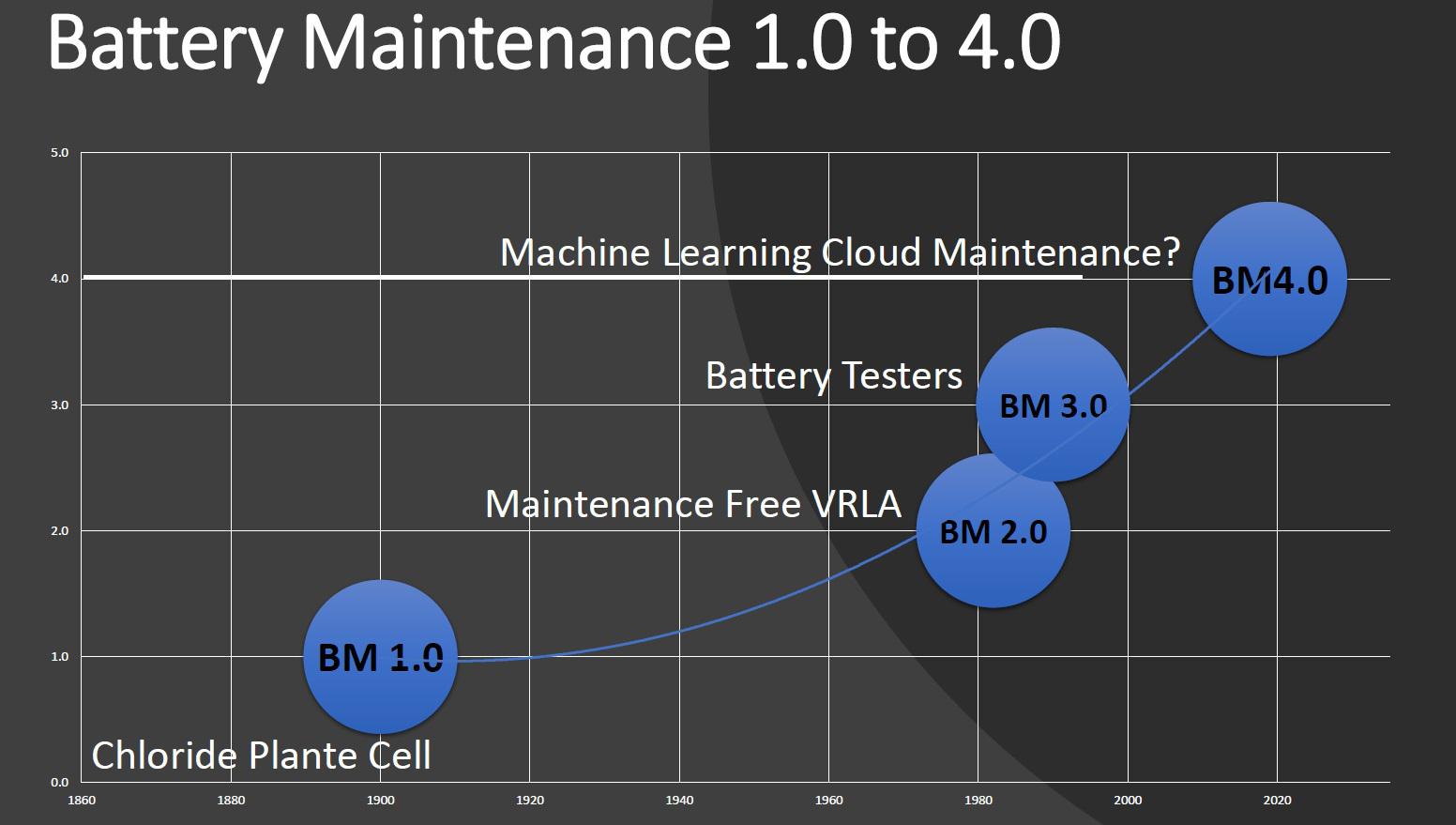
4 minute read
BATTERY STORAGE
PIONEERING BATTERY MAINTENANCE 4.0
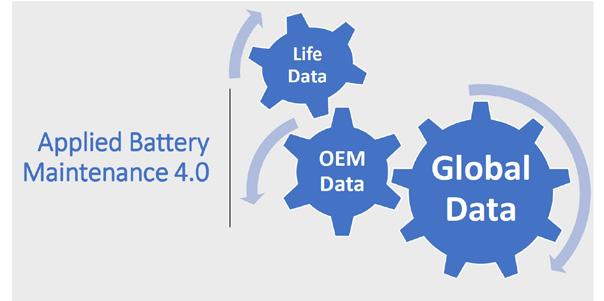
Advertisement
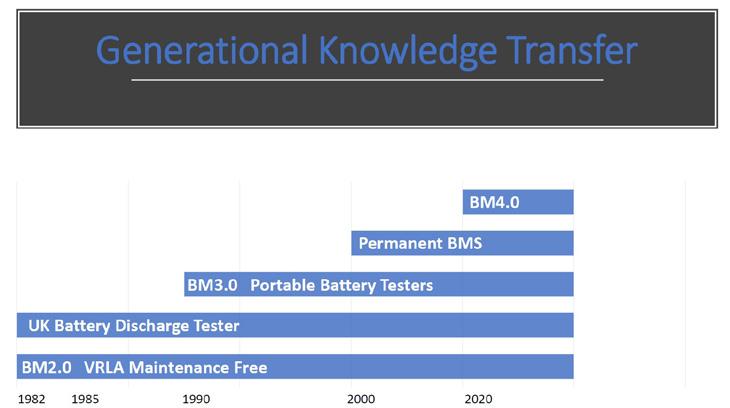
Is there a way of using the technology of tomorrow to learn from the experience of yesterday and improve the reliability of batteries used in standby power systems? Or put another way - can we combine technology and generational knowledge transfer to reach Battery Maintenance 4.0 In this second article from Paul Smethurst of Hillstone
Loadbanks he goes back to the pre-pandemic and reanalyses the presentation he gave at the 2019 NIBS
Battery Conference. Let’s first explore the background of how we arrived at
Industry 4.0 and how we can draw comparisons to the history of batteries. Industry 1.0 defines Steam Power, circa 1750, with great people like Abraham Darby, James Watt and
Isambard Brunel. Industry 2.0 followed with Electrification, circa 1850, and for a battery article fits nicely with the Frenchman
Glaston Plante. Industry 3.0 in recognized with Factory Automation from the early 1970’s being pivotal from the advances of computer technology and software in programmable logic controllers ( PLC ), as derived from mathematicians such as Alan Turin. This leaves today’s advances into Cloud Computing and the innovation of the world wide web from Sir Tim
Burners Lee for Industry 4.0 However the industrial revolution cannot just be left in a 4 point summary without recalling the effort of the observation, by William Stanley Jevon in 1865, of the consequences on the demand for power in reaching for technological advances. “Whereby the efficiency of James Watts steam engine did not reduce the amount of coal that was used, in fact it increased coal consumption due to the wide spread success of the steam engine.” Which became the Jevon
Paradox The relevance of Jevon Paradox can be seen today in terms of electricity demand. There is an increasein the use of batteries within renewables generation to reduce the need for coal. It can also be used on Category 5 genset efficiencies and also on PUE calculations in data centres. If we apply a Jevon paradox to battery maintenance, we can create a series of interesting analogies across the past 120 years of reliability and performance of standby batteries. The starting point can only be the production of the
Plante cell, circa 1900, and the subsequent maintenance procedures which evolved to achieve a design life of 25 years becoming the operating life of the Plante cell. While this article is not going to list all the maintenance procedures involved with wet cells, we can define this as Battery Maintenance 1.0 It took 80 years and the advent of the ‘maintenance-free’ sealed Value Regulated Lead Acid ( VRLA ) battery, to advance into Battery Maintenance 2.0, but this was not without issues, such as: “Why does a maintenance-free battery need maintenance?” and “How can it be sealed if it need a breather valve to release the build-up of gases?” But in comparison, BM2.0 was a big improvement to BM1.0, especially as VRLA didn’t need to be topped up and live in a wet room ( with sulphuric acid splashing about all over the floor ). Note: North America grid and some European & Middle East telecom companies retain open-vented batteries and perform detailed maintenance to retain the design life in operation. The implementation of VRLA however did not go without issue and with this design came the loss of capacity over its design life which created the need to perform discharge testing. So in the 1980s designs of DC load banks were delivered into the market. Today there are other load bank manufacturers but there is only one Hillstone! It didn’t take long to move towards Battery Maintenance 3.0 which has been in existence now for 30 years with the development of battery monitoring systems, and battery conductance, impedance and resistance meters and systems. All these technologies are based on historical trending of data which requires the experienced interpretation to anticipate when to replace the battery before it fails. I’m sure they all claim they are the one to use, but a load bank discharge test is the method to determine the capacity and true age of the battery. Lead acid batteries are still very sustainable with 95-98% recyclability, but with the threat to reliability now being seen from commoditising the battery production and sales the perception to replace rather than to maintain creates a financial trade-off to valuing the loss of power to the loss of service. The introduction of VRLA batteries has seen the an increase in their mass production in order to sell in volume a low cost product. The trade-off in quality increases premature failure, but that can also be seen in higher quality products as well, especially if maintenance is not correctly performed. For example: if a 10-year design life battery fails at 5 years or a 5-year design life battery fails at 2-years, we are not achieving greatness of efficiency or purpose within a reliable standby power system. Does the opportunity to harness the power of cloud compute to run algorithms and one day for machine learning, give Battery Maintenance 4.0 the ability to solve the issues of wide scale and cost-effective battery maintenance? The closing consideration falls back to using a Jevon’s Paradox and applying it to battery maintenance. The resource efficiency needs to increase, i.e. More performed battery maintenance Harnessing technological advances; i.e. Cloud Computing. The global increase in the use and application of batteries is increasing exponentially, which will result in a demand for an increase in the battery maintenance performed. Therefore could the answer be that we start developing the concept of Battery Maintenance 4.0?