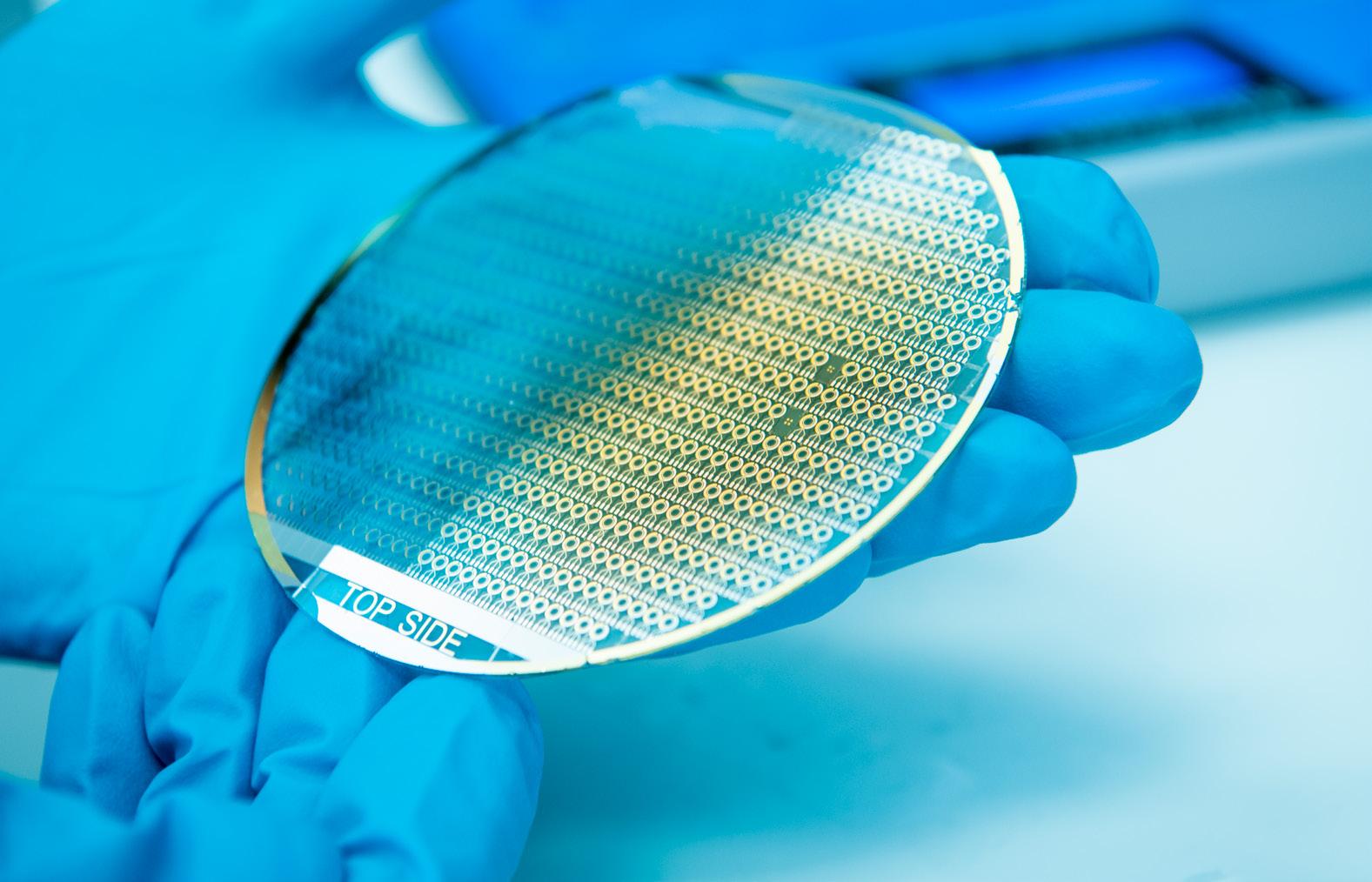
3 minute read
NANOTECHNOLOGY AND SYSTEM-ON-CHIP
IoT devices rely on a combination of components that enable them to sense their surroundings and to store, process and transmit data. The design and configuration of these components involves numerous challenges – they must be very small, but also have the processing capacity needed for their task, and the ability to generate and use power efficiently. To be viable for mass production, they also need to be fabricated using low-cost materials that have minimal environmental impact.
Our researchers are at the forefront of fundamental research that is advancing these underpinning technologies at the micro and nanoscale. Using thin film deposition, nanowire electronics and other nanofabrication techniques, they are working on new materials and designs for sensing, processing, memory and power generation components, and optimising the integration of these components into multi-layered on-chip systems. Their work takes advantage of Southampton’s exceptional cleanroom complex, which includes state-of-the-art microfabrication and nanofabrication facilities.
Nanoscale sensing technology
One element of this research focuses on the development of sensor technology using zinc oxide nanowires. A class of metal oxide semiconductor, zinc oxide is a cheap, plentiful material with low toxicity and low environmental impact, making it an ideal material for IoT components. Ionic in nature, it reacts readily to positive or negative charges; by analysing these reactions it’s possible to identify particular micro or nanoscale targets – for example the presence of a particular type of pollution particle, or abnormalities in DNA that could indicate potential disease.
Our researchers are continuing to scale down the technology further, to detect micro ribonucleic acids (miRNAs), the building blocks of DNA. The initial aim is to develop this technique for use in disease diagnosis, drug trials and the design of tailored drug treatments. However, it’s a technology with many other IoT possibilities, such as distributed sensor networks for air, soil or water pollution, as well as smart biomedical applications.
On-Chip Power Management
Another stream of this research involves the design and integration of power management circuits into chip systems. Sensor technologies are power hungry, and the amount of power available to a sensor system limits how much data it can collect, process and transmit. Typically, current sensors can only work intermittently to sense, process and transmit their data, after which their power is depleted and they need time to recharge. This poses a problem if more frequent monitoring is needed. Our researchers are developing systems to maximise the energy efficiency of IoT devices using zinc oxide nanowire technology.
Powering on-chip systems
When it comes to powering on-chip IoT systems, nanoscale thermoelectric generation is a good fit as it produces energy in the microwatt to milliwatt range that these devices require. It can also be a useful solution in scenarios where solar or vibration energy harvesting isn’t possible.
It uses semiconductor materials that react to temperature differentials – when they get hotter on one side, carriers diffuse from the hotter to the colder side of the material, creating a current. There are two types of thermoelectric material, n-type and p-type, which create negative and positive charges respectively. When an n-type doped material is connected electrically in series and thermally in parallel across a temperature differential to a p-type doped material, current flows between the two. Across multiple pairings, this process generates the milliwatts needed to power IoT on-chip systems.
Our researchers are working on the development of thermoelectric materials that are abundant and cheap to manufacture, and looking at how to optimise these materials for maximum energy generation by varying doping concentrations and nanostructures. They are also designing, modelling and fabricating thin-film micro-generators to harvest the thermal energy, and looking at how best to integrate these components – for example, to incorporate many n-type and p-type pairs into a chip system for high-power performance.
Different environments have different thermal conditions, so another aspect of this research is device design optimisation, using AI technologies to accurately predict the performance of on-chip systems under different conditions. This will enable designs to be tailored to maximise device efficiency and performance in specific settings.
Memory optimisation for edge computing
One function of an IoT device is the transmission of data – traditionally to a central point – for processing. This takes a lot of power, so there has been a move towards edge computing – where some of the analysis and decision making happens at or near the device level – to minimise the amount of data that needs to be communicated. To do this, it’s essential to integrate memory into the system, so another area of research is the development of novel nanoscale memory devices that can be integrated into embedded systems.
Integrating components into on-chip systems
The development of novel components leads to another challenge – how best to integrate them into a single on-chip system. Research is underway to identify the best materials and the most effective three-dimensional configuration of sensors, microprocessors and transmitters in multilayered devices.