Ecological Engineering
Vegetated roofs show better performance as biodiversity attributes are increased
Manuscript Number:
Article Type: Research Paper
Keywords: urban ecosystems, biodiversity, performance, natives, microcosms
Corresponding Author: Natalia Cáceres
Instituto de Investigaciones en Recursos Naturales y Sustentabilidad José Sanchez
Labrador S.J. Universidad Católica de Córdoba -CONICET Córdoba, Córdoba ARGENTINA
First Author: Natalia Cáceres
Order of Authors: Natalia Cáceres
Federico O. Robbiati, Dr. Emmanuel C. Hick
Mario Suárez, Dr. Evangelina Mattof
Leonardo Galetto, Dr. Lelia Imhof, Dr.
Abstract:
Urban ecosystems like vegetated roofs contribute to both the cooling service and the resilience of socio-environments by providing ecosystem functions. We hypothesized that increasing biodiversity attributes in the arrangements of the potential species that can be used in vegetated roofs will improve their performance in the semiarid region of Central Argentina. We aimed to: (i) determine functional traits for the selected species to characterize different arrangements for the evaluation of the experimental treatments; (ii) evaluate their performance in microcosms arrangements comparing biodiversity attributes (from monocultures to combined species, life-forms, and functional traits), and (iii) evaluate the relationship among the best performer arrangements, the cooling service at the substrate level, and the provided ecosystem services. We expect those treatments with higher biodiversity attributes (combinations of species from ≥2 life-forms and ≥2 different functional groups) will show better performances than simpler ones for cooling services. Plant diversity attributes as indicators of ecosystem functions of eight species corresponding to different life-forms ( Sedum acre , S. lineare , S. reflexum , Phyla nodiflora , Glandularia x hybrida , Grindelia cabrerae , Eustachys distichophylla , and Nassella tenuissima ) were evaluated through a trial using 22 microcosmos’s treatments during a 12-month period. A principal component analysis was performed to detect species functional groups according to leaf (leaf area, specific lead area, and leaf dry matter content), and height traits. Likewise, plant performance parameters (survival dynamics, monthly coverage, and growth increments) were evaluated. A performance index was used to rank the best treatments and to relate the cooling service with substrate temperature. Species were clustered into three functional groups. After the selected period, all treatments reached over 86% survival rate. Eight treatments showed the best performance using a combined index that includes plant coverage, growth, and the variation coefficient of each treatment. All of them showed reductions in substrate temperatures with respect to the non-vegetated module. Although some of the monoculture treatments ( S. acre ; P. nodiflora , and E. distichophylla ) reached comparable values for the cooling service, our recommendation is to select those plant mixtures combining species, lifeforms, and functional groups because they also contribute to a wide spectrum of ecosystem services in urban environments.
IRNASUS Universidad Católica de Córdoba CONICET Av.ArmadaArgentina3555.Prov.Córdoba,RepúblicaArgentina Tel:+54 351 5426800
September22th,2021
To Prof.Dr.JanVymazal Editor in chief EcologicalEngineering
DearDr.Vynazal
Please find our article (Research Paper) entitled “Vegetated roofs show better performanceasbiodiversityattributesareincreased”co authoredbyFedericoO.Robbiati, Emmanuel C. Hick, Mario, Suárez, Evangelina Mattof, Leonardo Galetto, Lelia Imhof, and my selfforconsiderationforpublicationinEcologicalEngineering Journal.
Hopeyoufindthisarticlesuitableforpublication
Sincerelyyours
NataliaCáceres GraduateinEnvironmentalManagement FellowNationalResearchCouncilArgentina(CONICET) IRNASUS UCC CONICET
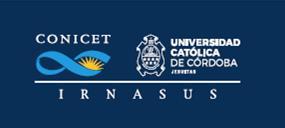
Declaration of interests
☒
The authors declare that they have no known competing financial interests or personal relationships that could have appeared to influence the work reported in this paper.
☐
The authors declare the following financial interests/personal relationships which may be considered as potential competing interests:
Biodiversity attributes improve cooling services of green roofs in semiarid regions.
Polycultures microcosms perform better than simpler ones in green roofs systems.
A Vegetated Roof Performance Index allowed ranking the best plant arrangements.
Vegetated roofs show better performance as biodiversity attributes are increased
Natalia Cáceres1,*, Federico O. Robbiati2, Emmanuel C. Hick1, Mario Suárez1 , Evangelina, Mattof3 , Leonardo Galetto4, Lelia Imhof1
1Laboratorio de Recursos Genéticos y Sustentabilidad Bioclimática, Instituto de Investigaciones en Recursos Naturales y Sustentabilidad José Sanchez Labrador S. J. (IRNASUS), Universidad Católica de Córdoba (UCC) CONICET. Av. Armada Argentina 3555, Córdoba, Argentina
2Facultad de Ciencias Exactas Físicas y Naturales, Universidad Nacional de Córdoba, Av. Velez Sarsfield 1611, Córdoba, Argentina.
3Agencia de Extensión Rural Córdoba, Instituto Nacional de Tecnología Agropecuaria (INTA), Presidente Roca, La Coruña esq, X5000, Córdoba, Argentina
4Departamento de Diversidad Biológica y Ecología, Facultad de Ciencias Exactas, Físicas y Naturales, Instituto Multidisciplinario de Biología Vegetal (IMBIV), Universidad Nacional de Córdoba (UNC) CONICET. Av. Velez Sarsfield 1611. Córdoba, Argentina
* Corresponding author:
E mail address: naacaceres@gmail.com (N. Cáceres)
Urban ecosystems like vegetated roofs contribute to both the cooling service and the resilience of socio environments by providing ecosystem functions. We hypothesized that increasing biodiversity attributes in the arrangements of the potential species that can be used in vegetated roofs will improve their performance in the semiarid region of Central Argentina. We aimed to: (i) determine functional traits for the selected species to characterize different arrangements for the evaluation of the experimental treatments; (ii) evaluate their performance in microcosms arrangements comparing biodiversity attributes (from monocultures to combined species, life forms, and functional traits), and (iii) evaluate the relationship among the best performer arrangements, the cooling service at the substrate level, and the provided ecosystem services. We expect those treatments with higher biodiversity attributes (combinations of species from ≥2 life forms and ≥2 different functional groups) will show better performances than simpler ones for cooling services. Plant diversity attributes as indicators of ecosystem functions of eight species corresponding to different life forms (Sedum acre, S. lineare, S. reflexum, Phyla nodiflora, Glandularia x hybrida, Grindelia cabrerae, Eustachys distichophylla, and Nassella tenuissima) were evaluated through a trial using 22 microcosmos’s treatments during a 12 month period. A principal component analysis was performed to detect species functional groups according to leaf (leaf area, specific lead area, and leaf dry matter content), and height traits. Likewise, plant performance parameters (survival dynamics, monthly coverage, and growth increments) were evaluated. A performance index was used to rank the best treatments and to relate the cooling service with substrate temperature. Species were clustered into three functional groups. After the selected period, all treatments reached over 86% survival rate. Eight treatments showed the best performance using a combined index that includes plant coverage, growth, and the variation coefficient of each treatment. All of them showed reductions in substrate temperatures with respect to the non vegetated module. Although some of the monoculture treatments (S. acre; P. nodiflora, and E. distichophylla) reached comparable values for the cooling service, our recommendation is to select those plant mixtures combining species, life forms, and functional groups because they also contribute to a wide spectrum of ecosystem services in urban environments.
Keywords: urban ecosystems, biodiversity, performance, natives, microcosms
Introduction
It was recently reported that more than 2.5 billion of the earth´s inhabitants would be concentrated in cities by the middle of the 21st century (UN DESA, 2019). This upcoming situation strongly suggests that transition to a predominantly urban life will require a transformative adaptation to future environmental pressures by boosting cities with biophilic perspectives (Wolfram et al., 2019; Nash et al., 2019; Collof et al., 2020; McDonald and Beatley, 2021). Based on Nature Solutions, constructed ecosystems (i.e., living roofs and walls, bioswale) are currently implemented as multi integrative alternatives to moderate urbanization effects such as urban temperature excess and contribute to global climate change adaptation and mitigation (Ahern et al., 2014; Hobbie and Grimm, 2020).
Vegetated Roofs (VRs), commonly named green roofs, can offer provision, regulation, cultural, and supportive ecosystem services simultaneously (Cook-Patton and Bauerle, 2012; Li and Yeung, 2014; Kotze et al., 2020). Some of the most promoted benefits correspond to energy saving and thermal insulation (La Roche and Berardi, 2014; Imhof et al., 2021), as well as flash flood management (Nawaz et al., 2015). Likewise, enhanced mineral retention, act as filters for air and noise pollution (Johnson et al., 2016; Azeñas et al., 2018; Van Renterghem, 2018), while providing refuge for a diversity of organisms (Fabián et al., 2021) and incrementing the connectivity of ecological networks (Mayrand and Clergeau, 2018). Additionally, as a part of a socio ecological system, VRs also deliver amenities and cultural values, offering welfare to modern citizens (Lee et al., 2014).
It is likely that VRs are exposed to environmental and structural barriers, creating hostile settings for vegetation development; thus, the living layer has to deal with high solar radiation, rapid speed wind velocities, growing medium composition and depths, periodic drought, among others (Emilsson, 2008; Butler and Orians, 2011). These climate and constructive boundaries that often limit the diversity of plants that VRs can hold also restrict all their potential benefits supported by a higher diversity (Farrell et al., 2012). Usually, the habitat template and trait base approaches have become commonly applied methods to explore and select suitable plants for VRs (Lundholm et al., 2015; Van Mechelen et al., 2014a; Van Mechelen et al., 2015; Rayner et al., 2016; Lundholm and Walker, 2018; Nagase and Tashiro Ishii, 2018). Plant functional
diversity has been demonstrated to improve ecological processes in nature such as designed systems, being the VRs an example of the latter (Cadotte, 2013; Cook-Patton, 2015; Córdova Tapia and Zambrano, 2015). Succulents, particularly from the genus Sedum, continue to be one of the most extended life forms used in the living roofs industry mainly for its stress tolerance strategies (Pérez et al., 2020). Naturally, these stonecrops grow under shallow soils and their leaf traits let them store water and use it strategically under environmental limited conditions (Rayner et al., 2016). On the other side, “Sedum designs” do not always contribute to integrate multiple functions as it is expected, sought as it tends to homogenize and simplify the assembly (Cook Patton and Bauerle, 2012). Nonetheless, species richness by itself is not warrantee to increase the system functionality; it is also important to increase traits divergence among the species implemented (Cadotte, 2013; Xie et al., 2018). To counteract this, one strategy has been through the studies of roof systems with plant microcosms displaying interactions between different life-forms (Lundholm et al., 2010). Plant communities can enhance their structural complexity by incorporating mixtures of succulents with other life forms (i.e., mosses, grasses, herbaceous), representing strategies of differential growth patterns and resources uptake (Vasl and Heim, 2016; Vasl et al., 2017) Biodiverse roofs are generally associated with optimization in plant survival and coverage, variables that have an influence on the VRs resilience (Nagase and Dunnett, 2010; MacIvor et al., 2013; Tran et al., 2019), and has been demonstrated to foster processes such as storm water regulation, thermal insulation; or nutrient leach out retention (Wolf and Lundholm, 2008; Castleton et al., 2010; Johnson et al., 2016). Among landscapers and ecologists, there is a growing interest for the adoption of native germplasm in urban areas, mainly because of their local environmental adaptation and for its contribution to biodiversity conservation and restoration (Butler et al., 2012; Azeñas et al., 2018; Nagase and Tashiro Ishii, 2018; Fabián et al., 2021). Even so, it is important to improve our knowledge on each species contribution to the VRs performance by selecting the best suitable botanical arrangements (Van Mechelen et al., 2014b; Heim et al., 2017). Determining appropriate living roof vegetation will enhance their benefits to urban environments and the available options to the consumer, the “green” roof industry, and environmental policy decisions Based on these considerations, we hypothesized that increasing biodiversity attributes (i.e., composition: different species; structure: different life forms; and functional: ecosystem services) in the arrangements of the potential species that can be used in VRs, will improve living roof performance and
ecosystem services in the semiarid region of Central Argentina. The objectives of this study were to: (i) determinate functional traits for the selected species to characterized different arrangements for the evaluation of the experimental treatments; (ii) evaluate their performance in different microcosms arrangements comparing biodiversity attributes (from monocultures to combined species, life forms, and functional traits); and (iii) evaluate the relationships between the performance of the best arrangements, the cooling service at the substrate level, and the ecosystem services provided. We expect those treatments combinations of species from ≥2 life forms and ≥2 different functional groups will show better performances than simpler ones for cooling services and, in consequence, these combinations of species with increased biodiversity attributes will be recommended to be used in VRs because they provided both higher energy saving and more ecosystem services.
2. Material and methods
2.1. Study site
A 78m2 vegetated roof (Figure 1 a) was constructed over a classroom workshop approximately 5m above ground level at the School of Architecture of the Catholic University Córdoba Campus (Córdoba Province, Argentina) (31 °29 '12.0 " S 64 °14 ' 32.2"W) The Province is located in the semiarid region of Central Argentina, characterized by high thermal seasonal amplitude, with hot and rainy summers (mean temperature 26ºC, with peaks up to 40ºC) and cold and dry winters (mean temperature 10ºC). The mean annual temperature 17.4ºC and the mean annual rainfall is in the range of 700 to 900mm (Derguy et al., 2019; Merlo et al., 2020). According to the Köppen Geiger climate classification, the region corresponds to a transition between a warm semi arid climate (type “BS”) and a humid subtropical climate (type “Cwa”) (Kottek et al., 2006; Susca, 2019). The experiment lasted for a full annual cycle, from September 2018 to September 2019.
Figure 1. (a) Vegetated roof trial overview at Faculty of Architecture at the Catholic University of Córdoba. (b) Technical scheme showing treatments distribution over the roof. Grey modules were planted with all the species and life forms used here but they were not weed (grey modules of 1m2); and the lasted 5/9m2 were planted with three native species (Scoparia montevidensis, Hyalis argentea, and Leymus arenarius) (grey modules of 0.5m2).
2.2. Vegetated roof modular system
An extensive modular system designed by our research team was used for the living roof construction. Each module presents an area of 0.5x1m2 with 0.15m of depth. The modules can be assembled between each other making an area of 1m2 From bottom to up, the first 0.03m of the module corresponded to moisture cells where only commercial perlite is added; over it, a thin anti root blanket was placed to avoid substrate mixed with perlite, followed by the substrate mixture. The substrate was prepared with wasted soil, compost, peanuts shells, and perlite (proportion: 3:1:1:1). Small holes were drilled in the base of the module to drain the excess water (94 holes m 2) (Supplementary Figure 1). For details of the physical and chemical properties of the substrate composition see Cáceres et al. (2018).
2.3. Plant material
A total of eight perennial species were chosen for the assessment. Plant species were provided by our lab team (Laboratorio de Recursos Genéticos y Sustentabilidad Bioclimática) from the Catholic University of Córdoba. Four of them were earlier characterized in experimental studies (in plots placed 1m above the ground level) and were selected by their success on vegetated roof modules in monoculture: Sedum acre
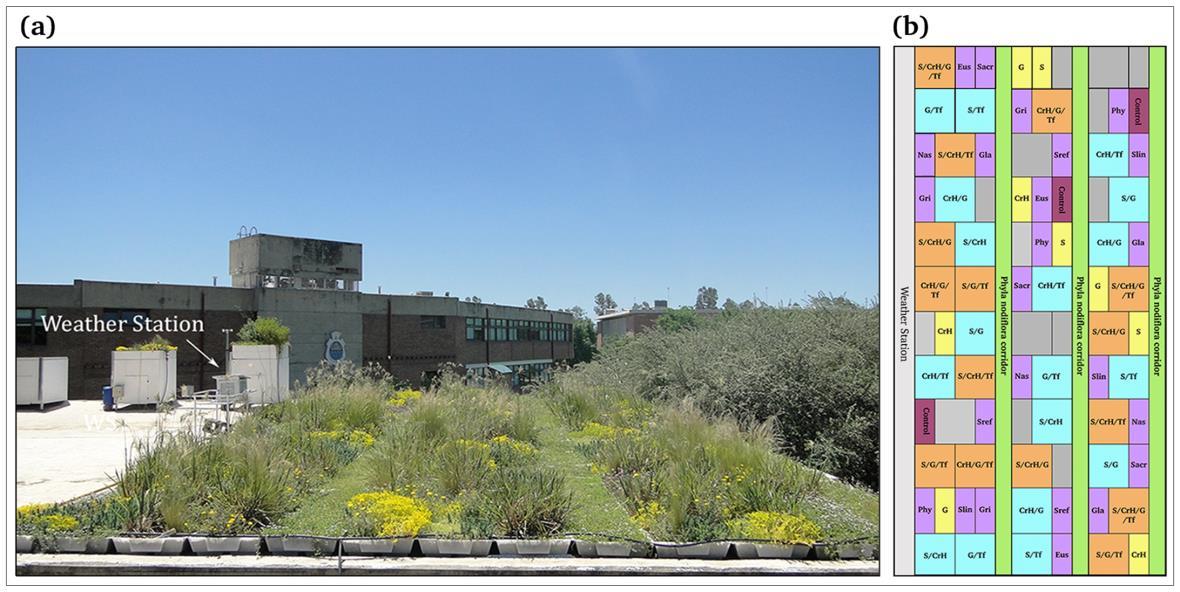
L. (Crassulaceae), Grindelia cabrerae Ariza (Asteraceae), Phyla nodiflora (L.) Greene, and Glandularia x hybrid (Verbenaceae) (Cáceres et al., 2018; Suárez et al., 2019). To enhance species richness within life forms, we added two non natives succulent accessions, Sedum lineare Thunb and Sedum reflexum L. (Crassulaceae), and two native grasses (Poaceae), Eustachys distichophylla (Lag.) Ness and Nassella tenuissima (Trin.)
Barkworth, both frequently used in local urban gardens. All the species were asexually propagated from stem cuttings and mat division and were cultivated under greenhouse conditions for about 30 days.
After this period and once plant materials managed to root, transplantation to the modular system was carried out on September 19th, 2018. Plant density was 20 24 individuals.m 2/treatment. The variations (20 to 24 plants.m 2) among the treatments were related to maintaining an equal initial green coverage within the plots. In addition, we mixed randomly both bigger and smaller plants of each species onto the plot configuration to reduce differences in size and growth between individuals. Species belonging to the same life form group in mixture treatments were distributed as dispersed as possible at the module surface.
2.4. Experimental setup
A total of twenty two treatments with three replicates were arranged in a randomized design over the roof (Figure 1 b). Treatments (i.e., microcosms) included combinations of (a) species growing in monoculture (3x8, n=24), (b) mixtures of species in one single life form (succulents; creeping herbs; and grasses; 3x3, n=9; tall forbs were excluded because this life form was represented by a single species), (c) mixtures of two, three, and all four life forms together (i.e., succulents/grasses; succulents/grasses/creeping herbs respectively; 3x11, n=33) (Table 1). For monocultures and single life form treatments, each plot had a total surface of 0.5m2, and for 2, 3 and all life form treatments, each plot had a total surface of 1m2. A total 49.5m2 were occupied by all the treatments To facilitate easy accesses for measurements to the treatments, three perpendicular rows were planted with the creeping herb P. nodiflora (corresponding to a surface of 18m2), keeping the edges of the rows cut by weeding for not to invade any plot A total of 10.5m2 of the VRs area were not consider for vegetation assessment in this study. 4/9m2 were planted with all the species and life forms used here, but any spontaneous species that grow was not remove from modules (grey modules of 1m2),
and the lasted 5/9m2 were planted with other three Argentinian native species (Scoparia montevidensis, Hyalis argentea, and Leymus arenarius) (grey modules of 0.5m2).
Finally, 1.5m2 were not planted with vegetation, and were used to measure the surface temperature of the bare substrate (dark red modules indicated as: “control”) (Figure 1 b).
Table 1. Vegetated roof with treatment configuration (n= 22, r:3) considering eight plant species and four life forms. Codes assigned for each species in monoculture and in mixture microcosms: 1 single life form, and two, three, and four life forms’ combinations.
Treatments
Monocultures
Code assigned
Sedum acre L. Sacr
Sedum lineare L. Slin
Sedum reflexum L. Sref
Glandularia x hybrida Gla
Phyla nodiflora (L.) Greene. Phy
Grindelia cabrerae Ariza Gri = Tf*
Eustachys distichophylla (Lag.) Ness. Eus
Nassella tenuissima (Trin.) Barkwoth. Nas
1 Single Life Form (species included)
Succulents (Sacr + Slin + Sref) S
Creeping herbs (Gla + Phy) CrH
Grasses (Eus + Nas) G
2 Life-Forms (species included)
Succulents (Sacr + Slin + Sref)/ Creeping herbs (Gla + Phy) S/CrH
Succulents (Sacr + Slin + Sref)/Grasses (Eus + Nas)
Succulents (Sacr + Slin + Sref)/Tall forb (Gri)
Creeping herbs (Gla + Phy)/ Grasses (Eus + Nas)
Creeping herbs (Gla + Phy)/Tall forb (Gri)
Grasses (Eus + Nas)/ Tall forb (Gri)
3 and 4 Life forms (species included)
Succulents (Sacr + Slin + Sref)/ Creeping herbs (Gla + Phy)/ Grasses (Eus + Nas)
Succulents (Sacr + Slin + Sref)/ Creeping herbs (Gla + Phy)/Tall forb (Gri)
S/G
S/Tf
CrH/G
CrH/Tf
G/Tf
S/CrH/G
S/CrH/Tf
Succulents (Sacr + Slin + Sref)/ Grasses (Eus + Nas)/ Tall forb (Gri) S/G/Tf
Creeping herbs (Gla + Phy)/ Grasses (Eus + Nas)/ Tall forb (Gri)
Succulents (Sacr + Slin + Sref)/ Creeping herbs (Gla + Phy)/ Grasses (Eus + Nas)/Tall forb (Gri)
*Tf: tall forb life form corresponding to G. cabrerae
CrH/G/Tf
S/CrH/G/Tf
An automatic irrigation system was used. To ensure plant establishment watering was applied intensively for the first two weeks to field capacity point, afterwards, modules were irrigated only by drips (2 Lh 1) twice a week for 30 minutes. To maintain initial treatments composition, spontaneous species were removed fortnightly, but new seedlings of the species under study were left to grow. The roof was fully sun exposed, without buildings next to it. Air temperature and rain frequency parameters were gathered from a weather station installed next to the planting roof during the period assessed (Figure 2). The evaluated period was particularly dry (mean annual precipitation: 455.9mm) when compared to the mean annual value (814mm) recorded from the period 1981 to 2010 by the National Meteorological Service (2021) (Observatorio Hidro Meteorológico Córdoba Weather Station) in an area close to our station.
Figure 2. Climatic data recorded by the weather station from September 2018 to September 2019. Minimum, maximum and mean air temperature (ºC in the points of the horizontal lines) and total monthly rainfall (mm in the bars).
2.5. Data collection
2.5.1. Functional traits of the species
To group species according to functional traits, specific leaf area (SLA), leaf area (LA), leaf dry matter content (LDMC), leaf water content (LWC), and plant size expressed as plant height (HGT) were recorded for each species following the methodology proposed by Pérez Harguindeguy et al. (2016). In February 2019 (first growing season) fifteen healthy, young and full fresh expanded leaves were selected from five individuals of the species growing in monoculture’s treatments. Leaves samples were analyzed at the Department of Forage of the University´s School of Agricultural Sciences. SLA was calculated as the mean one side area per fresh leaf divided by the mean oven dry leaf mass. Once leaves were collected, their fresh mass was quickly weighted to avoid water loss; subsequently, they were scanned (HP Scanjet 3770) to get leaf area, which was processed by digital image analysis (DIA) using ImageJ (vs.1.52a NIH, USA). Finally, LDMC (mg.g 1) was calculated as the oven dry leaf mass (mg; oven dried at 62°C for 72h), divided by its fresh weight (g). Leaf water content (LWC) was calculated as the difference between fresh and dry leaves values (g g 1). Vegetative height (HGT) was measured as the maximum distance (m) from the substrate, excluding reproductive structures (flowers or fruits). For each species the height values were obtained from three randomly selected individuals.
2.5.2. Performance measurements for the treatments
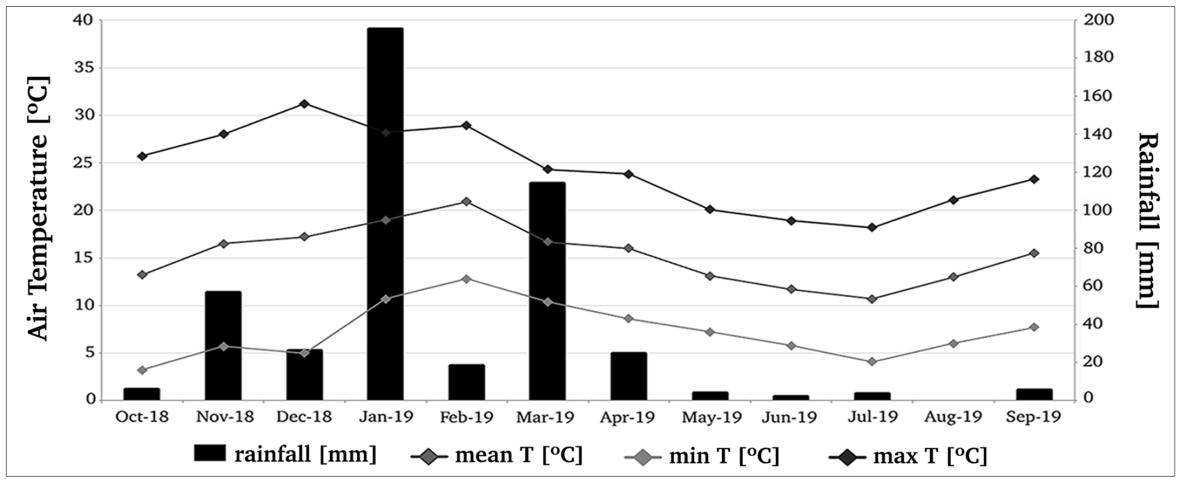
Survival percentages (S%) were measured by counting the number of individuals at a given time divided by the initial number of individuals planted of each species per treatment. Survival was counted with a bi monthly frequency (new seedlings of the species among the treatments were not counted).
Green coverage (Cov %) was measured through monthly photographs taken over each plot 1 meter above the vegetated roof module surface at late afternoon (from 06:00 to 07:30 pm to avoid shadows) in a horizontal plane. We set a 10cm scale as a reference for distance calibration of the images. Images were saved in Tiff format and then they were analyzed with the digital analysis program to obtain the green area percentage in the plot; finally, with the values of the green area, the coverage percentage for each plot was estimated for a given moment during September 2018 to September 2019 (ImageJ, vs.1.52a NIH, USA). We used the threshold tool to contrast the images and select the region of interest (ROI: i.e., green cover) with the wand tool. When it was not possible to contrast the green cover with the bare substrate, there was used the freehand tool by drawing carefully the outline of the plots’ surface covered by the vegetation
Annual Growth Increment (GrI) was calculated as the difference between final and initial coverage values (Cov Sep2019 Cov Sep2018) for each plot of the different treatments. Box plots containing means and quartiles were graphed to show GrI.
2.5.3 Vegetated Performance Index (PIndex)
We adapted the index from Chamas and Mattes (2000) and Suárez et al. (2019) to systematized, rank, and select the best treatments according to the final coverage percentage corresponding to September 2019 (Cov) and the greatest growth increment values (GrI). We also considered the coefficient of variation of these variables (CovCV; GrICV). With these data set we categorized the treatments calculating the performance index as follows:
VPIndex= (Cov x 0.25) + CovCV x 0.25) + (GrI x 0.25) + (GrICV x 0.25)
Each term of the formula had the same weight (1/4= 0.25) and the sum of scores had a maximum value of 1.0. In consequence, we transformed the values of these variables to obtain proportions considering the followings categories: final coverage (Cov) after twelve months of assessment (%) = outperforming coverage when treatments reached
>90% = 1; optimum values between 89 to 60% = 0.7; intermediate coverage 59 to 45%= 0.4; and poor coverage values below 44% = 0; for greatest growth increment (GrI) the values >85%= 1; between 84 and 60% = 0.7; values within 59 to 45% = 0.4; and below 44% = 0. For the coefficient of variation (CV) of the two variables, the proportions were assigned equally: minor to 5 = 1; between 5 and 10 = 0.7; between 10 and 20 = 0.4; and major to 20 = 0. The lower values for Cov and GrI were greatly penalized because (i) these species were previously selected with their high final coverage percentage from previous studies; and (ii) because the need to find the best species combinations in their performance (i.e., substrate temperature reduction) and ecosystem functions for semi arid regions.
2.5.4 Substrate temperature
The surface temperature was measured on February 27th, 2019 at solar noon (from 12:00 to 14:00pm) in one plot (= 1 replicate) of each treatment by inserting a hand thermometer probe at 2.5cm deep of the growing substrate in the center of each module away from irrigation drippers. A module with an exposed substrate represented the control condition when comparing cooling values with vegetated modules.
2.6. Data analysis
To determine functional groups for the studied species, we used a principal component analysis (PCA). To graphic coverage dynamics (monthly mean coverage values ± standard deviation), multivariate profiles were run to each treatment with statistical analysis program InfoStat (Di Rienzo, 2020). In addition, box plots containing medians and quartiles were prepared to showed growth increment. To evaluate the differences in growth increment among the treatments we used a Kruskal Wallis test. To relate the substrate surface temperature with the percentage of coverage achieved in summer for the treatments selected by the VPIndex, a linear regression analysis was run. Both analyses were run in R (version .3.0.2) (R Development Core Team, 2019).
3. Results
3.1 Species functional traits
The eight species were characterized according to different leaf traits and vegetative height measurements at the end of the first growing season (i.e., February 2019) The
largest leaf area (LA) value corresponded to Eustachys distichophylla, while the succulent Sedum reflexum held the smallest; the other species showed intermediate values. S. lineare had the highest value for specific leaf area (SLA), and N. tenuissima showed the lowest. Leaf dry matter content (LDMC) showed high values on native species concerning the exotic succulents. In contrast, leaf water content (LWC) was major within succulent species with respect to the five native species. On the other hand, the grasses E. distichophylla, Nassella tenuissima, the tall forb Grindelia cabrerae, and S. lineare reached similar high heights (HGT), followed by intermediate values for the species S. acre, S. reflexum, and Glandularia x hybrida; while Phyla nodiflora showed the smallest values (Supplementary Table 1).
The first three axes of the PCA analysis were retained and explained 93.9% of the total variance (47.7%, 30.3%, and 15.9% respectively) for these species and variables, and were projected on a two dimensional plane to observe the relationships among species (Figure 3 a, b). The plot of PC1 vs. PC2 showed two defined functional groups, one consisting of all specimens of S acre, S reflexum, P nodiflora, and G x hybrid, but this group was not clearly associated to any trait. The other group included all E. distichophylla specimens. The remaining sampled specimens of three species (G. cabrerae, S lineare, and N tenuissima) in this plot did not show a clear clustering pattern. The plot of PC1 vs. PC3 displayed a similar clustering pattern for the first two groups, but the main difference was that G. cabrerae, S. lineare, and N. tenuissima appeared grouped in the upper quadrants of Euclidean spaces. LA and LWC contributed in the clustering mostly associated with PC1; and SLA, HGT, and LDMC mostly associated with PC2 and PC3 (Figure 3 a, b; Supplementary Table 2). The dendrogram obtained in CA (cophenetic correlation coefficient r = 0.91) showed a similar clustering pattern to that obtained in the PCA; however, the samples of G. cabrerae and S. lineare appeared grouped (Supplementary Figure 2). Summarizing and to analyze treatment performance, we defined three functional groups of species (group 1: S acre, S reflexum, P. nodiflora, and G. x hybrid; group 2: E. distichophylla; group 3: S. lineare; G cabrerae, and N tenuissima).
Figure 3. Principal Component Analyses for species functional traits (93.9% of the variation explained). (a) plot of PC 1 and PC 2. (b) plot of PC 2 and PC 3. Traits are shown with black colored symbols: LA: Leaf area, SLA: Specific leaf area, LDMC: Leaf Dry Matter Content; LWC: Leaf Water Content, HGT: height. Species are shown with colored symbols. b: Species code: Sacr: Sedum acre; Sref: S. reflexum; Gla: Glandularia x hybrida; Phy: Phyla nodiflora; Slin: S. lineare; Gri: G. cabrerae; Nas: N. tenuissima; Eus: E. distichophylla.
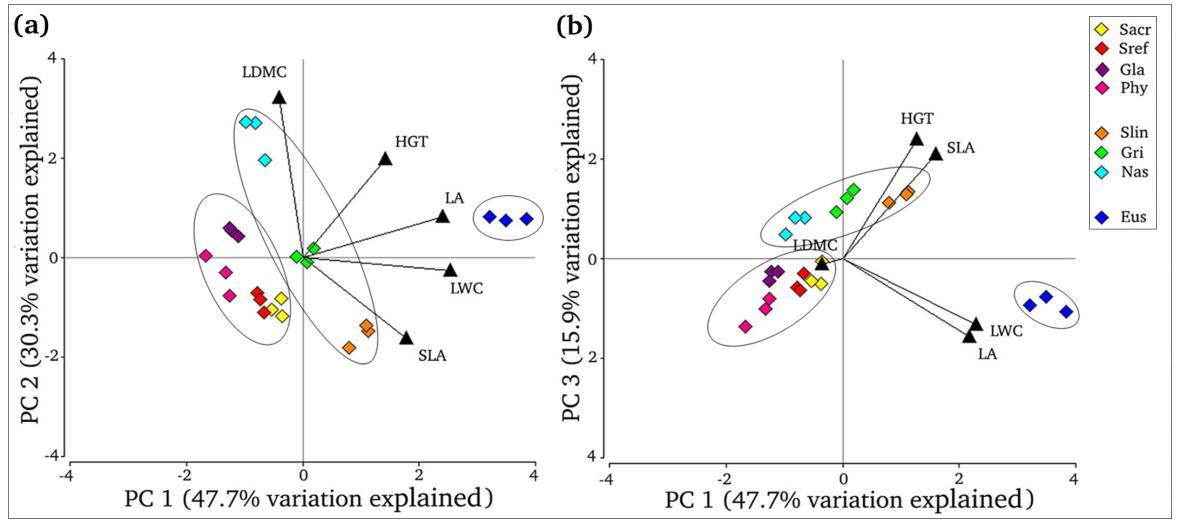
3.2. Vegetation performance
3.2.1. Survival dynamics
The 22 treatments showed high survival values (≥86%) at the end of the assessment, independently if species were in monoculture treatments or grouped within a single life form or combined with different ones (Table 2). The reduction in the number of individuals generally occurred during periods of extreme temperatures between December (maximum monthly mean temperature T: 31.2ºC) and July (minimum monthly mean temperature T: 4.1ºC). When increasing mixtures to two life forms, natives and succulents’ treatments showed 100% in survival to the end of the assessment (S/CrH; S/G; S/Tf). When the different life forms treatments were composed of native species (i.e., CrH/G; CrH/Tf; G/Tf), those that shown mortality (in at least one of the treatments replicates) were G. x hybrida, G. cabrerae and N. tenuissima. The treatments with 3 or 4 life forms showed high survival rates, and it was also observed that G. x hybrida and G. cabrerae were affected but to the end of the assessment period (Table 2).
Table 2. Dynamics of the survival (%) for the treatments through the experimental period. Measurements were obtained with a bimonthly frequency; M0: September 2018; M1: December 2018; M2: February 2019; M3: April 2019; M4: July 2019; M5: September 2019. Codes: Sacr: Sedum acre; Slin: S. lineare; Sref: S. reflexum; Gla: Glandularia x hybrida; Phy: Phyla nodiflora; Eus: Eustachys distichophylla; Nas: Nassella tenuissima; Gri = Tf: Grindelia cabrerae= tall forb); II- 1 life form mixtures (S: succulents, CrH: Creeping herbs; G: grasses); III: 2 life forms mixtures (S/CrH: succulents/creeping herbs; S/G: succulents/grasses; S/Tf: succulents/tall forb; CrH/G: creeping herbs/grasses; CrH/Tf: creeping herbs/tall forb; G/Tf: grasses/tall forb); IV 3 and 4 life forms mixtures (S/CrH/G: succulents/creeping herbs/grasses; S/CrH/TF: succulents/creeping herbs/tall forbs; S/G/Tf: succulents/grasses/tall forbs; CrH/G/Tf: creeping herbs/grasses/tall forb; S/CrH/G/Tf: succulents/creeping herbs/grasses/tall forb.
Treatments M0 M1 M2 M3 M4 M5
Sacr 100 100 100 100 100 100
Srefl 100 100 100 100 100 100
Slin 100 100 100 100 91.7 91.7
Phy 100 100 100 100 100 100
Gla 100 100 93.3 93.3 93.3 93.3
Phy 100 100 100 100 100 100
Eus 100 100 100 100 100 100
Nas 100 91.7 91.7 91.7 91.7 91.7
Gri = Tf 100 91.7 91.7 91.7 91.7 91.7
S 100 100 100 100 100 100
G 100 100 100 100 100 100
CrH 100 94.4 94.4 94.4 86.1 86.1
S/CrH 100 100 100 100 100 100
S/G 100 100 100 100 100 100
S/Tf 100 100 100 100 100 100
G/Tf 100 100 100 100 100 97.8
CrH/G 100 94.8 94.8 94.8 94.8 94.8
CrH/Tf 100 88.9 88.9 88.9 88.9 88.9
S/CrH/G 100 100 100 100 92.9 92.9
S/G/Tf 100 100 100 100 100 100
CrH/G/Tf 100 100 100 100 96.4 92.8
S/CrH/Tf 100 100 100 100 92 6 92.6
S/CrH/G/Tf 100 100 100 100 100 98.6
3.2.2 Coverage dynamics
Coverage percentage for each treatment was analyzed at three different stages: 1) establishment coverage, considered here as the growth after the first 30 days of transplantation to the VR system; 2) coverage after the first growing season (end of February 2019); and 3) final coverage at the end of the experimental period (September 2019) Four treatments showed a rapidly ground cover (>75%) after 30 days since transplantation, as the single creeping herbs life form (mean ± SE: CrH= 90.83 ± 2.61%), the mixture of succulents, creeping herbs, and tall forb (S/CrH/Tf=
81.21 ± 4.50%, followed by the treatment with four life forms mix (S/CrH/G/Tf= 81.02 ± 0.86%, and the monoculture S. acre (Sacr=74.76 ± 10.60%) (Figure 4 a, b, c, d). The rest of the treatments (18/22) reached an initial coverage percentage that varied between 63% and 14% during the first month. By the end of the first growing season (five months after transplantation), most treatments (16/22) displayed a coverage superior to 75%. In particular, they were the monocultures Sacr, Slin, and Phy (97.59 ± 1.67%; 88.4 ± 1.29%; 76.77 ± 4.91%, respectively, Figure 4 a), all single life form treatments (G= 91.19 ± 2.15%; S=89.50 ± 4.40%; and CrH= 88.37 ± 5.29%, Figure 4 b), five of the six 2 life forms microcosms (S/CrH= 93.70 ± 3.05%, S/G= 89.94 ± 3.52; CrH/G= 86.81 ± 3.18; S/Tf=80.65 ± 9.53; and CrH/Tf= 79.80 ± 2.92, Figure 4 c), and all the 3 and 4 life forms microcosms (S/CrH/G/Tf= 97.37 ± 0.94%; S/CrH/Tf= 96.90 ± 1.07; S/CrH/G= 95.75 ± 0.59; CrH/G/Tf= 95.05 ± 0.91; and S/G/Tf= 81.28 ± 4.04, Figure 4 d). By the end of the year round experimental period, 13/14 of mixture treatments and 5/8 of the monocultures reached a final mean coverage over 80% (18/22) (Figure 4 a, b, c, d). Compared to previous ones, the monocultures N. tenuissima and G. x hybrida showed lower ground coverage percentages (Nas: 65.96 ± 13.21 and Gla: 65.91 ± 8.79 respectively), while G. cabrerae (Gri: 46.84 ± 6.23%) reached the lowest value (Figure 4 a; Supplementary Figure 3).
Figure 4. Annual coverage dynamics performed by the treatments (n=22). (a) monocultures treatments; (b) 1 single life form; (c) 2 life forms; (d) 3 and 4 life forms (bars showed standard error). e: I- monocultures treatments (Sacr: Sedum acre; Slin: S. lineare; Sref: S. reflexum; Gla: Glandularia x hybrida; Phy: Phyla nodiflora; Eus: Eustachys distichophylla; Nas: Nassella tenuissima; Gri= Tf: Grindelia cabrerae= tall
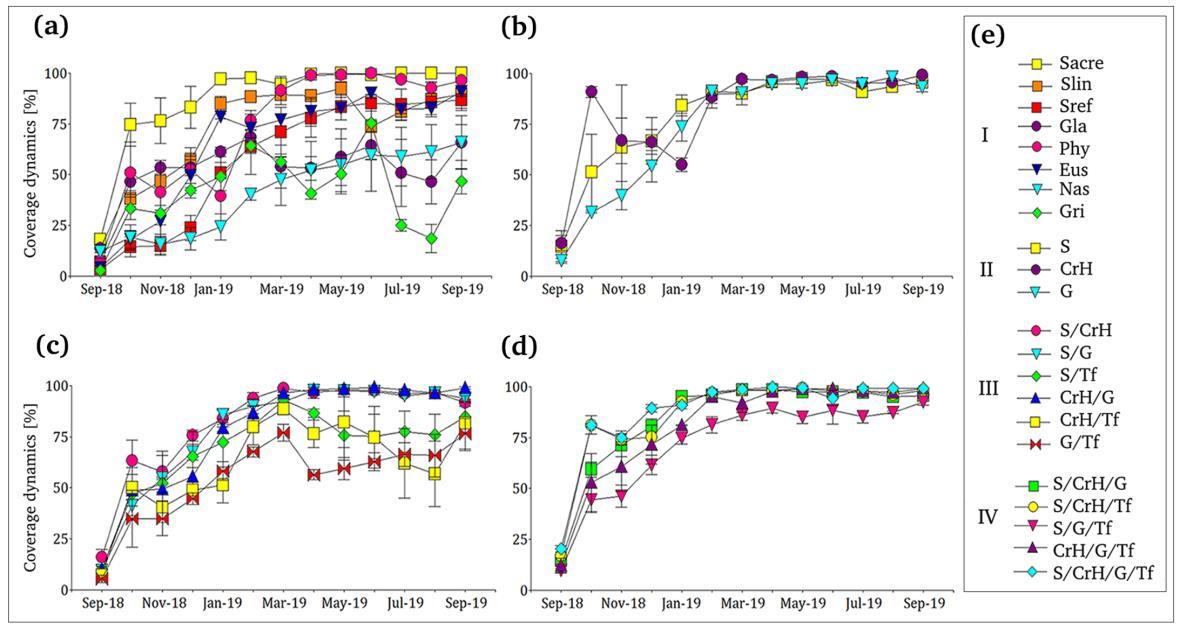
forb); II 1 life form mixtures (S: succulents, CrH: Creeping herbs; G: grasses); III: 2 life forms mixtures (S/CrH: succulents/creeping herbs; S/G: succulents/grasses; S/Tf: succulents/tall forb; CrH/G: creeping herbs/grasses; CrH/Tf: creeping herbs/tall forb; G/Tf: grasses/tall forb); IV 3 and 4 life forms mixtures (S/CrH/G: succulents/creeping herbs/grasses; S/CrH/TF: succulents/creeping herbs/tall forbs; S/G/Tf: succulents/grasses/tall forbs; CrH/G/Tf: creeping herbs/grasses/tall forb; S/CrH/G/Tf: succulents/creeping herbs/grasses/tall forb.
3.2.3. Annual growth increment
Most of the treatments had an increase in growth greater than 75% with exception of two mixtures (CrH/Tf = 74.57 ± 14.81%; G/Tf= 71.14 ± 5.83%) and the three monoculture treatments with the lowest values (Nas= 53.79 ± 11.51 %), Gla= 52.14 ± 8.80%, and Gri= 44.02 ± 5.64 %) (Figure 5), which were the same that reached the minor percentage in final coverage.
Figure 5. Box plot showing variability in growth increment from September 2018 to September 2019 for all the treatments studied (n=22, r=3). Dissimilar letters show significant differences between coverage rates among treatments, at p<0.05 Kruskal Wallis Test. Codes: Monocultures (Sacr: Sedum acre; Slin: S. lineare; Sref: S. reflexum; Gla: Glandularia x hybrida; Phy: Phyla nodiflora; Eus: Eustachys distichophylla; Nas: Nassella tenuissima; Gri: Grindelia cabrerae); 1 life form mixtures (S: succulents, CrH: Creeping herbs; G: grasses); 2 life forms mixtures (S/CrH: succulents/creeping herbs; S/G: succulents/grasses; S/Tf: succulents/tall forb; CrH/G: creeping herbs/grasses; CrH/Tf: creeping herbs/tall forb; G/Tf: grasses/tall forb); 3 and 4 life forms mixtures (S/CrH/G: succulents/creeping herbs/grasses; S/CrH/TF: succulents/creeping herbs/tall forbs; S/G/Tf: succulents/grasses/tall forbs; CrH/G/Tf: creeping herbs/grasses/tall forb; S/CrH/G/Tf: succulents/creeping herbs/grasses/tall forb.
3.3. VPIndex
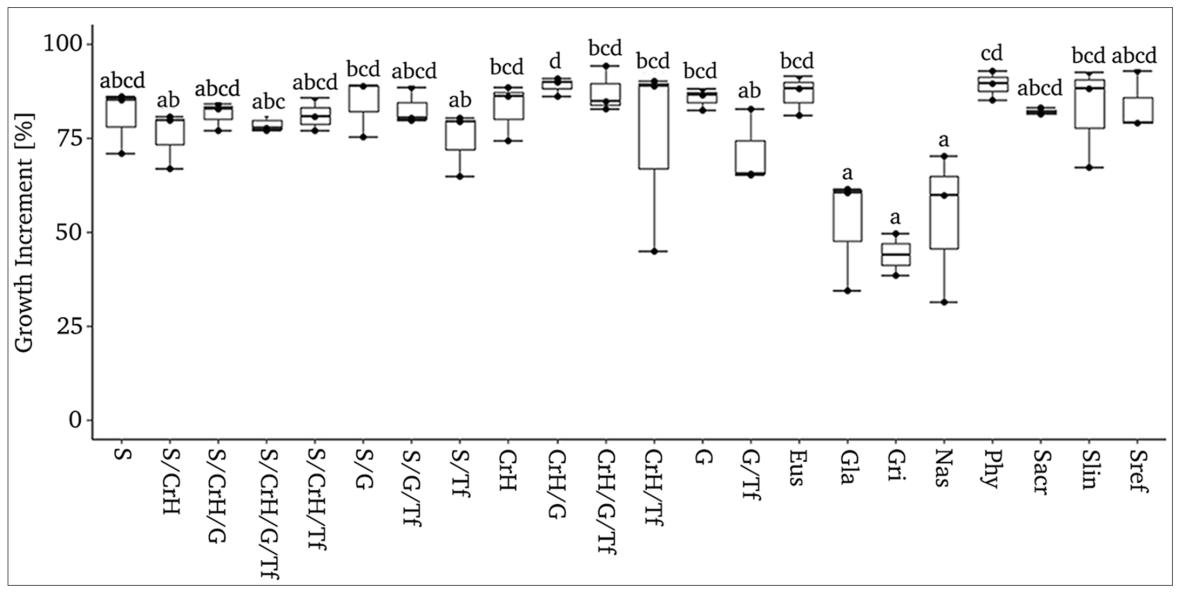
Eight treatments were selected over a cut line of 0.9 value of the VPIndex (G; Phy; Sacr; Eus; CrH/G; S/CrH/G; S/CrH/Tf; S/CrH/G/Tf) (marked in bold, Table 3). These treatments showed the higher coverage values and the greatest growth increment over a year period studied. Both variables (Cov: coverage and GrI: annual growth increment) showed minimal variations on these treatments Among the best eight treatments, four of them combined ≥2 life forms and ≥2 species from different functional groups (CrH/G; S/CrH/G; S/CrH/Tf; S/CrH/G/Tf); four other combinations matching with these criteria (S/G; S/CrH; S/G/Tf; S/CrH/Tf) showed close values in their index (0.85) to the first group of treatments; on the contrary, three groups combining ≥2 life forms and ≥2 species from different functional groups (G/Tf; S/Tf; CrH/Tf) showed low values for the VPIndex (Table 3).
Table 3. Vegetated Performance Index (VPIndex) for the treatments assessed (n=22). The first eight treatments were the best ranked (showed in bold capital). Cov: final coverage (values corresponded to September 2019); CovCV: variation coefficient for coverage; GrI: growth increment, and GrICV: variation coefficient for growth increment. The value of 0.25 corresponds to the weight of each variable involved in the performance index. Codes: Sacr: Sedum acre; Slin: S. lineare; Sref: S. reflexum; Gla: Glandularia x hybrida; Phy: Phyla nodiflora; Eus: Eustachys distichophylla; Nas: Nassella tenuissima; Gri= Tf: Grindelia cabrerae= tall forb); II 1 life form mixtures (S: succulents, CrH: Creeping herbs; G: grasses); III: 2 life forms mixtures (S/CrH: succulents/creeping herbs; S/G: succulents/grasses; S/Tf: succulents/tall forb; CrH/G: creeping herbs/grasses; CrH/Tf: creeping herbs/tall forb; G/Tf: grasses/tall forb); IV- 3 and 4 life form mixtures (S/CrH/G: succulents/creeping herbs/grasses; S/CrH/TF: succulents/creeping herbs/tall forbs; S/G/Tf: succulents/grasses/tall forbs; CrH/G/Tf: creeping herbs/grasses/tall forb; S/CrH/G/Tf: succulents/creeping herbs/grasses/tall forb.
Treatments Cov. x 0.25 CovCV x 0.25 GrI x 0.25 GrICV x 0.25 VPIndex
Sacr 0.25 0.25 0.25 0.25 1
Phy 0.25 0.25 0.25 0.25 1
G 0.25 0.25 0.25 0.25 1
CrH-G 0.25 0.25 0.25 0.25 1
Eus 0.25 0.25 0.175 0.25 0.925
S/CrH/G 0.25 0.25 0.175 0.25 0.925
CrH/G/Tf 0.25 0.25 0.25 0.175 0.925
S/CrH/G/Tf 0.25 0.25 0.25 0.175 0.925
CrH 0.25 0.25 0.175 0.175 0.85
S/G 0.25 0.25 0.175 0.175 0.85
S/CrH 0.25 0.25 0.175 0.175 0.85
S/G/Tf 0.25 0.25 0.175 0.175 0.85
S/CrH/Tf 0.25 0.25 0.175 0.175 0.85
S 0.25 0.25 0.175 0.1 0.775
Sref 0.175 0.1 0.175 0.175 0.625
G/Tf 0.75 0.1 0.175 0.1 0.55
S/Tf 0.175 0.1 0.175 0.1 0.55
Slin 0.175 0.1 0.175 0.1 0.55
CrH/Tf 0.75 0 0.175 0 0.35
Gri 0.1 0.1 0 0.1 0.3
Gla 0.175 0 0.1 0 0.275
3.4. Substrate temperature
The eight treatments selected according to the highest values of the VPIndex (marked in bold, Table 3) were used to analyze the correlation between coverage and temperature of the substrate (summer season, date 27th February 2019). The mean values for each treatment showed that a green coverage greater to 60% conducted to an important decrease (ca. 25%) on substrate temperature in comparison with control (module with exposed substrate) (Figure 6). Among these treatments, four of them combine ≥2 life forms, and ≥2 species from differential functional groups (CrH/G; S/CrH/G; S/CrH/Tf; S/CrH/G/Tf) and showed a considerable reduction in the substrate temperature (Figure 6).
Figure 6. Substrate temperature for the selected treatments (n=8) according to the Vegetated Performance Index (VPIndex) (R= 0.90, p= 0.05); Control: bare substrate; Sacr: S. acre; Phy: P. nodiflora; Eus: E. distichophylla; G: grasses; CrH/G: creeping herbs/grasses S/CrH/G: succulents/creeping herbs/grasses; CrH/G/Tf: creeping herbs/grasses/tall forb; S/CrH/G/Tf: succulents/creeping herbs/grasses/tall forb.
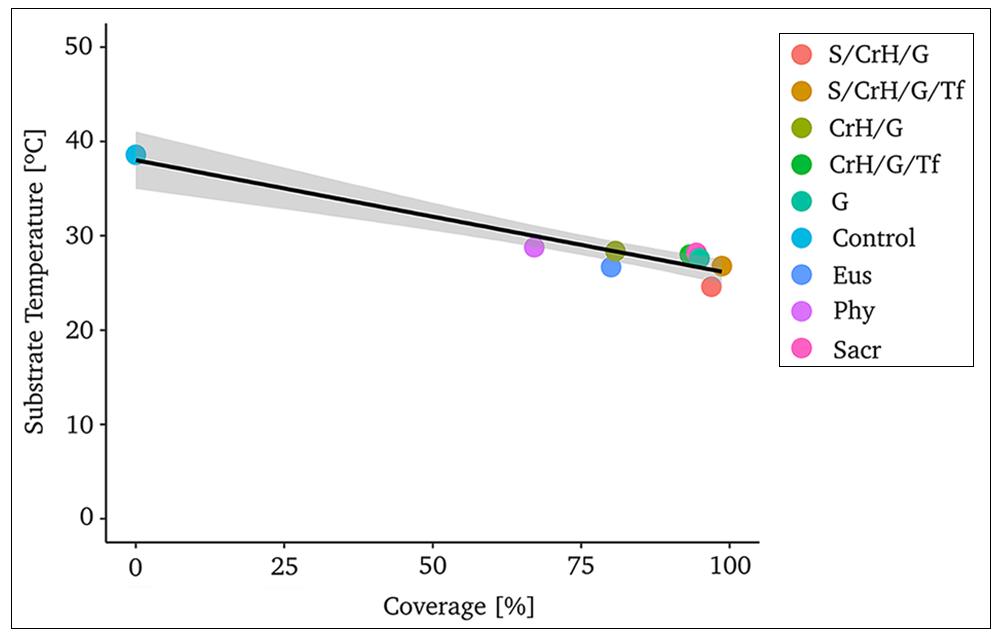
Discussion
Functional description
Plant biodiversity composition and structure have been associated with an improvement of services for humans’ societies from natural and also from built ecosystems (Díaz et al., 2007; Lundholm et al., 2010; Van Mechelen et al., 2015). Likewise, it is important to consider the functional attribute of biodiversity (Cook Patton and Bauerle, 2012; Lundholm et al., 2015; Xie et al., 2018) because it is related to many ecological processes. Traits related to leaf morphology and physiology, as well as plant height have been used as indicators for plant selection since they showed strength in predicting several of the ecosystem functions offered by VRs (Van Mechelen et al., 2014a; Lundholm et al., 2015). Moreover, these easy measurable plant traits (i.e., LA, SLA, LDMC, LWC, HGT) contribute to growth, survival and can be related to species life history strategies (Lundholm et al., 2010, 2014; Rayner et al., 2016). Nevertheless, not all the species in a diverse community assembly contribute equally to the functional attribute (Hector and Bagchi, 2007; Cadotte, 2013); thus, it is important to characterize each species according to their traits and also identify those shared among species.
From our results, one group composed of two different life forms (succulents and the creeping herbs), didn’t show a clearly association with any of the functional traits. However, both life forms were characterized by small LA, low SLA, and low HGT.
Experimental data on extensive VRs had shown that high values of SLA and higher HGT are correlated, directly or indirectly, through microcosms properties such as plant canopies, growth rates and albedo, and those to functions of the VRs such as substrate temperature reductions and water uptake (Lundholm et al., 2015). We found some species, as one of the succulent species (S. lineare), showing high values of SLA and similar values in HGT with respect to the tallest species (the tall forb Grindelia cabrerae and the grasses Nassella tenuissima and Eustachys distichophylla). In consequence, individually, these four species could be the best options for improving the performance and ecosystem services in VRs. Moreover, and after multivariate analysis, E. distichophylla constitutes a separate functional group, and this species can be characterized by its large LA. This trait is associated with higher productivity and transpiration, processes that improve cooling and water retention services (Lundholm and Williams, 2015).
LDMC varied among species but was greater in the native species. This trait is related to major productivity and leaf lifespan, being those species with high LDMC assumed to be less vulnerable to physical hazards such as herbivory (Pérez Harguindeguy et al.,
2016). Thus, these species could present a better survival performance in VRs when herbivores or physical phenomena produce leaf damages. On the contrary, succulents generally have low values of LDMC and SLA, and high leaf thickness (Pérez Harguindeguy et al., 2016). Nevertheless, survival is improved in succulents because LWC is higher in this group compared with other life forms. This trait is related to succulence and is useful to improve species survival in the arid and semiarid conditions of VRs (Farrell et al., 2012; Razzaghmanesh et al., 2014b; Rayner et al., 2016). All the succulents assessed here showed the highest values for LWC, while the lowest values corresponded to the native species.
These pros and cons among species and considering different traits could result in comparable survival under the stressful conditions of VRs. Nevertheless, we found differences between three of the native’s species which showed mortality in their individuals (G. x hybrid, G. cabrerae, N. tenuissima), but the other two species (P. nodiflora and E. distichophylla) with similar LWC values did not. This pattern is supported by evidence from a recent study that failed to observe a relationship between the degree of succulence and the survival of shrubs and forbs species (Guo et al., 2021).
4.2. Vegetation performance assessment
In general, watering on extensive VRs have been demonstrated to enhance plant performance (Nagase and Dunnett, 2010; Rayner et al., 2016; Vestrella et al., 2018). For example, a study carried out in Australia revealed that vegetation survival over extensive systems subsidized only with rainwater was not enough, but under watering provision plant survival reached up 80% (Razzaghmanesh et al., 2014a). Benvenuti and Bacci (2010), who tested species survival in dry VR conditions, also reinforced the need for supplemental watering for periods conditioned by stress factors (i.e., high temperatures, drought). In this study, all the treatments tested over a year with annual rainfall below the mean values outperformed high survival rates (>86%) at minimal irrigation conditions Plant mortality mainly occurred during periods of extreme temperatures during the summer and the winter in the same three species (G. x hybrida, G. cabrerae, and N. tenuissima), independently of the experimental treatment. The microcosms with these species (monocultures or in combination with other species) also showed the lowest coverage and the lowest growth increment compared to the rest of the treatments. Additionally, both species had been observed to become woody to the
second growing season which could affect their performance over time (Cáceres et al., 2018; Suárez et al., 2019).
By increasing species composition within the same life form, all the treatments outperformed in survival and achieved a stable coverage plateau towards the end of the first growing season. The creeping herb treatment (CrH) showed a rapidly spread over the module surface, mainly occupied by P. nodiflora with respect to G. x hybrid, which was also affected by high summer temperatures. Both species are phylogenetically close, belonging to the same botanical family (Verbenaceae), and may compete for space, making probably coexistence unstable (Burns and Strauss, 2011; Cadotte, 2013).
The high ability of P. nodiflora to reproduce vegetatively and its fast resprouting has been recently reported (Sosa et al., 2017; Cáceres et al., 2018). Interestingly, asexual reproduction is considered a criterion for VRs species selection, because it allows stability in the system in the long term (Van Mechelen et al., 2014b) and contribute to replacing species with slower growth development (Vasl and Heim, 2016) or with higher mortality. For succulent and grasses single life forms treatments (S; G), no losses in individual’s survival were registered. Despite that individual species growth in mixtures treatments was not measured, greater growth increments after twelve months were observed by S. acre and S. lineare with respect to S. reflexum, and also for E. distichophylla with respect to N. tenuissima. Lundholm et al. (2014) found greater biomass on S. acre over the other species of the same single life form, and the highest abundance in Poa compresa with respect to Deschampsia flexuosa. This is frequently attributed to the differential responses comparing the lower growth rates of species growing in co plantings mixtures compared to monocultures conditions (Lundholm et al., 2014). Mechanisms such as competitive exclusion were proposed to explain these differences when co planting species with similar growth patterns (Grime, 1973; Lundholm et al., 2014; Vasl and Heim, 2016).
For microcosms with 2 life forms, we found that the treatments that included succulent’s species enhanced the survival of the other three life forms (creeping herbs, grasses, or tall forb) because individuals of the less performing native species (G. x hybrida, G. cabrerae, or N. tenuissima) were not lost. The combination of different species on extensive VRs have been proved to enhance survival when co planting with Sedums (Butler and Orians, 2011). Moreover, coverage for the treatments including Sedum (S/CrH; S/G; S/Tf) was stable over time. Succulent plants are commonly used
because ameliorate the other species performance (commonly known as “nursering effect”) driven by interspecific facilitation mechanisms such as water availability for species that are less stress tolerant or by providing surface temperature reductions (Yeaton and Cody, 1976; quoted from Nagase and Dunnett, 2010; Heim and Lundholm, 2014).
The combination between grasses with creeping herbs, and grasses with the tall forb treatments (CrH/G; G/Tf respectively) also increase the overall performance of the treatments. On the other hand, similarly as above, the creeping herbs and tall forb treatment (CrH/Tf), increased the overall coverage with respect to G. x hybrida and G. cabrerae in monoculture, but both species were conditioned by the high growth displayed by P. nodiflora. Among the treatments, both species showed long flowering periods and can be offered to increased native species’ public acceptance, as well as for attracting pollinators by prolonging blooms (Nagase et al., 2017), and species survival experienced a minimal decrease at winter season.
Our main finding showed that by increasing microcosm biodiversity in composition, structure, and functional traits, performance was improved, including the treatment composed only by native species. Here, we also found key monocultures (all Sedum spp ; and two natives, P. nodiflora and E. distichophylla) that outperformed a comparable performance as the treatment with mixtures of different species, life forms, and functional groups. However, and including a wider perspective, others authors demonstrated that an increment in life forms complexity enhances ecosystem processes, offering multi functions instead of species isolated as well as improves VRs resilience (Lundholm, 2015; Johnson et al., 2016; Tran et al., 2019). When multiple species perform better than a species in monoculture it is known as transgressive overyielding and could be considered as a mixture advantage (Cadotte, 2013; Xie et al., 2018).
The FLL guidelines (FLL, 2008) point out that vegetation coverage in such constructed systems should achieve a minimum of 80%. Our best treatments present comparable coverages but also optimum values for a VPIndex that integrates indicators for VRs after a year of assessment under minimal irrigated conditions. Here, we adapted the index to rank those treatments with less variation (variation coefficients) in plant coverage and growth Although some of the monoculture treatments (Sedum acre, P. nodiflora, and E. distichophylla) or the grasses single life form treatment reached
comparable values, our recommendation is to select those plant mixtures combining species, life-forms, and functional groups because they provide more ecosystem services in urban environments as well as excellent cooling services for the building. In addition, biological corridors in urban environments are promoted increasing biodiversity on many groups of organisms and consequently VRs with many native species surely contribute to this goal.
4.3. Surface cooling service
The eight treatments were used to relate their performance index with substrate temperature as an indirect indicator of VRs cooling effect during the summer season. Our results supported a previous thermal performance study carried out in parallel by our research team. The vegetated roof (i.e., without differentiating in vegetation treatments) compared with a white exposed roof had reduced 5 6ºC the inside surface temperature (Imhof et al., 2021). In this sense, thermal studies emphasize that the presence of coverage and its seasonal variation plays a fundamental role in heat insulation since it varies among climates (La Roche and Berardi, 2014; Bevilacqua et al., 2015).
The surface cooling effect is known to be augmented by increasing species richness and life forms, and the variations in the VRs responses to be related to vegetation traits (i.e., leaf traits: SLA, height, transpiration rate or stomatal resistance, plant shading), to physical properties of the substrate, to climatic conditions (i.e., air temperature, albedo, solar radiation), or to building characteristics (De Nardo et al., 2005; Bevilacqua et al., 2015; Steinfort et al., 2018; Cascone et al., 2019). Depending on climate conditions, the only use of succulents may be delaying the cooling effect on the substrate because this life form presents high stomatal resistance, and therefore the evapotranspiration mechanisms are reduced (Monteiro et al., 2016; 2017). As an alternative, herbaceous plants showed higher transpiration rates (Lundholm et al., 2015) improving cooling effects.
More studies focused on variations on morphological and eco physiological traits of the plants used in the VRs and then related with longer climatic measurements and on the building are also needed to understand the real contribution on the thermal efficiency of life forms co plantings on semiarid vegetated roofs. As vegetation originally implanted
over VRs changes over time as well as its functional diversity (Ksiazek Mikenas and Köhler, 2018), long-term evaluations are also needed in this field to better understand plant successional stages and how these variations in species composition impact over constructed ecosystems.
5. Conclusions
From the results provide here, our recommendation is to design VRs considering diverse plant assemblages with at least two or more species, different life forms, and divergence in plant traits to increase system functionality and to improve their contribution to the provision of different ecosystem services Besides, knowledge about species survival rate, their coverage dynamics, and their growth increment, both in monoculture and in mixtured microcosms, can optimize rooftop landscaping designs adjusting planting density, which in turn would optimize performance stability and improve species interactions. Promoting designs with native flora can contribute to reduced water inputs, and enhance other services such as pollination, fruit dispersion, and seed bank that contribute to temporal sustainability of constructed ecosystems.
Author Contributions
Natalia Cáceres: Conceptualization, Methodology, Data curation, Formal analysis, Writing Original draft, Writing Review & Editing. Federico O. Robbiati: Data curation, Formal analysis, Writing Original draft, Writing Review & Editing.
Emmanuel C. Hick: Data curation, Technical support for roof construction. Mario, Suárez: Data curation, Technical support for roof construction. Evangelina, Mattof: Data curation, Technical support for roof construction. Leonardo Galetto: Conceptualization, Methodology, Writing Original draft, Writing Review & Editing, Supervision, Funding acquisition. Lelia Imhof: Conceptualization, Methodology, Writing Original draft, Writing Review & Editing, Supervision, Funding acquisition.
Declaration of Competing Interest
The authors declare that they have no known competing financial interests or personal relationships that could have appeared to influence the work reported in this paper.
Funding sources
This work was supported by grants to Lelia Imhof (PIODO, 2017) and Leonardo Galetto (SECyT-UNC 411/18A and PICT 2015-0538).
Acknowledgments
We thank to Department of Forage of the University´s School of Agricultural Sciences, IRNASUS (UCC CONICET), IMBIV (UNC CONICET), SECyT (UNC), MINCyT Córdoba, MINCyT Argentina.
REFERENCES
Ahern, J., Cilliers, S., Niemelä, J., 2014. The concept of ecosystem services in adaptive urban planning and design: a framework for supporting innovation. Lands. Urban Plan. 125(Special issue), 254 259. https://doi.org/10.1016/j.landurbplan.2014.01.020
Azeñas, V., Janner, I., Medrano, H., and Gulías, J., 2018. Performance evaluation of five Mediterranean species to optimize ecosystem services of green roof under water limited conditions. J. Environ. Manage. 212, 236 247. https://doi.org/10.1016/j.jenvman.2018.02.021
Benvenuti, S., Bacci, D., 2010. Initial agronomic performances of Mediterranean xerophytes in simulated dry green roofs. Urban Ecosys. 13(3), 349 363. https://doi.org/10.1007/s11252 010 0124 9
Bevilacqua, P., Coma, J., Pérez, G., Chocarro, C., Juárez, A., Solé, C., …and Cabeza, L.F., 2015. Plant cover and floristic composition effect on thermal behavior of extensive green roofs. Build. Environ. 92, 305 316. https://doi.org/10.20868/ade.2020.4502
Butler, C., Orians, C.M., 2011. Sedum cools soil and can improve neighboring plant performance during water deficit on a green roof. Ecol. Eng. 37, 1796 1803. https://doi.org/ 10.1016/j.ecoleng.2011.06.025
Butler, C., Butler, E., Orians, C.M., 2012. Native plants enthusiasm reaches new heights: Perceptions, evidence, and the future of green roofs. Urban For. Urban Green. 11, 1 10. https://doi.org/10.1016/j.ufug.2011.11.002
Burns, J.H., Strauss, S.Y., 2011. More closely related species are more ecologically similar in an experimental test. PNAS. 108(13), 5302-5307. https://doi.org/10.1073/pnas.1013003108
Chamas, C.C., and Mattes, L.A.F., 2000. Método para levantamento de espécies nativas con potencial ornamental. Ornam. Hortic. 6, 53 63. https://doi.org/10.14295/rbho.v6il.63.
Cáceres, N., Imhof, L., Suárez, M., Hick, E.C., and Galetto, L., 2018. Assessing native germplasm for extensive green roof systems of semiarid regions. Ornam. Hortic. 24, 466 476. https://doi.org/10.14295/oh.v24i4.1225
Cadotte, M.W., 2013. Experimental evidence that evolutionarily diverse assemblages result in higher productivity. PNAS. 110(22), 8996 9000.
Cascone, S., Coma, J., Gagliano, A., Pérez, G., 2019. The evapotranspiration process in green roofs: a review. Build. Environ. 147, 337 355. https://doi.org/10.1016/j.buildenv.2018.10.024
Castleton, H.F., Stovin, V., Beck, S.B.M., Davison, J.B., 2010. Green roofs, building energy savings and the potential for retrofit. Energy Build. 42(10), 1582 1591. https://doi.org/10.1016/j.enbuild.2010.05.004
Colloff, M.J., Wise, R.M., Palomo, I., Lavorel, S., and Pascual, U., 2020. Nature´s contribution to adaptation: insights from examples of the transformation of social ecological systems. Ecosys. People. 16(1), 137 150.
Cook Patton, S.C., Bauerle, T.L., 2012. Potential benefits of plant diversity on vegetated roofs. J. Environ. Manage. 106, 85 92. https://doi.org/10.1016/j.jenvman.2012.04.003
Cook Patton, S.C., 2015. “Plant biodiversity on Green Roofs” In Green Roofs Ecosystems (pp.193 209). Springer, Cham.
Córdova Tapia, F., and Zambrano, L., 2015. La diversidad funcional en la ecología de comunidades. Ecosistemas. 24(3), 78 87. https://doi.org/10.7818/ECOS.2015.24 3.10
De Nardo, J.C., Jarret, A.R., Mambeck., H.B., Beattie, D.J., Berghage, R.D., 2005. Stormwater mitigation and surface temperature reduction by green roofs. ASAE. 48(4), 1491 1496. ISSN 0001 2351.
Derguy, M.R., Frangi, J.L., Drozd, A.A., Arturi, M. F., Martinuzzi, S., 2019. “Holdrigdge life zone map Republic of Argentina.” USDA Forest Service, International Institute of Tropical Forestry General Technical Report IITF GTR xx
Díaz, S., Lavorel, S., de Bello, F., Quétier, F., Grigulis, K., and Robson., M., 2007. Incorporating plant functional diversity effects in ecosystem service assessments. Proc. Natl Acad. Sci. USA. 104, 52, 12 2007; 20684 20689.
Di Rienzo, J., Casanoves, F., Balzarini, M., González L., Tablada, M., and Robledo, Y. C., 2020. Grupo InfoStat, FCA, Universidad Nacional de Córdoba, Argentina. URL https://www.infostat.com.ar/
Emilsson, T., 2008. Vegetation development on extensive vegetated green roofs: Influence of substrate composition, establishment method and species mix. Ecol. Eng. 33, 265 277. https://doi.org/10.1016/j.ecoleng.2008.05.005
Fabián, D., González, E., Sánchez Domínguez, M.V., Salvo, A., Fenoglio, M.S., 2021.
Towards the design of biodiverse green roofs in Argentina: Assessing key elements for different functional groups of arthropods. Urban For. Urban Green. 61, 127107. https://doi.org/10.1016/j.ufug.2021.127107
Farrell, C., Mitchell, R.E., Szota, C., Rayner, J.P., and Williams, N.S.G., 2012. Green roofs for hot and dry climates: Interacting effects of plant water use, succulence, and substrate. Ecol. Eng. 49, 270 276. https://doi.org/10.1016/j.ecoleng.2012.08.036
Forschungsgessellschaft Landschaftsentwicklung Landschaftbau e V. (FLL)., 2008. “Guidelines for the planning, execution, and upkeep of green roof sites.” Bonn, Germany.
Grime, J.P., 1973. Competitive exclusion in herbaceous vegetation. Nature 242(5396), 344 347. https://doi.org/10.1038/242344a0
Guo, B., Arndt, S., Miller, R., Lu, N., Farrell, C., 2021. Are succulence or trait combinations related to plant survival on hot and dry green roofs? Urban For. Urban Green. 64, 127248. https://doi.org/10.1016/j.ufug.2021.127248
Hector, A., Bagchi, R., 2007. Biodiversity and ecosystem multifunctionality. Nature 448(7150), 188 190. https://doi.org/10.1038/nature05947
Heim, A., Lundholm, J., 2014. Species interaction in green roofs vegetation suggest complementary planting mixtures. Landsc. Urban Plan. 130, 125 33. https://doi.org/10.1016/j.landurbplan.2014.07.007
Heim, A., Lundholm, J., 2016. Phenological complementarity in plant growth and reproduction in a green roof ecosystem. Ecol. Eng. 94, 82 87. https://doi.org/10.1016/j.ecoleng.2016.05.018
Heim, A., Appleby Jones, S., Lundholm, J., 2017. Green roof thermal and stormwater performance comparison between natives and industry standard plant species. CATE. 9(1), 6. https://digitalcommons.lmu.edu/cate
Hobbie, S.E. and Grimm N.B., 2020. Nature based approaches to managing climate change impacts in cities. Phil. Trans. R. Soc. B 375:20190124. doi: http://dx.doi.org/10.1098/rstb.2019.0124
Imhof, L., Suárez, E., Cáceres, N., Robbiati, F., Cáceres, C., Broilo, A., et al. Galetto, L., 2021. Thermal performance of and extensive green roof under semi arid conditions in Central Argentina. J. Green Build. 6(1), 17 42. https://doi.org/10.3992/jgb.16.1.17
Johnson, C., Schweinhart, S., and Buffan, I., 2016. Plant species richness enhances nitrogen retention in green roof plots. Ecol. Appl. 26(7), 2130 2144. https://doi.org/10.1890/15 1850.1
Kotze, D.J., Kuoppamäki, K., Niemikapee, J., Mesimäki, M., Vaurola, V., Lehvävirta, S., 2020. A revised terminology for vegetated rooftops based on function and vegetation. Urban For. Urban Green. 49, 126644. https://doi.org/10.1016/j.ufug.2020.126644
Kottek, M., Greiser, J., Beck, C., Rudolf, B., Rubel, F., 2006. World Map of the Köppen-Geiger climate classification update. Meteorol. Z. 15(3), 259-263. https://doi.org/10.1127/0941 2948/2006/0130
Ksiazek Mikenas, K., and Köhler, M., 2018. Traits for stress tolerance are associated with long term plant survival on green roofs. J. Urban Ecol. 1 10. https://doi.org/10.1093/jue/juy016
La Roche, P., Berardi, U., 2014. Comfort and energy savings with active green roofs. Energy Build. 82, 492-504. https://doi.org/10.1016/j.enbuild.2014.07.055
Li, W.C., Yeung, K.K.A., 2014. A comprehensive study of green roof performance from environmental perspective. Int. J. Sustain. Built Environ. 3(1), 127 134. https://doi.org/10.1016/j.ijsbe.2014.05.001
Lee, K.E., Williams, K.J.H., Sargent, L.D., Farrell, C., Williams, N.S., 2014 Living roof preference is influenced by plant characteristics and diversity. Landsc. Urban Plan. 122, 152-159. https://doi.org/10.1016/j.landurbplan.2013.09011
Lundholm, J., MacIvor, J.S., MacDougall, Z., Ranalli, M., 2010. Plant species and functional group combinations affect green roof ecosystem functions. PLoS ONE 5(3): e9677. https://doi.org/10.1371/journal.pone.0009677
Lundholm, J., Heim, A., Tran, S., Smith, T., 2014. Leaf and life history traits predict plant growth in a green roof ecosystem. PLoS ONE 9(6), e101395. https://doi.org/10.1371/journal.pone.0101395
Lundholm, J.T., 2015. Green roof plant species diversity improves ecosystem multifunctionality. J. App. Ecol. 52(3), 726 734. https://doi.org/10.1111/1365 2664.12425
Lundholm, J., Tran, S., Gebert, L., 2015. Plant functional traits predict green roof ecosystem services. Environ. Sci. Technol. 49(4), 2366 2374. https://doi.org/10.1021/es505426z
Lundholm, J.T., Walker, E.A., 2018. Evaluating the habitat template approach applied to green roofs. Urban Naturalist S 1, 39 51.
Lundholm, J., Williams, N., 2015. Effects of Vegetation on Green Roof Ecosystem Services. In: Sutton R. (eds) Green Roof Ecosystems. Ecological Studies (Analysis and Synthesis), vol 33. Springer, Cham. https://doi.org/10.1007/978 3 319 14983 7_9
MacIvor, J.S., Margolis L., Puncher, C.L., Matthews, B.J.C., 2013. Decoupling factors affecting plant diversity and cover on extensive green roofs. J. Environ. Manage. 130, 297 305. https://doi.org/10.1016/j.jenvman.2013.09.014
Mayrand, F., Clergeau, P., 2018. Green roofs and green walls for biodiversity conservation: a contribution to urban connectivity? Sustainability 10(4), 985. https://doi.org/10.3390/su10040985
McDonal, R., Beatley, T., 2021. Biophilic Cities: Vision and Emerging Principles. In Biophilic Cities for an Urban Century (Why nature is essential for the success of cities). pp. 63 85). Palgrave Pivot, Cham. https://doi.org/10.1007/978 3 030 51665 9
Merlo, C., Vázquez, C., Iriarte, A.G., Romero, C.M., 2020. Chemical and spectroscopic characterization of humic substances from sediment and riparian soil of a highly polluted urban river (Suquía River, Córdoba, Argentina). Int. J. Sediment Res. 35(3), 287 294.
Monteiro, M.V., Blanusa, T., Verhoef, A., Hadley, P., and Cameron, R.W.F., 2016. Relative importance of transpiration rate and leaf morphological traits for the regulation of leaf temperature. Aust. J. Bot. 64(1), 32 44. https://doi.org/10.1071/BT15198
Monteiro, M.V., Blanusa, T., Verhoef, A., Richardson, M., Hadley, P., Cameron, R.W.F., 2017. Functional green roofs: Importance of plant choice in maximizing summertime environmental cooling and substrate insulation potential. Energy Build. 141, 56 68. https://doi.org/10.1016/j.enbuild.2017.02.011
Nagase, A., Dunnett, N., 2010. Establishment of an annual meadow on extensive green roofs in the UK. Landsc. Urban Plan. 112, 50 62. https://doi.org/10.1016/j.landurbplan.2012.12.007
Nagase, A., Dunnett, N., Choi, M.S., 2017. Investigation of plant growth and flower performance on a semi extensive green roof. Urban For. Urban Green 23, 61 73. https://doi.org/10.1016/j.ufug.2017.01.013
Nagase, A., Tashiro Ishii, Y., 2018. Habitat template approach for green roofs using a native rocky see coast plant community in Japan. J. Environ. Manage. 206, 255-265. https://doi.org/10.1016/j.jenvman.2017.10.001
Nash, C., Anca Ciupala, M., Gedge, D., Lindsay, R. Connop, S., 2019. An ecomimicry design approach for extensive green roofs. JLIV 6(1), 62 81. https://doi.org/10.46534/jliv.2020.06.01.062
Nawaz, R., McDonald, A., Postoyko, S., 2015. Hydrological performance of a full scale extensive green roof located in a temperate climate. Ecol. Eng. 82, 66-80. https://doi.org/10.1016/j.ecoleng.2014.11.061
Pérez Harguindeguy, N., Díaz, S., Garnier, E., Lavorel, S., Poorter, H., Jaureguiberry, P., …et al. Cornelissen, J.H.C., 2016. Corrigendum to: new handbook for standardized measurement of plant functional traits worldwide. Aust. J. Bot. 64(8), 715 716. https://doi.org/10.1071/BT12225_CO
Pérez, G., Chocarro, C., Juárez, A., Coma, J., 2020. Evaluation of the development of five Sedum species on extensive green roofs in a continental Mediterranean climate. Urban For. Urban Green. 48, 126566. https://doi.org/10.1016/j.ufug.2019.126566
Servicio Meteorológico Nacional Argentino., 2021. https://www.argentina.gob.ar/smn [Accessed September 3, 2021]
Sosa, A.J., Cardo, M.V., Julien, M.H., Moonen, A.C., 2017. Predicting weed distribution at the regional scale in the native range: environmental determinants and biocontrol implications of Phyla nodiflora (Verbenaceae). Weed Res 57(3), 193 203. https://doi.org/10.1111/wre.12247
Steinfort, U., Arriola Cepeda, R., Vera, S., Albornoz, F., 2018. Experimental study on the stomatal resistance of green roof vegetation of semiarid climates for building energy simulations. IBPC. https://doi.org/10.14305/ibpc.2018.gb2.01
Suárez, M., Galetto, L., Cáceres, N., Hick, E., Mattof, E., Imhof, L., 2019. Performance of native plant genotypes (Glandularia Verbenaceae) on semi intensive green roofs with low maintenance requirements. CATE. 12(2), 1 17. https://digitalcommons.lmu.edu/cate/vol12/iss2/3
Susca, T., 2019. Green roofs to reduce building energy use? A review on key structural factors of green roofs and their effects on urban climate. Build. Environ 162, 106273. https://doi.org/10.1016/j.buildenv.2019.106273
R Development Core Team., 2019. R: A language and Environment for Statistical Computing. Vienna, Austria: R Foundation for Statistical Computing.
Rasband, W.S., Image J, U.S.A., National Institutes of Health, Bethesda, Maryland, USA, https://imagej.nih.gov/ij/, 1997 2018
Rayner, J.P., Farrell, C., Raynor, K.J., Murphy, S.M., Williams, N.S., 2016. Plant establishment on a green roof under extreme hot and dry conditions: the importance of leaf succulence in plant selection. Urban For. Urban Green. 15, 6 14. https://doi.org/10.1016/j.ufug.2015.11.004
Razzaghmanesh, M., Beecham, S., Brien, C.J., 2014a. Developing resilient green roofs in a dry climate. Sci. Total Environ. 490, 579 589. https://doi.org/10.1016/j.scitotenv.2014.05.040
Razzaghmanesh, M., Beecham, S., Kazemi, F., 2014b. The growth and survival of plants in urban green roofs in a dry climate. Sci. Total Environ. 476, 288 297. https://doi.org/10.1016/j.scitotenv.2014.01.014
Tran, S., Lundholm, J.T., Staniec, M., Robinson, C.E., Smart, C., Voogt, J.A., O´Carroll, D.M., 2019. Plant survial and growth on extensive green roofs: a distributed experiment in three climate regions. Ecol. Eng.127, 494 503. https://doi.org/10.1016/j.ecoleng.2018.09.027
United Nations, Department of Economic and Social Affairs, Population, Division., 2019. World Urbanization Prospects: The 2018 Revision (ST/ESA7SER.A/420). New York: United Nations. https://doi.org/10.18356/b9e995fe en
Van Mechelen, C., Dutoit, T., Kattge, J., Hermy, M., 2014a. Plant trait analysis delivers an extensive list of potential green roof species for Mediterranean France. Ecol. Eng. 67, 48 59. https://doi.org/10.1016/j.ecoleng.2014.03.043
Van Mechelen, C., Dutoit, T., Hermy, M., 2014b. Mediterranean open habitat vegetation offers great potential for extensive green roofs design. Landsc. Urban Plan. 121, 81 91. https://doi.org/10.1016/j.landurbplan.2013.09.010
Van Mechelen, C., Dutoit, T., Hermy, M., 2015. Adapting green roof irrigation practices for a sustainable future: A review. Sustain. Cities Soc. 19,74 90. https://doi.org/10.1016/j.scs.2015.07.007
Van Renterghem, T., 2018. Improving the noise reduction by green roofs due to solar panels and substrate shaping. Build. Acoust. 25(3), 219-231. https://doi.org/10.1177/1351010X18776804
Vasl, A., Heim, A., 2016. Preserving plant diversity on extensive green roofs theory to practice. Isr. J. Ecol. Evol 62(1 2), 103 111. https://doi.org/10.1080/15659801.2015.1035507
Vals, A., Shalom, H., Kadas, G.J., Blaustein, L., 2017. Sedum Annual plant interactions on green roofs: facilitation, competition and exclusion. Ecol. Eng. 108, 318-329. https://doi.org/10.1016/j.ecoleng.2017.07.034
Vestrella, A., Biel, C., Savè, Robert., Bartoli, F., 2018. Mediterranean Green Roof Simulation in Caldes de Montbui (Barcelona): Thermal and Hydrological Performance Test of Frankenia laevis L., Dymondia margaretae Compton, and Iris lutescens Lam. Appl. Sci. 8(12), 2497. https://doi.org/10.3390/app8122497
Wolf, D., Lundholm, J.T., 2008. Water uptake in green roof microcosms: effects of plant species and water availability. Ecol. Eng. 33(2), 179 186. https://doi.org/10.1016/j.ecoleng.2008.02.008
Wolfram, M., Borgström, S., Farrelly, M., 2019. Urban transformative capacity: From concept to practice. Ambio. 48(5), 437 448. https://doi.org/10.1007/s13280 019 01169 y
Xie, G., Lundholm, J., MacIvor, S., 2018. Phylogenetic diversity and plant trait composition predict multiple ecosystem functions in green roofs. Sci. Total Environ. 628 629, 1017 1026. https://doi.org/10.1016/j.scitotenv.2018.02.093
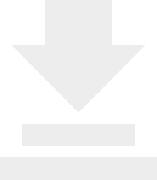