent and accurate diagnoses for all families.20,21,23 While AI and omics offer promising avenues for reducing diagnostic errors, realworld implementation is still hindered by financial and logistical barriers, particularly in low-resource settings.7,21
Dr. Tapas Mondal is a Professor in the Department of Pediatrics at McMaster University and a practicing cardiologist at McMaster Children’s Hospital. His research and practical expertise focus on congenital heart disease, pediatric echocardiography, and pediatric heart failure.
Dr. Adrienn Szabo is an Assistant Professor in the Department of Pediatrics at McMaster University. After completing a two-year clinical research fellowship at The Hospital for Sick Children, she joined the McMaster Pediatric Cardiology Team in August 2022. Her areas of expertise include fetal echocardiography and general pediatric cardiology.
1. Song L, Wang Y, Wang H, Ma N, Meng Q, Zhu K, et al. Clinical profile of congenital heart diseases detected in a tertiary hospital in China: a retrospective analysis. Front Cardiovasc Med. 2023;10(1). Available from: doi:10.3389/fcvm.2023.1131383.
2. Nelle M, Raio L, Pavlovic M, Carrel T, Surbek D, Meyer-Wittkopf M. Prenatal diagnosis and treatment planning of congenital heart defects: Possibilities and limits. World J Pediatr. 2009;5(1):18-22. Available from: doi:10.1007/s12519-009-0003-8.
3. Zhang J, Xiao S, Zhu Y, Zhang Z, Cao H, Xie M, et al. Advances in the application of artificial intelligence in fetal echocardiography. J Am Soc Echocardiogr. 2024;37(5):550-61. Available from: doi:10.1016/j.echo.2023.12.013.
4. He F, Wang Y, Xiu Y, Zhang Y, Chen L. Artificial intelligence in prenatal ultrasound diagnosis. Front Med. 2021;8(1):729978. Available from: doi:10.3389/fmed.2021.729978. 5.
5. Chen L, Guan J, Wei Q, Yuan Z, Zhang M. Potential role of “omics” technique in prenatal diagnosis of congenital heart defects. Clin Chim Acta . 2018;482(1):185-90. Available from: doi:10.1016/j.cca.2018.04.011.
6. Smith VJ, Marshall A, Lie M, et al. Implementation of a fetal ultrasound telemedicine service: women’s views and family costs. BMC Pregnancy Childbirth . 2021;21(1):38. Available from: doi:10.1186/s12884-020-03532-4.
7. Londoño Trujillo D, Castro García PA, Rojas López KK, Moreno-Medina KJ, Dominguez Torres MT, Dennis Verano RJ, et al. Cost-effectiveness of the prenatal detection of congenital heart diseases: a systematic literature review. J Health Econ Outcomes Res. 2024;11(1):141148. Available from: doi:10.36469/001c.116147.
8. Vankevičienė R. Fetal echocardiography in Lithuania: traditions, significance and problems. Acta Med Litu . 2011;17(3-4):116-22. Available from: doi:10.2478/v10140-010-0015-7.
9. Abqari S, Gupta A, Shahab T, Rabbani MU, Ali SM, Firdaus U. Profile and risk factors for congenital heart defects: a study in a tertiary care hospital. Ann Pediatr Cardiol. 2016;9(3):216-21. Available from: doi:10.4103/0974-2069.189119.
10. Binder J, Carta S, Carvalho JS, Kalafat E, Khalil A, Thilaganathan B. Evidence for uteroplacental malperfusion in fetuses with major congenital heart defects. PLoS One. 2020;15(2). Available from: doi:10.1371/journal.pone.0226741.
11. Donofrio MT. Predicting the future: delivery room planning of congenital heart disease diagnosed by fetal echocardiography. Am J Perinatol . 2018;35(6):549-52. Available from: doi:10.1055/s-0038-1637764.
12. Waern M, Mellander M, Berg A, Carlsson Y. Prenatal detection of congenital heart disease - results of a Swedish screening program 2013–2017. BMC Pregnancy Childbirth. 2021;21(1):579. Available from: doi:10.1186/s12884-021-04028-5.
13. Albu CC, Albu DF, Albu SD, Patrascu A, Musat AR, Goganau AM. Early prenatal diagnosis of an extremely rare association of Down syndrome and transposition of the great vessels. Rev Chim. 2019;70(7):2574-78. Available from: doi:10.37358/RC.19.7.7383.
14. Yagel S, Weissman A, Rotstein Z, Manor M, Hegesh J, Anteby E, et al. Congenital heart defects: Natural course and in utero development. Circulation 1997;96(2):550-7. Available from: doi:10.1161/01.CIR.96.2.550.
15. Sun HY. Prenatal diagnosis of congenital heart defects: echocardiography. Transl Pediatr. 2021;10(8):2210-24. Available from: doi:10.21037/tp-20-164
16. Chaubal NG, Chaubal J. Fetal echocardiography. Indian J Radiol Imaging . 2009;19(1):60-8. Available from: doi:10.4103/0971-3026.44524.
17. Chaoui R. The four-chamber view: Four reasons why it seems to fail in screening for cardiac abnormalities and suggestions to improve detection rate. Ultrasound Obstet Gynecol. 2003;22(1):3-10. Available from: doi:10.1002/uog.187.
18. Kaur A, Hornberger LK, Fruitman D, Ngwezi D, Eckersley LG. Impact of rural residence and low socioeconomic status on rate and timing of prenatal detection of major congenital heart disease in a jurisdiction of universal health coverage. Ultrasound Obstet Gynecol. 2022;60:359-66. Available from: doi:10.1002/uog.26030.
19. Hill GD, Block JR, Tanem JB, Frommelt MA. Disparities in the prenatal detection of critical congenital heart disease. Prenat Diagn . 2015;35(1):859-63. Available from: doi:10.1002/ pd.4622.
20. Zhang J, Xiao S, Zhu Y, Zhang Z, Cao H, Xie M, Zhang L. Advances in the application of artificial intelligence in fetal echocardiography. J Am Soc Echocardiogr 2024;37(5):55061. Available from: doi:10.1016/j.echo.2023.12.013.
21. Yeo L, Luewan S, Romero R. Fetal intelligent navigation echocardiography (FINE) detects 98% of congenital heart disease. J Ultrasound Med. 2018;37(11):2577-93. Available from: doi:10.1002/jum.14616.
22. Liu X, Zhang Y, Zhu H, Jia B, Wang J, He Y, Zhang H. Applications of artificial intelligencepowered prenatal diagnosis for congenital heart disease. Front Cardiovasc Med 2024;11(1):1345761. Available from: doi:10.3389/fcvm.2024.1345761.
23. Chen L, Guan J, Wei Q, Yuan Z, Zhang M. Potential role of “omics” technique in prenatal diagnosis of congenital heart defects. Clin Chim Acta. 2018;482(1):185-90. Available from: doi:10.1016/j.cca.2018.04.011.
1 Bachelor of Science (Honours), Class of 2027, McMaster University
2 Bachelor of Science (Honours Integrated Science), Class of 2026, McMaster University
3 Bachelor of Health Sciences (Honours), Class of 2026, McMaster University
ARTIST:
FIONA DUYU4
4 Bachelor of Science (Honours Integrated Science), Class of 2027, McMaster University
Mitochondrial metabolism is the primary energy source which supplies the heart with adenosine triphosphate (ATP) through the process of oxidative phosphorylation. It is estimated that mitochondria within cardiomyocytes produce between 6-30 kg of ATP per day to sustain blood circulation, accounting for approximately 8% of total ATP consumption in the body.1–3 Beyond energy production, mitochondria are essential in regulating the levels of reactive oxygen species (ROS) and calcium ions within the myocardium, which is essential for maintaining cellular integrity. Age, lifestyle, genetics, and the environment can impair mitochondrial function, subsequently driving the pathophysiology of various cardiovascular diseases. Mitochondrial dysfunction results in reduced ATP production, excessive ROS accumulation, and calcium imbalances, leading to weaker cardiomyocytes and poorer heart health.1,4 In acute conditions such as myocardial infarction, non-functional scar tissue replaces damaged heart tissues and lacks the contractile ability of healthy myocardium, further compromising ATP production.5 While conventional treatments target related symptoms, mitochondrial dysfunction is largely left unaddressed.4 This gap highlights the potential use of mitochondrial transplantation (MT), a novel therapeutic strategy that introduces healthy mitochondria directly into damaged cardiac tissue.6 By restoring cellular energy balance, this approach could redefine treatment pathways for various heart diseases.
MT begins with organelle isolation through differential centrifugation, where cellular components are separated based on density.6 Traditionally, larger cell debris is first removed through low-speed centrifugation, followed by a high-speed sequence to isolate the smaller mitochondria from the cytosol. Although quite effective, this method often results in contamination
by other similar-sized organelles such as lysosomes. In addition, high-speed spins risk compromising mitochondrial membrane Recently, new protocols employing gradient ultracentrifugation with a sucrose density gradient can achieve This protocol includes an updated buffer system with sucrose osmolarity between 250300 mM, a physiological pH, and antioxidants such as Bovine serum albumin. These features help minimize osmotic stress and membrane permeability changes, preserving mitochondrial structure and reducing oxidative damage during isolation, thereby yielding a purer and more viable mitochondrial sample.7,8
Once isolated, mitochondria are delivered to damaged cardiac tissue through methods such as direct injection or intracoronary infusion via a coronary guiding catheter.9 Direct injections deliver mitochondria to specific areas of ischemic damage in the myocardium, improving cardiac function locally.10 In contrast, intracoronary infusion allows for a more extensive distribution of mitochondria across the myocardium and shows greater promise in cases of diffuse myocardial damage.10 Both targeted delivery methods enable effective mitochondrial integration, supporting rapid repair and recovery, with several studies noting significant improvement within 10 minutes.10
Following transplantation, mitochondria are internalized by cardiomyocytes through macropinocytosis.11 Internalized mitochondria then actively contribute to cellular respiration, restore ATP production, rebalance calcium levels, and reduce ROS accumulation.12 While research continues to refine mitochondrial isolation and transplantation methods, current advancements demonstrate potential in restoring bioenergetics and stability within damaged cardiac tissue.
MT has been shown to improve ischemia-reperfusion injury (IRI), a condition where the exacerbation of cellular dysfunction after restoration of blood flow is caused by damaged mitochondria and excess ROS production. A pilot study of autologous MT induced significant cardioprotection in pediatric patients recovering from postcardiotomy cardiogenic shock.13 MT improved ventricular strain and duration of functional recovery post-revascularization, which resulted in the successful weaning of patients from ECMO, an extracorporeal life support system. In vivo and in vitro studies of autologous MT
induced significant cardioprotection in pediatric patients recovering from postcardiotomy cardiogenic shock.13 MT improved ventricular strain and duration of functional recovery post-revascularization, which resulted in the successful weaning of patients from ECMO, an extracorporeal life support system. In vivo and in vitro studies of autologous MT in ischemia reported improved cell survival immediately before reperfusion in rats, with macropinocytosis occurring in as little as 10 minutes.14,15 Results demonstrated a significant decrease in cardiac enzymes, specifically creatine kinase MB and cardiac troponin-I, which are indicators of cardiac trauma, apoptosis, and infarct size in areas of reperfusion injury. Furthermore, normal contraction was restored 10 minutes after reperfusion. Notably, a delayed postreperfusion MT of autologous mitochondria may still have efficacy in cardioprotection.16 Blitzer et al. conducted an intracoronary delivery of mitochondria two hours after reperfusion in vivo, which resulted in cardioprotective effects, lower infarct size, and elevated cardiac output.16 These findings highlight the potential of MT in IRI treatment, which could lower long-term mortality rates. MT may also enhance organ transplantation techniques. In a heart transplantation performed on mice, contractile strength and ejection fraction were significantly improved, while necrosis and neutrophil infiltration were reduced within 24 hours of reperfusion. These results suggest that MT can decrease graft failure and enhance outcomes in transplant patients.17
Despite the promising outcomes of cardiovascular MT, the novelty of this research is associated with various risks and challenges. Although evidence indicates that mitochondria are internalized by cardiac tissue, some studies report as little as 2-7% of transplanted mitochondria detected in cardiomyocytes following MT.15 It is possible that enveloped mitochondria experience damage or membrane disruption while retaining their markers, potentially leading to misinterpretation of successful internalization. The source of mitochondria may also limit the clinical efficacy of transplantation. Generally, most studies use autologous mitochondria, which reduces the risk of immune rejection. However, subsequent extraction and transplantation remains a challenge. For aging patients or those with congenital
mitochondrial disease, autologous mitochondria may cause defects in other tissues and make harvesting more challenging, although recent studies have shown successful transplantation without any immune rejection in the heart and lungs.6 Furthermore, some studies indicate that transplanted mitochondria may induce an inflammatory response through damage-associated molecular patterns (DAMPs).18 However, more recent studies on intraperitoneal delivery of syngeneic and allogeneic mitochondria demonstrate successful injections without direct or indirect alloreactivity or DAMPs response, highlighting the progress in safety and efficacy of the MT technique.26 Although many animal and human studies have investigated direct injection of mitochondria into target tissues, gaining access to these tissues remains a challenge.18 Thus, addressing these challenges requires continued research to improve the efficacy and safety of MT.
While direct or intra-arterial injections are the most studied delivery modes of MT, they are invasive, costly, and often associated with extended recovery periods.13,20 Researchers have investigated alternative approaches such as coincubation and centrifugation methods as current clinical trials continue to explore the outcomes of MT through injection routes.6,21 There is growing interest in developing less invasive techniques, though they face challenges related to transportation across multiple biological barriers. Wu et al. reported a non-invasive oral delivery of MT through nanomotors. They attached methacrylate, a derivative of l-arginine, to the mitochondrial surface which releases nitric oxide (NO), a key signaling molecule in chemotaxis. This allows mitochondria to move towards injury sites containing high NO synthase and ROS concentration to facilitate MT.22 Alternatively, research in extracellular-vesicle-mediated MT has emerged as a promising delivery technique, inspired by human physiological mechanisms. Mature osteoblasts can secrete mitochondria and mitochondria-derived vesicles to support the differentiation of osteoprogenitors, while astrocytes can release functional mitochondria to hypoxic neurons.23,24 These mechanisms demonstrate the natural role of extracellular vesicles in transferring mitochondria in the body and indicate its potential in exogenous delivery.25 The future of mitochondrial transplantation entails more research in less invasive techniques, such as oral or exosomemediated deliveries, that take advantage of biotechnology to optimize costs, effectiveness, and safety of MT.
REVIEWED BY: DR. AYBUKE CELIK (PhD) & DR. JAMES MCCULLY (PhD)
Dr. Aybuke Celik is a researcher at the Boston Children’s Hospital and Harvard Medical School. His research focuses on mitochondrial transplantation as a therapeutic strategy.
Dr. James McCully is an Associate Professor of Surgery at Boston Children's Hospital and Harvard Medical School, where his research focuses on the mechanisms of biochemical molecular events contributing to myocardial cell death.
EDITED BY: ZAHRA TAUSEEF & LIZA NOORISTANI
ARTIST: ELAINE WANG
Bachelor of Health Sciences (Honours), Class of 2026, McMaster University
We believe storytelling has the power to transform. Here, on the Video Team, we create content for your knowledge and entertainment. We report on diverse topics, including interviews with McMaster faculty and students, medical
By Ken Yu, Jeffrey Sun, Yiming Zhang & James Wang
phenomena, scientific breakthroughs, and ethical dilemmas in medicine. Our mission is to inform, inspire, and engage— shaping the way we see and understand the world of healthcare.
Want to bring your stories to life? For the first time, the Meducator will be offering students the chance to submit ideas and collaborate with the Video Team to create a video of their choosing.
By David Klitovchenko and Ken Yu
Tooth decay is a problem that affects roughly over a third of the population. Although treatments like fillings and root canals can take care of this issue, sometimes the problem is so severe that teeth need to be extracted and replaced by implants. However, this is a complex and costly procedure that cannot always be performed in certain patients. In this video, we describe the alternative possibility of adult tooth regeneration by exploring how sharks naturally regenerate thousands of teeth over their lifetime.
In this video, we explore the ongoing threat of tuberculosis (TB), a bacterial airborne disease that, like COVID-19, spreads through coughing or sneezing. Learn how TB can affect not only the lungs but also the brain, bones, and other organs. From the deadly variations of pulmonary TB to the silent dangers of latent infections, we break down the science behind TB’s unpredictable pathogenesis, risk factors, and how it can be treated.
By Kavi Madan
As a result of organ donor shortage, 17 people die each day waiting for an organ transplant. In this video, we shed light on an emerging clinical procedure where organs from animals are transplanted into humans (commonly known as xenotransplantation). Scientists are hard at work, relentlessly engineering and testing strategies that mitigate patient immune responses to foreign organs. By navigating the complex landscape of xenotransplantation, we are making promising strides where the promise of organ availability will be met with clinical success and unwavering respect for all life.
EDITORS-IN-CHIEF
Florence Deng & Audrey Dong
EDITORIAL BOARD
Managing Editors
Dominic Gangemi, Veronica Grignano, & Ria Patel
Editors
Aarani Selvaganesh
Aditya Misra
Angela Hong
Atta Yazdy
Cynthia Duan
David Gou
Devlyn Sun
Emily Wang
Evan Sun
Evan Zhao
Firdose Khan
Ian Kim
Aleena Khan
Dina Wei
Elena Armand
VIDEO TEAM
Video Managers
Cindy Lin & Ryan Liu
Video Producers
Mahitaj Rashid
Megan Lee
Shauna Vanderhorst
Joel Abraham
Kathy He
Liza Nooristani
Matthew Olejarz
Nirujah Sutharsan Parth Arora
Ruhani Khattra Saejin (Grace) Hur
Will Zhang
Zahra Tauseef Zahrah Talawala
Jacqueline Chen Jia Jia Lu
GRAPHICS & DESIGN
Creative Directors
Elaine Wang & Henin Ye
Graphic Designers
Aymen Saeed
Aleeya Li
Ameya Gupta
Camela Temacini
Fiona Duyu
Iris Qian
Jessica Luo
Jessica Palfy
Michelle Wan
Nicole Kim Parisa Mishal Hossain
Sabrina Viloria
Sophie Li
Titus Tan
Tram Nguyen Yuewen Gao
Eleojo Audu
Kian Torabiardakani
Kobe Li
Krish Devgan
Noelle Di Perna
Michelle Giang
Sophie Ying
Tiffany Vo
MEDUPROMO
Director
Serena Wei
Medupromoters
Jessica Li Tenzin Tsarong
MEDUCOLLAB Directors
Raymond Qu & Zahra Tauseef MEDUFINANCE Directors
David Gou & Eric An MEDUEVENTS Directors
Olivia Kim & Aarani Selvaganesh
Meduevent Planners
Aiza Baano
Angeline Vo Hemtoj Deo Morgan Puusaari
SENIOR ADVISORS
Nishaad Sheth & Natalie Chu
WE WOULD LIKE TO THANK OUR SPONSORS FOR THEIR GENEROUS SUPPORT
Honours Health Sciences Program
McMaster Student Union
Office of the President