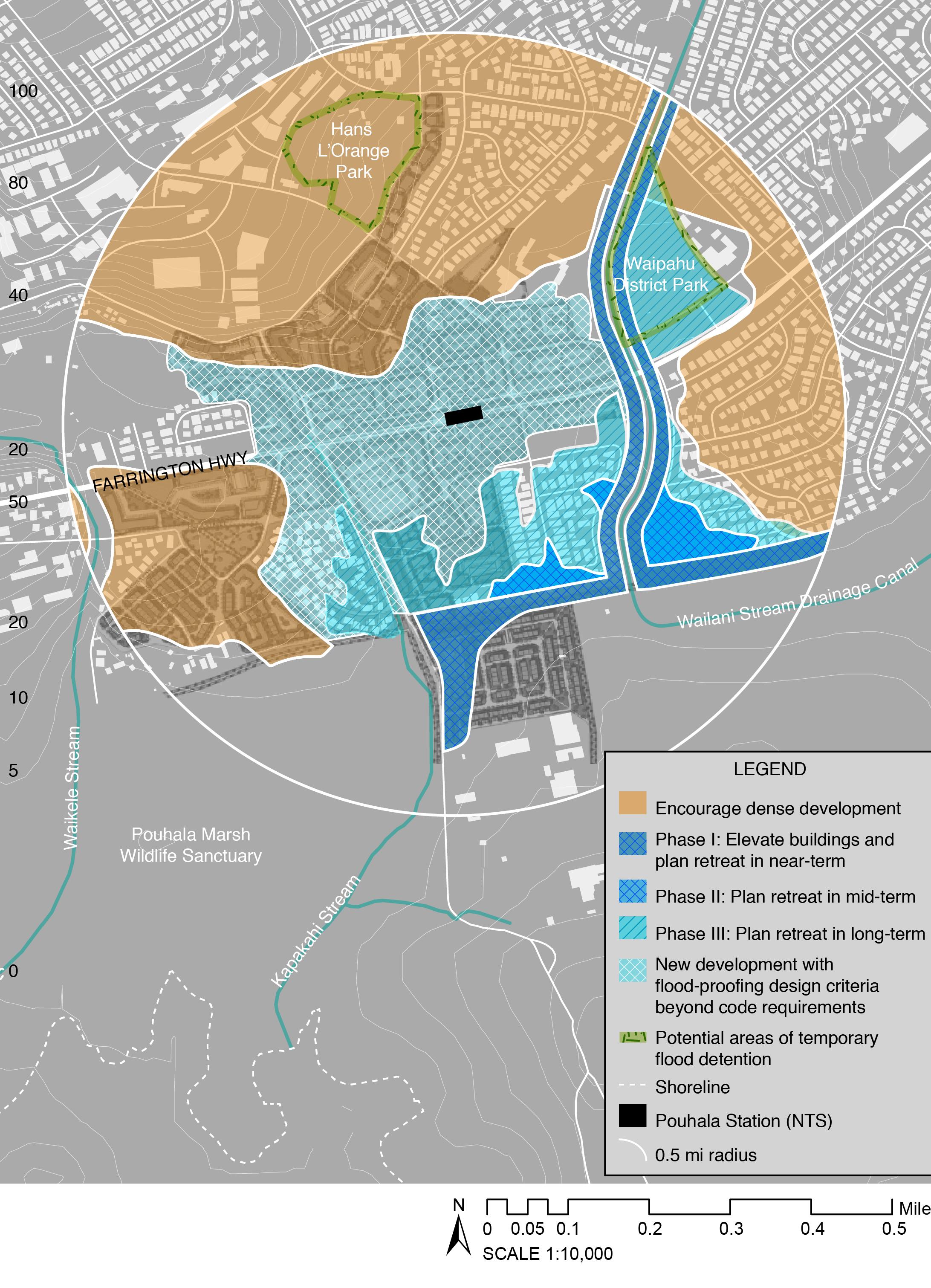
42 minute read
FLOOD RESILIENCE DESIGN CRITERIA
This section of the report focuses on potential flood mitigation design criteria for the buildings, roads, and rail in the Waipahu TOD area at three scales: planning, site, and building scale. Addressing flood mitigation at the planning scale promotes the most comprehensive flood mitigation strategies. The discussion and illustrations are site-specific, but the process and references provided are applicable to other sites.
Planning-Scale Strategies
Advertisement
Comprehensive flood hazard mitigation strategies begin with an analysis of the site, as demonstrated in the replicable process of mapping earlier in this report. Design teams should then proceed as follows:
1. Consider phased retreat of buildings and infrastructure from areas that will be chronically flooded. Prioritize areas that will flooded soonest, such as those affected by 3.2 feet of sea level rise. Consider how the future flooded areas may be used for multiple benefits, such as human recreation and habitat creation. 2. Prioritize smart urban redevelopment outside of the SLR-XA and limit development within the area, as recommended by the Hawaii Sea Level Rise Vulnerability and Adaptation Report 3. Determine if FEMA flood zones should be used as criteria for the location of new development. 4. Identify large open spaces for temporary above grade water detention and potential for below grade water detention.
The diagrammatic map (see Figure 9) illustrates some of the planning-scale recommendations above, tailored for the Waipahu site.
Retreat should be considered for existing buildings within the areas affected by 3 feet of sea level rise, which primarily consists of buildings located immediately adjacent to waterways. These properties will experience flooding from sea level rise first, and roads and infrastructure serving these areas are expected to become permanently flooded as sea levels continue to rise. In the mid and long-term, retreat should be considered from areas affected by 4 feet and 6 feet of sea level rise. Avoiding new development within these areas is consistent with the RELi standard to avoid building on sites affected by 2’6” of sea level rise. When retreat occurs, consider using the areas for multiple benefits, as described in the upcoming section on widening stream floodways and creating a multi-use berm. If retreat is not a viable strategy, the building-scale adaptation with wet or dry-flood proofing strategies discussed later in this report may be considered to prolong the use of and access to those properties.
Second, “Prioritize smart urban redevelopment outside of the SLR-XA and limit development within the area,” as recommended by the Hawaii Sea Level Rise Vulnerability and Adaptation Report. The proposed Waipahu Neighborhood Plan created in 2014 should be re-evaluated to incorporate new knowledge about sea level rise. A portion of the new development is predicted to experience flooding with four to six feet of sea level rise. For example, it is evident that the proposed new residential neighborhoods near the Waipahu Refuse Convenience Center will experience flooding. A different site should be considered for development, outside of the areas affected by sea level rise.
Third, “Determine if FEMA flood zones should be used as criteria for location of new
Pouhala Station
(E) Waipahu Refuse Convenience Center
Figure 9 Waipahu TOD Area Potential Future Flood Mitigation Strategies Diagram
development.” The diagrammatic map of potential strategies (see Figure 9) encourages dense development in the areas outside of the 100-year flood zone (1-percent-annual-chance flood event in FEMA FIRM Zone AE). This is a challenge because much of the study area is within the 100year flood zone. For reference, the RELi rating system takes an even more stringent approach, avoiding sites within a 500-year flood plain (Zone X).
Fourth, “Identify large open spaces for temporary above grade water detention, and potential for below grade water detention.” During a large rain event, riverine flooding event, or storm surge, these temporary detention areas could hold large volumes of water to reduce the flood depths at buildings, roads, and rail. Principles and precedent projects are presented in an upcoming section of this report.
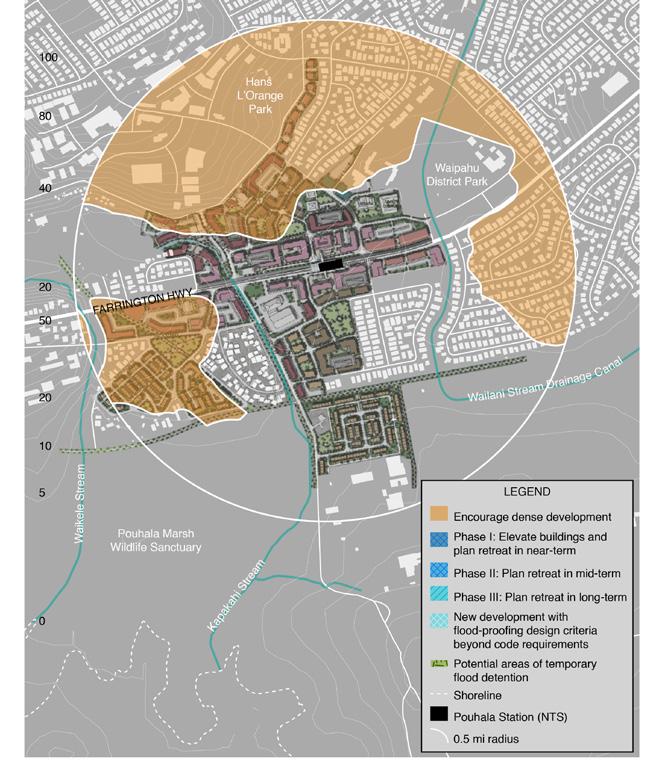
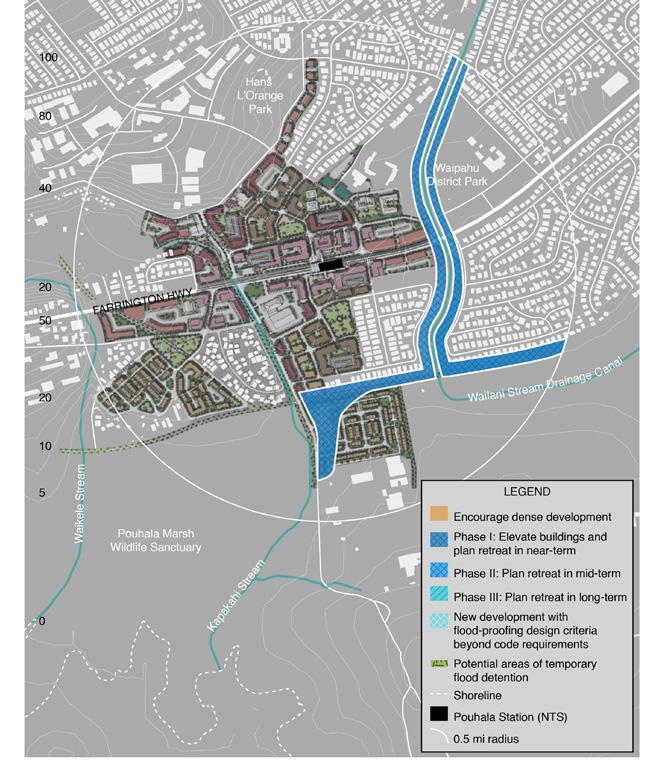
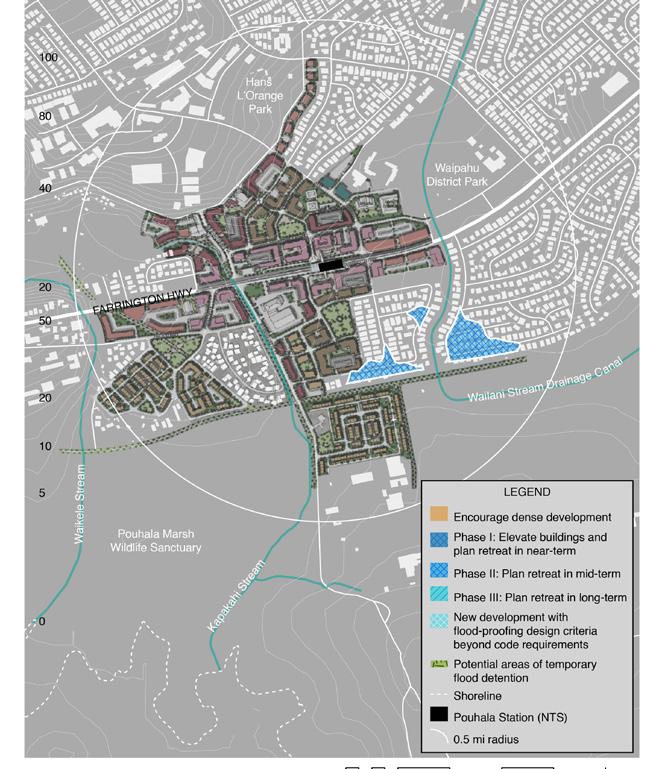
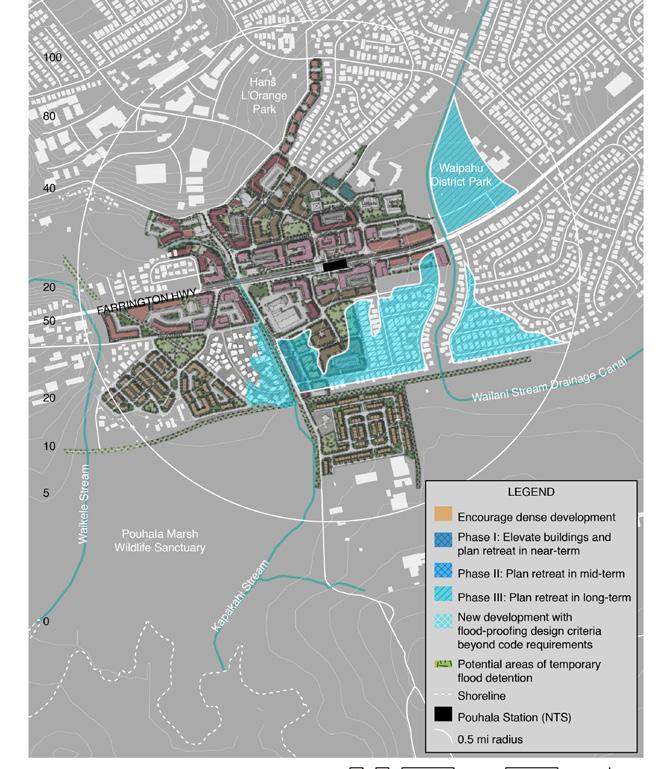
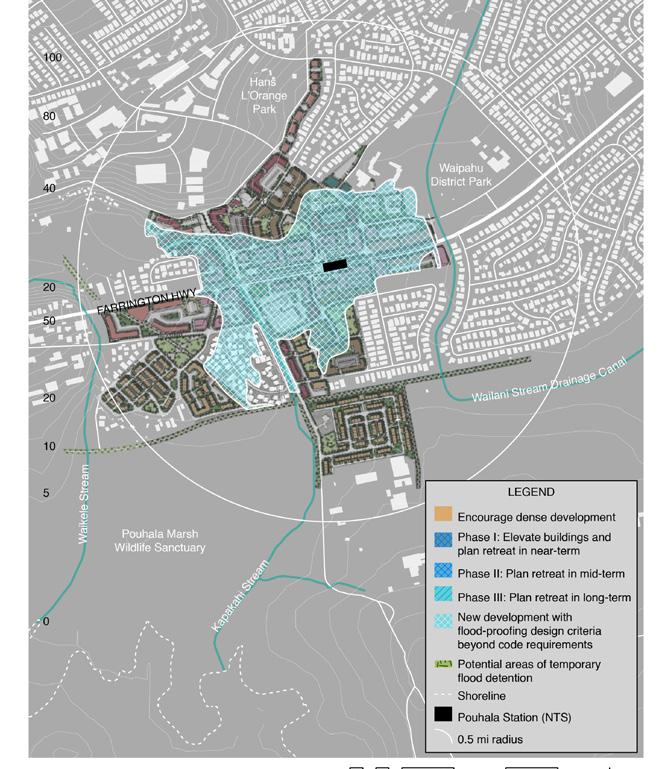
Figure 9 (continued) Waipahu TOD Area Potential Future Flood Mitigation Strategies Diagram - Breakdown
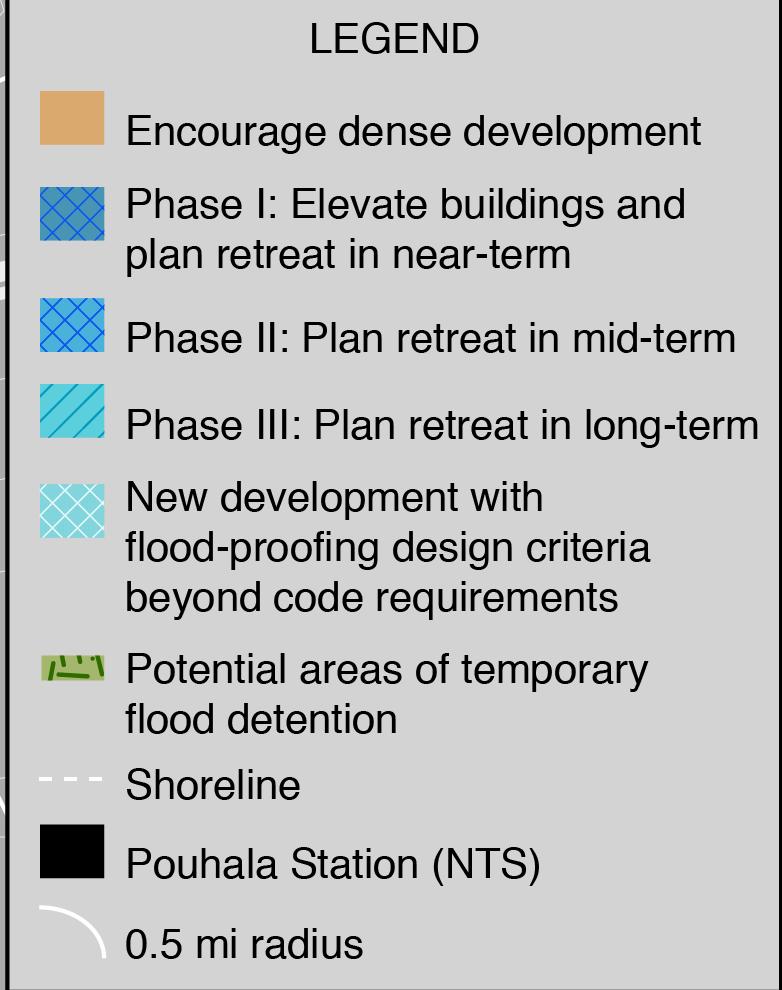
RETREAT, EXPAND FLOODWAY, CREATE MULTI-USE BERM
At the Waipahu TOD site, there is an opportunity to retreat buildings on properties adjacent to streams and rivers, many of which are affected by 3.2 feet of sea level rise, create a wider floodway, and a multi-use berm. Modification to the Wailani and Kapakahi streams widths and banks presents an opportunity for flood protection, ecological restoration, human transportation, and recreation. The potential retreat, floodway, and berm are described and illustrated in this section of the report.
Retreat
Widening the floodways and creating a multi-use berm will encroach onto private property. This presents the opportunity for managed retreat from properties that will experience flooding from sea level rise soonest.
Buildings within areas of permanent inundation by the mid to end of the century may find that floodproofing methods may no longer be an option for extending the use of the structure. Property owners may incur costly maintenance or difficulty in accessing the structure. In this case, a government-led retreat would be a strategy aligned with the Hawaii Sea Level Rise Vulnerability and Adaptation Report recommendation to “incentivize improved flood risk management.”
Some houses that can be lifted may have the ability to be relocated to a new suitable location. Other houses may be “bought out.”
Although the financial mechanisms for retreat or buy out are not the focus of the report, they are briefly mentioned to show their potential and precedent. For houses that cannot be moved, funding to aid flood victims exist such as: “reverse mortgages offered by the state and funded by general obligation bond issues wherein owners turn over homes at the end of receiving a fixed period of payments; a homeowner donation program; tax relief for businesses or families in exchange for later ownership transfer…; transferrable development rights for businesses; county, state, and federal land conservation funds; land swaps…; conservation easements that pay owners to manage their land for the environment; and others”.
One precedent for government buy-out in the aftermath of several flood events occurred in Wisconsin, when the entire business district of Soldier’s Grove experienced multiple flooding events and decided to relocate. “The relocation cost of $7.1 million was raised primarily through innovative financing. Federal funds of just under $1 million were contributed only after the town refused to accept disaster relief funds slated for standard reconstruction”.
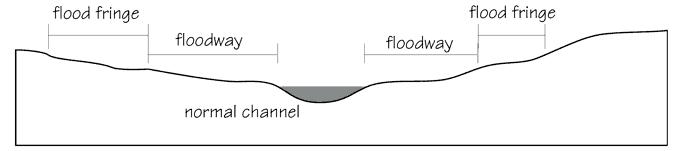
Figure 10. The section diagram above from Building Green describes the engineering terms for segments of a river basin, including the floodway.*
* Malin, Nadav. Letting Floodplains Do Their Job. Building Green. September1, 1993.https://www.buildinggreen.com/feature/letting-floodplainsdo-their-job.
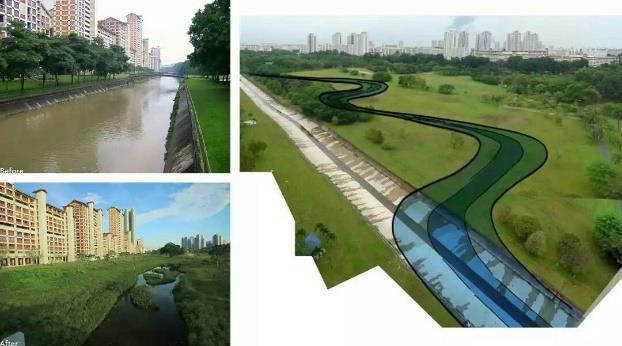
Figure 11 A stream de-channelization and park project in Singapore is a useful precedent for Wailani Stream and Kapakahi Stream in Waipahu.*
* Green, Jared. “New Park in Singapore Shows What Rivers Can Do.” The Dirt. https://dirt.asla.org/2012/07/25/new-park-in-singapore-shows-whatrivers-can-do/
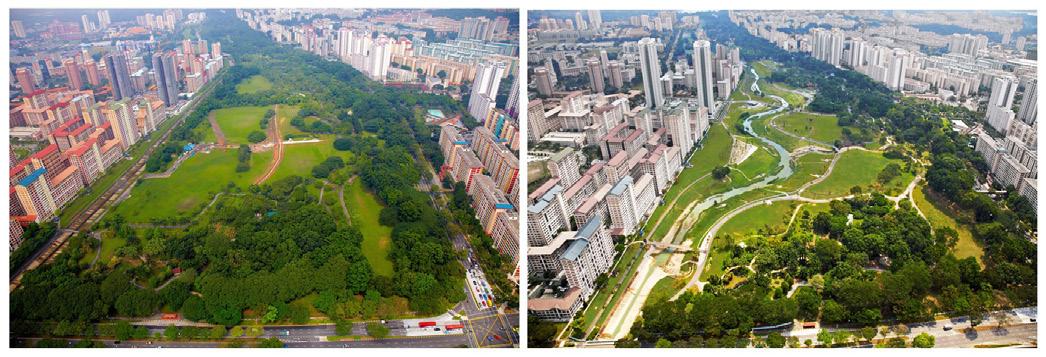
Figure 12 Before and after aerial photos of the Kallang River de-channelization.*
* Wikimedia Commons. Author Pagodashophouse. https://commons. wikimedia.org/wiki/File:Before_and_After_Aerial_View_of_Kallang_River.jpg One example of guidelines that discourage building within high flood risk areas is the resilient design rating system, RELi, which suggests that building within the 500-year flood zone or coastal zones affected by 2’-6” of SLR inundation be prohibited. Within the study area, properties higher than the 500-year flood zone are identified as Zone X, minimal flood hazard (solid orange areas in Figure 4). Much of the proposed new TOD buildings are in the 500-year flood zone, therefore, building scale flood mitigation strategies will be needed and are discussed later in this report.
Allow the Floodway to Flood
A potential strategy to prevent overtopping of stream and canal banks is to increase the width of the floodway adjacent to the waterways. Challenges include modification of the existing stream channel or bed.
Widening the flood way could increase the volume of the stream, as compared to the current channelized streams. See Figure 10 for a section diagram of the floodway, the “expanded channel in which water flows during floods.” In addition, the stream banks could be elevated in an effort to protect surrounding areas from stream overtopping. The potential expansion of the floodway and elevated bank height would require calculation of anticipated water volumes, and the conceptual idea is presented here. The expanded floodway would be a strategy aligned with the Hawaii Sea Level Rise Vulnerability and Adaptation Report recommendation to “support sustainable and resilient land use and community development.”
Potentially changing portions of Kapakahi and Wailani streams from concrete channels to natural stream banks may have potential for ecological restoration. Concrete channelized streams are problematic because faster moving water swiftly carries sediment and pollutants downstream, to the detriment of estuaries, coastal fisheries, reefs, and offshore species. The channelized stream “offers no habitat to native species” and encourages development within adjacent flood plains. Future modified stream banks could be modified to restore the natural ecology. “Ideally, streams in Hawaii should have natural beds and banks. Cool, clear water should meander through features such as pools and riffles that also provide habitat to native species. Naturally overhanging vegetation should provide shade from the hot subtropical sun for the indigenous ecology.”
In natural floodplain areas, streams and rivers are allowed to overflow. This process has numerous benefits for the surrounding habitat including benefits to plants, invertebrates, birds, and animals that live on the banks. This also improves habitats, natural erosion and deposition, and potential removal of fine silt, which increases water clarity, in riparian and
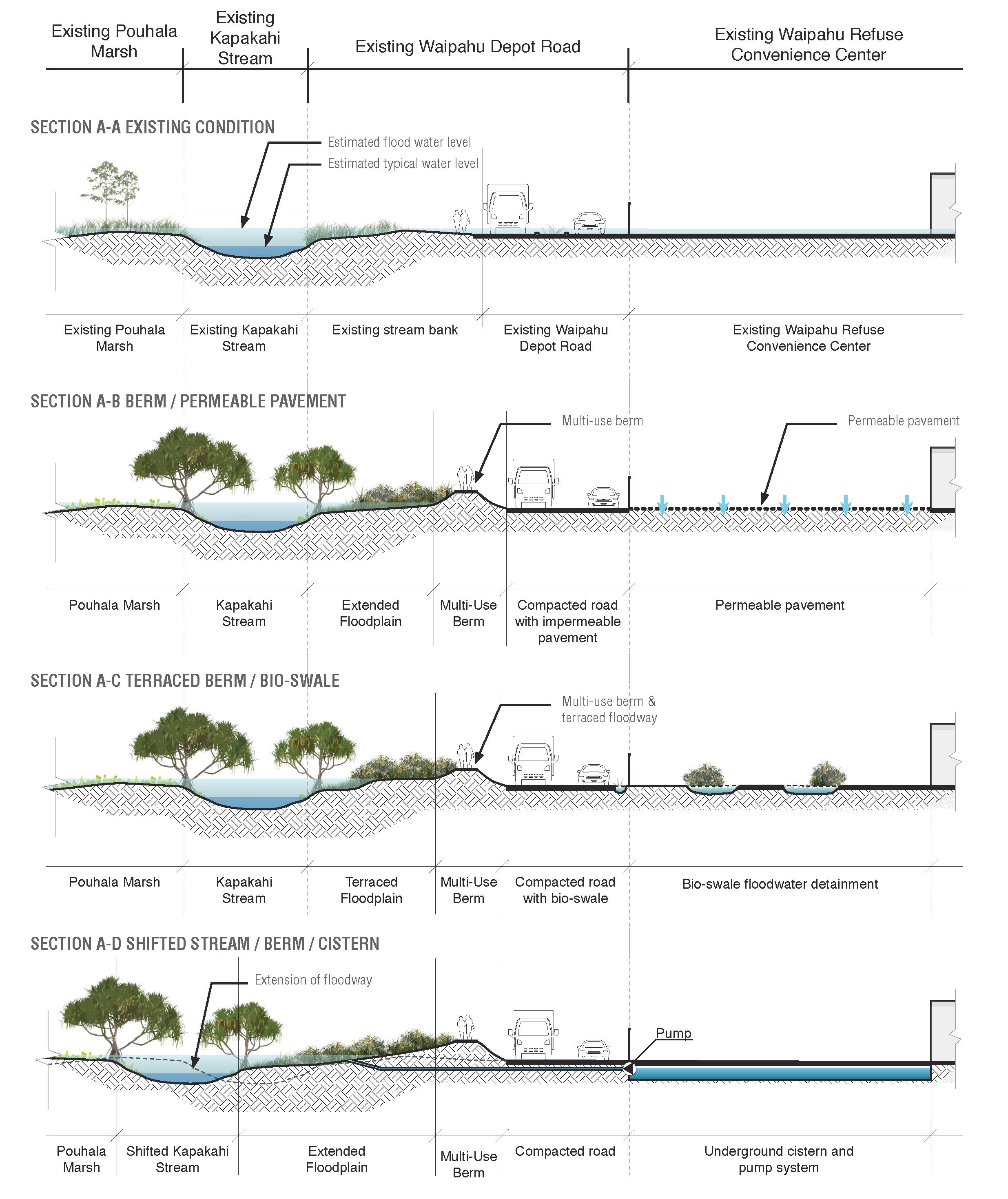
Figure 13. Proposed changes to the existing Kapakahi Stream along Waipahu Depot Road include features that serve the natural ecology from Pouhala Marsh as well as pedestrians.
Figure 14 Penn/Olin’s design for a multi-use levee for the Rebuild by Design competition. *
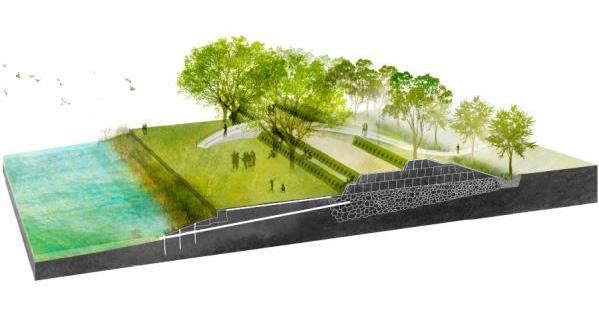
* PennDesign/Olin. “Hunts Point Lifelines”. Rebuild by Design, http:// www.rebuildbydesign.org/data/files/677.pdf
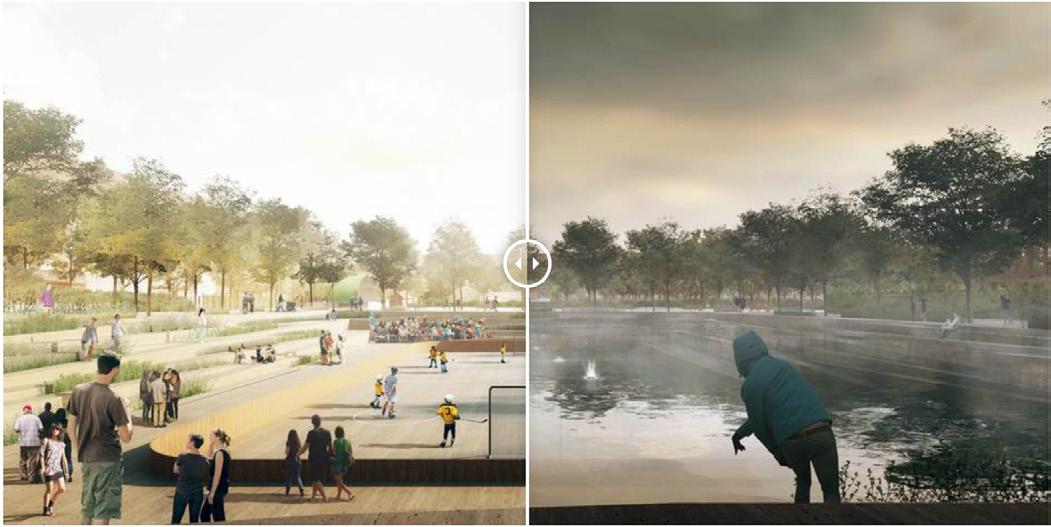
Figure 15 The competition winning entry by Third Nature for Enghaveparken shows a sunken space used for recreational public gathering when dry, and to hold stormwater during heavy rains. Image credit: Third Nature.*
* Tredje Natur. “Enghaveparken Now”. http://tredjenatur.dk/en/portfolio/ enghaveparken-now/
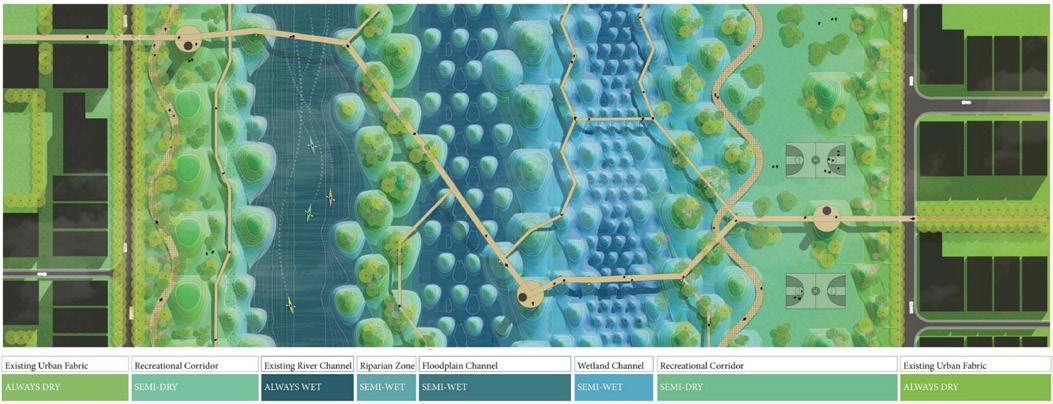
Figure 16 Analysis image from MIT’s “Strategies for Urban Stormwater Wetlands” shows a portion of a redesigned stream corridor utilizing optimized topographical elements that support human and ecological functions.*
* MIT Center for Advanced Urbanism, Nepf, Heidi, et al. “Design Guidelines for Urban Stormwater Wetlands.” MIT, http://lcau.mit.edu/project/ strategies-urban-stormwater-wetlands. Fall 2016. floodplain zones.
One challenge to modifying the existing Kapakahi and Wailani streams include relocation of existing buildings adjacent to the streams, which primarily consist of detached homes. The other challenge is the engineering and logistical planning required, which is addressed effectively in the following precedent project.
A built precedent project shows past success dechannelizing a stream and designating a flood plain adjacent to the stream. In Singapore, 1.7 miles of the previously channelized Kallang River was revived into a 1.8 mile, natural meandering waterway, in order to reduce peak flooding while increasing water security by slowing and collecting storm water runoff. “In a feat of sequenced engineering, [landscape architect Herbert] Dreiseitl managed to re-engineer soils, add bio-engineered plant systems along with trees, break up the existing concrete channel and reuse the rubble to stabilize the entire system — all while the river was still running” (see Figure 11). In addition to reducing dangerous flooding, biodiversity increased, adjacent property values rose, and people in the city have much-desired access to nature. This is an excellent example of public infrastructure that also serves ecological and human recreation purposes.
The canal resembles the channelized stream in Waipahu between to Waipahu District Park and the State owned land parcels. One may envision the stream de-channelized, with planned temporary storm water flood detention in Waipahu District Park to prevent or reduce downstream flooding near the new rail stop.
Even at the federal level, the Army Corps of Engineers has begun to shift their focus away from grey-infrastructure elements. In Massachusetts, a plan for damming the Charles River basin was altered from a $100 million plan to install greyinfrastructure elements such as dams and channelizing the river, to a $10 million plan to protect upstream wetlands. “The money was used to buy acreage and easements preventing landowners from making alterations to the wetlands. According to Corps studies, this approach has performed quite well at preventing floods, and a large area of wetlands in New England’s most densely populated river basin is protected from development.”
Section drawings later in this report illustrate widening the floodway and adding a vegetated multi-use berm concept at the Waipahu TOD study area (see Figure 43).
Vegetated Multi-Use Berm
After increasing the width of the floodway adjacent to the Kapakahi and Wailani streams, another potential strategy to minimize overtopping of the stream and canal banks is to
increase the height/elevation of the stream banks and create a vegetated multi-use berm. The vegetated multi-use berm is primarily used to reduce stream overtopping onto surrounding properties. The term “multi-use” refers to its capacity for varying uses including storm water management, ecological habitat and corridor, and recreational use properties. Terracing along the berm could allow for recreational paths to be installed for biking and walking, nature walk with signage, and for public access to the waterway (see Figure 13). The streams could serve as a mauka to makai, mountains to ocean, contiguous green way, habitat corridor, and alternative transportation way.
The multi-use berm diagram references the RELi set of resilient design guidelines to identify and visualize storm water management goals for Waipahu. The section Hazard Preparedness (PH) addresses floodplain functions in PolyRequirement 2: Minimum Protection for Prime Habitat & Floodplain Functions. The sub-heading titled Preserve Floodplain Functions references the Envision NW1.3 document that suggests “four main ways to improve ecosystem functions: 1. Maintain or enhance hydrologic connection, 2. Maintain or enhance water quality, 3. Maintain or enhance habitats, and 4. Maintain or enhance sediment transport.” It also suggests to “avoid or mitigate impacts, maintain infiltration and water quality, enhance riparian and aquatic habitat, and enhance connectivity and sediment transport.” Another precedent project, is a winning Rebuild By Design competition entry called, “Hunts Point Lifelines” by PennDesign/Olin et. al. (see figure 14). The process is a useful precedent because of the analysis of flood depths and integration of waterfront functions to create a floodable green space along the water’s edge. A ¬part of the multi-faceted design features a levee built adjacent to a river. It features walkable space and access to the water on dry days and a protected walkway during flooding events (see Figure 12).
Large, Above-Grade Water Detention
When re-developing the half-mile radius surrounding the new rail station, there is an opportunity to utilize large open spaces for multiple benefits, including stormwater detention, flood mitigation, recreation, and habitat creation. Parks or plazas are ideal amphibious spaces – serving as public space during dry times and floodable space during rainy times to store, infiltrate, and/or slowly release significant volumes stormwater to municipal systems. One may consider redesigning Waipahu’s major green spaces to better receive and detain stormwater runoff from surrounding areas. Areas of interest include Hans L’Orange Park, Waipahu District Park, Hawaii’s Plantation Village, and Ted Makalena Golf Course (see Figure 9 for map). The following local, national, and international precedent projects provide excellent examples for Waipahu of
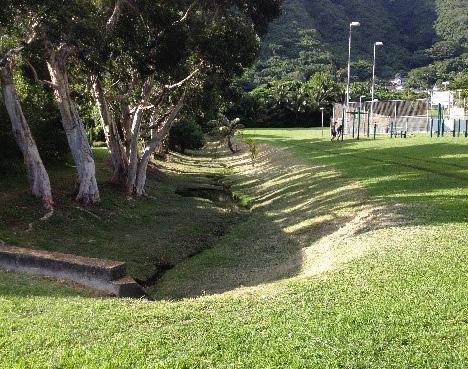
Figure 17 Green space with swale in Honolulu, HI. Photo credit: W. Meguro.
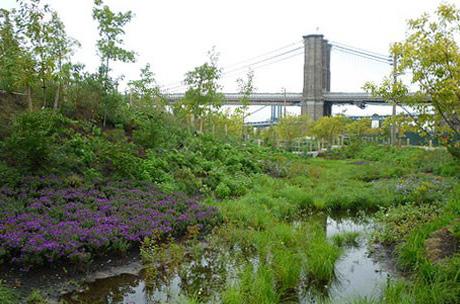
Figure 18 Floodable landscape for stormwater management in Brooklyn Bridge Park, NY. Photo credit: W. Meguro
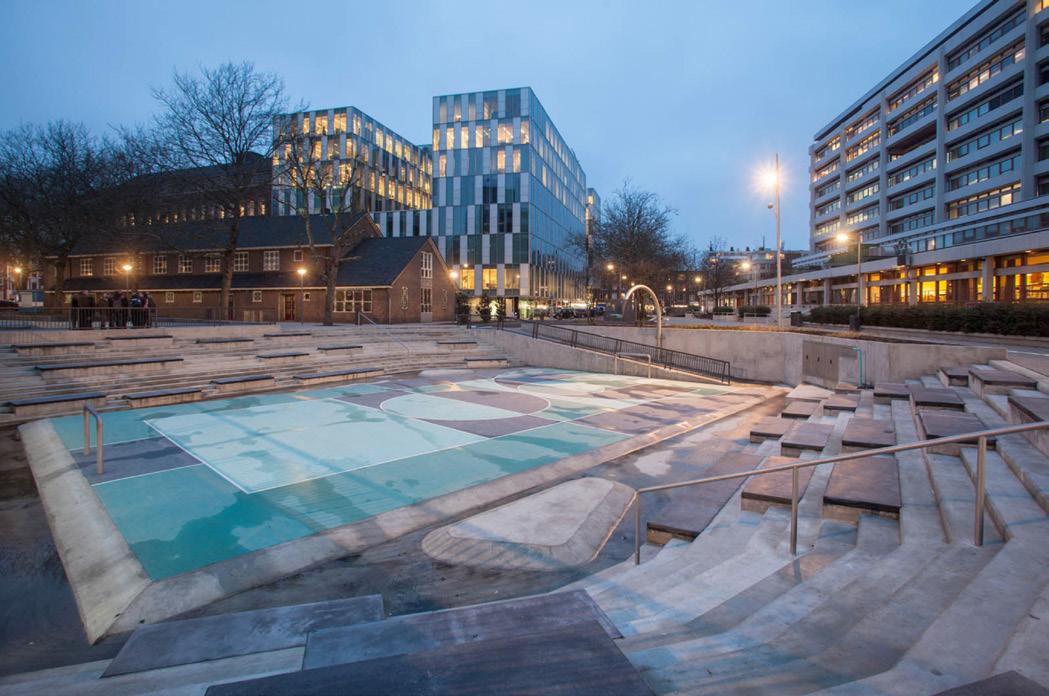
Figure 19. Floodable urban space, Water Square, in Rotterdam, NL Square by De Urbanisten.*
* “Water Square Benthemplein.” De Urbanisten, http://www.urbanisten. nl/wp/?portfolio=waterplein-benthemplein
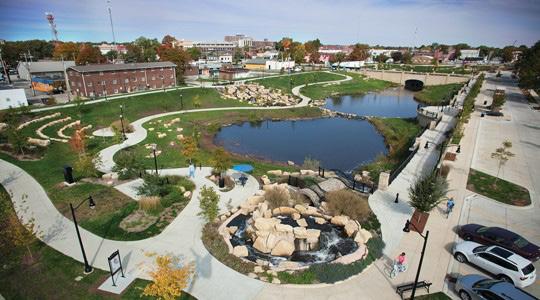
Figure 20. The basin project in Illinois “provides 100-year flood protection by containing the 15 million gallons of stormwater generated during a 100-year storm event.”* * Landscape Architecture Foundation. “Landscape Performance Series: Boneyard Creek Restoration: Scott Park and the Second Street Detention Basin”. https://www.landscapeperformance.org/case-study-briefs/boneyardcreek-restoration. infrastructure with multiple protective, ecological, and social benefits.
Copenhagen’s Enghaveparken public park is an example of a multi-functional park that is designed to accommodate community gathering during dry seasons, and water during rainy seasons. The park can hold 24,000 square meters of storm-water runoff from nearby neighborhoods and an additional 11,500 cubic meters underground, and pumps are required for restoring recreational spaces to non-flood conditions. Along three of its lowest edges, are levees that serve as the park’s main storm water management element and also serves as seating and provides activities “such as a bulkhead and small openings for play.” Currently under construction, and estimated to be completed in 2019, the design for the park was envisioned through a design competition and won by the team Third Nature (see Figure 15). The design aims to maintain the original structure of the park and visibly manage stormwater by using the park as a retention basin and directing remaining water to a closed underground reservoir.
The following study method is a useful precedent for iterative design, analysis, and resulting guidelines for urban stormwater wetlands in Waipahu. The MIT Center for Advanced Urbanism study considers regional-scale digital simulation to design engineered green spaces, to the “capture and purify stormwater while delivering ecosystem and recreational benefits.” Researchers at MIT released the “Design Guidelines for Urban Stormwater Wetlands” report in 2018 a study on maximizing efficiency of wetlands through digital modeling and simulation for Los Angeles, California and Houston, Texas sites. A large portion of this study looks at the engineered approach to “optimal topographic forms” (see Figure 16) to minimize water pollution. However, their guidelines also encourage the combination of three elements: new habitats, public programming, and recreation to create a “unique palette for design” which results in “programming opportunities for people and nature”.
The following photos demonstrate public green spaces that support human recreation while directing stormwater runoff to slow and infiltrate in low-lying areas. There is a bioswale adjacent to playing fields at Manoa District Park in Honolulu, HI (see Figure 17). There is a constructed wetland with pedestrian bridges (not shown) for stormwater management at Brooklyn Bridge Park in New York City, NY (see Figure 18).
Floodable public spaces comprised of hardscape may be more appropriate than a green space in some urban contexts. Water Square Benthemplein in Rotterdam, NL was designed and constructed by De Urbanisten for the Rotterdam Climate
Initiative and demonstrates how a municipality can use infrastructure to serve multiple benefits (see Figure 19). The square “combines water storage with the improvement of the quality of urban public space.” The square is used for recreation, in dry weather. In wet weather, gutters direct water to three basins in a “dramatically gush the rain water visibly onto the square.” Stormwater either “flows into an underground infiltration devices” or is detained and slowly released to the municipal combined sewer system. The description of the project time line is helpful in understanding the steps and schedule relevant for similar studies and potential application at the Waipahu Transit Oriented Development (TOD) area. The Water Square project moved from research in 2005, to policy in 2007, to a pilot study in 2008, to a publication in 2010, to design in 2011-2012, to opening in 2013.
The Second Street Basin project is a small portion of the larger Boneyard Creek Restoration effort in Champaign, Illinois. The once channelized stream resembled the Waipahu Drainage Canal that runs through the study site. Efforts to de-channelize the stream pushed designers to increase the allowable floodplain and make the stream accessible to the public. Completed in 2010, the final design is capable of containing 15 million gallons of storm-water caused by a 100-year storm (see Figure 20). Like the soil of Waipahu, the soil within the Second Street Basin parcel has a high clay content. To facilitate better drainage into the ground, pea gravel and installation of drains were added to the design to also aid vegetation survival. The entirety of the Boneyard Creek restoration effort connects large green spaces to open up floodplains that total 47 acres of run-off management and 5 acres of recreational use during non-flood conditions.
Large, Below-Grade Water Detention
Utilizing large underground cisterns to temporarily store large quantities of stormwater runoff, riverine flooding, or storm surge water may mitigate flooding in the Waipahu TOD Water would be pumped out and the space cleaned after a flood event. Section drawings illustrating potential new underground cisterns are illustrated in Section A-D.
One of the world’s most well-known examples of underground cisterns is Japan’s G-Cans project. The system consists of five silos, each 65 meters deep and 32 meters in diameter, and 6.5 kilometers of connecting tunnels that lead to a 25.4 meter high by 177 meter long “Underground Temple.” Tokyo’s G-Cans have a capacity to store a 200-year flood event below city streets (see Figure 21 and Figure 22). After a storm event, large pumps actively pump the floodwater into the Edogawa River.
Another example of below-grade large volume water storage is a museum carpark in Rotterdam (see Figure 23). The car park
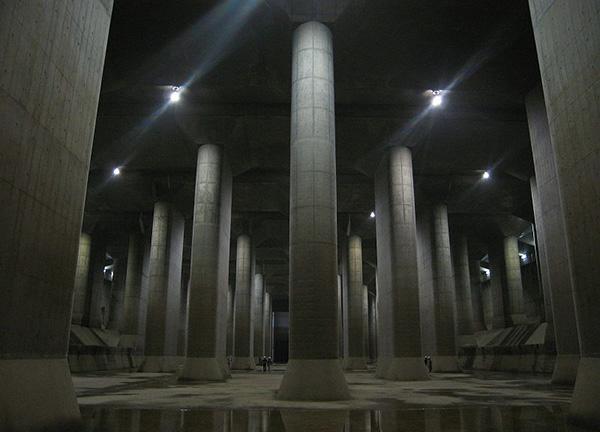
Figure 21. Water storage tank in Japan’s G-Cans project.* * Water Technology, “G-Cans Project, Kasukabe, Saitama, Greater Tokyo Area” https://www.water-technology.net/projects/g-cans-project-tokyojapan/.
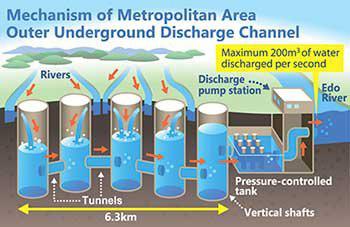
Figure 22. Diagram of Japan’s G-Cans Project. *
* Hill, Ed. “G-Cans Project, Tokyo – Japan’s $2.6 Billion Flood Tunnel.” Flood List, http://floodlist.com/protection/g-cans-project-tokyo-flood-tunnel
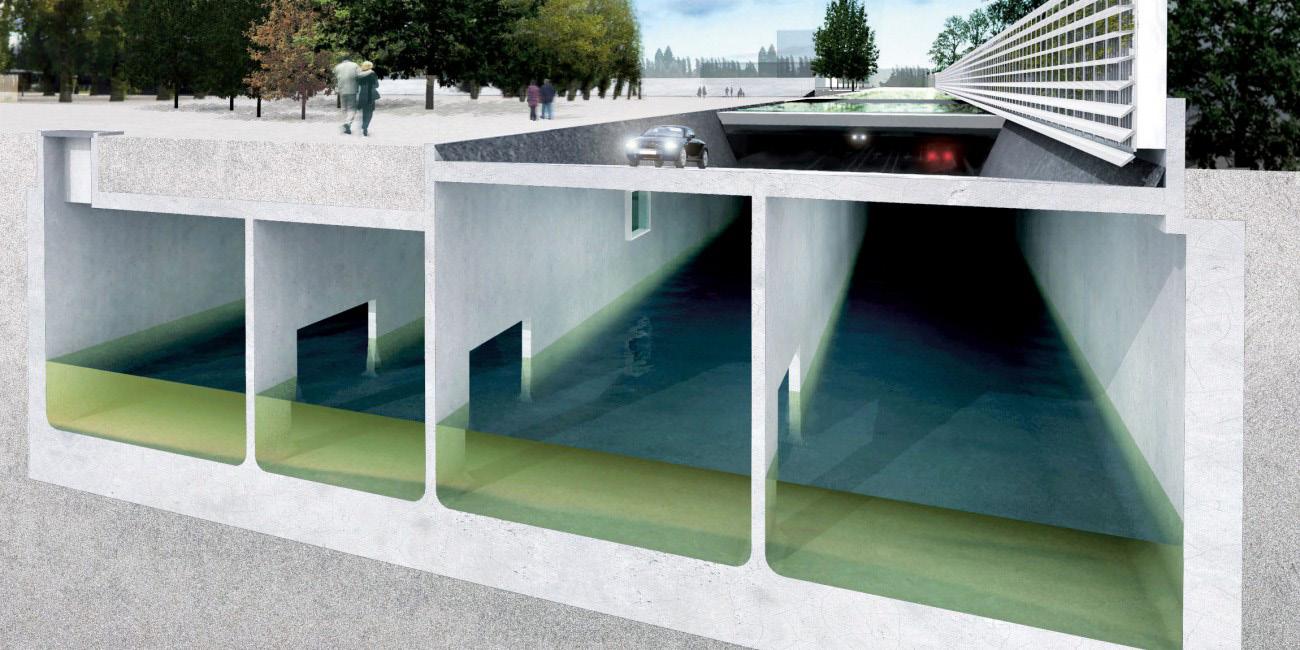
Figure 23 MuseumPark Car Park water storage in Rotterdam, Netherlands*
* Rotterdam Climate Initiative. “Underground Water Storage Facility with a Capacity of 10 million litres” http://www.rotterdamclimateinitiative.nl/ uk/news/underground-water-storage-facility-with-a-capacity-of-10-millionlitres?news_id=743&p=5
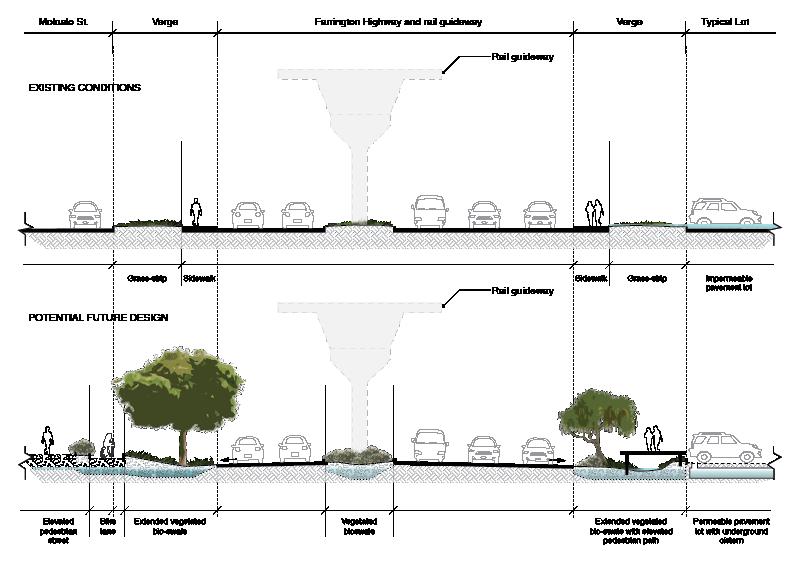
Section Drawing A Existing Conditions and Proposed Future Design at the rail guideway/Farrington Hwy.
accommodates 1,150 cars and also houses “one of the largest underground water reservoirs in the Netherlands, a reservoir with a capacity of 10,000 m3” (over 2.64 million gallons).
Site-Scale Strategies
At the scale of a building site in the Waipahu TOD area, on-site storm water infiltration and detention should be incorporated to mitigate flooding of the built environment.
Future design teams may refer to existing, well-developed stormwater and green infrastructure best management practices in the following resources.
• Honolulu Stormwater Best Management Practices (BMP) Guide for New and Redevelopment • US Green Building Council Leadership in Energy and Environmental Design (USGBC LEED) for New Construction Sustainable Sites credit on Rainwater Management • The separate section of this Waipahu TOD report on green infrastructure by author Dr. Suwan Shen of the University of Hawaii Department of Urban and Regional Planning
The above resources include design requirements or criteria that limit surface runoff into neighboring properties, maintain or decrease peak runoff volume into municipal stormwater systems, and maintain or improve runoff water quality. For example, future building and site design teams should set targets for stormwater management, such as the LEED criteria to, “In a manner best replicating natural site hydrology processes, manage on site the runoff from the developed site for the 95th [or 98th] percentile of regional or local rainfall events using low-impact development (LID) and green infrastructure.” Stormwater management requirements specific to Waipahu TOD area could be incorporated into the area’s new and re-development requirements.
This section of the report illustrates, at a proof of concept level, how several green infrastructure stormwater management strategies could be integrated into the Waipahu TOD study area, including bioswales and permeable pavement. When designing on-site stormwater infiltration, clay-based soils with low permeability indicate some areas may require installation of a more permeable gravel bed below grade to facilitate drainage. Future design teams would need to determine if the top of the below-grade water table is below the potential the gravel storage bed.
Bioswales and detention areas
A bioswale is a “stormwater control feature that uses a combination of an engineered basin, soils, and vegetation to slow and detain stormwater, increase groundwater recharge,
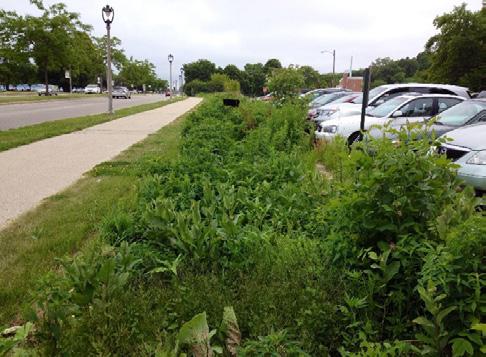
Figure 24. Bioswale between the roadway, sidewalk, and parking lot in Milwaukee, WI. Photo credit: W. Meguro
Figure 26. Vegetated stormwater detention basin in Seattle, WA. Photo credit: W. Meguro
Figure 28. The sidewalk, bioswale, bicycle, filter strip, and road in Long Island, NY is a useful precedent for Waipahu TOD multi-modal transportation. Photo credit: W. Meguro Figure 25. Bioswale with curb cuts adjacent to urban buildings, sidewalk, and road in Milwaukee, WI. Photo credit: W. Meguro
Figure 27. Bioswale, curb, cut, and pervious asphalt at the Center for Sustainable Landscapes in Pittsburgh, PA. Photo credit: W. Meguro
Figure 29. HOLD, by DLANDSTUDIO directs stormwater runoff from downspouts to gravel sedimentation basins and remediative plants.*
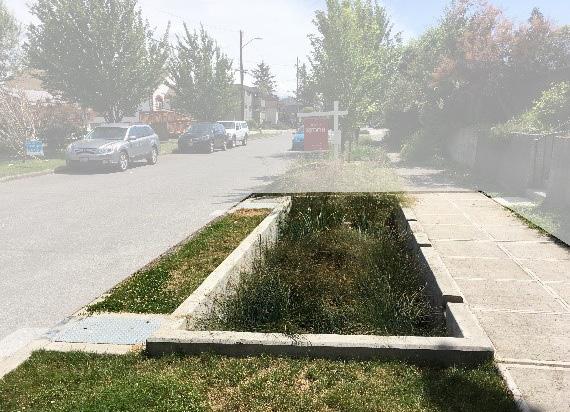
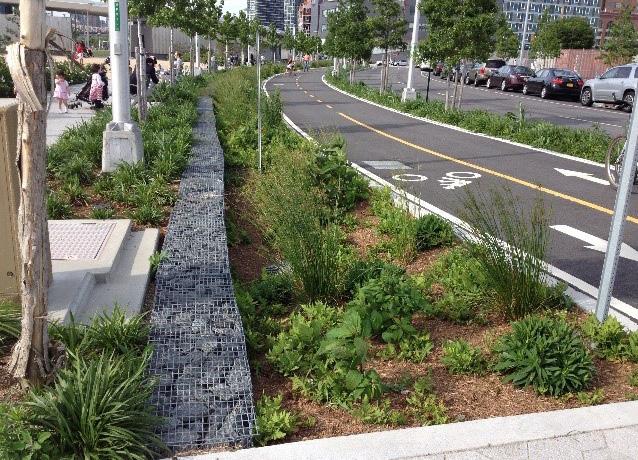
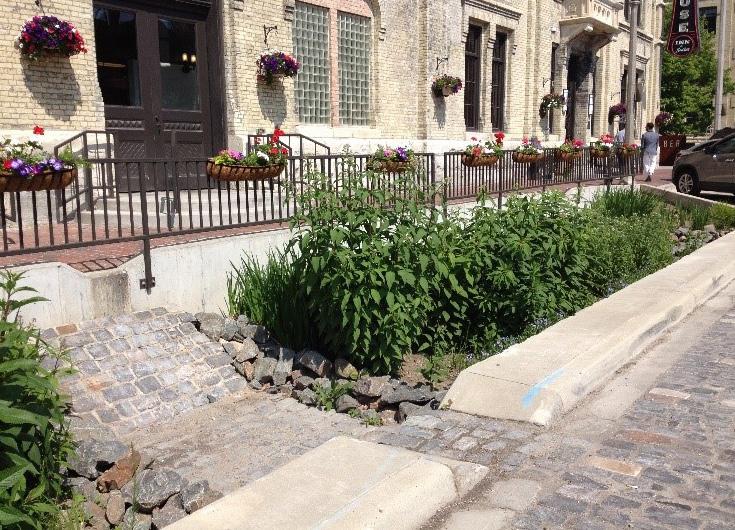
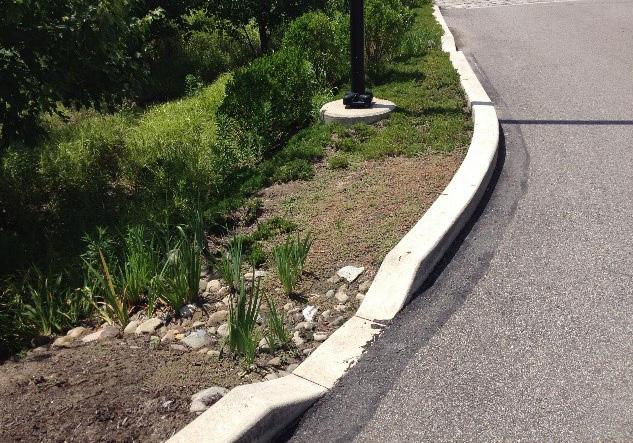
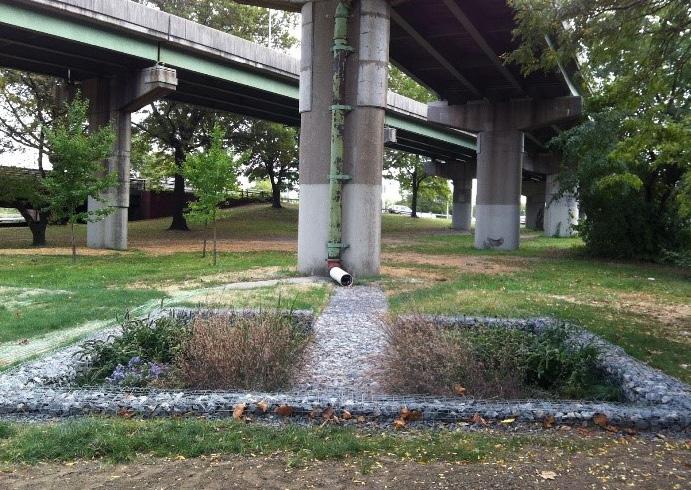
* DLANDSTUDIO, et al. Hold System Flushing Bay Regional Plan Association.
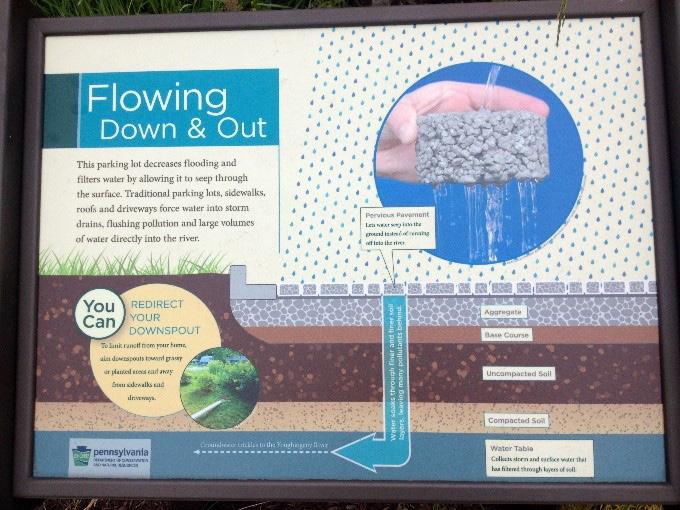
Figure 30. Illustration section drawing of permeable paving stormwater infiltration by the Pennsylvania Department of Conservation and Natural Resources. Photo credit: W. Meguro
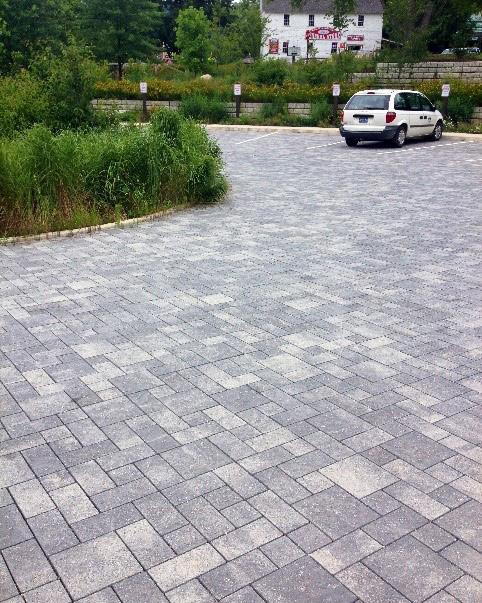
Figure 31. Permeable paving in parking lot in Pennsylvania. Photo credit: W. Meguro Figure 32. Permeable grass-crete in parking lot in Honolulu, HI. Photo credit: W. Meguro and reduce peak stormwater runoff.” Bioswales filter sediment and pollution that flow off streets before they enter storm drains, streams, and ultimately the ocean. The following “Section Drawing A” illustrates conceptual incorporation of bioswales immediately adjacent to a roadway and in a parking lot and illustrates potential vegetated bioswale below the elevated rail guideway below structural columns. Section A also illustrates how new bioswales (with gravel beds) adjacent to the roads can filter and absorb runoff from the road. A relocated sidewalk or elevated walkway enhances the pedestrian experience by providing shade trees and a buffer from Farrington Highway. On the right side of Section A the existing impermeable surface parking lots is redesigned to include permeable paving and cisterns for water detention below grade for slow release to the storm sewer.
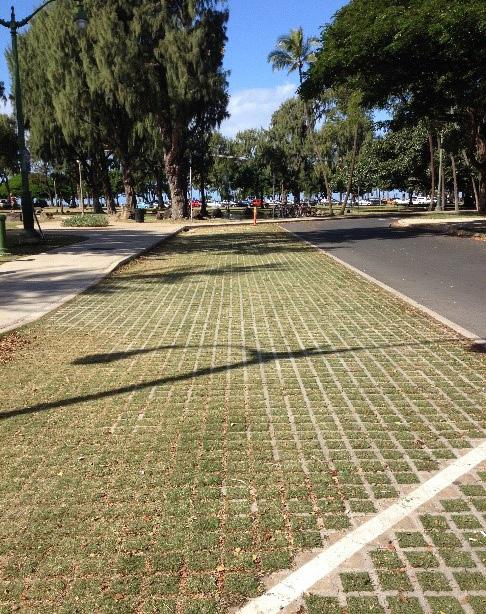
Consideration of creating interspersed containment spaces over large surfaces such as parking lots and plazas may capture urban run-off and lower the total volume of storm-water that may affect downstream areas. In areas of extensive flooding such as the residential neighborhood north of the Ted Makalena Golf Course, bio-retention using permeable paving atop a gravel storage bed may store storm water runoff and flooding from riverine overtopping. There are many areas with light vehicular traffic, such as residential driveways and residential road shoulders used for walking and parallel parking.
Several images of precedent projects (right) demonstrate how bioswales are integrated into roadway and elevated highway designs, as part of a complete street that accommodates pedestrians, bicyclists, and parking. Figure 24 shows a heavily vegetated bioswale at a lower elevation than the surrounding the large roadway, sidewalk, and parking lot in Milwaukee, WI. Figure 25 shows a bioswale with curb cuts integrated in a dense urban area between buildings, sidewalk, and road in Milwaukee, WI. Figure 26 shows a bioretention basin approximately four feet deep with curb cuts and overflow outlet to storm sewer in a detached house residential community in Seattle, WA. Figure 27 shows a bioswale, curb, cut, and pervious asphalt at the Center for Sustainable Landscapes in Pittsburgh, PA. Figure 28 shows the sidewalk, bioswale, bicycle lanes, filter strip, and road in Long Island, NY, which is a useful precedent for Waipahu TOD to plan for pedestrians, bicyclists, cars, and stormwater. Figure 29 shows a project named “HOLD” by DLANDSTUDIO direct stormwater downspouts runoff to gravel sedimentation basins and remediative plants, and is a useful precedent for the structural columns for the elevated rail guideway in Waipahu.
Another relevant precedent for the Waipahu TOD area is the method used to identify the best performing stormwater management designs to guide future development produced
through a municipal study in Wisconsin. The city of La Crosse, Wisconsin published a study, “Using Green Infrastructure to Mitigate Flooding in La Crosse, WI: An Assessment of Climate Change Impacts and System Wide Benefits” in which they utilized the EPA’s Storm Water Management Model to test the effectiveness of permeable pavement and bio-retention areas under different storm scenarios. Their study concluded that a deeper storage gravel bed in bio-retention areas and wider use of permeable streets mitigate flooding from increasingly intense storm events. Future Waipahu TOD design team members may provide design guidance in a format similar to the La Crosse study’s resulting clear, tangible design guidance below.
• Permeable pavement with a 4 foot gravel storage bed was determined to be the highest performing system in the basin that can be used to meet the City’s objectives for flood control. • Implementing permeable pavement on 25 percent of the potential street area represents the knee-of-the-curve solution to mitigate flooding from the 3-month, 2-hour storm event. • Implementing permeable pavement on 75 percent of the potential street represents the knee-of-the-curve solution to mitigate flooding from the 10-year, 2-hour storm event.
Permeable Pavement
Permeable pavement is “a paved surface with a higher than normal percentage of air voids to allow water to pass through it and infiltrate into the subsoil”. A section drawing on signage by the Pennsylvania Department of Transportation illustrates the layers below permeable paving including a base course, uncompacted soil, compacted soil, and finally the water table (see Figure 30). Materials such as grass pavers or permeable pavers may be used in lieu of typical asphalt (see Figure 31 and Figure 32).
When re-developing the Waipahu TOD area, large impermeable areas such as less-trafficked roads, parking lots, residential street shoulders, sidewalks, driveways, alleys, bike paths, patios, and public plazas should be evaluated for permeable paving. In Waipahu’s residential neighborhoods, there are many areas with light vehicular traffic, such as residential driveways and residential road shoulders used for walking and parallel parking.
Because permeable paving may be more susceptible to compression and its air voids are necessary to allow water to pass through it, only light vehicular traffic areas are recommended.
As mentioned earlier, anecdotes of soils with high clay content and low water permeability, as well as the location of the water
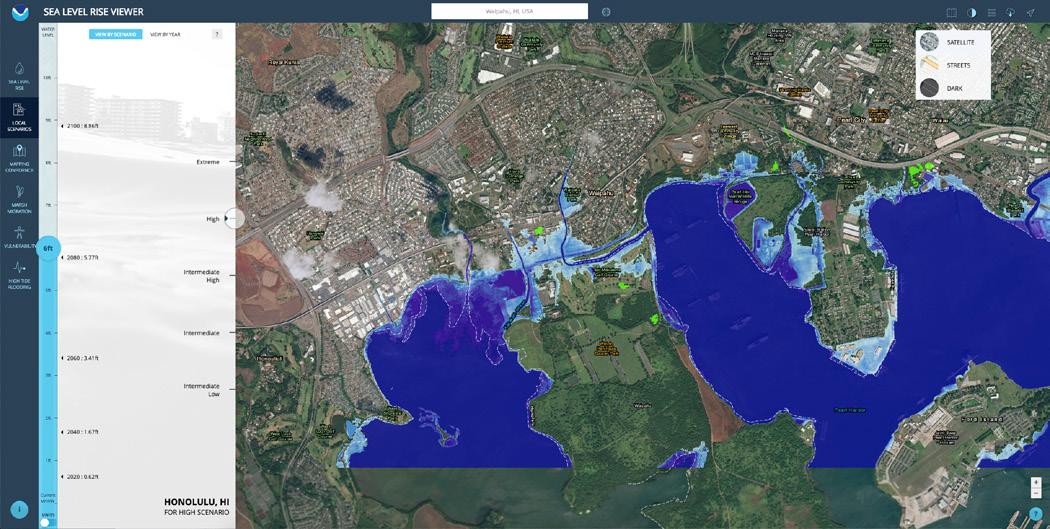
Figure 33. NOAA Sea Level Rise Viewer year 2080 High Scenario estimates 5.77 feet of sea level rise, and the map illustrates anticipated flooded areas in light blue with 6 feet of sea level rise.*
* NOAA, https://coast.noaa.gov/digitalcoast/tools/slr.html
Sea level rise
(for year 2080 high scenario from NOAA)
+ Storm surge
(from
NOAA
SLOSH model)
+ Base
Flood
Elevation
(from
FEMA
FIRM, 1 in 100 year)
= Total water level
per LEED credit calculation
5.7 feet, water depth on land varies and is qualitatively expressed with a blue gradient __ feet ___ feet above local tidal datum ___ feet above local tidal datum
Figure 34 LEED Credit Total Water Level Calculation Note: Values would need to be determined for each specific site.
Total water level
per LEED credit calculation
__ feet above local tidal datum - Elevation at the building site
__ feet above local tidal datum
= Elevation above grade for habitable floors
__ feet above grade at building site
Figure 35 Design Flood Elevation Example Note: Values would need to be determined for each specific site. table would need to be confirmed. Dredging and installing a gravel storage bed below new permeable paving in order to create space for water infiltration may address low permeability. Sub-surface perforated pipes may also slowly transport water to stormwater sewers or retention basins.
Building-Scale Flood Strategies
This section summarizes resources for a building owner to: estimate the flood related hazards on the building’s land parcel; consider the BFE and potential design flood elevation; consider wet floodproofing strategies; and consider dry floodproofing strategies. This section includes design criteria that go beyond current requirements of the Revised Ordinances of Honolulu (ROH) for the voluntary use by building design teams. In addition, the City of Honolulu or State of Hawaii may consider developing incentives or requirements using the strategies and references in this section.
SITE-SPECIFIC ASSESSMENT OF NATURAL HAZARDS
At the beginning of a building and site design, the site analysis includes an assessment of site-specific flood-related hazards. This section summarizes flood-related portions of emerging voluntary resilient building design rating systems, which may be used by future design teams to conduct a natural hazard assessment. Working with a hydrological engineer is necessary for design calculations, but the following guidance is useful when starting the design process.
The USGBC LEED pilot credits on Resilient Design were updated and re-released in early 2019, while this report was being finished. Design teams should consider using the LEED pilot credit, “IPpc98 Assessment and Planning for Resilience”, which requires teams to consider and plan for their site’s vulnerability to natural hazards and changing climate conditions. The required Hazard Assessment includes the following specific requirement, “Sea Level Rise and Storm Surge for 2022 and beyond should use the NOAA 2012 Sea Level Rise year 2080 High Scenario combined with a 1 in 100year floodplain (Extreme Flood) to determine water levels for planning purposes.”
Regarding SLR, for Honolulu, the NOAA Sea Level Rise viewer estimates 5.77 feet of SLR in the 2080 High Scenario (see Figure 33). As discussed earlier, portions of the proposed new construction in the Waipahu Action Plan would be flooded with 6 feet of sea level rise. The depths of the anticipated flooding are shown in the blue gradient, although the flooding depth is not quantitatively expressed in the NOAA tool.
Regarding storm surge, the LEED credit suggests using the “NOAA Sea, Lake, and Overland Surges from Hurricanes
(SLOSH) Model to interpolate storm surge” but it appears that model is only available when there is an impending hurricane near Hawaii, so the additional depth for anticipated storm surge is unknown at this time.
The 1 in 100-year BFE varies from approximately seven to eleven feet above the local tidal datum in Waipahu TOD study area (see the FEMA FIRMette in Figure 36). The FIRMs are based on hydraulic modeling of present day flood risk and do not include future increases in flood hazards with SLR. In the Waipahu TOD study areas that experience flooding with three to six feet of SLR, the land elevation on the topography map is between zero to five feet (see Figure 7).
If the depth of the sea level rise at a particular site and the storm surge were known, they could be added to the BFE to determine the total water level (see Figure 34). If the elevation at the building site is subtracted from the total water level, then the elevation above grade for habitable floors could be calculated.
After documenting the flood hazards at the building site, the LEED credit IPpc98 requires the design team to “assess potential exposure, sensitivity and vulnerability to each hazard based on the project goals, program and intended service for the life of the building” and implement “Climate Resilient Planning” or “Emergency Preparedness Planning”.
Design teams should also consider that future rainfall may not follow historical patterns of intensity, duration, frequency, due to climate change. “It is projected that Hawaii will see more drought and heavy rains causing more flash flooding, harm to infrastructure, runoff, and sedimentation.” Design team engineers may consider using sources of information for projected future rainfall. One information source is WeatherShift Rain, which “uses data from 21 global climate models (GCMs) to generate projected climate change-shifted [in years 2035 and 2090] rainfall Intensity-Duration-Frequency (IDF) curves and 27 GCMs to generate daily time series rainfall data for use in drainage infrastructure design, such as sizing storm drain networks, pump stations, treatment plants, and rainwater harvesting tanks.”
BASE FLOOD ELEVATION AND DESIGN FLOOD ELEVATION
FEMA’s FIRMs include flood zones and a property’s BFE, which is “the computed elevation to which floodwater is anticipated to rise during the base flood”. A base flood is defined as “having a one percent chance of being equaled or exceeded in any given year (also called the “100-year flood”)”.
“Freeboard” is defined by FEMA as “a factor of safety usually expressed in feet above a flood level for purposes of floodplain
Figure 36 FEMA FIRMette shows the Base Flood Elevation (BFE) measured from the local tidal datum.*
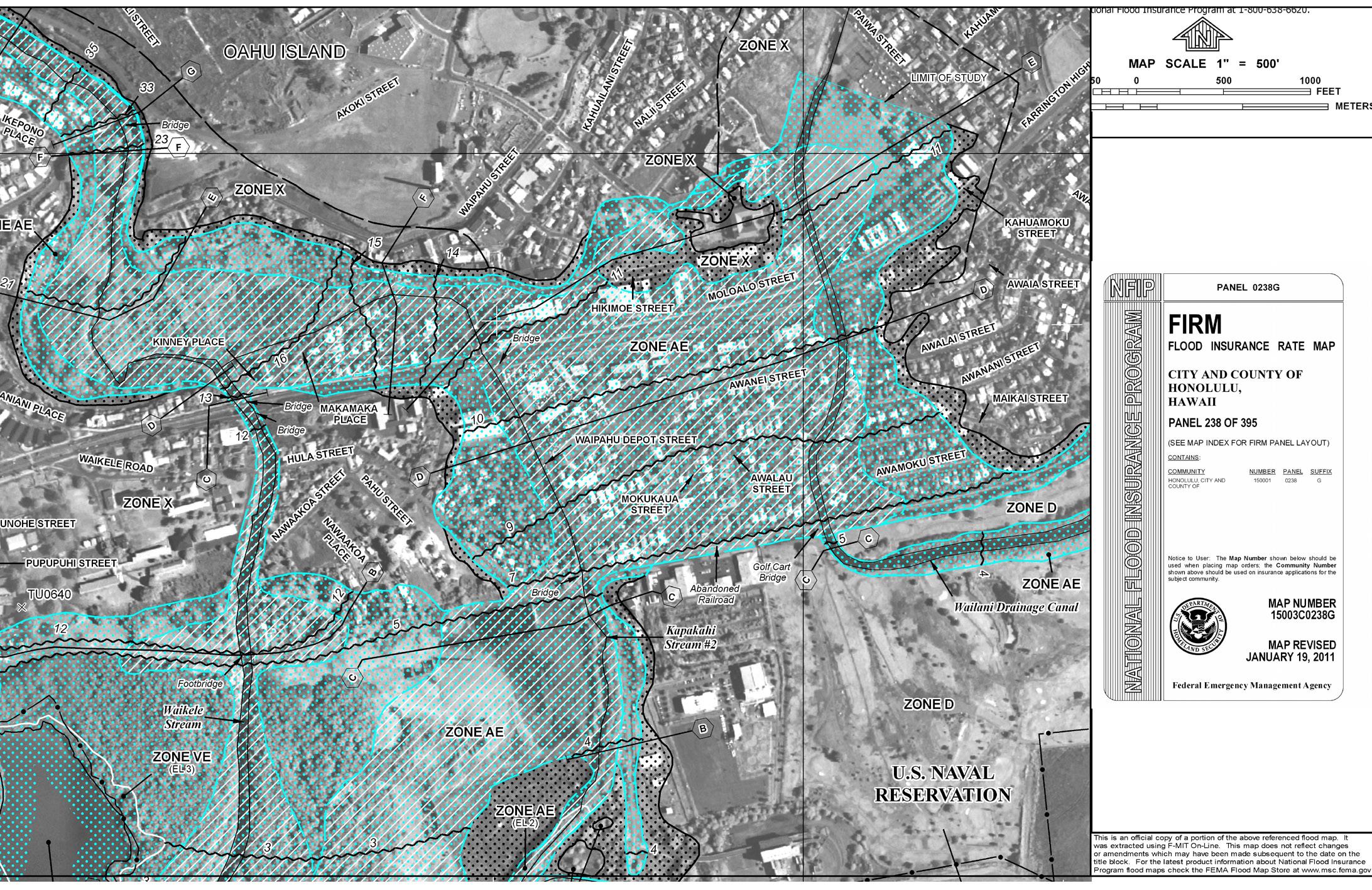
* FEMA Flood Map Service Center, https://msc.fema.gov/portal/home
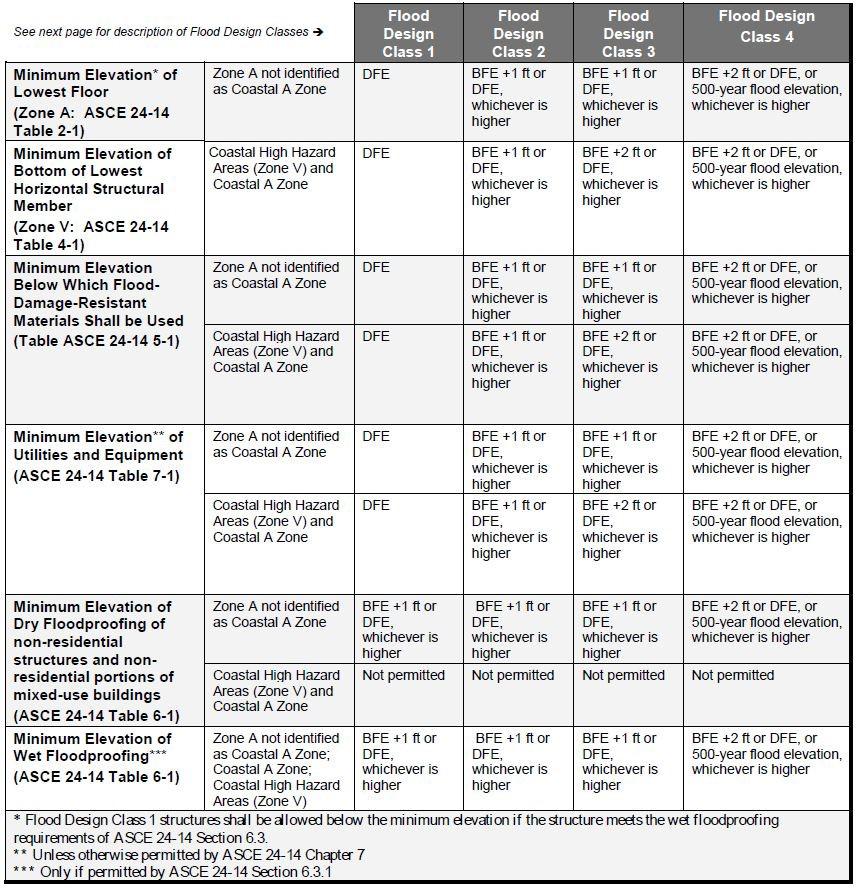
Figure 37 An excerpt from the ASCE 24-14 reference sheet shows habited floor elevations based on Flood Design Classes
Standard FEMA LEED IPpc98
Freeboard for Design Flood Elevation (DFE) Suggestion (feet above FEMA BFE)
+1 to +2 depending on building “class”, or at 500-year flood (if known), according to ASCE 24-14 Varies depending on 1% chance annual flood, sea level rise, and storm surge
LEED IPpc99 RELi Fortified Enterprise MultiFamily Housing NIBS
+5 Plan for 500-year floods +3 or 500year flood elevation (if known) +1 to +2 Depending on region (up to +6 in areas around Hurricane Sandy devastation)
Figure 38 Suggested elevation above base flood elevation (BFE)
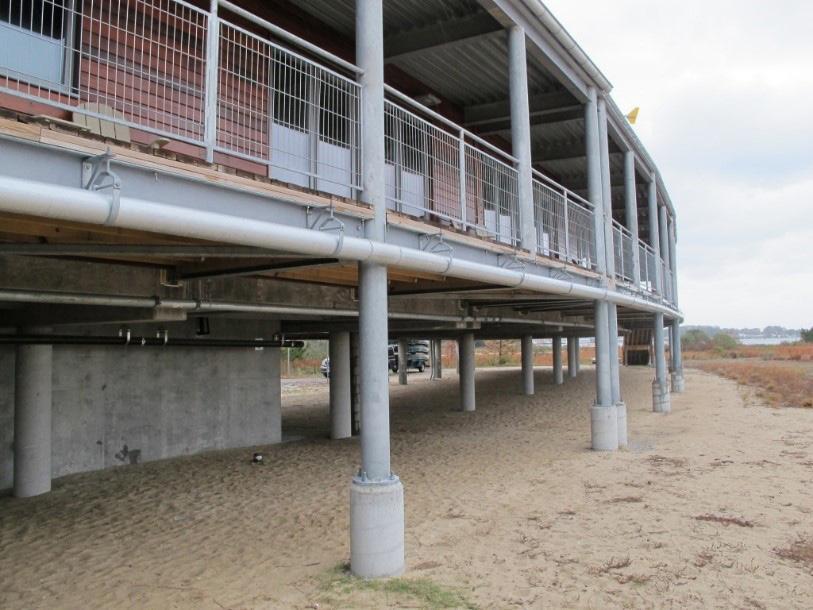
Figure 40 The rock Environmental Center in Virginia is elevated to exceed the standard for a 500-year storm event and future projected sea level rise flooding.*
* Chesapeake Bay Foundation. “Making of a Green Building”. N.d. Accessed November 1, 2018. http://www.cbf.org/about-cbf/locations/virginia/ facilities/brock-environmental-center/about/making-of-a-green-building/index. html management. Freeboard tends to compensate for the many unknown factors that could contribute to flood heights greater than the height calculated for a selected size flood and floodway conditions, such as wave action, bridge openings, and the hydrological effect of urbanization of the watershed”. In some cities across the United States, building codes reference design flood elevations (DFE). DFEs are typically higher than BFEs and are “the minimum height at which residential units may be constructed and utilities like the boiler, the water heater and electrical equipment may be located. It also sets the minimum height for dry or wet flood-proofing measures for buildings generally”.
The Hawaii State Office of Planning may consider establishing freeboard and DFEs that address flooding from simultaneous sea level rise, storm surge, and heavy rainfall events.
ELEVATION
A logical approach to flood-proofing is to elevate existing or new habited spaces and critical infrastructure above the BFE. This chapter addresses the resources design teams or policy makers may refer to when determining the height to elevate a structure above the BFE. This section on elevation will discuss existing codes and regulations, explore resiliency guidelines, provide suggestions, as well as a case study.
FEMA determines minimum elevations based on building function and location/hazard exposure (see Figure 37). The table below is an excerpt from the ASCE 24-14 reference sheet from the FEMA website. Higher flood class numbers indicate more critical building functions. For example, sheds and storage fall under Flood Design Class 1, while hospitals fall under Flood Design Class 4. FEMA references ASCE 24-14 and deems the document to “meet or exceed the minimum National Flood Insurance Program (NFIP) requirements for buildings and structures”. IBC 2006-2012 “requires dwellings in floodways to be designed in accordance with ASCE 24” and the 2015 edition of the IBC allows use of ASCE 24 for dwellings in any flood hazard area.
Current building code and regulation in the Revised Ordinances of Honolulu (ROH) Chapter 21 Article 9 require buildings within flood fringe zones to elevate habited floors up to BFE. The table below (see figure 38) compares the design flood elevation (DFE) from various reference documents and resiliency guidelines for consideration by planning officials and design teams. Resiliency guideline sources exceed the ASCE 24-14, but as the NIBS elevation requirement suggests, each region of the country experiences different levels of storm surge.
The City of Boston’s Climate Resiliency Guide provides a useful precedent for Honolulu. Boston requires large projects to take
sea level rise, local land subsidence, and rainfall into account in determining BFE and DFE. If the project site is located in a Sea Level Rise Flood Hazard Area (SLR-FHA), teams use an online mapping tool, which takes into account a 1% annual chance flood event with 40 inches of sea level rise. Next, to calculate the Sea Level Rise-Design Flood Elevation (SLR-DFE), a minimum 24” of freeboard is added for critical facilities and infrastructure and buildings with ground floor residential units or a minimum 12” of freeboard for all other buildings and uses. Flooding and other climate change related issues for large projects are reported to city officials through a Climate Resiliency Checklist, which is due with the initial project filing, design/building permit filing, and construction/certificate of occupancy filing.
Owners of detached houses in the Waipahu TOD study area anticipated to experience flooding with three feet of sea level rise may consider elevating the houses to or above the BFE. FEMA 347 Above the Flood: Elevating Your Flood Prone House provides several techniques, each with corresponding case studies:
1. Extend the walls of the house upward and raise the lowest floor; 2. Convert the existing lower area of the house to non-habitable space and live in the existing second or third story space (see Figure 41); 3. Lift the entire house, with the floor slab attached and build a new foundation to elevate the house.
The Chesapeake Bay Foundation’s Brock Environmental Center was the world’s first LEED Platinum building and designed to be self-sustaining and resilient. The shoreline setback distance, storm water management, and building elevation are useful examples for the Waipahu TOD study area. In addition to passive daylighting, heating and cooling, and on site energy production, the center’s site is located 200 feet from the shoreline, which is “100 feet … beyond Virginia’s standard 100-foot Chesapeake Bay Preservation Act Resource Protection Area”. The site also treats run off on site and “prevents increases in peak runoff volume and net increases in runoff volume and pollutants. Permeable roads and paths allow water to filter into the soil, while rain garden treatment, rain water harvesting, and water re-use reduce the volume of runoff and meet pollution-reduction objectives. The site design ensures that all runoff on CBF’s property is filtered through the vegetation and the soil, not flowing untreated into local waters”. The building itself is elevated 13.8 feet above sea level “to exceed the standard for a 500-year storm event and future sea level rise projected flooding” (see Figure 40.)
Existing and new buildings should elevate utilities (e.g., water
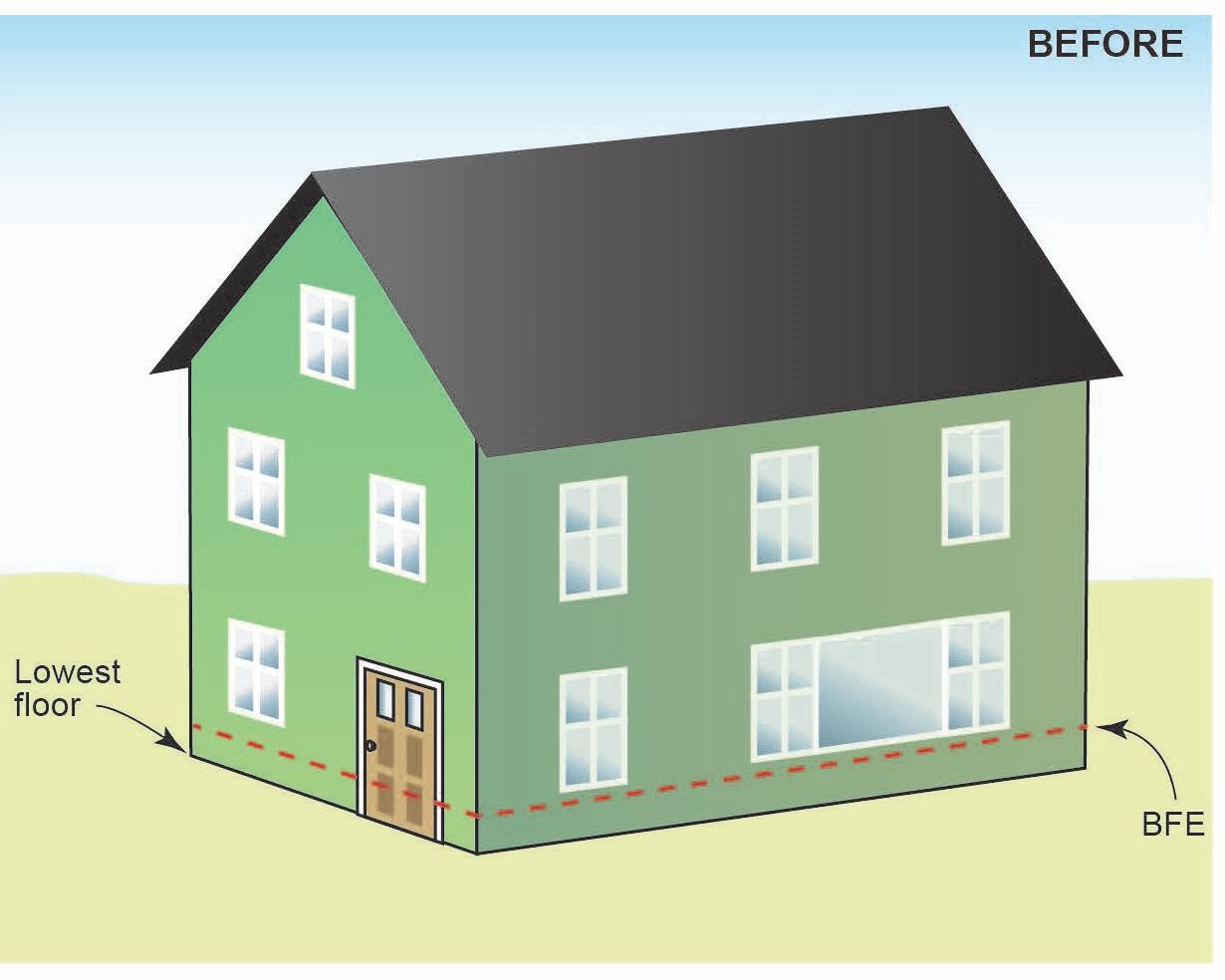
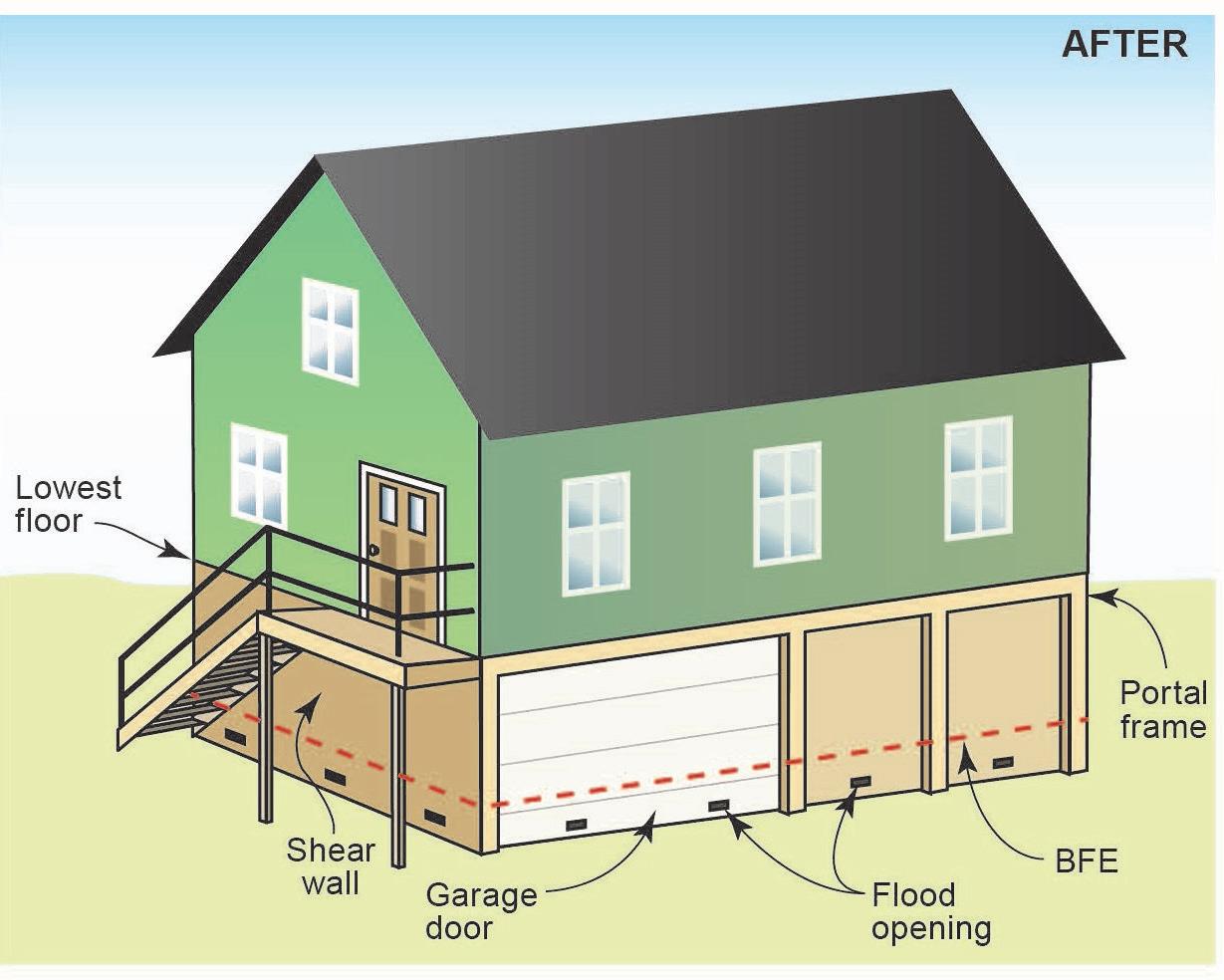
Figure 41 One wet-flood proofing option is to abandon the lowest floor and replace it with non-occupied space and install flood openings and/or break away walls.*
* FEMA. “P-1037 Reducing Flood Risk to Residential Buildings that Cannot Be Elevated”. September 2015.
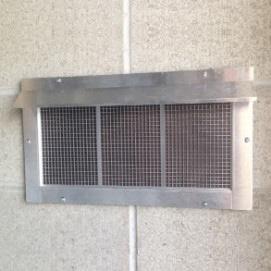
Grate style
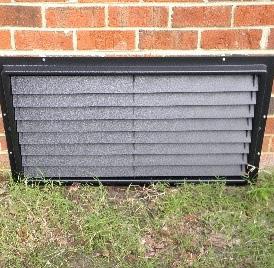
Louver style Hinge style
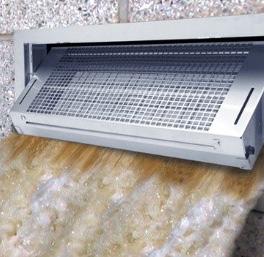
Permeable/Break away walls
Figure 42. Four examples of wet-floodproofing fixtures. heaters, air conditioning equipment, electric meters, and septic tanks) above the BFE to protect them from damage or loss of function from flooding. Homeowners may elevate outdoor equipment on platforms, or move indoor equipment to higher floors or attics, or build an elevated utility room.
WET FLOODPROOFING
After elevating critical building functions, design teams may consider wet floodproofing methods to allow water to pass through non-critical areas of the building with minimal harm. As described by FEMA, the benefits of wet floodproofing are that “if floodwaters are allowed to enter the enclosed areas of the home and to quickly reach the same level as the floodwaters outside, the effects of hydrostatic pressure, including buoyancy, are greatly reduced”. Flood proof materials should be used to minimize structural damage and critical machinery and equipment should be moved to higher floors. Electrical systems including outlets should be elevated to avoid contact with flood waters.
Other benefits of wet floodproofing include becoming NFIP compliant only if: “(1) the area is limited to parking, access, or storage, (2) designed to allow for automatic entry and exit of flood waters through the use of flood openings, and (3) uses only flood damage-resistant materials below the DFE”.
General wet floodproofing guidelines include the following.
1. Spaces below the DFE should not contain critical equipment, mechanical or electrical systems, or be a habitable space.
Functions such as garage, storage, or building access are appropriate for floors below the DFE. “Discounts are currently available under the NFIP for elevating building utilities in the V Zone. There are also discounts available for this activity in the A Zone when utilizing the NFIP’s Submit for Rate Guide. Unlike other alternative mitigation measures, elevating building utilities is effective against coastal floods or high velocity riverine flood forces, provided that indoor utilities are elevated to a higher floor or inside an elevated utility room supported on piles.” (FEMA P-1037)
2. If the area must be enclosed, walls should be constructed to break away during a flood event or should include flood vents to allow water to enter.
“The benefit of wet floodproofing is that, if floodwaters are allowed to enter the enclosed areas of the home and to quickly reach the same level as the floodwaters outside, the effects of hydrostatic pressure, including buoyancy, are greatly reduced.” (FEMA P-312) A break away wall is a “wall that is not part of the structural support of the building and is intended to collapse under specific lateral loading forces, without causing damage
to the elevated portion of the building”. Alternatively, one may install flood vents in existing walls that allow for the automatic entry and exit of floodwaters. Several different flood vent options are shown in Figure 42.
3. Materials below the DFE should also be flood damage resistant.
Building materials installed in floodable spaces—including framing, wallboard, flooring and ceiling paneling—should be able to survive water exposure without major damage, promoting mold or mildew, or absorbing contaminants. Building materials under the DFE should also be able to withstand contact with floodwaters for up to 72 hours without requiring more than cosmetic repairs. Examples include flood damage-resistant building finish materials such as non-paperfaced gypsum board and terrazzo tile flooring versus traditional drywall and carpeted flooring, vinyl flooring over a concrete slab, metal doors, glass block windows, and concrete walls. Wet floodproofing is also considered a relatively low cost measure with an expected useful life ranging from 15 to 20 years with some limited annual maintenance costs. In coastal areas with salt water, corrosion of metals may be a problem. Materials that might dry out and be usable after exposure to fresh water may be damaged beyond repair by salt water.
For buildings that cannot be elevated, several interior modifications may be possible, along with wet floodproofing techniques.
1. Abandon the lowest floor. Convert the existing lower area of the house to non-habitable space and live in the existing second or third story space (see Figure 41). 2. Elevate the lowest interior floor to make floor(s) with lower floor to ceiling heights (e.g., modifying from ten feet to eight feet).
DRY FLOODPROOFING
Dry floodproofing involves “sealing your home to prevent floodwaters from entering” and may be necessary for buildings that cannot be elevated. Building owners can dry flood proof their homes by:
• using waterproof coatings or coverings to prevent floodwater from passing through the walls; • installing waterproof shields or flood gates over doors and openings; • installing devices that prevent sewer and drain backup • sealing cracks and openings in walls and foundation; • protect against seepage by installing a sump pump Dry floodproofing can withstand hydrostatic loads up to a height of three feet. Dry floodproofing is not recommended for homes with frame walls at low levels or with basements or crawlspaces.
Below the DFE, equipment should be waterproofed. Existing or new building utilities (e.g., water heaters, air conditioning equipment, electric meters, septic tanks) may also be modified with dry floodproofing. For example, outdoor equipment may be placed behind a wall or watertight, passive utility enclosure up to or above the BFE to protect them from damage or loss of function from flooding.
Below the DFE, materials should be flood proof for easy clean up. Because floodwaters often carry debris and hazardous materials, cleaning up a dry floodproofed home after a flood may involve removing mud and disinfecting surfaces.
Dry floodproofing may not be as cost effective as other methods because “construction of a passive dry floodproofing system is generally considered a relatively high-cost measure with an expected useful life ranging from 15 to 30 years and extensive annual maintenance costs needed to maintain the various elements of a floodproofing system”. The cost of the system is highly dependent on the area size, wall type, number of existing openings, plumbing and electrical lines, etc. However, there are currently no flood insurance premium rate discounts available under the current program.
Before finalizing a plan for dry floodproofing, an engineer should take into consideration the expected flood depth, flow velocity, erosion and scour, debris impact, wave action, flood duration, post-flood clean up, and active human interaction required for setting up barriers such as shields.