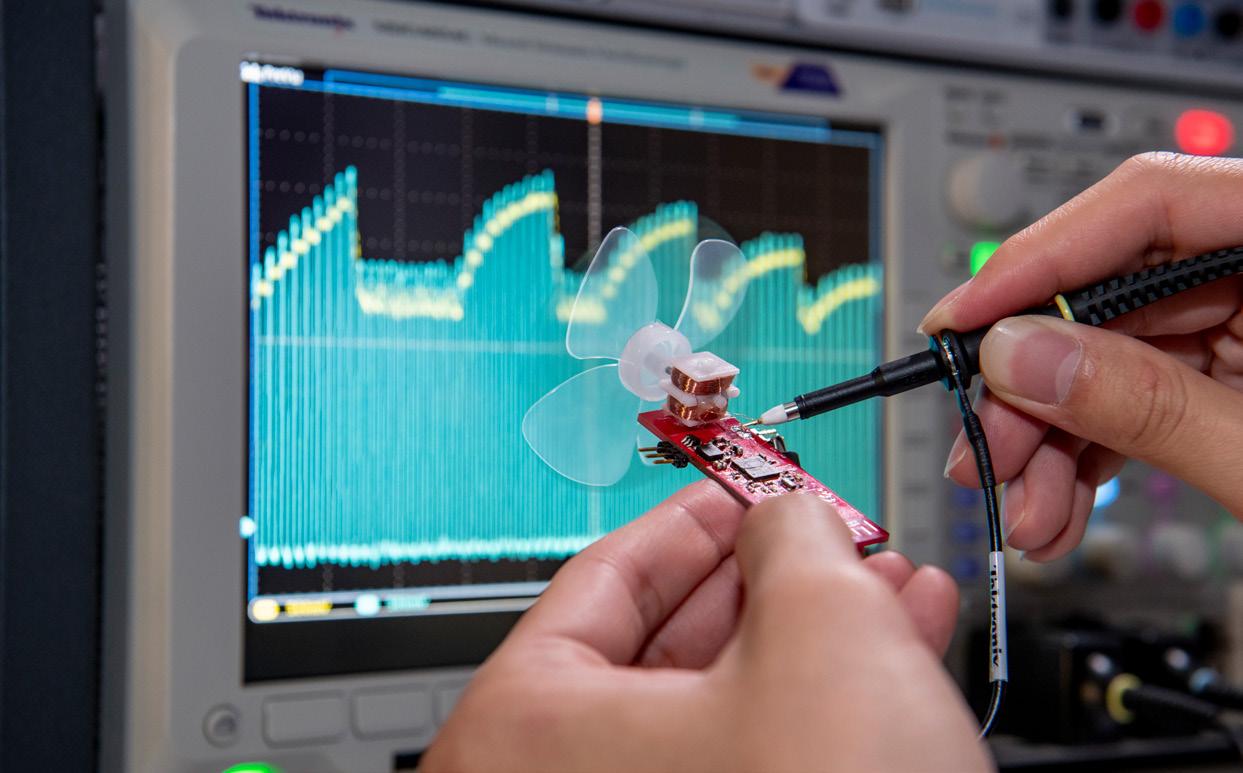
5 minute read
POWERING THE IOT
charging systems. It will also look at smart energy and smart transportation more broadly, for example examining ride sharing and on-demand transport systems and how people can be incentivised to provide information about their needs truthfully to optimise these systems.
Optimising crowdsourced data
Another area where people and devices interact to collect data is crowdsourcing. Our researchers have looked into the optimisation of crowdsourced data – for example the design of algorithms to advise people of the best time or place to collect pollution data. This could be to get a good spread of readings in terms of timing and geographical location, or to validate ‘noisy’ data by getting multiple readings at the same place and time. This work has the potential to inform measures to reduce the impact of pollution, for example by diverting traffic to prevent pollution built-up or alerting people to avoid areas of high pollution.
Putting users in control
From smart doorbells and heating systems to virtual assistants, the use of home IoT technology is growing fast. Generally the terms of use mean users have to accept that the data these devices generate will be shared with service providers and perhaps sold on to other entities; however, this raises issue of data privacy and consent. To address this, our researchers are looking at access control –that is, the ability of IoT device owners to specify what subsets of data other entities can access and how they can process it. The aim is to give users more fine-grained control over their data so they can make informed choices about what and how much they share. For example, someone may be comfortable with sharing data from individual devices but would prefer data from different devices not to be aggregated, as this would reveal more about their lifestyle and activities.
Research in this area is tackling technical challenges such as how decentralised infrastructures can help to enforce access control by keeping as much data as possible at the edge of a network rather than combining it in a centralised store, the use of metadata to enable more fine-grained control, how data management models can enable more meaningful consent about IoT data use, and ways to ensure users’ consent preferences are honoured.
There is also a sociotechnical aspect, such as enabling people to have the digital literacy to participate in the wider dialogue about privacy and control, and designing user interfaces that allow people to make informed decisions about data consent in a manageable way.
Another branch of research is finding ways to overcome the operational and cultural barriers that prevent businesses from sharing mutually beneficial data, through risk assessments that build trust between stakeholders.
Work at Southampton on the sociotechnical aspects of data infrastructures and access control is contributing to wider debate in this area. For example, our researchers contributed their expertise to a report on the European digital infrastructure and data sovereignty 4 , using different scenarios to demonstrate to policy makers what would happen if data protection and infrastructure controls were tightened or loosened.
However, traditional battery power is not the ideal solution, given the impracticality and expense of replacing or recharging spent batteries in billions of devices, and the environmental impact of disposing of them. Since undertaking the first EPSRC-funded energy harvesting project in 1999, Southampton has a longstanding track record of innovation in the field of energy harvesting technologies. These are the components within IoT devices that locally generate electrical power from ambient sources such as light, vibration and temperature differences. For example, vibration-powered sensors developed by our researchers have been successfully commercialised
5 http://eh-network.org/ through spin-out company Perpetuum. We are continuing to investigate new ways to power the IoT, to make devices as energy efficient as possible and to balance the trade-offs between functionality and a low ‘power budget’.
Recognised internationally as a centre of excellence in this field, Electronics and Computer Science at Southampton hosts the UK’s Energy Harvesting Network5 established in 2010, which aims to encourage collaboration and disseminate the latest advances.
3 https://ccais.soton.ac.uk
4 https://www.eitdigital.eu/newsroom/news/archive/article/new-report-on-european-digital-infrastructure-and-data-sovereignty/
System-level and energy-driven design
One area of research takes a system-level approach to IoT device design, aiming to co-design the energy harvesting and sensor components for maximum efficiency and reliability, tailored to the required application. Work is also ongoing to build intelligence into devices to enable them to adapt their performance to the available energy supply –known as energy neutral behaviour.
Our researchers have used this holistic approach to develop wireless energy harvesting sensor systems to monitor the health of engines and components in aircraft, marine vessels and other vehicles. One example is a credit-card sized, self-powered smart sensor designed to be integrated into a helicopter blade. The device uses a screen-printed piezoelectric generator to harvest energy from the blade’s vibrations, integrated with energy management, sensing and data transmission components, to sense and send data about the condition of the blade. In a similar vein, researchers are looking at how to harness the heat or motion inside jet engines to power a real-time monitoring system for their bearings.
application requirements and designs them in tandem. This approach is particularly useful for problems where the energy harvesting method dovetails with the application.
One example is a step counter that harvests energy from the insole of a shoe. Each step generates a small spike of power which indicates that a step has occurred while also powering the processing and communication of step data. The sensor dies (its power runs out) between each step and there is no need for energy storage. Unlike traditional step counters that are powered continuously to monitor motion and process data to detect steps, the power itself is used as a way of indicating that the step has occurred.
Another example is a bike computer that generates energy each time a magnet on the wheel passes a device mounted on the fork – the energy spike can be used to extrapolate information about speed and distance as well as powering the device, removing the need for any energy storage.
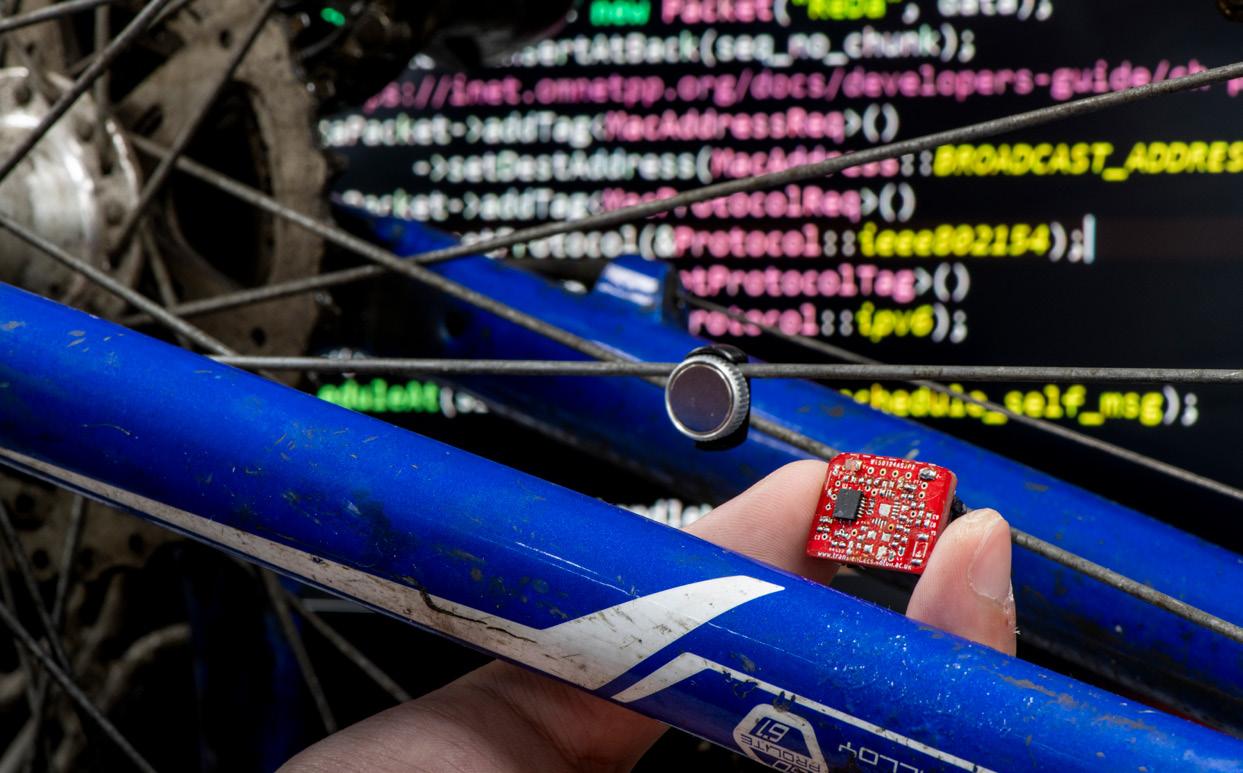
Energy harvesting innovation and optimisation
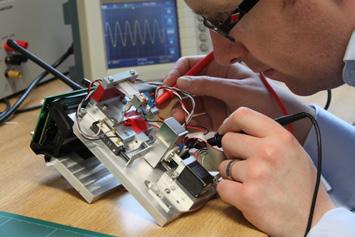
As well as looking at whole-system approaches, our researchers are working on enhancing existing energy harvesting technologies and developing new ones, building on a long heritage of innovation in this field.
Other longstanding areas of development include piezoelectric energy harvesting, which turns mechanical pressure into power, electromagnetic energy harvesting, which generates power using vibration or motion (the technology exploited by spin-out company Perpetuum), and thermoelectric energy harvesting, which uses temperature differentials.
We are also developing new areas, such as temperature-driven phase change generators, that exploit the feature that gases have bigger volume than liquids for a given mass. By turning a liquid into a gas and back again using daily temperature variations, the resulting change in pressure can power an energy harvester. Such systems offer the potential to be more robust than solar power for some environmental IoT applications.
Work is also ongoing in the field of wireless power transfer (WPT), through ambient radio waves such as mobile phone signals, or direct energy broadcast. While commercial systems exist for energy broadcast, there is lots of potential for improvement – for example looking at the design of antennae to make the transmission more directional. Another area of our WPT research is inductive coupling, which involves the transfer of power via coils that can be printed onto clothing or equipment. By tuning the coils and operating them at a specific resonant frequency, it’s possible to increase the range over which power can be transmitted. One application for this work is in