December - January 2023
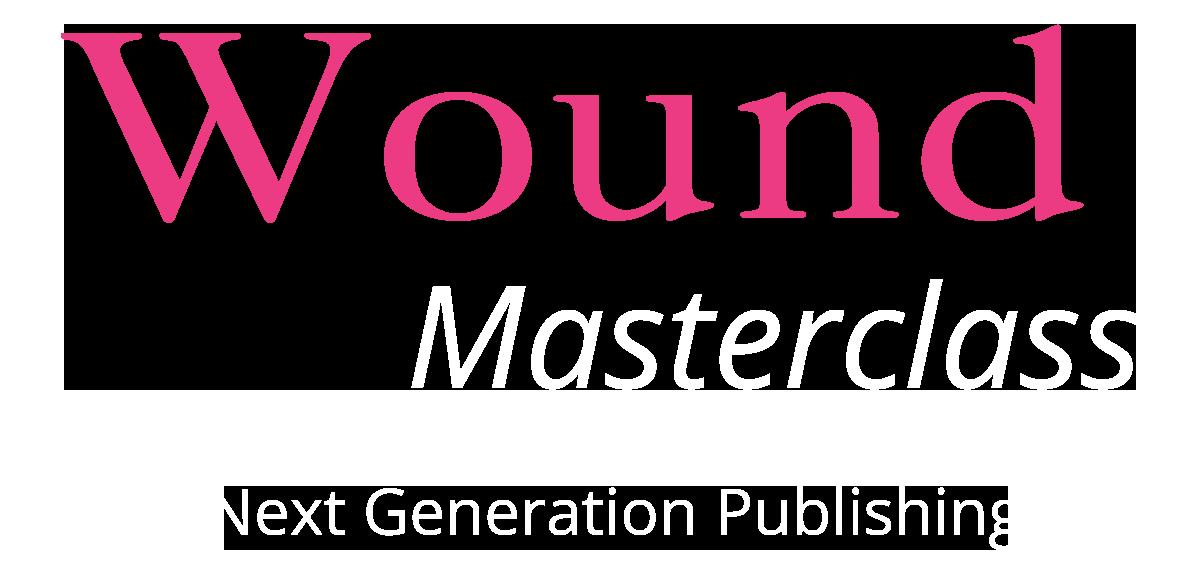
December - January 2023
Current standard-of-care wound dressings are passive and do not actively respond to variations in the wound environment. Smart bandage technologies address these challenges, with their ability to integrate multi-modal sensors and stimulators for real-time monitoring and active wound care treatment with little intervention from medical providers required. This article provides an overview of the role of wireless, closed-loop, smart bandages with integrated sensors and stimulators.
Chronic non-healing wounds are a major healthcare burden, with approximately 2.2 million people with a chronic wound in the UK, with DFUs and PUs costing £1 billion and £531 million, respectively.1 In the United States, the total number of prevalent cases of DFU was 4,551,498 cases in the year 2020. Unhealed ulcers and foot infections are the leading cause of diabetes related amputations, with diabetic foot ulcers preceding 85% of amputations.2 In the United States, DFU patients are twice as costly to US Medicare as those with diabetes alone. These wounds are associated with loss of function and mobility, increased social stress and isolation, depression and anxiety, prolonged hospitalization and overall increased morbidity and mortality.2
Current standard-of-care wound dressings are passive and do not actively respond to variations in the wound environment.2 Smart bandage technologies address these challenges, with their ability to integrate multi-modal sensors and stimulators for real-time monitoring and active wound care treatment with little intervention from medical providers required.3-9 As a wound heals, skin impedance increases, but when a wound becomes infected wound impedance decreases due to the development of biofilm.10-11 Further development of infection causes an increase in wound temperature through local inflammation.12 These signals can be captured by low-cost sensors embedded in a wearable device to act as a sentinel for impending wound infection.2 These biophysical signals provide rapid, robust and accurate information about
wound conditions in real time, creating an opportunity to diagnose and monitor a nonhealing wound quickly and autonomously in a closed-loop fashion.2
Current smart bandage technologies can detect a variety of physiological changes including pH,1,3-4, temperature,15-18 oxygenation,19 impedance,10,20-1 motion22-3 and enzymatic fluctuations,18, 24-5 of the wound.
Electrical stimulation can reduce bacterial colonization and biofilm infection and restore normal wound healing.26 It also improves tissue perfusion, stimulates immune cell function and accelerates keratinocyte migration through a process known as galvanotaxis.27-9
We developed a battery-free flexible bioelectronic system consisting of wirelessly powered sensing and stimulation circuits with tissue-interfacing tough hydrogel electrodes using a biocompatible conducting polymer.2 We designed a miniaturized flexible printed circuit board (FPCB) containing an energy-harvesting antenna, a microcontroller unit, a crystal
oscillator and filter circuits for dual-channel continuous sensing of wound impedance and temperature, and a parallel stimulation circuit to deliver programmed electrical cues for accelerated wound healing.2To ensure efficient signal exchange and energy delivery between the circuits and the soft skin tissue, a lowimpedance and adhesive hydrogel electrode was used, which has lower impedance across the entire frequency domain compared to wellestablished ionically conducting hydrogels which results in more efficient charge injection during stimulation.30-1 To mitigate secondary skin damage when peeling off the adhesive electrodes we used a thermally controlled reversible phase transition mechanism to the hydrogel backbone and achieved two orders of magnitude lower adhesion at elevated temperature when compared with normal skin temperature.2 Multiple preclinical animal models demonstrated that their smart bandage could continuously monitor skin physiological signals and deliver directional electrical cues, leading to accelerated wound closure, increased neovascularization and enhanced dermal recovery.2 The wireless nature of their smart bandage also allowed us to use complex animal models, such as parabiosis, to investigate the possible underlying mechanisms behind the observed effect of electrical stimulation, which suggested that the beneficial wound-healing outcomes could be attributed to the activation of proregenerative genes in the monocyte and macrophage cell populations.2
The wound management system that we designed was a battery free, wirelessly powered FPCB for simultaneous wound treatment and monitoring and a tissue-interfacing conductive adhesive hydrogel interface for robust and gentle skin integration.2 The smart bandage was flexible and can be comfortably attached to wound surfaces.2 It can be inductively coupled with a radio frequency identification (RFID) reader.2 Through the radiofrequency (RF) energy-harvesting process, the antenna
can provide power to apply electric bias across the wound for programmed treatment and, at the same time, drive the microcontroller unit (MCU)and other integrated circuits (for example, oscillator and filter) for continuous monitoring of wound impedance and temperature via a near-field communication (NFC) transponder in the MCU.2
We first confirmed that mice wearing their wireless devices were able to move freely, with a distance travelled similar to that of mice with no device attached, demonstrating an ideal therapeutic modality for patient use, ie., lightweight and untethered with cables.2 The temperature and impedance sensors were able to monitor wound state continuously as the mice moved freely in the cage.2 The hydrogel was biocompatible and did not initiate any sensitization or irritation after continuous contact with the skin over 15 days, demonstrating absence of adverse reactivity signs compared with normal skin.2 To test the functionality of the platform in a biological system, a splinted excisional wound mouse model was used in which stimulated mice were treated with continuous electrical pulses, while control mice received standard sterile wound dressings without electrical stimulation.2 We found that stimulation resulted in accelerated wound closure and a significant increase in wound impedance to attain a faster impedance plateau, signifying a return to an unwounded state.2,10,20 Stimulation of wounds also improved functional tensile recovery with increased dermal thickness, collagen deposition and overall dermal appendage count.2 Compared with a wired modality, our smart bandage allowed for longer and potentially continuous treatment durations, which have been linked to accelerated wound closure.2, 26 Stimulated wounds also showed an increase in collagen fibre heterogeneity, resulting in more random, shorter and less aligned fibre orientations.2
We observed a significant increase in
“The wound management system that we designed was a battery free, wirelessly powered FPCB for simultaneous wound treatment and monitoring and a tissueinterfacing conductive adhesive hydrogel interface for robust and gentle skin integration.2”
neovascularization among stimulated wounds, with increased microvessel count and higher expression of CD31 and α-smooth muscle actin (α-SMA), with similar results observed in a murine burn wound healing model.2 The smart bandage significantly reduced infection in the wound, decreasing overall bacterial colony count and by continuous monitoring of wound impedance and temperature, their wireless smart bandage could detect early onset of infection and modulate treatment in a closed-loop manner to avoid further wound complications.2
In current clinical practice, doctors still rely on qualitative markers such as swelling or erythema to identify wound infections, which are often difficult to judge in the early stages of biofilm development, but with quantitative biophysical signals recorded by our smart bandage, treatment can be provided when clinically used markers are still ambiguous, enabling timely treatment of chronic wounds, reduction in hospital readmissions and medical cost and improvement in patient woundhealing outcomes.2,32 We also validated their system in a streptozotocin (STZ)-induced diabetic excisional wound model, which most closely resembles type 1 diabetes in patients, also observing an accelerated time to wound closure, improved dermal collagen fiber heterogeneity and increased vascularization.33-4 On the cellular level, we demonstrated the expected ability of our device to prompt cell alignment and migration, inducible with a directional electric field.2
Although the beneficial effects of electrical stimulation have been reported, the cellular and molecular mechanisms for this remained unclear.27 In our work, due to the lightweight and untethered nature of our smart bandage, we were able to evaluate the long-term effects of electrical stimulation on circulating cells involved in wound repair using a complex parabiosis model.2,35 This would not have
been possible previously because, with a conventional wired modality, parabiosis under a long anaesthesia regimen would not survive.2 To do this, we performed parabiosis of five green fluorescence protein (GFP)-positive mice to wild-type (WT) mice. WT mice were wounded and either subjected to electrical stimulation or left untreated.2 Wound tissues from both groups were explanted on day 5 and their transcriptional profiles analysed by singlecell RNA sequencing (scRNA-seq) using the 10X Genomics Chromium platform (figure 1).2 Of all the circulating inflammatory cells activated by our smart bandage, monocytes and macrophages had the highest number of differentially expressed genes in both electrically stimulated and untreated wounds. Even with many neutrophils present, the magnitude of differentially expressed genes did not reach statistical significance.2 Similarly, while there were higher numbers of B and T cells in the stimulated group, signifying greater recruitment of these cells from the circulation, the overall number of cells was low and the amount of differentially expressed genes was nominal.2
"The smart bandage significantly reduced infection in the wound, decreasing overall bacterial colony count and by continuous monitoring of wound impedance and temperature, their wireless smart bandage could detect early onset of infection and modulate treatment in a closed-loop manner to avoid further wound complications." 2
To specifically investigate macrophages and monocytes, we performed a series of evaluations to validate and define the high number of differentially expressed genes observed.2 First, we re-embedded our macrophages and monocytes and used cellular trajectory reconstruction analysis using gene counts and expression (CytoTRACE) to confirm that our defined monocytes possessed less differentiated cell states based on the distribution of unique messenger RNA transcripts.2 We then overlaid the stimulated and unstimulated macrophages and monocytes and performed RNA velocity and pseudo time analyses using scVelo and Monocle 3, respectively, to combine RNA velocity information with trajectory inference to compute a map of potential fates that the macrophages and monocytes can undergo in response to electrical stimulation.2 We first used scVelo to infer our root node and transcriptional directionality across the manifold, based on mRNA splicing of macrophages and monocytes. We found three general transcriptional vector paths in which mRNA splicing could occur within individual cells, with a relatively higher amount of differentiated individual cells found on the left of the embedding and less differentiated cells on the right, further confirming CytoTRACE.2 We then performed pseudo time analysis with Monocle 3,using a root node identified with scVelo to infer terminal cell states.2 Our analysis once again revealed three distinct transcriptional trajectories.2 To further understand why macrophages and monocytes had a higher amount of differentially expressed genes activated by our smart bandage, we performed uniform manifold approximation and projection (UMAP)-based clustering, which revealed five transcriptionally distinct clusters.2 Of the five clusters, cluster 0, consisting of both macrophages and unstimulated control cells, had a higher expression of genes such as JUN and FN1,60,61 which have previously been associated with wound healing, whereas clusters 1, 2 and 3, consisting predominantly of stimulated monocytes and macrophages,
demonstrated elevated expression of genes involved in the wound repair process, such as CD74, SELENOP, APOE, MRC1, CD163 and FABP5.2,36-9 When we looked at the stimulated and control feature plots of highly expressed genes in macrophages and monocytes, we saw that cells with a strong enrichment for previously reported proregenerative markers, notably CD163 and MRC1 (CD206), as well as SELENOP and APOE, all localized around Seurat cluster 2 and trajectory 2 (middle), which primarily contained stimulated macrophages.2 CD163 and MRC1 (CD206) have previously been described as M2 anti-inflammatory macrophage markers40 while SELENOP has been found to be anti-inflammatory, regulating macrophage invasiveness and other inflammatory mediators responsible for pathogen clearance and tissue repair, and is linked to M2 macrophage markers such as STAB1, SEPP1 and ARG1.2 APOE has been also shown to enhance in vitro phagocytosis of macrophages,increasing muscle and soft tissue regeneration.2, 41-2 We further confirmed these transcriptional changes at the protein level, performing flow cytometry on GFP-positive cells circulating to wounds in our parabiosis model. We identified a higher percentage of CD163-positive cells in stimulated wounds as compared with controls.2 This was further confirmed by immunofluorescent staining of healed tissue, with significantly higher CD163 and CD206 expression observed in stimulated as compared with untreated wounds.2
These data suggest that electrical stimulation may drive macrophages towards a more regenerative phenotype, and could underlie the accelerated wound healing observed in our preclinical studies.2 The high predominance of regenerative macrophages could, in part, be due to macrophages responding to local micro environmental stimuli. Modulation of the cell membrane electric potential with electrical stimuli could activate more ATP-sensitive potassium ion channels, which has previously
“To specifically investigate macrophages and monocytes, we performed a series of evaluations to validate and define the high number of differentially expressed genes observed.” 2
been shown to affect macrophage differentiation plasticity and function.2,43-4 Taken together, our pre-clinical studies attribute one mechanism by which electrical stimulation may coordinate and regulate macrophage functions, including those essential for microbial clearance and wound healing. Our smart bandage, in turn, will enable further biological discovery and allow for researchers to explore hypotheses previously less well studied due to current treatment modality limitations and animal model complexities.2
1. https://www.bjfm.co.uk/spotlight-on-managing-chronic-wounds-diabetic-foot-ulcersand-pressure-ulcers#:~:text=There%20are%20approximately%202.2%20million,and%20 %C2%A3531%20million%2C%20respectively. Chadwick P. Spotlight on managing chronic wounds, diabetic foot ulcers and pressure ulcers. British Journal of Family Medicine, 25/6/20
2. Jiang Y et al. Wireless, closed-loop, smart bandage with integrated sensors and stimulators for advanced wound care and accelerated healing. Nat Biotechnol (2022).
3. McLister A et al.New developments in smart bandage technologies for wound diagnostics. Adv. Mater. 28, 5732–5737 (2016).
4. Derakhshandeh H et al. Smart bandages: the future of wound care. Trends Biotechnol. 36, 1259–1274 (2018)
5. Long Y. et al. Effective wound healing enabled by discrete alternative electric fields from wearable nanogenerators. ACS Nano 12, 12533–12540 (2018).
6. Liu A et al. Accelerated complete human skin architecture restoration after wounding by nanogenerator-driven electrostimulation. J. Nanobiotechnol. 19, 280 (2021).
7. Farahani M, Shafiee A. Wound healing: from passive to smart dressings. Adv. Healthc. Mater. 10, e2100477 (2021)
8. Dincer, C. et al. Disposable sensors in diagnostics, food, and environmental monitoring. Adv. Mater. 31, e1806739 (2019)
9. Barros Almeida I et al. Smart dressings for wound healing: a review. Adv. Skin Wound Care 34, 1–8 (2021).
0. Kekonen A. et al. Bioimpedance sensor array for long-termmonitoring of wound healing from beneath the primary dressingsand controlled formation of H2O2 using low-intensity directcurrent. Sensors 19, 2505 (2019).
11. Lukaski HC, Moore M. Bioelectrical impedance assessment ofwound healing. J. Diabetes Sci. Technol. 6, 209–212 (2012).
12. Chanmugam, A. et al. Relative temperature maximum in woundinfection and inflammation as compared with a control subjectusing long-wave infrared thermography. Adv. Skin Wound Care30, 406–414 (2017).
13. Tamayol A. et al. Flexible pH-sensing hydrogel fibers forepidermal applications. Adv. Healthc. Mater. 5, 711–719 (2016).
14. Xu G et al. Battery‐free and wireless smart wound dressingfor wound infection monitoring and electrically controlled on‐demand drug delivery. Adv. Funct. Mater. 31, 2100852 (2021).
15. Trung TQ et al. Anall-elastomeric transparent and stretchable temperature sensorfor body-attachable wearable electronics. Adv. Mater. 28,502–509 (2016).
16. Hattori, Y. et al. Multifunctional skin-like electronics forquantitative, clinical monitoring of cutaneous wound healing.Adv. Healthc. Mater. 3, 1597–1607 (2014).
17. ShiX, Wu P. A smart patch with on-demand detachableadhesion for bioelectronics. Small
17, e2101220 (2021).
18. Pang, Q. et al. Smart flexible electronics-integrated wounddressing for real-time monitoring and on-demand treatment ofinfected wounds. Adv. Sci. 7, 1902673 (2020).
19. Marks H. et al. A paintable phosphorescent bandagefor postoperative tissue oxygen assessment in DIEP flapreconstruction. Sci. Adv. 6, eabd1061 (2020).
20. Swisher SLet al. Impedance sensing device enables earlydetection of pressure ulcers in vivo. Nat. Commun. 6, 6575 (2015).
21. McCafrey C et al. Flexible bioimpedancespectroscopy system for wound care monitoring.
In 2019 IEEEBiomedical Circuits and Systems Conference (BioCAS) 1–4 (IEEE,2019).
1. Department of Chemical Engineering, Stanford University, Stanford, CA, USA.
2. Department of Surgery, Division of Plastic and Reconstructive Surgery, Stanford University School of Medicine, Stanford, CA, USA.
3. Department of Biology, Stanford University, Stanford, CA, USA.
4. BOE Technology Center, BOE Technology Group Co., Ltd, Beijing, China.
5. Stanford Distinguished Careers Institute, Stanford University, Stanford, CA, USA.
6. Department of Genetics, Stanford University School of Medicine, Stanford, CA, USA.
7. Department of Surgery, University of Arizona College of Medicine, Tucson, AZ, USA.
8. Department of Materials Science and Engineering, Stanford University, Stanford, CA, USA.
9. These authors contributed equally: Yuanwen Jiang, Artem A. Trotsyuk, Simiao Niu.
Our preclinical work showed proof of concept, but future work must extend their smart bandage to a human-sized form factor and running preliminary tests in large-animal models followed by human trials.2 The manufacturing cost needs reduced to make this device affordable.2 The technology used in this device platform may be transferred and adapted for use in other diseases in bioelectronic medicine.2
22. Kalidasan V. et al. Wirelessly operated bioelectronic sutures forthe monitoring of deep surgical wounds. Nat. Biomed. Eng. 5,1217–1227 (2021).
23. Zhao Yet al. Skin‐Inspired antibacterial conductive hydrogels forepidermal sensors and diabetic foot wound dressings. Adv. Funct.Mater. 29, 1901474 (2019).
24. Ciani I et al. Development of immunosensors for direct detectionof three wound infection biomarkers at point of care usingelectrochemical impedance spectroscopy. Biosens. Bioelectron.31, 413–418 (2012).
25. Gao Y et al. A flexible multiplexed immunosensor forpoint-of-care in situ wound monitoring. Sci. Adv. 7, eabg9614(2021).
26. Thakral Get al. Electrical stimulation to accelerate woundhealing. Diabet. Foot Ankle 4, 22081 (2013).
27. Kloth LC Electrical stimulation technologies for wound healing.Adv. Wound Care 3, 81–90 (2014).
28. Zhao M et al. Electrical signals control wound healing throughphosphatidylinositol-3-OH kinase-gamma and PTEN. Nature 442,457–460 (2006).
29. Cohen DJ, Nelson WJ,Maharbiz MM. Galvanotactic controlof collective cell migration in epithelial monolayers. Nat. Mater.13, 409–417 (2014).
30. Liu Y et al. Soft and elastic hydrogel-based microelectronicsfor localized low-voltage neuromodulation. Nat. Biomed. Eng. 3,58–68 (2019).
31. Jiang Y et al. Topological supramolecular network enabledhigh-conductivity, stretchable organic bioelectronics. Science375, 1411–1417 (2022).
32. Negut I et al. Treatmentstrategies for infected wounds. Molecules 23, 2392 (2018)
33. Chen H. et al. Dissolved oxygen from microalgae-gel patchpromotes chronic wound healing in diabetes. Sci. Adv. 6,eaba4311 (2020).
34.Wu, J. & Yan, L. J. Streptozotocin-induced type 1 diabetes inrodents as a model for studying mitochondrial mechanisms ofdiabetic cell glucotoxicity. Diabetes Metab. Syndr. Obes. 8,181–188 (2015).
35. Duyverman AM et al. A transient parabiosis skin transplantation model in mice. Nat. Protoc. 7, 763–770 (2012).
36. Farr L, GhoshS, MoonahS. Role of MIF cytokine/CD74receptor pathway in protecting against injury and promotingrepair. Front. Immunol. 11, 1273 (2020).
37. Carlson, B. A. et al. Selenoproteins regulate macrophageinvasiveness and extracellular matrix-related gene expression.BMC Immunol. 10, 57 (2009).
38. Lin JD et al.Single-cell analysis of fate-mapped macrophagesreveals heterogeneity, including stem-like properties, duringatherosclerosis progression and regression. JCI Insight 4,e124574 (2019).
39. Huang ZH, Reardon CA, Mazzone T. Endogenous ApoEexpression modulates adipocyte triglyceride content andturnover. Diabetes 55, 3394–3402 (2006).
40. Martinez FO, Gordon S. The M1 and M2 paradigm ofmacrophage activation: time for reassessment. F1000Prime Rep.6, 13 (2014).
41. Arnold Let al. CX3CR1 deficiency promotes muscle repair andregeneration by enhancing macrophage ApoE production. Nat.Commun. 6, 8972 (2015).
42. Wang, Y. et al. Tissue-resident macrophages promoteextracellular matrix homeostasis in the mammary gland stroma ofnulliparous mice. eLife 9, e57438 (2020).
43. Li C, Levin M, Kaplan DL. Bioelectric modulation ofmacrophage polarization. Sci. Rep.
6, 21044 (2016).
44. Hoare JI et al. Electric fields are novel determinants of human macrophagefunctions. J. Leukoc. Biol. 99, 1141–1151 (2016).
“The technology used in this device platform may be transferred and adapted for use in other diseases in bioelectronic medicine.” 2