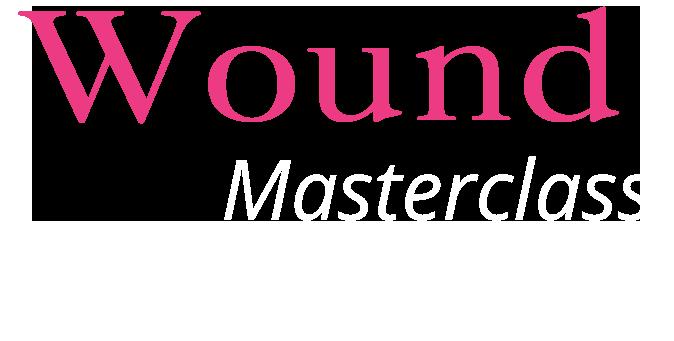
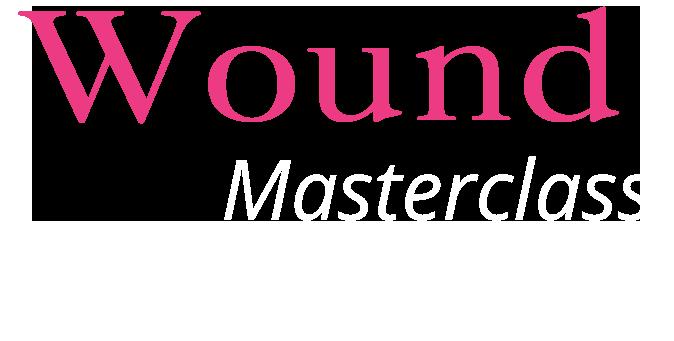
Paradigm Shift in Wound Care:
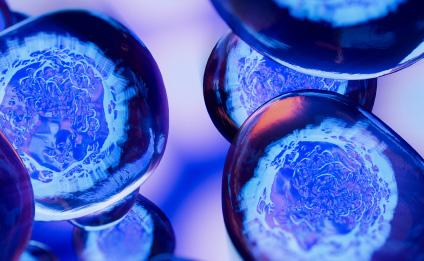

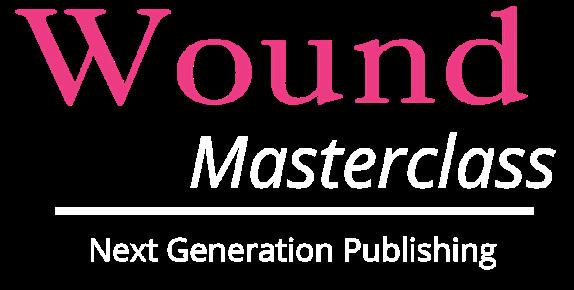
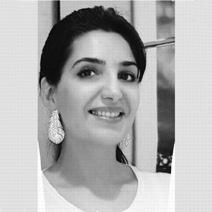
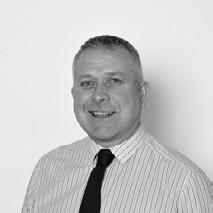
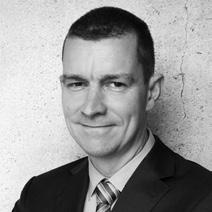
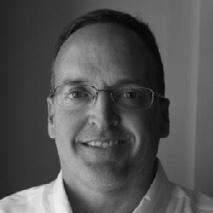
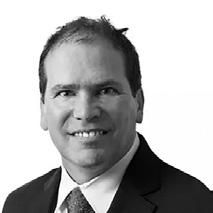
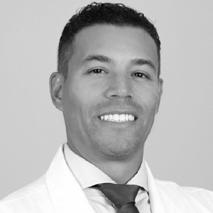
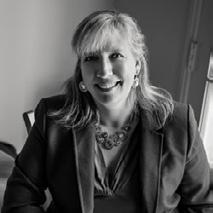
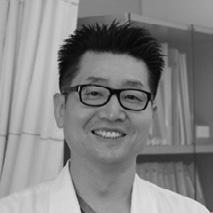
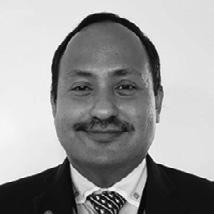
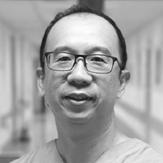
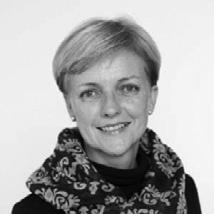
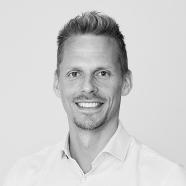
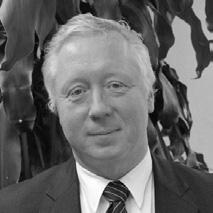
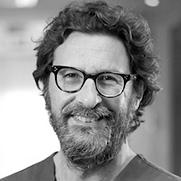
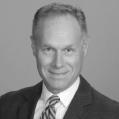
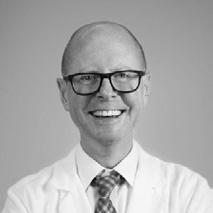
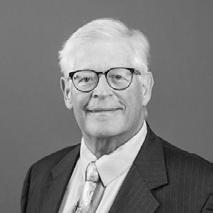
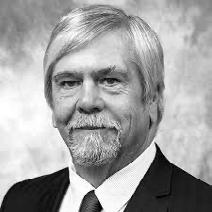
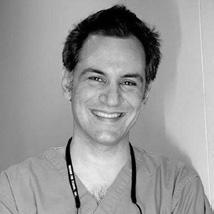
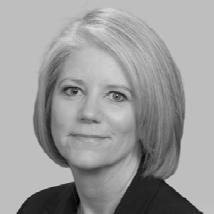
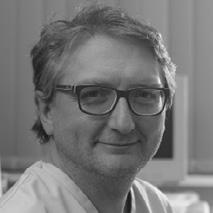
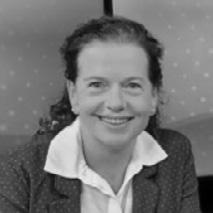
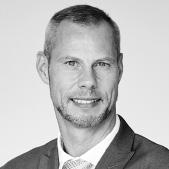
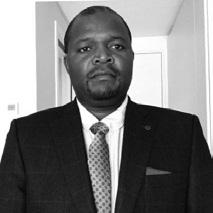
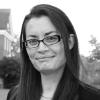
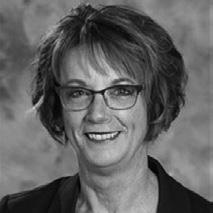
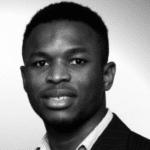
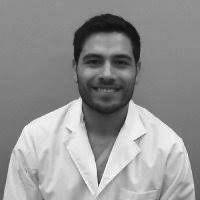
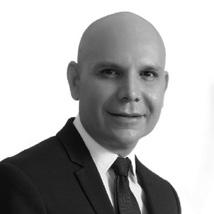
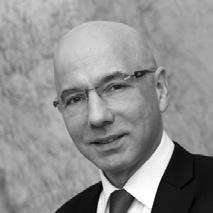
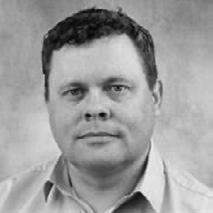
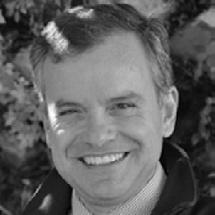

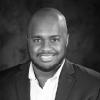
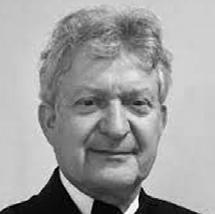
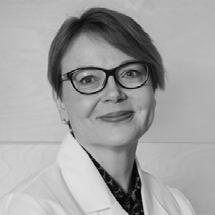
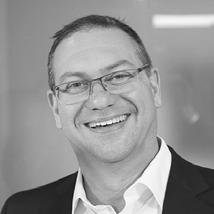
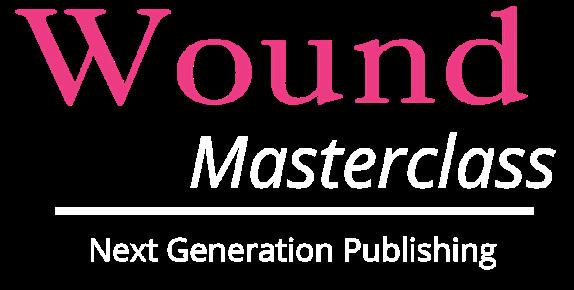
Chief Editor
Miss Negin Shamsian
Commercial Director
Mr Alec Wright
Contact Editor
editor@woundmasterclass.com
Commercial Inquiries
commercial@woundmasterclass.com
Article Submissions
submissions@woundmasterclass.com
Harnessing the Power of Bioactive Wound Care: Unlocking the Potential for Accelerated Healing | Dr Negin Shamsian
Advancing Regional Efforts Against Antimicrobial Resistance in the Americas: The Imperative for Collaborative Action | Dr Negin Shamsian

How Can A Biodegradable Matrix Offer Limb-Saving Options for Chronic Ischemia? | Ms Victoria Bristow
Complex Refractory Wounds: How to Overcome Treatment
Recalcitrance and Restore the Healing Trajectory Using Innovative Bioactive Glass | Dr Elvis Castillo Garcia, Dr Phuong Thuy Nguyen
Can Bioactive Glass Matrix be Used to Facilitate Pain Reduction and Healing for Patients with Pyoderma Gangerosum Ulcers? | Dr Luis A. Laurentin-Perez, Dr Donald W. Buck II, Mr Dagoberto Rivera
Published by
Clarus Communications Ltd., Oxford, United Kingdom
No part of this issue is to be copied or reproduced without permission of the publisher
© Clarus Communications Ltd.
This publication is intended for online distribution and this issue is not suitable for print in this form
To inquire about obtaining a printable version of this issue or any article therein, please contact the editor
Dermal Repair Powder: A New Option to Manage Irregular Wounds | Prof Dr Luca Dalla Paola
How Can Novel Bioactive Glass Wound Matrix Optimise Hard-to-Heal Venous Leg Ulcers in Geriatric Patients with Multiple Comorbidities? | Dr Martin L. Johnson, Ms Erianthe Ortega, Prof David G. Armstrong
How to Reduce Treatment Costs for Hard-to-Heal Wounds: The Bioactive Glass Wound Matrix Option| Dr P Rathinasamy, Dr JC Beckford
MasterSeries 60 Minutes Interactive: DFU Masterclass
MasterSeries 60 Minutes Interactive Convexity Masterclass
Bioelectric Stimulation, Electric Fields and the Current of Injury: Have We Been Overlooking One of the Most Important Coordinators of the Wound Healing Process? | Dr Robin Martin
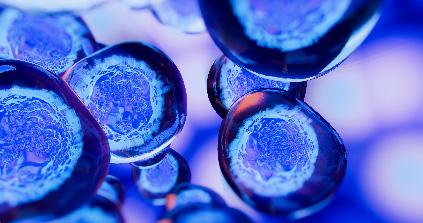
Masterclass GUIDES MOIST Concept
Bioactive Glass Wound Matrix
Wound
Randomised self-controlled trial published in the International Wound Journal
The geko™ device reduces pain1 and doubles the rate of healing in venous leg ulcers versus compression alone2
VLUs affect one in 500 adults in the UK 3 , costing the national healthcare system around £2 billion annually 3
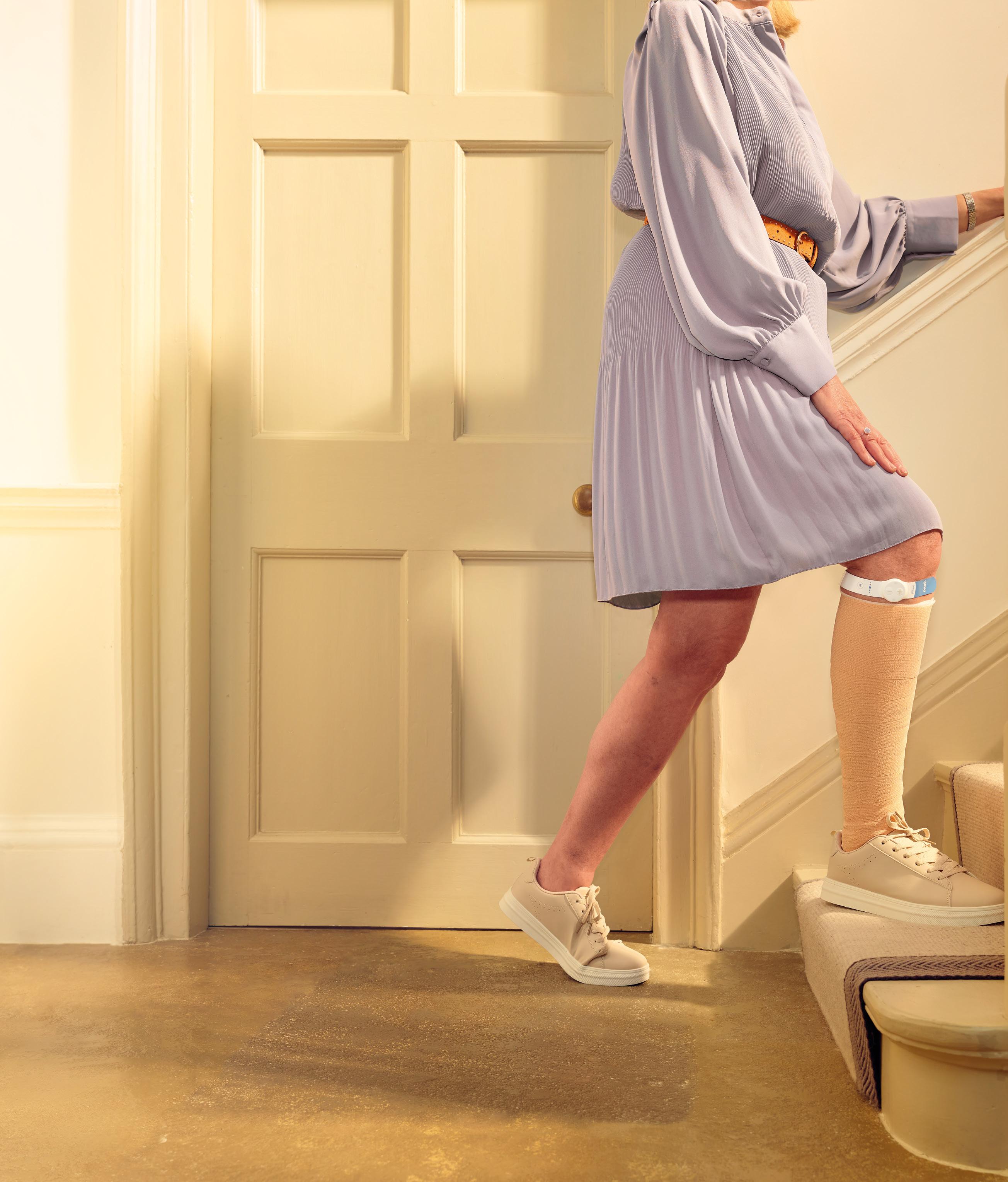
Reduces pain1
Accelerates healing2
Improves concordance5
Now available on prescription
The study compared standard of care with and without the geko™ device in patients with hard-to-heal VLUs2
VLUs, venous leg ulcers.
1. Jones N et al. Br J Nurs 2018; 27(20): S16-S21. 2. Bull, RH, Clements, D, Collarte, AJ, Harding, KG. The impact of a new intervention for venous leg ulcers: A withinpatient controlled trial. Int Wound J. 2023; 1- 9. doi:10.1111/iwj.14107. CJ, Humphreys, I, Thayer, D, et al. Cost of managing patients with venous leg ulcers. Int Wound J. 2020; 17: 1074– 1082. https://doi.org/10.1111/iwj.13366. Wound J 2021; 18(2): 187-93. 5. Harris et al. Evaluation of a muscle pump-activating device for non-healing venous leg ulcers 2017. Medicalhelplines.com Inc and John Wiley & Sons Ltd doi: 10.1111/iwj.12784. www.gekodevices.com MADWND0700v3
The geko™ device, a neuromuscular electrostimulator, increases venous, arterial and microcirculatory blood flow1 , transporting oxygenated blood to the wound bed accelerating wound healing4
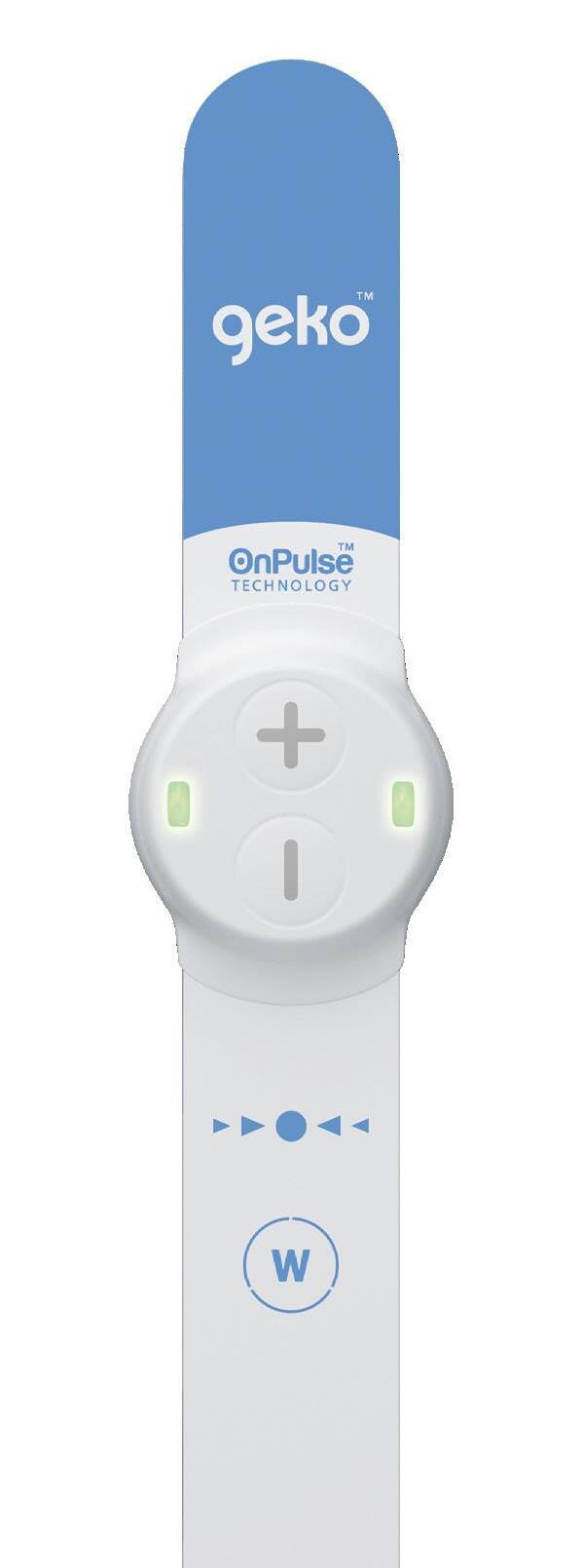
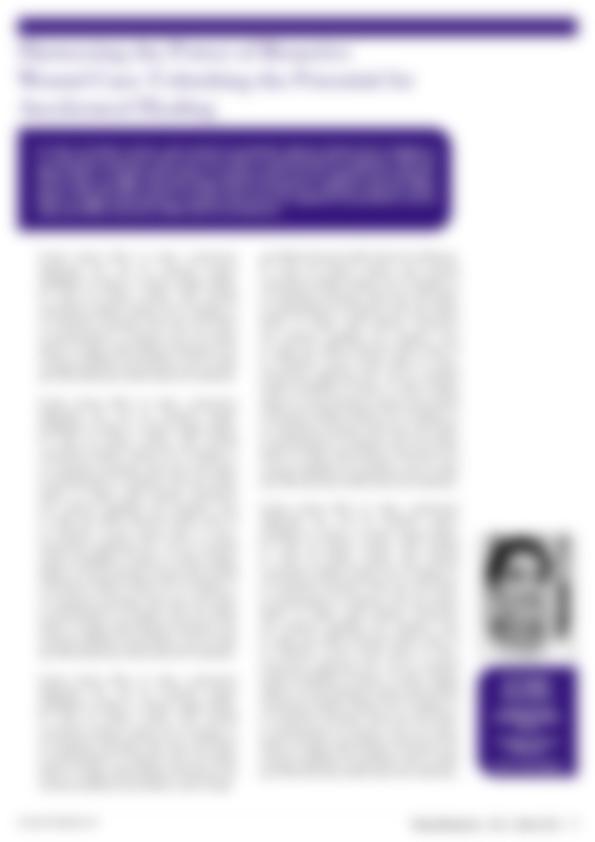
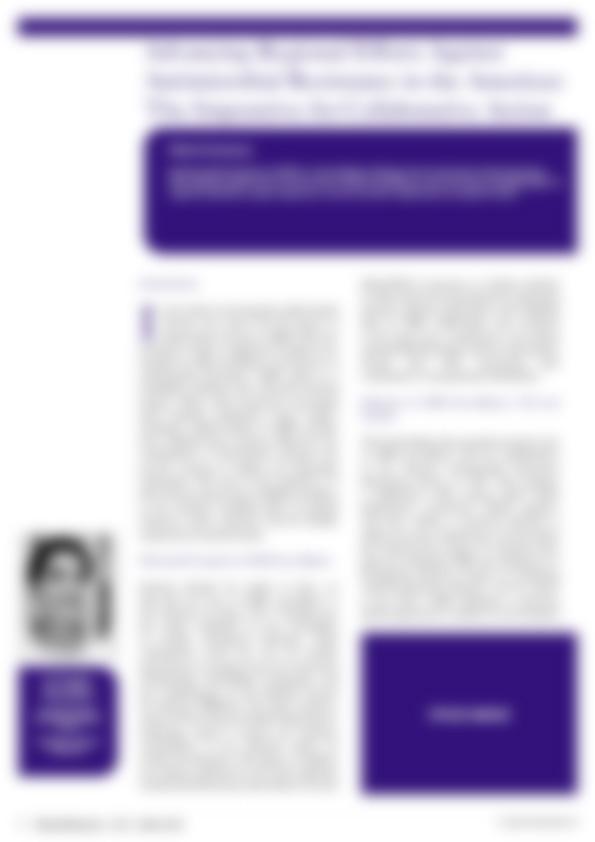
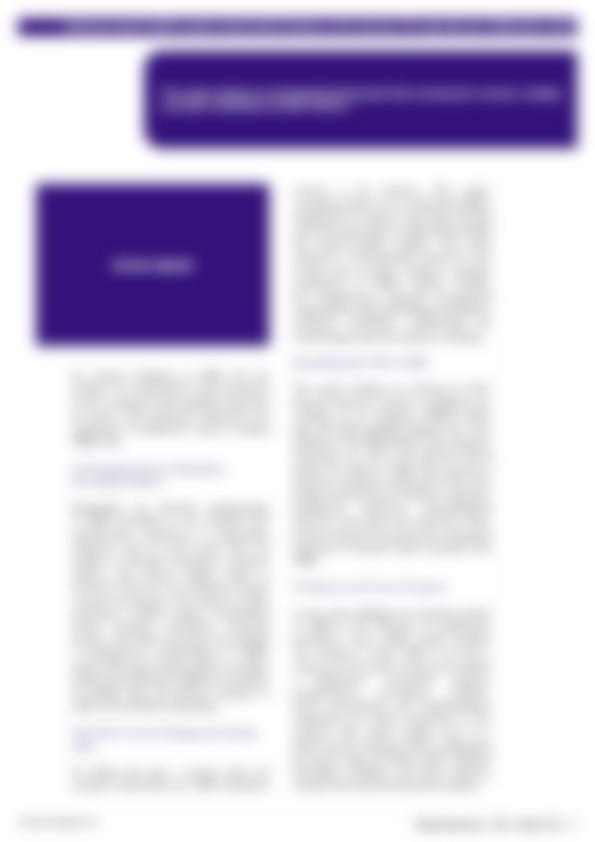
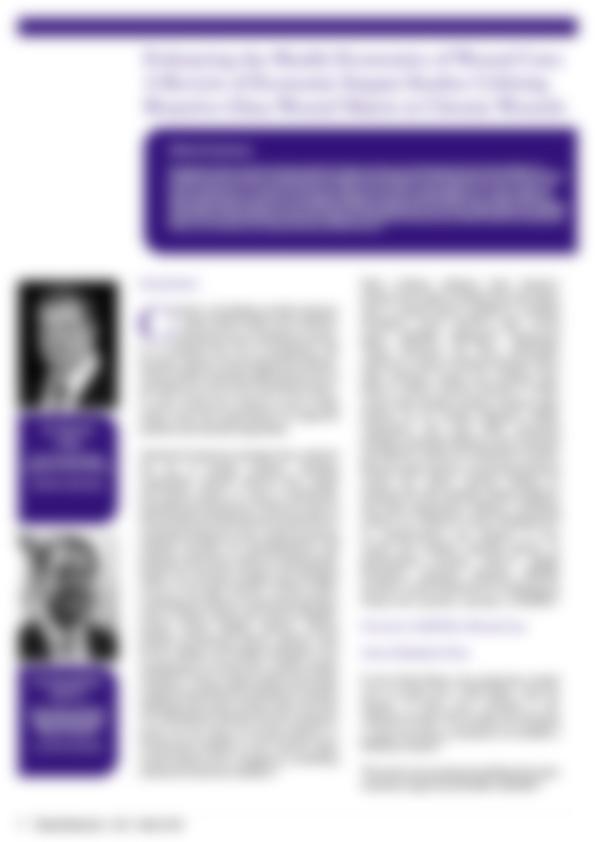
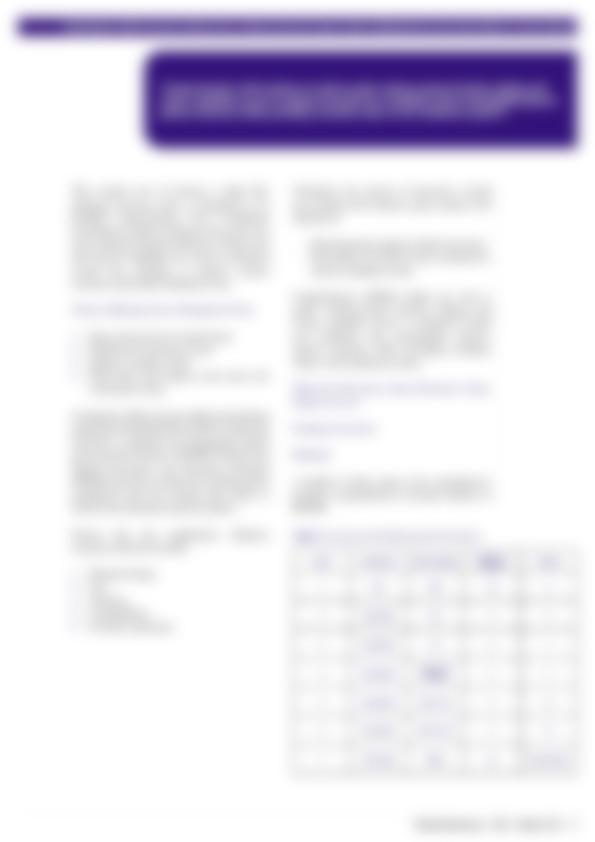
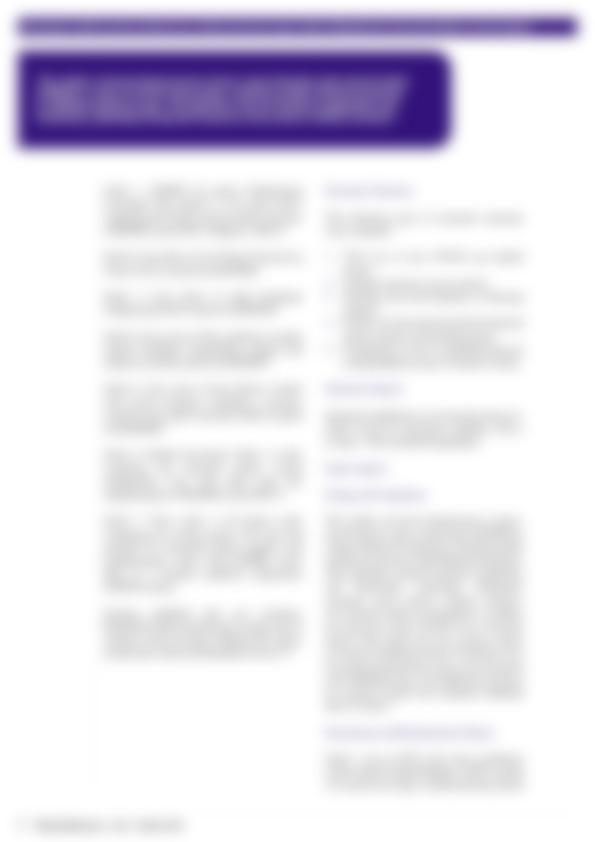
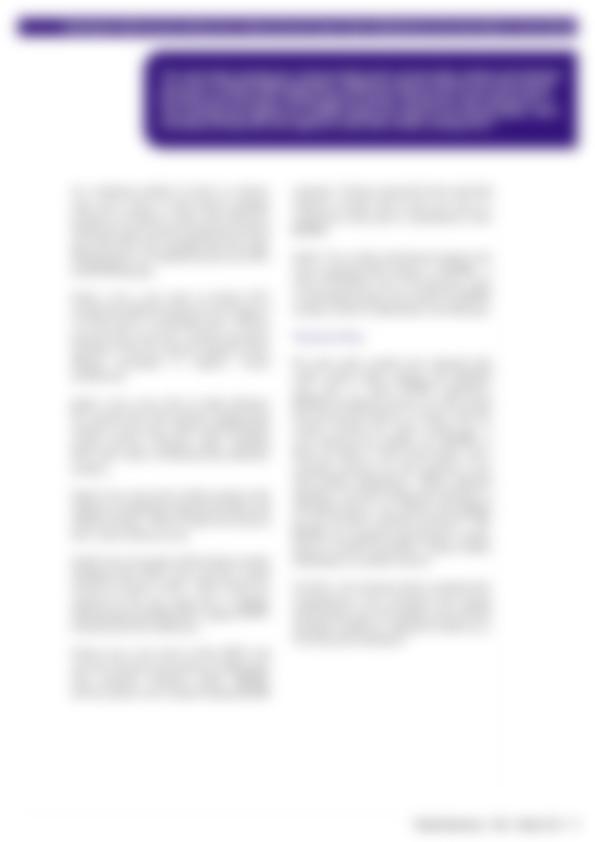
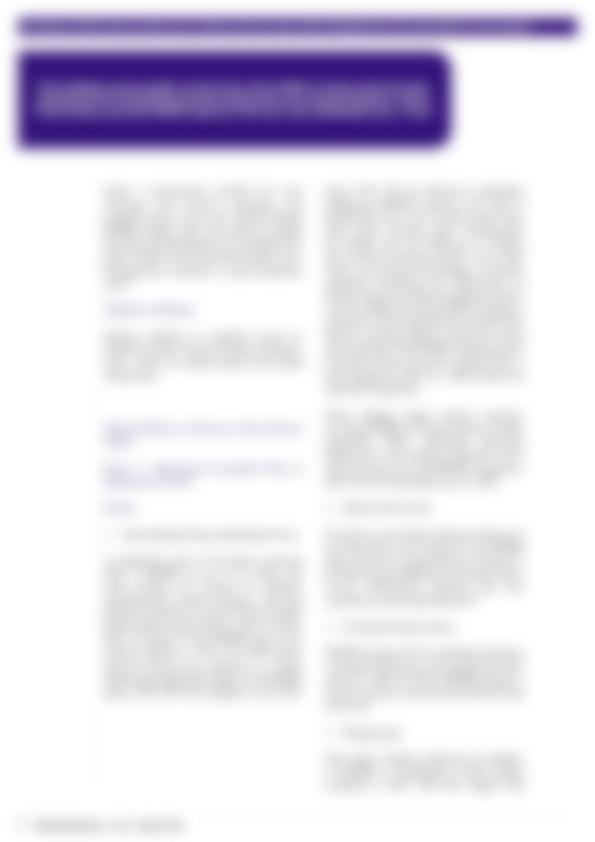
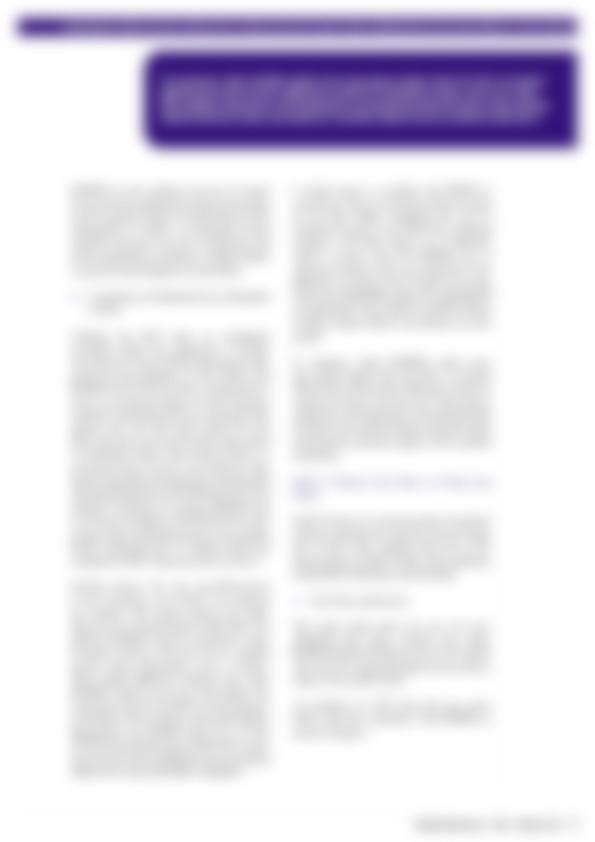
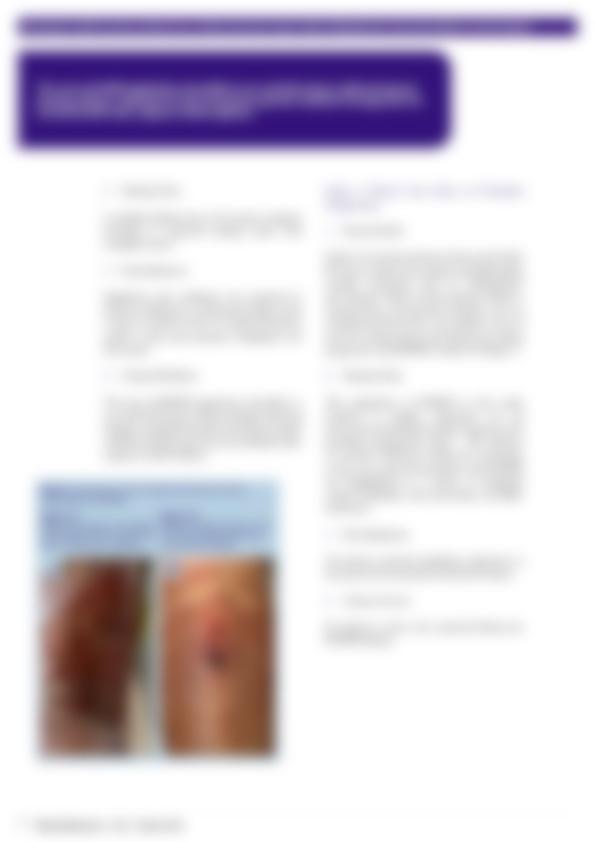
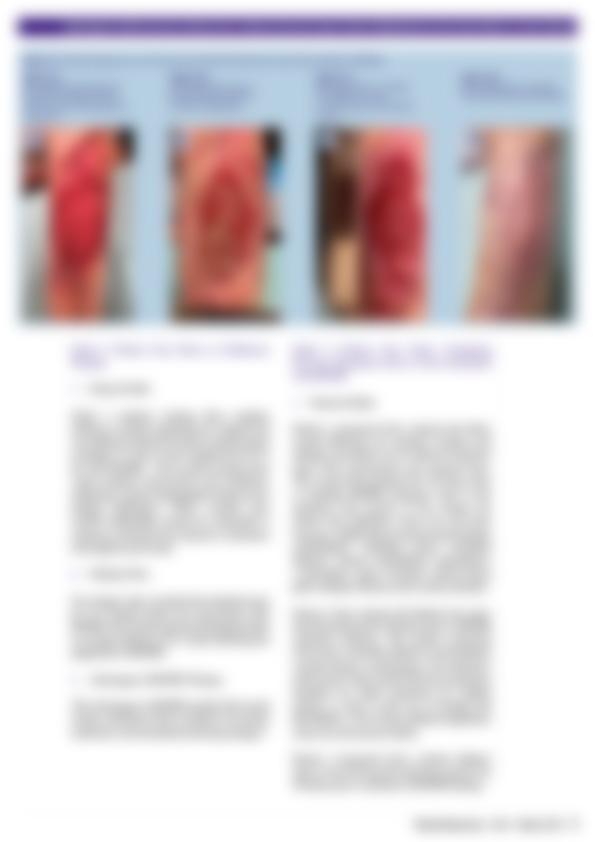
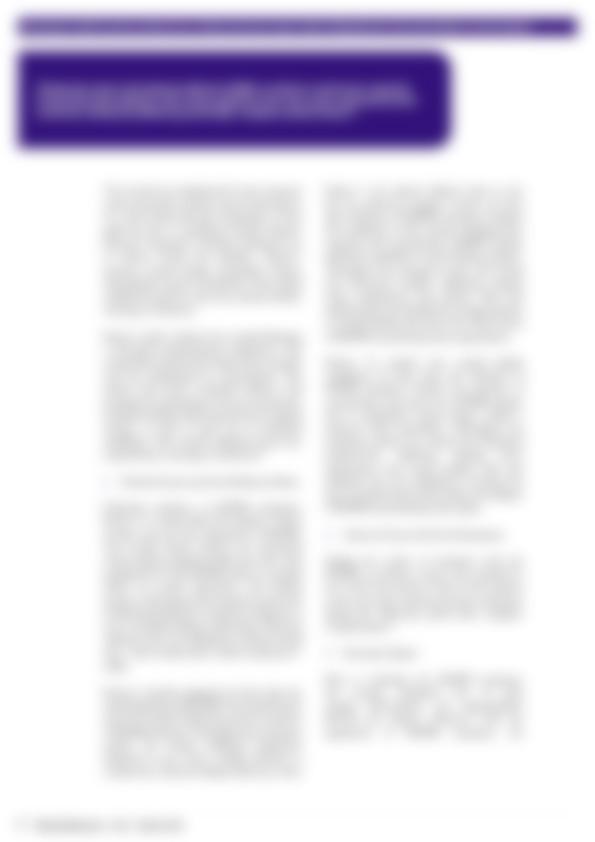
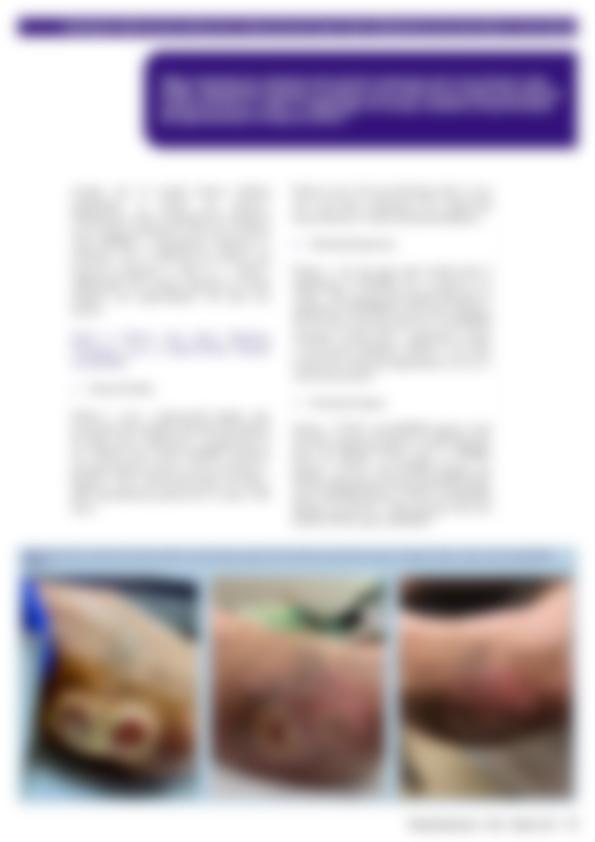

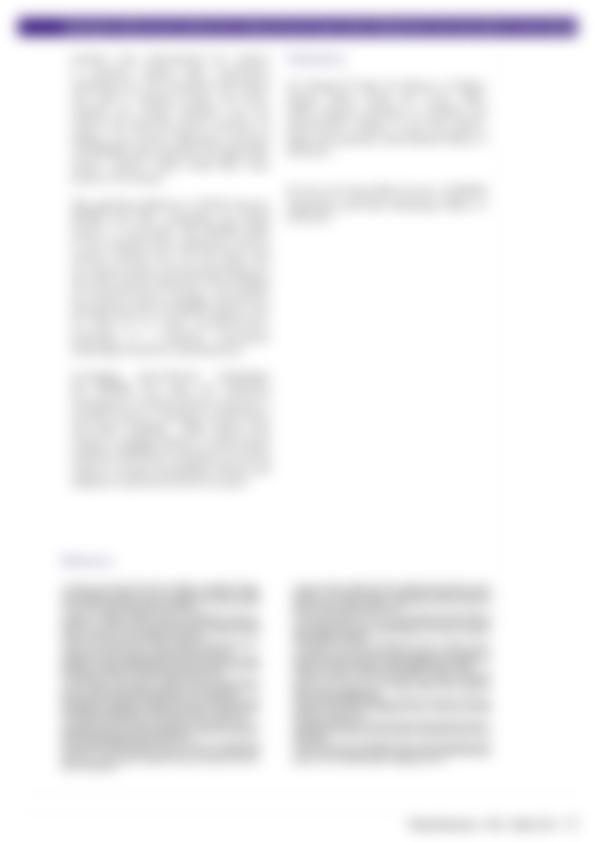
Bioactive glass: Clinically
Shown to Support Wound Closure
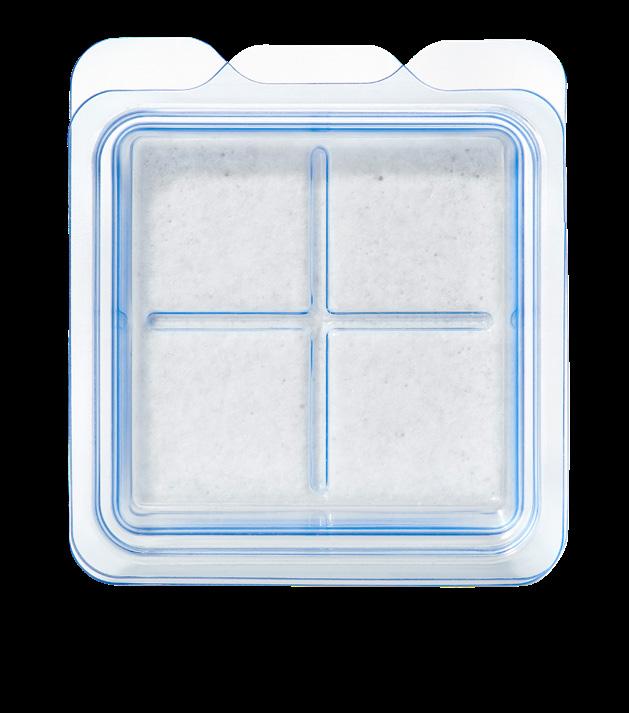
To learn more please visit our website at www.ets123.com.
79.4% vs. 36.5%1
In a randomized controlled trial, Mirragen doubled the wound area reduction as 12 weeks (79.4% vs. 36.5%) as compared to the standard of care (collagen alginate dressing).
2.8x1
Mirragen increased healing rate 2.8x vs. SOC in the 12 week trial.
0 infections1
SOC had 5 patients withdrew due to infection; Mirragen had 0 infections during the 12 week trial.
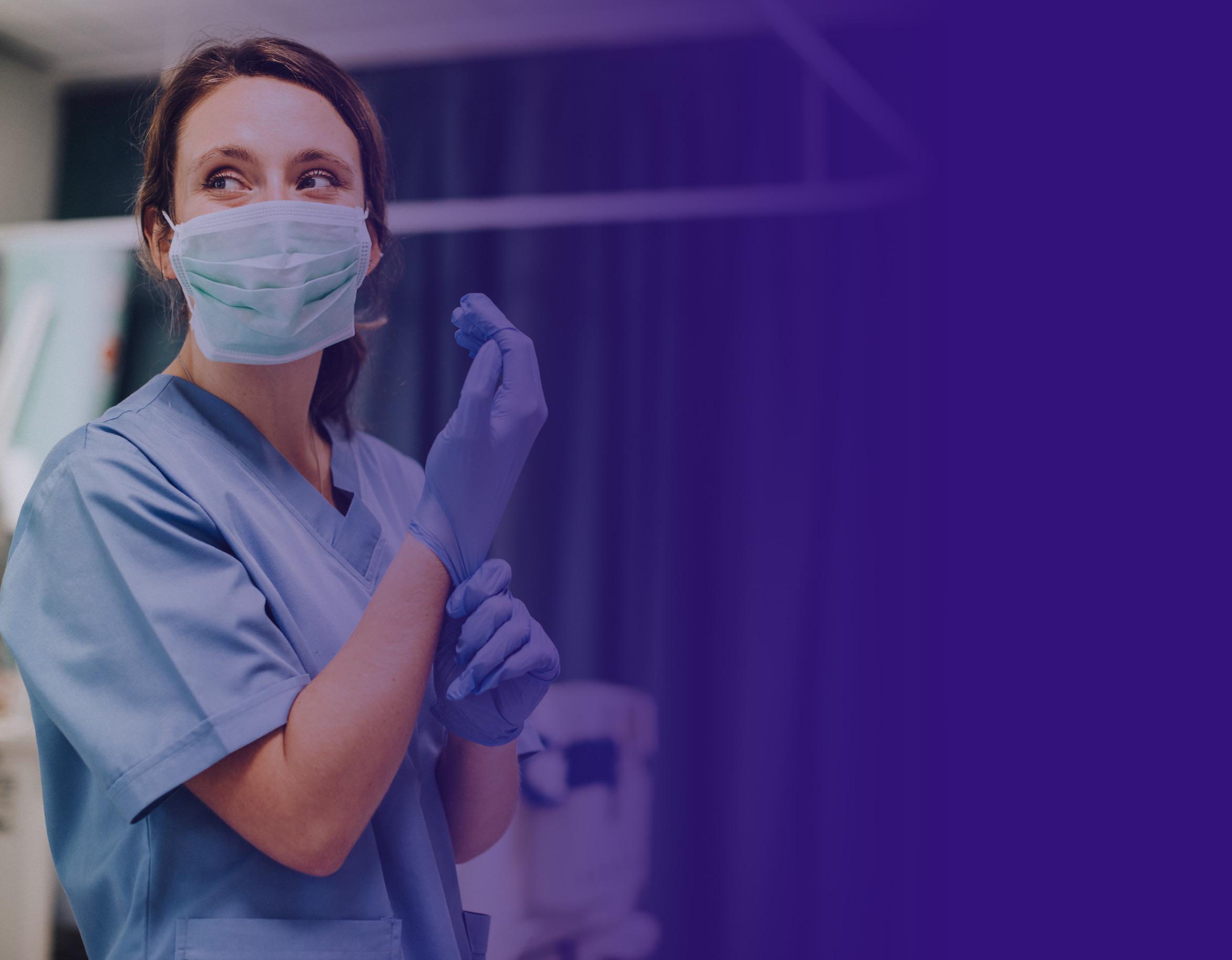
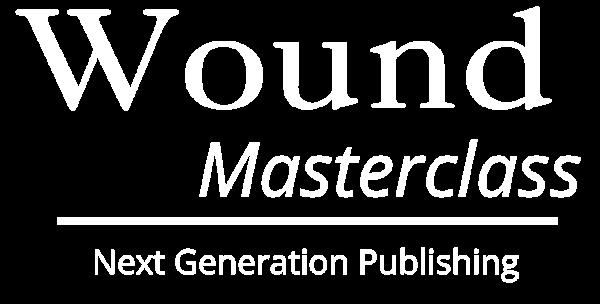
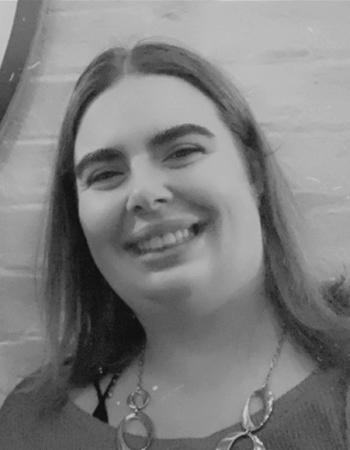
How Can A Biodegradable Matrix Offer
Limb-Saving Options for Chronic Limb Threatening Ischaemia?
Editorial Summary
Chronic limb-threatening ischaemia, marked by severely reduced blood flow, poses a major wound healing challenge and threat of amputation.1-3 Emerging biotechnology however brings new promise through biodegradable matrices. These innovative extracellular matrix products aim to stimulate angiogenesis and revascularization in limbs previously deemed unsalvageable.4 Findings suggest biodegradable temporising matrices (BTM), properly integrated with standard of care, can achieve significant wound closure and limb salvage.
Introduction
For wound specialists, chronic limb ischaemia is among our most recalcitrant diagnoses - often resistant even to goldstandard revascularization techniques. These limb-threatening conditions result from severe arterial blockages depriving tissues of sufficient perfusion for cell viability and healing. Critical limb ischaemia (CLI), also known as chronic limb-threatening ischaemia (CLTI), represents the most advanced state of peripheral arterial disease (PAD) and manifests as rest pain, nonhealing ulcers, and/or gangrene. Ulceration, infection, and necrosis abound as oxygen saturations plunge perilously below the 3050mmHg required for remodeling. More than 10% of patients with PAD may develop CLTI, and 55% of patients hospitalised with CLTI are readmitted within one year. It is vital that these patients are identified early in our clinical practice. 1-4
It is reported 10% of PAD patients may progress to develop CLTI, the most severe form of PAD. PAD itself is very common, affecting over 200 million people worldwide, so this translates to a large number of patients at risk for CLI. 2
Readmission Rates
The one-year readmission rate for patients hospitalized with CLTI is 55%, which indicates the chronic and complex nature of this condition. Readmissions are often due to recurrent pain, non-healing wounds, infections, or other complications.3
Importance of Early Identification:
Identifying PAD patients who are progressing toward CLTI is crucial for preventing amputations, disability, and death. Earlier diagnosis allows for more aggressive risk factor modification (e.g. smoking cessation, glycaemic control in diabetes) and treatment (e.g. revascularization procedures).
Rest pain
Numbness
Skin changes: shiny, smooth or dry skin
Thickening of toenails
Absent/diminished pulses legs/feet
Non-healing ulcers
Gangrene
All clinicians should assess for PAD signs/ symptoms like claudication, non-healing ulcers, absent pulses, and incorporate tools like the ankle-brachial index, or the use of toe pressures.
In summary, CLTI has a high prevalence and identifying these high-risk patients early is essential for improving outcomes and reducing the very high rates of readmission and complications. Increasing awareness of CLI among clinicians across specialties who care for patients with vascular disease is an important priority. 1-3
Ms Victoria Bristow
Vascular Specialist Nurse,
Table 1: Features of Critical Limb Ischaemia.
How
“Achieving adequate wound coverage and promoting healing in complex wounds remains a significant challenge in surgical practice. Biodegradable Temporising Matrix (BTM) is a synthetic dermal scaffold that has gained increasing attention in recent years due to its unique properties and potential benefits in wound management. Evidence has been well established to support studies on the applications and outcomes of BTM in various clinical settings, including burns, trauma, and chronic wounds."
The Reconstructive Challenge: What is the evidence for BTM?
Achieving adequate wound coverage and promoting healing in complex wounds remains a significant challenge in surgical practice. NovoSorb® Biodegradable Temporising Matrix (BTM) (PolyNovo Biomaterials Pty Ltd., Port Melbourne, VIC, Australia) is a synthetic dermal scaffold that has gained increasing attention in recent years by clinicians due to its unique properties and potential benefits in wound management. Evidence has been well established to support studies on the applications and outcomes of BTM in various clinical settings, including burns, trauma, and chronic wounds. 4-18
BTM is composed of a biodegradable polyurethane foam with a outer sealing membrane, designed to provide a temporary wound coverage while allowing for the development of a neodermis. 4,18
Mechanism of Action:
BTM acts as a temporary dermal scaffold, providing a protective barrier and a supportive matrix for the ingrowth of host cells and neovascularization. The porous structure of the BTM allows for the migration of fibroblasts and endothelial cells, promoting the formation of a neodermis.15 As the wound heals, the BTM gradually biodegrades, leaving behind a wellvascularized bed suitable for skin grafting or secondary wound closure. 4,18
Applications in Burns:
The use of BTM in the management of burn injuries has been extensively studied. In a retrospective case review by Greenwood et al., BTM was used in the reconstruction of fullthickness burns wounds. 15
The authors reported successful wound
closure and favorable long-term outcomes, with minimal complications and good cosmetic results. BTM has been studied reasonably extensively in patients with burns, necrotising fasciitis and, more recently, in diabetic foot disease.
In a comparative study by Selig et al., BTM was compared to split-thickness skin grafting (STSG) in the treatment of deep partial and fullthickness burns.19 The authors found that BTM was associated with reduced pain, improved scar quality, and better patient satisfaction compared to STSG. These findings suggest that BTM may offer advantages over traditional burn management techniques in select patient populations. 19
Applications in Reconstructive Surgery:
BTM has also been utilized in the management of and in reconstructive surgical procedures. In a retrospective review by Wagstaff et al., BTM was used in the reconstruction of free flap donor sites in a variety of surgical procedures, including head and neck reconstruction, breast reconstruction, and extremity reconstruction. The authors found that BTM was associated with reduced donor site morbidity, improved healing times, and better cosmetic outcomes compared to traditional donor site management techniques. 17
Applications in Chronic Wounds:
The use of BTM in the management of chronic wounds, such as diabetic foot ulcers and venous leg ulcers, has emerging evidence suggesting that BTM may offer benefits in this patient population.
“This dermal matrix can cover important structures to maintain structural function such as vessels, joint capsules, bone and tendons. This is important in vascular patients to enable them to continue to be mobile avoiding major limb amputation which could then lead to a longer hospital admission and for some, loss of independent living. The matrix is initially placed over the defect to create a neodermis.”
Review of Clinical Case
BTM is a dermal matrix that is commonly used in extensive and hard to heal wounds. Dermal matrices can cover important structures to maintain structural function such as vessels, joint capsules, bone and tendons. These are important in vascular patients to enable them to continue to be mobile avoiding major limb amputation which could then lead to a longer hospital admission and for some, loss of independent living. The matrix is initially placed over the defect to create a neodermis.
A 62-year-old male presented to the emergency department (ED) with extensive tissue necrosis involving the dorsum of the foot, multiple toes, and the calf as shown in Figure 1. Consent was gained from the patient to share photos in this case review. Physical examination revealed a palpable femoral pulse on the right leg, with no distal pulses palpable. The patient and his daughter reported three prior missed diagnoses at a local district hospital. The patient was admitted to the vascular ward for further evaluation and management. Revascularization was achieved through iliac angioplasty and a femoral-to-femoral crossover graft using polytetrafluoroethylene (PTFE).
Given the extensive tissue loss, the team deliberated on the salvageability of the limb. The patient was discharged home to allow for further demarcation, with regular follow up as an outpatient.
Having recently attended a presentation on the use of Biodegradable Temporising Matrix (BTM) in burn patients, a consultant colleague and I proposed this novel approach. The patient was counseled on the innovative nature of this treatment and the potential for limb salvage, with the understanding that a belowknee amputation would be the alternative. The patient expressed a strong desire to attempt limb preservation. During outpatient follow-up, sharp debridement was performed, revealing the eschar's role in protecting vital structures such as tendons, bone, and blood vessels. The patient was scheduled for BTM application under general anesthesia in a sterile operating theatre setting.
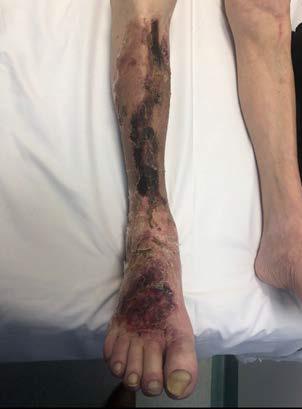
Figure 1: Right Lower Leg showing extensive necrosis.
How Can A Biodegradable Matrix
“The matrix was applied in the operating theatre under general anaesthesia in full sterile conditions. With the BTM the wound needs to be able to bleed in a controlled manner through the matrix to encourage angiogenesis and create a neodermis.”
The necrosis continued to demarcate as shown in Figure 2 and the patient underwent amputation of some toes. Intraoperatively, the BTM was applied to the dorsum of the foot and calf, allowing for controlled bleeding through the matrix to promote angiogenesis and neodermis formation as shown in Figure 3. The matrix was secured with staples, and negative pressure wound therapy (NPWT) was employed for protection and exudate management.
Weekly dressing changes were conducted for seven weeks, with wound photography and NPWT replacement on the forefoot and posterior calf. Visible wound contraction was noted. At week seven, the BTM was delaminated by removing the staples and peeling back the sealing membrane. Upon removal, some slough
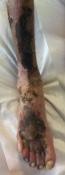
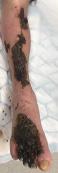
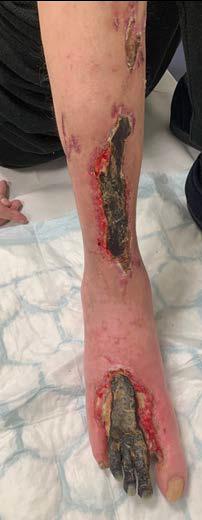
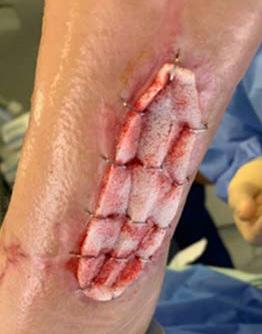
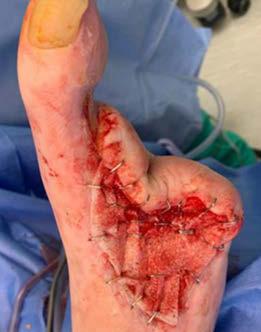
tissue and islands of epithelialization were present. Simple dressings were then applied with silver as the primary dressing as per the instruction for use from the manufacturers. This was carried out by district nurses until week 24 at which the wound had fully healed.
The patient continued to be monitored closely in the outpatient setting until fully healed. This patient highlights the potential for BTM to facilitate limb salvage in CLTI patients with extensive tissue loss, alongside traditional revascularization methods. Careful patient selection, multidisciplinary collaboration, and close post-operative monitoring are essential for the successful implementation of this innovative approach.
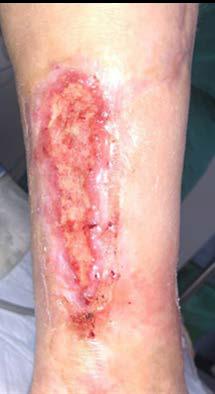
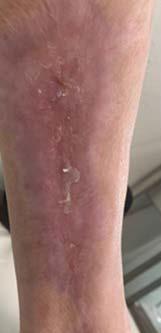
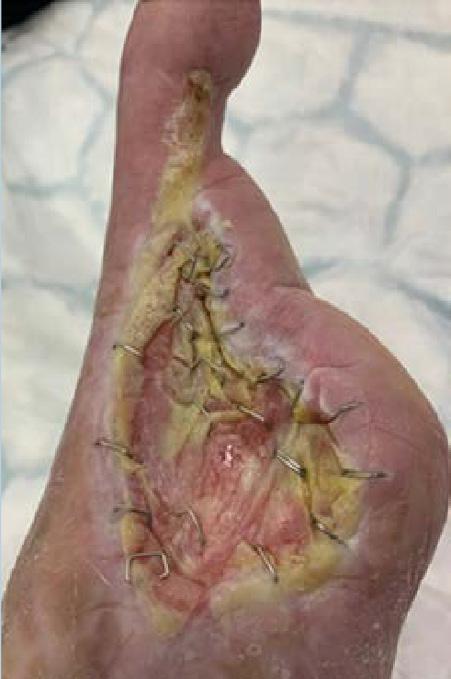
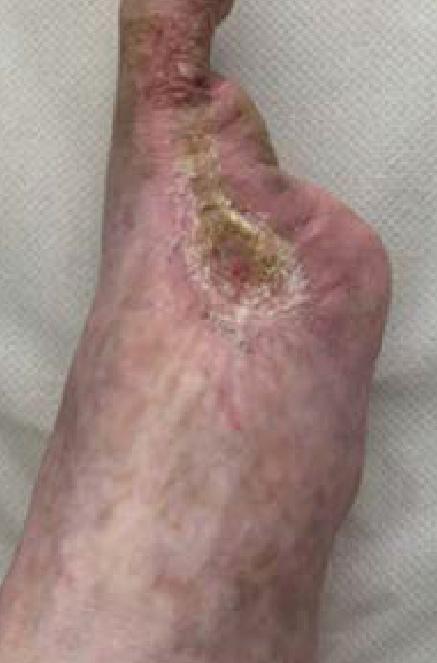
Figure 2: Overview of the necrosis demarcation.
Figure 3: Fixation of BTM with staples after wound debridement.
Figure 4: Overview of healing progression.
“Conventional treatments, such as endovascular angioplasty and bypass surgery, often fail to adequately restore distal perfusion, necessitating innovative solutions to promote wound healing and prevent limb loss. Biodegradable temporising matrix (BTM) has emerged as a promising adjunct therapy for limb salvage in CLTI patients with extensive tissue loss."
Discussion
CLTI, the most advanced stage of PAD, presents a significant challenge for wound care professionals. Characterized by the triad of rest pain, non-healing ulcers, and gangrene, CLTI results from severe arterial obstruction, leading to critically reduced tissue perfusion. This clinical scenario also has an added higher risk of amputation and mortality, and high rehospitalization rates, .1-3
Conventional treatments, such as endovascular angioplasty and bypass surgery, often fail to adequately restore distal perfusion, necessitating innovative solutions to promote wound healing and prevent limb loss. Biodegradable temporising matrix (BTM) has emerged as a promising adjunct therapy for limb salvage in CLTI patients with extensive tissue loss.4
Reconstructive Methods in CLTI
The management of CLTI involves a multidisciplinary approach, with the primary goal of restoring adequate perfusion to the affected limb and promoting wound healing. Revascularization techniques, such as endovascular angioplasty and bypass surgery, are the mainstay of treatment for CLTI. However, these interventions may not always be successful in restoring distal perfusion, particularly in patients with extensive tissue loss and poor distal runoff.17
Additional Reconstructive Methods Include:
Split-thickness skin grafting (STSG): STSG involves harvesting a thin layer of skin from a donor site and applying it to the wound bed. While STSG can be effective in promoting
wound closure, it requires a well-vascularized wound bed and may result in donor site morbidity and suboptimal cosmetic outcomes.
Local flaps: Local flaps involve mobilizing adjacent tissue to cover the wound defect. This technique can provide better cosmetic results and maintain sensation in the affected area. However, local flaps may not be feasible in patients with extensive tissue loss or compromised vascularity in the surrounding tissue. 16
Free flaps: Free flaps involve harvesting tissue from a distant site and transferring it to the wound defect using microvascular techniques. This method can provide well-vascularized tissue coverage but requires specialized surgical expertise and may be associated with longer operative times and higher complication rates. 18
Dermal substitutes: Dermal substitutes, such as acellular dermal matrices (ADMs) and BTM, provide a temporary scaffold for neodermis formation and promote wound healing. These products can be used in combination with STSG or as standalone treatments, depending on the wound characteristics and patient factors. 1-18
BTM in CLTI Reconstruction
Novosorb® BTM is a synthetic dermal scaffold designed to provide temporary wound coverage while facilitating the development of a neodermis.4 The porous structure of BTM allows for cellular migration and neovascularization, promoting the formation of a well-vascularized wound bed suitable for skin grafting or closure.4 Several studies have demonstrated the effectiveness of BTM in various clinical settings, including burns, trauma, and chronic wounds. 1-18
BTM may reduce the risk of amputation and improve patient outcomes in CLTI. 21
How Can A Biodegradable Matrix
“In the context of CLTI, BTM may offer several advantages over traditional reconstructive methods. Firstly, BTM can be applied directly to the wound bed, even in the presence of exposed tendons, bones, or hardware, providing a suitable environment for wound healing. Secondly, BTM does not require a separate flap donor site, reducing the risk of donor site morbidity and improving patient comfort.”
In the context of CLTI, BTM may offer several advantages over traditional reconstructive methods. Firstly, BTM can be applied directly to the wound bed, even in the presence of exposed tendons, or bones, providing a suitable environment for wound healing.4 Secondly, BTM does not require a separate free flap donor site, reducing the risk of donor site morbidity and improving patient comfort.4 Thirdly, BTM can be used in combination with other reconstructive methods, such as STSG, to improve wound healing outcomes and reduce the need for additional surgical interventions. 4 This patient presented demonstrates the successful application of BTM in a patient with CLTI and extensive tissue loss. Following revascularization and debridement, BTM was applied to the wound bed, allowing for bleeding through the matrix to encourage angiogenesis and neodermis formation. Negative pressure NPWT was used for protection and exudate control. After seven weeks, the graft was delaminated, revealing granulation tissue with islands of epithelialization. Complete wound healing was achieved at 24 weeks.
This case highlights the potential of BTM to aid wound healing in revascularized CLTI patients with extensive tissue loss, particularly when exposed tendons and bones are present by providing a suitable environment for wound healing and promoting neovascularization,
Challenges and Future Directions
Despite the promising results of BTM in various clinical settings, its widespread adoption in managing CLTI wounds remains challenging. Robust randomized controlled trials clarifying optimal patient selection, timing of application, and product choice are required . Furthermore, the integration of BTM into standard wound care protocols requires a multidisciplinary approach, with close collaboration between vascular surgeons, wound care specialists, and
other healthcare professionals.
BTM represents a promising solution for limb salvage in CLTI patients with extensive tissue loss, offering a viable option when conventional revascularization techniques have failed. By providing a temporary scaffold for neodermis formation and promoting neovascularization, BTM can aid in wound healing and may avoid amputation. The case presented highlights the potential to improve patient outcomes, reduce amputation rates, shorter hospital stays, and improve quality of life for patients.
As the field of regenerative medicine advances, BTM is very likely to play an increasingly important role in the management of complex wounds and the prevention of limb loss in CLTI patients. However, further research is needed to establish the optimal use of BTM in CLTI management and to address the challenges of integrating this innovative therapy into standard wound care protocols. By expanding our understanding of BTM and its role in CLTI reconstruction, we can improve patient outcomes and reduce the burden of this debilitating condition on patients and healthcare systems alike. Innovations in regenerative medicine using biodegradable matrices to deliver progenitor cells/proteins restoring the microcirculation offer glimmers of new hope but meaningful real-world integration remains challenging. Robust randomised controlled trials clarifying optimal patient selection, timing of application and product choice are sparse. Thoughtful, multidisciplinary consideration balancing matrix benefits/risks and health economics are sure to follow.
To conclude, we have found that BTM provides a useful adjunct to aid wound healing in revascularised CLTI patients with extensive tissue loss, especially when exposed tendons and bones are present.
How Can A Biodegradable Matrix Offer Limb-Saving Options for Chronic Ischaemia?
“BTM
References
1. Conte MS, et al. Global vascular guidelines on the management of chronic limb-threatening ischemia. J Vasc Surg. 2019 Jun;69(6S):3S-125S.e40. doi: 10.1016/j.jvs.2019.02.016. Erratum in: J Vasc Surg. 2019 Aug;70(2):662. PMID: 31159978.
2. Duff S, Mafilios MS, Bhounsule P, Hasegawa JT. The burden of critical limb ischemia: a review of recent literature. Vasc Health Risk Manag. 2019;15:187-208. doi: 10.2147/VHRM. S209241. PMID: 31417268.
3. Agarwal S, Sud K, Shishehbor MH. Nationwide trends of hospital admission and outcomes among critical limb ischemia patients: from 2003-2011. J Am Coll Cardiol. 2016;67(16):19011913. doi: 10.1016/j.jacc.2016.02.040. PMID: 27102506.
4. Lim P, Chong SJ, Goh J, Oon HH, Cheng A, Tay SC, Tan BK. Novosorb® Biodegradable Temporising Matrix (BTM) and its Applications. Surg Technol Int. 2023;42:27-36. PMID: 37053370.
5. Betar N, Li W, Gawaziuk JP, Logsetty S. Clinical outcomes and resource utilisation in patients with major burns treated with NovoSorb® BTM. Burns. 2023;49(4):961-969. PMID: 37344307.
6. Lo CH, Deleyiannis FWB, Dini M, Douglas HE, Biedermann T, Reichmann E, et al. Long-term scarring outcomes and safety of patients treated with NovoSorb® Biodegradable Temporizing Matrix (BTM): An observational cohort study. JPRAS Open. 2023;35:224-233. PMID: 37360978.
7. Schlottmann F, Held M, Medina D, Kerrigan CL. Treatment of Complex Wounds with NovoSorb® Biodegradable Temporising Matrix (BTM)-A Retrospective Analysis of Clinical Outcomes. J Pers Med. 2022;12(12):2020. PMID: 36556223.
8. Harvey NM, Farroha A, Womack D, Ead JK. Artificial dermal templates: A comparative study of NovoSorb™ Biodegradable Temporising Matrix (BTM) and Integra® Dermal Regeneration Template (DRT). Burns. 2022;48(6):1412-1419. PMID: 35462204.
9. Li H, Tan A, Lim P, Lee SSJ, Leow MQH, Chong SJ. Experience with NovoSorb® Biodegradable Temporising Matrix in reconstruction of complex wounds. ANZ J Surg. 2021;91(12):2651-2655. PMID: 34085755 Free PMC article.
10. Farroha A, Frew Q, El-Muttardi N, Philp B, Dziewulski P. Treatment of Necrotizing Fasciitis with NovoSorb® Biodegradable Temporizing Matrix™ and RECELL® Autologous Skin Cell Suspension: A Case Series. Wounds. 2021;33(2):48-54. PMID: 33449542
11. Austin CL, Hanson SE, Travis TE. Treatment of Necrotizing Fasciitis with NovoSorb® Biodegradable Temporizing Matrix™ and RECELL® Autologous Skin Cell Suspension: A Case Series. J Burn Care Res. 2023;44(2):269-273. PMID: 38085950
12. Wu SS, Friedrich JB, Friedrich PF, Moran SL, Haddock NT. Upper Extremity Wounds Treated with Biodegradable Temporizing Matrix versus Collagen-Chondroitin Silicone Bilayer. J Hand Microsurg. 2022;14(3):175-180. PMID: 38152680 Free PMC article.
13. Knightly N, Jones S, Soni M. NovoSorb Biodegradeable Temporizing Matrix for Reconstruction of Multiplanar Degloving Injury of the Upper Limb. Plast Reconstr Surg Glob Open. 2023;11(5):e4851. PMID: 37020984 Free PMC article.
14. Greenwood JE, Dearman BL. Split skin graft application over an integrating, biodegradable temporizing polymer matrix: immediate and delayed. J Burn Care Res. 2012;33(1):7-19. PMID: 21941194
15. Greenwood JE, Schmitt BJ, Wagstaff MJD. Experience with a synthetic bilayer Biodegradable Temporising Matrix in significant burn injury. Burns Open. 2018;2(1):17-34. doi: 10.1016/j. burnso.2017.11.002.
16. Wagstaff MJD, Schmitt BJ, Caplash Y, Greenwood JE. Biodegradable Temporising Matrix (BTM) for the reconstruction of defects following serial debridement for necrotising fasciitis: A case series. Burns Open. 2019; 3:12-30.
17. Wagstaff MJD, Schmitt BJ, Coghlan P, Finkemeyer JP, Caplash Y, Greenwood JE. A biodegradable polyurethane dermal matrix in reconstruction of free flap donor sites: a pilot study. ePlasty 2015; 15:102-18.
18. Masterclass Guide: Synthetic Dermal Matrix: Novosorb Biodegradeable Temporising Matrix (BTM). Wound Masterclass. Volume 1. No 1. June 2022.
19. Selig HF, Lumenta DB, Giretzlehner M, Jeschke MG, Upton D, Kamolz LP. The properties of an "ideal" burn wound dressing--what do we need in daily clinical practice? Results of a worldwide online survey among burn care specialists. Burns. 2012;38(7):960-966. doi: 10.1016/j. burns.2012.04.007.
20. Kinlay S. Management of Critical Limb Ischemia. Circ Cardiovasc Interv. 2016 Feb;9(2):e001946. doi: 10.1161/CIRCINTERVENTIONS.115.001946.
21. Guerriero FP, Clark RA, Miller M, Delaney CL. Overcoming barriers to wound healing in a neuropathic and neuro ischaemic diabetic foot cohort using a novel bilayer biodegradable synthetic matrix. Biomedicines. 2023;11(3):721.https://doi.org/10.3390/biomedicines11030721
22. Kuang B, Pena G, Cowled P, Fitridge R, Greenwood J, Wagstaff M, Dawson J. Use of Biodegradable Temporising Matrix (BTM) in the reconstruction of diabetic foot wounds: A pilot study. Scars Burn Heal. 2022;8:20595131221122272. doi: 10.1177/20595131221122272
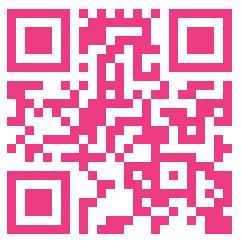
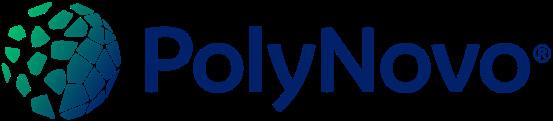
BTM supports cellular migration and formation of neodermis.1,2
• It provides a porous framework that bioabsorbs, leaving a robust vascularized dermal layer.3
• A temporary sealing membrane protects the wound while the body heals.4
Infected Diabetic Foot Ulcer
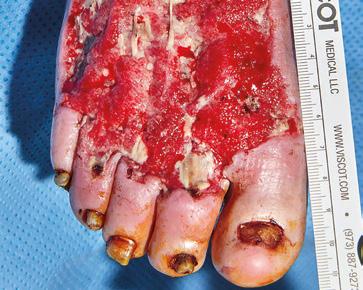
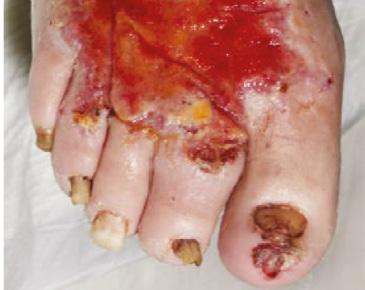
Indicated for full or deep partial thickness burns, traumatic wounds, complex vascular and surgical and reconstructive wounds. Wound prior to debridement
of BTM after week 4.5
Biodegradable Temporising Matrix Discover more at: polynovo.com
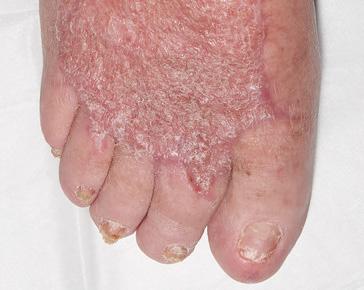
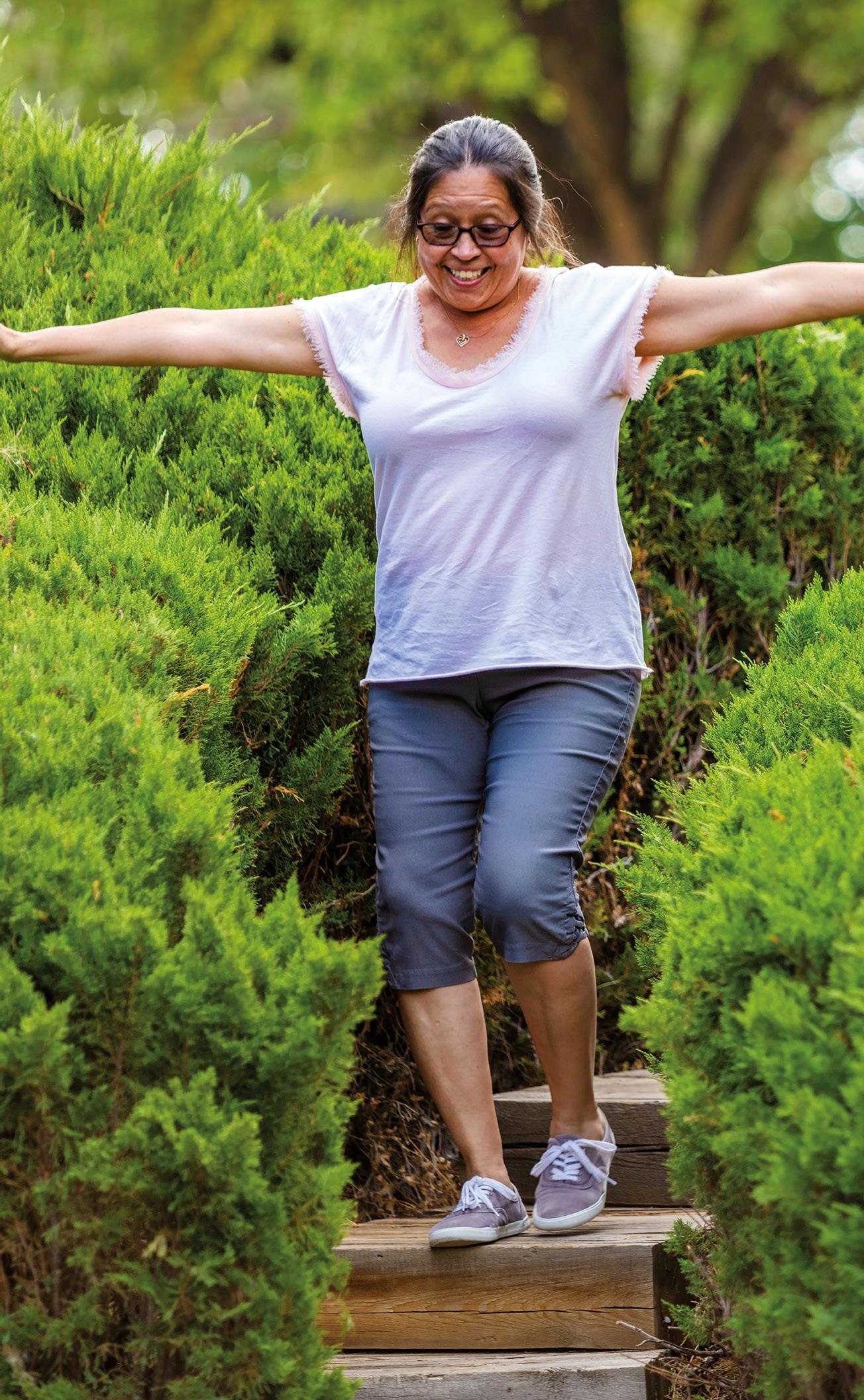
up at 4 months post grafting
Refer to the Instructions For Use for full device details. References: 1. Greenwood JE, Schmitt BJ, Wagstaff MJD. Experience with a synthetic bilayer Biodegradable Temporising Matrix in significant burn injury. Burns Open. 2018;2(1):17-34. 2. Wagstaff MJD, Salna IM, Caplash Y, Greenwood JE. Biodegradable Temporising Matrix (BTM) for the reconstruction of defects following serial debridement for necrotising fasciitis: A case series. Burns Open. 2019; 3:12–30. 6. Data on file. 3. Wagstaff MJD, Schmitt BJ, Coghlan P, Finkemeyer JP, Caplash Y, Greenwood JE. A biodegradable polyurethane dermal matrix in reconstruction of free flap donor sites: a pilot study. ePlasty 2015; 15:102–18. 4. Greenwood JE, Dearman BL. Split-skin graft application over an integrating, biodegradable temporising polymer matrix: immediate and delayed. J Burn Care Res 2012; 33(1):7–19. ® PolyNovo and NovoSorb are registered trademarks of PolyNovo Biomaterials Pty Ltd. Discover more: polynovo.com
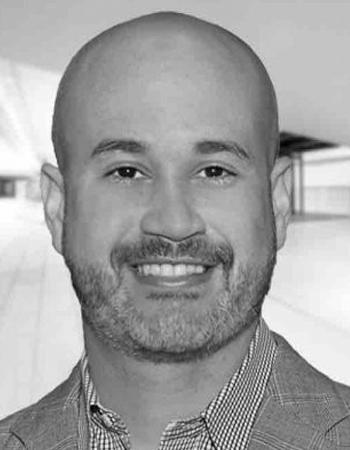
Dr Elvis Castillo Garcia
Infectious Diseases and Wound Care Physician, Florida Medical Clinic
Wesley Chapel FL, United States
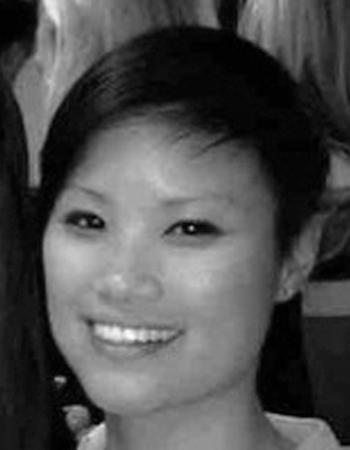
Complex Refractory Wounds: How to Overcome Treatment Recalcitrance and Restore the Healing Trajectory Using Innovative
Bioactive Glass
Editorial Summary
This case series evaluates the efficacy of a novel borate-based bioactive glass wound matrix (BGWM) for treating complex, refractory chronic wounds that failed to heal with multiple previous advanced therapies. Chronic non-healing wounds pose a significant health burden, and new treatment approaches are needed. BGWM is a biocompatible, water-soluble matrix designed to support wound healing. Patients with longstanding surgical and radiation wounds of mean 13.2 month duration were treated with serial BGWM applications per a standardized protocol. Despite previously proving recalcitrant to other costly advanced wound care modalities, all three wounds achieved complete closure after a mean of 8.1 weeks of BGWM use. The mean initial wound volume of 1.9 cm3 across this cohort highlights the severity of the wounds treated. This small case series provides promising preliminary evidence that the BGWM represents an effective new therapeutic option capable of facilitating healing in complex, chronic wounds refractory to other treatments.
Introduction
The profound clinical and socioeconomic ramifications of chronic, non-healing wounds continue to escalate at an alarming rate, representing one of the most formidable challenges facing modern healthcare systems globally. It is estimated that chronic wound prevalence now afflicts over 6 million individuals in the United States alone, culminating in a heavy annual economic burden exceeding $50 billion in associated treatment costs.1,2 This fiscal strain is projected to intensify further as our population ages, with a concurrent rise in age-related comorbidities like diabetes mellitus, obesity, and cardiovascular disease that critically impair physiologic wound healing pathways.3
Implementing advanced, cost-effective wound care strategies that synergistically target the multifactorial impediments underlying each phase of chronic wound pathogenesis is therefore imperative to promote timely and durable healing while reducing downstream healthcare utilization and expenditures.
A novel borate-based bioactive glass wound matrix (BGWM) , (Mirragen® Engineered Tissue Solutions, MO) composed of watersoluble borate compounds has recently emerged as a promising potential solution, demonstrating notable capabilities to facilitate healing in complex chronic wounds based on initial clinical experiences.2 This novel biomaterial represents a distinctly engineered class of biocompatible, biodegradable wound dressings designed to temporarily integrate into the wound microenvironment and
support native wound repair processes over a finite resorption period.5 Herein, we present our preliminary clinical experience evaluating the safety, tolerability, and therapeutic efficacy of BGWM in treating a series of complex, recalcitrant chronic wounds that had proven refractory to a multitude of other advanced wound care strategies and costly interventions over an extended period.
Chronic wounds are detrimental to patient quality of life and a significant strain on the healthcare economy.1 To reduce healthcare utilization, use of advanced wound care products that address essential components of the healing phases of chronic wounds is critical. A recently developed novel boratebased bioactive glass wound matrix (BGWM) has been shown to facilitate healing in complex chronic wounds.2 Borate-based bioactive glasses are biocompatible water-soluble materials that have been formulated to degrade in a wound over a period of days or weeks. These bioactive glasses have shown promise in wound
Dr Phuong Thuy Nguyen
Infectious Diseases and Wound Care Physician, Florida Medical Clinic
Wesley Chapel FL, United States
Complex Refractory Wounds:
“Steroids have wide-ranging metabolic effects that hinder key phases of healing— inflammation, proliferation, and remodelling. Determining appropriate candidacy for steroidal therapy requires assessing individual disease state, risk factors, and benefitrisk profile.”
healing.3-5 We report our experience with application of BGWM in recalcitrant, nonhealing wounds previously treated with multiple advanced wound care modalities.
Radiation exposure can significantly impair and delay the normal healing process of tissues and organs.6 When ionizing radiation interacts with cells, it can directly damage DNA structure as well as produce free radicals that attack cell membranes and intracellular proteins and enzymes. This cellular damage triggers programmed cell death (apoptosis) and impairs cellular reproduction.7 Additionally, radiation prompts inflammatory responses that can persist chronically. These effects inhibit the major phases of healing—inflammation, proliferation, and remodelling.
During the inflammatory phase, radiation diminishes the immune cells crucial for debris removal and fighting infection.8 Key growth factors and cytokines for new tissue formation are reduced while inflammatory factors like TGF-beta are elevated. In the proliferative phase, cell reproduction is constrained, slowing replacement of dead cells with viable ones.9 Angiogenesis is also hampered, decreasing oxygen and nutrient supply. Cells may persist in a prolonged state of metabolic stress. In remodelling, radiation causes decreased production and disorganization of the extracellular matrix while increasing stimulators of fibrosis like fibrocytes.9 The result is scar tissue formation rather than healthy replacement tissue.
The healing impairments from radiation are dose-dependent—higher exposures progressively inhibit healing capacity. The timing of radiation delivery can also influence effects, with continual exposures being more damaging than fractionated. Different
tissues display varying radiation sensitivity, related to their baseline regeneration ability and proportion of radiosensitive stem cells.10 However, the mechanisms of radiation-delayed healing are consistent across tissues. Effective strategies to mitigate radiation-impaired healing include limiting radiation dose and avoiding repeat exposures where feasible. Growth factors, stem cell therapies, oxygen radical scavengers, and pharmacologic anti-inflammatories hold promise to help kick-start the recovery process. Still the impact of radiation on healing should not be underestimated. Radiation protection principles and testing of regenerative biologics are key frontiers requiring further research and development. In healthcare, corticosteroids such as fluticasone, budesonide, hydrocortisone, and prednisone are commonly used for their anti-inflammatory and immunosuppressive properties. However, medicinal steroid use can significantly impair and delay the normal wound healing process.11 Steroids have wideranging metabolic effects that hinder key phases of healing—inflammation, proliferation, and remodelling. Determining appropriate candidacy for steroidal therapy requires assessing individual disease state, risk factors, and benefit-risk profile.
Inflammatory Bowel Diseases
Steroids are a foundational treatment for acute phases of inflammatory bowel diseases like Crohn’s and ulcerative colitis. Oral steroids like prednisone offer rapid reprieve from flareup symptoms like abdominal pain, frequent loose stools, bleeding, and malnutrition. They induce remission in moderate or severely active IBD before transitioning patients to maintenance treatments. Caution is warranted in IBD patients with higher complication risks including osteoporosis, diabetes and glaucoma.12
Chronic Obstructive Pulmonary Disease
Inhaled corticosteroids like fluticasone and
Complex Refractory Wounds: Innovative Bioactive Glass Wound Matrix
“Even minor wounds can demonstrate notable failure to progress through the overlapping healing stages. Strategies to restore healing progression include steroid tapering, switching to less potent steroids, and wound environment modification through moisture balance and debridement.”
budesonide are central to managing COPD symptoms and reducing exacerbation frequency. Oral steroids are deployed intermittently for COPD exacerbations to relieve acute respiratory distress without accentuating comorbidity risks associated with long-term oral steroid use. Contraindications include uncontrolled infections and avoidance in patients with significant untreated tuberculosis risk factors.13
Collagen Vascular Diseases
Autoimmune disorders like rheumatoid arthritis, systemic lupus erythematosus, granulomatosis with polyangiitis and polymyalgia rheumatica are all responsive to steroids. Intramuscular or intraarticular steroid injections can provide localized efficacy. However, ubiquitous comorbidities and immune status in this population necessitates prudent consideration before sustained high dose systemic steroid administration. First line treatments are often steroid-sparing alternatives like DMARDS or biologics when feasible to minimize exposure.
In the inflammatory phase, steroids blunt the acute immune response needed to clear debris and prevent infection. They reduce key innate immune cells like macrophages and neutrophils and inhibit new vessel permeability. Steroids also lower growth factor and cytokine levels critical to signaling tissue regeneration while raising immunosuppressive factors like IL-10 and lipocortin-1.
In the proliferative phase, cellular reproduction slows under steroid influence, constraining replacement of damaged tissue. Steroids make cells less responsive to growth stimuli and more susceptible to apoptosis. Reduced angiogenesis also limits blood and nutrient supply to heal the wound area. Remodeling is impacted through decreased collagen production and impaired crosslinking, leading to weaker scar tissue formation. Matrix metalloproteinases break down scaffolding proteins faster than they can
be rebuilt.
The dose and duration of steroid exposure correlates with the severity of healing impairment—higher doses and longer usage most significantly delay healing capacity. The potency of the particular steroid also matters, as does administration route. Inhalational and topical steroids exert more localized effects on wounds than systemic treatment.
Wounds with greater baseline severity may be most vulnerable to further steroid-induced delay. However, the mechanisms depressing healing are consistent across wound types. Chronic ulcers and lacerations heal slowly anyway and are pushed to further stagnation. Even minor wounds can demonstrate notable failure to progress through the overlapping healing stages. Strategies to restore healing progression include steroid tapering, switching to less potent steroids, and wound environment modification through moisture balance and debridement. Growth factors may also aid cellular proliferation as may supplemental nutrition. Still, the multilevel impacts of steroids on recovery pathways should not be underestimated. Further research into therapeutics to counteract steroid-mediated healing retardation remains vital.
In summary, steroids remain a potent weapon against inflammatory states across varieties of conditions and patient profiles. Determining appropriateness requires carefully weighing their immunosuppressive effects against their anti-inflammatory benefits for each patient’s situation. The therapeutic index demands judicious dispensing to maximize relief while minimizing adverse events.
Methods
This prospective case series was conducted at our outpatient wound care center between January 2022 and August 2022. All patients
provided informed consent, and the study was approved by the institutional review board. Patients were eligible if they had a chronic wound that failed to achieve >40% wound area reduction after >4 weeks of appropriate treatment with advanced wound care products. Exclusion criteria included uncontrolled diabetes (HbA1c >9%), untreated malignancy, end-stage renal disease, active immunosuppression, and wounds of vascular or arterial etiology.
Three patients with a total of 3 chronic wounds meeting inclusion criteria were treated with serial applications of BGWM over the study period. All patients underwent initial wound preparation involving sharp debridement of necrotic tissue and cleansing with sterile saline solution. BGWM* was comprised of bioactive borate glass fibers suspended in an aqueous gel matrix (95% type 45S5 bioactive glass).
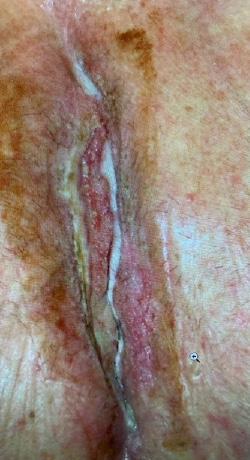
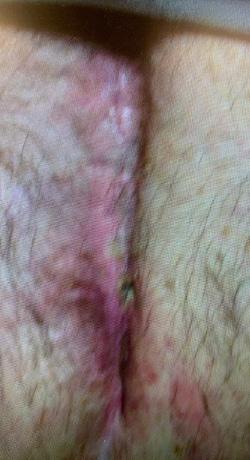
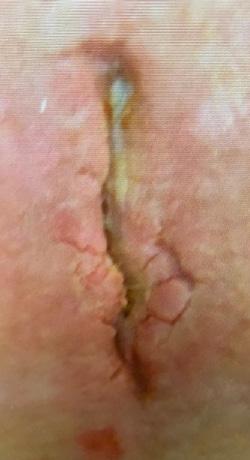
Results
The mean age of the patients was 72 years (range 56-84 years). Two of the wounds were chronic, recalcitrant post-surgical wounds and one a chronic radiation wound. All three wounds in this series had failed healing despite multiple previous advanced wound care
To apply BGWM, the fibers were first spread evenly across the wound bed to fully contact and cover the entire wound area with a 3-4 mm overlap extending onto the peri-wound skin. An occlusive secondary dressing was applied over the BGWM to immobilize the matrix and absorb wound exudate. Dressings were changed once weekly during follow-up visits when the wound and surrounding skin were reassessed and the BGWM reapplied as needed until complete wound closure. Systemic antibiotics were prescribed based on clinical criteria for evidence of wound infection.
Wound surface area and volume measurements were obtained using standardized wound imaging and analysis software at each dressing change. Pain levels were self-reported by patients on a 10-point scale. Healing was defined as full re-epithelialization of the wound surface.
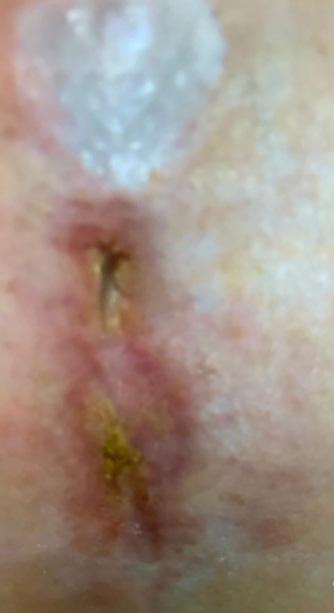
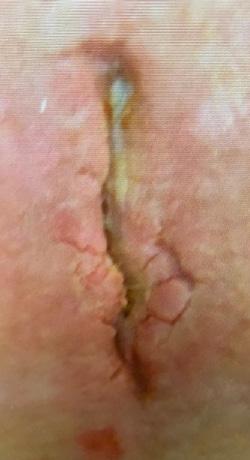
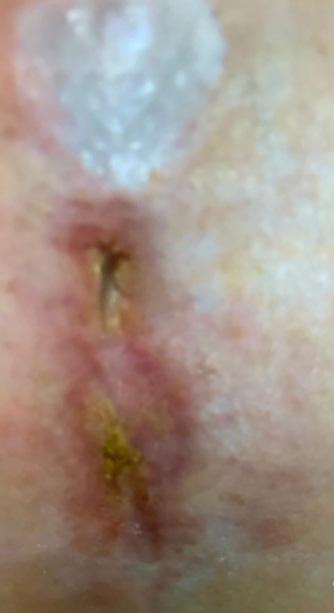
modalities including negative pressure wound therapy, bioengineered skin substitutes, and hyperbaric oxygen over a mean duration of 13.2 months (range 8-22 months). The mean initial wound size surface area and volume at the start of BGWM application were 12.7 cm2 (range 5.8-25.6 cm2) and 1.9 cm3 (range 0.8-4.1 cm3), respectively.
Figure 1-3 : Patients in the study showing pre and post BGWM results.
“Restoring adequate perfusion and oxygenation is critical for preventing oxidative stress, facilitating inflammatory cell influx, and supporting metabolic demands of wound healing pathways.”
All three wounds achieved complete closure after a mean duration of 8.1 weeks (range 5-11 weeks) with serial BGWM applications as the primary wound treatment. Figures 1-3 illustrate the sequence of healing in each case.
Mean patient-reported pain scores decreased from 6.7/10 at baseline to 1.3/10 after two weeks of BGWM application. BGWM was well tolerated by all patients without any adverse events. Two wounds required a short course of oral antibiotics early in treatment for clinical signs of superficial infection prior to achieving closure.
Discussion
The results of this case series demonstrate the promising potential of the borate-based bioactive glass wound matrix (BGWM) as an effective new therapeutic modality for complex, chronic wound pathologies that have proven recalcitrant to other advanced treatment approaches. Despite the inherent challenges to wound healing posed by the advanced age and significant comorbidities afflicting the patients in this cohort, complete wound closure was ultimately achieved in all three cases after a mean of just 8.1 weeks of treatment with serial BGWM applications. This noteworthy outcome is rendered even more remarkable given that these non-healing wounds had persisted for a mean duration of 13.2 months and remained stubbornly refractory to multiple previous advanced wound care therapies and surgical interventions at considerable healthcare expense.
While the small sample size and lack of a control arm preclude definitive conclusions, these preliminary findings align with the
hypothesized wound healing capabilities ascribed to borate-based bioactive glass.
Complex Refractory Wounds: Innovative Bioactive Glass Wound Matrix
Looking ahead, it is imperative that results from this promising initial case series catalyze larger, rigorously-designed randomized controlled trials to definitively establish the clinical efficacy of BGWM compared to current standards of care and other advanced wound care products. Systematic cost-effectiveness and quality of life analyses will also be essential to evaluate the full socioeconomic impact and patient-centered outcomes associated with implementing this novel therapy into chronic wound management protocols. Only through judicious evidence-based validation can the full clinical potential of borate-based bioactive glass wound matrices be realized.
Considerable opportunities remain for continued innovation to further optimize composition, structural properties, and therapeutic delivery of bioactive glass wound matrices. For instance, rational design of hybrid biomaterial composites can impart complementary wound healing capabilities like soft tissue filler or guided tissue regenerative functions. Controlled-release drug delivery systems could be engineered into the resorbable glass matrix for localized administration of
organic compounds like antimicrobials or biomolecules like growth factors and stem cells. As our understanding of chronic wound pathophysiology and the mechanisms by which bioactive glasses interact with wound environments grows, these next-generation wound dressings can be fine-tuned to present a personalized, diseased tailored therapy based on the distinctive wound characteristics and patient risk profile.
In conclusion, this preliminary case series provides compelling initial insights into the promising capacity of a borate-based bioactive glass wound matrix (BGWM) to overcome barriers to healing in complex, chronic wound pathologies that have remained refractory to other advanced treatment modalities. While inherent limitations preclude definitive conclusions, the ability to achieve full wound closure relatively rapidly in this cohort despite formidable challenges posed by patient age, comorbidities, and recalcitrance to previous therapies suggests BGWM may represent a powerful new tool in the wound care armamentarium. Larger, rigorously designed clinical trials are urgently warranted to comprehensively delineate the full therapeutic potential, patient outcomes, health economic impacts, and appropriate clinical utilization of this innovative biomaterial wound matrix therapy.
References
1. Sen CK. Human Wound and Its Burden: Updated 2020 Compendium of Estimates. Adv Wound Care (New Rochelle). 2021;10(5):281-292.
2. Armstrong DG, Orgill DP, Galiano RD, et al. A multi-centre, single-blinded randomised controlled clinical trial evaluating the effect of resorbable glass fibre matrix in the treatment of diabetic foot ulcers. Int Wound J. 2022 May;19(4):791-801.
3. Jung S, Day T, Boone T, et al. Anti-biofilm activity of two novel, borate based, bioactive glass wound dressings. Biomed. Glasses 2019; 5:67–75.
4. Rahaman MN, Day DE, Bal BS, et al. Bioactive glass in tissue engineering. Acta Biomater. 2011; 7(6): 2355–2373.
5. Mehrabi T, Mesgar AS, Mohammadi Z. Bioactive Glasses: A Promising Therapeutic Ion Release Strategy for Enhancing Wound Healing. ACS Biomater Sci Eng. 2020;6(10):53995430.
6. Haubner, Frank, et al. “Wound Healing after Radiation Therapy: Review of the Literature.” Radiation Oncology, vol. 7, no. 1, 24 Sept. 2012, https://doi.org/10.1186/1748-717x-7-162.
7. Orlita-Kozar, Angelika, et al. “Biological Adaptations of Three-Cells to Radiation Therapy.” Frontiers in Oncology, vol. 11, 24 Nov. 2021, https://doi.org/10.3389/fonc.2021.718636.
8. Wunderlich, Roland, et al. “Ionizing Radiation Reduces the Capacity of Activated Macrophages to Induce T-Cell Proliferation, but Does Not Trigger Dendritic Cell-Mediated Non-Targeted Effects.” International Journal of Radiation Biology, vol. 95, no. 1, 21 Aug. 2018, pp. 33-43, https://doi.org/10.1080/09553002.2018.1490037.
9. Dormand, Emma-Louise, et al. “Radiotherapy and Wound Healing.” International Wound Journal, vol. 2, no. 2, June 2005, pp. 112–127, https://doi.org/10.1111/j.1742-4801.2005.00079.x
10. Ottomeyer, M., Mohammadkah, A., Day, D., & Westenberg, D. J. (2016). Broad-spectrum antibacterial characteristics of four novel borate-based bioactive glasses, Advances in
11. Wray, P. (2011). “Cotton candy” that heals? Borate glass nanofibers look promising. American Ceramic Society Bulletin, 90(4), 25-29. 12. Hu, S., Chang, J., Liu, M., & Ning, C. (2009). Study on antibacterial effect of 45S5 Bioglass®. Journal of Materials Science: Materials in Medicine, 20(1), 281-286. https://doi. org/10.1007/s10856-008-3564-5
13. Romano, C. L., Logoluso, N., Meani, E., Romanò, D., De Vecchi, E., Vassena, C., & Drago, L. (2014). A comparative study of the use of bioactive glass S53P4 and antibioticloaded calcium-based bone substitutes in the treatment of chronic osteomyelitis: a retrospective comparative study. The Bone & Joint Journal, 96-B(6), 845-850. https://doi. org/10.1302/0301-620X.96B6.33360
14. Lin, Y., Brown, R. F., Jung, S. B., & Day, D. E. (2014). Angiogenic effects of borate glass microfibers in a rodent model. Journal of Biomedical Materials Research Part A, 102(12), 44914499. https://doi.org/10.1002/jbm.a.35120
15. Zhao, S., Li, L., Wang, H., Zhang, Y., Cheng, X., Zhou, N., Rahaman, M. N., Liu, Z., Huang, W., & Zhang, C. (2015). Wound dressings composed of copper-doped borate bioactive glass microfibers stimulate angiogenesis and heal full-thickness skin defects in a rodent model. Biomaterials, 53, 379-391. https://doi.org/10.1016/j.biomaterials.2015.02.112
16. Jia, T. B., Fu, T., & Liu, J. (2019). The effects of copper-doped borosilicate bioactive glass on the proliferation and osteogenic differentiation of bone marrow-derived mesenchymal stem cells. Journal of Biomaterials Applications, 33(7), 1005-1018. https://doi. org/10.1177/0885328218815770
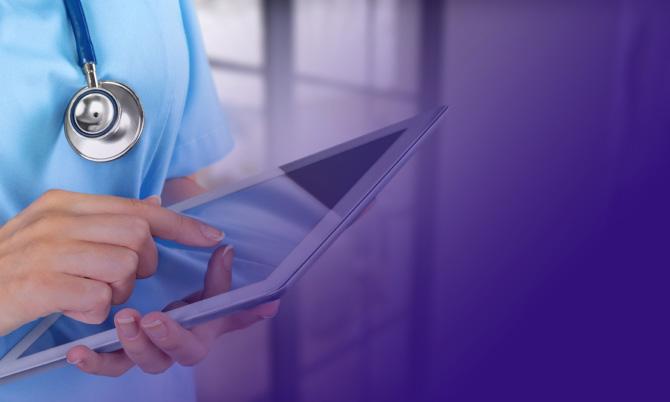
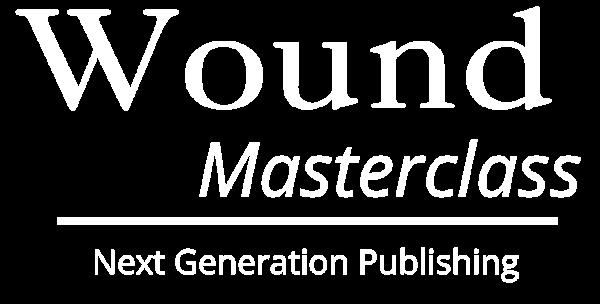

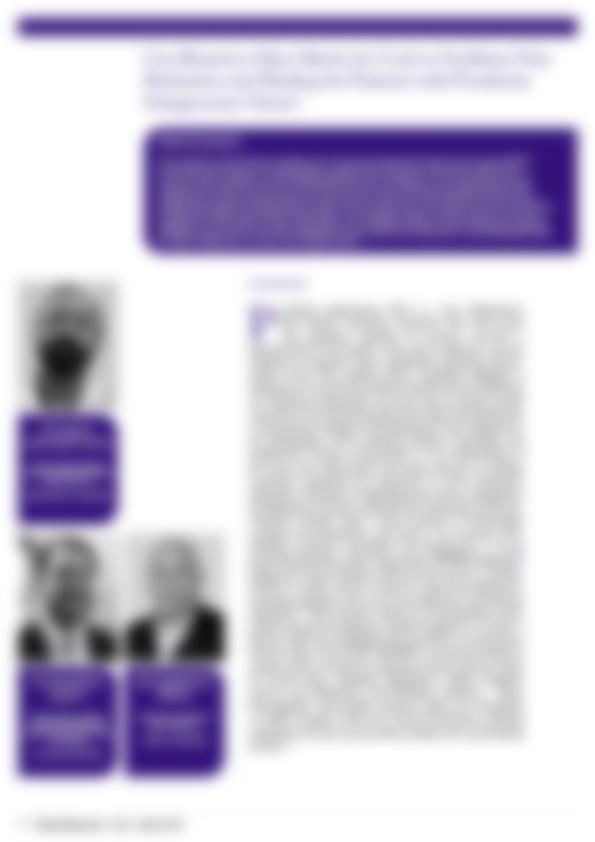
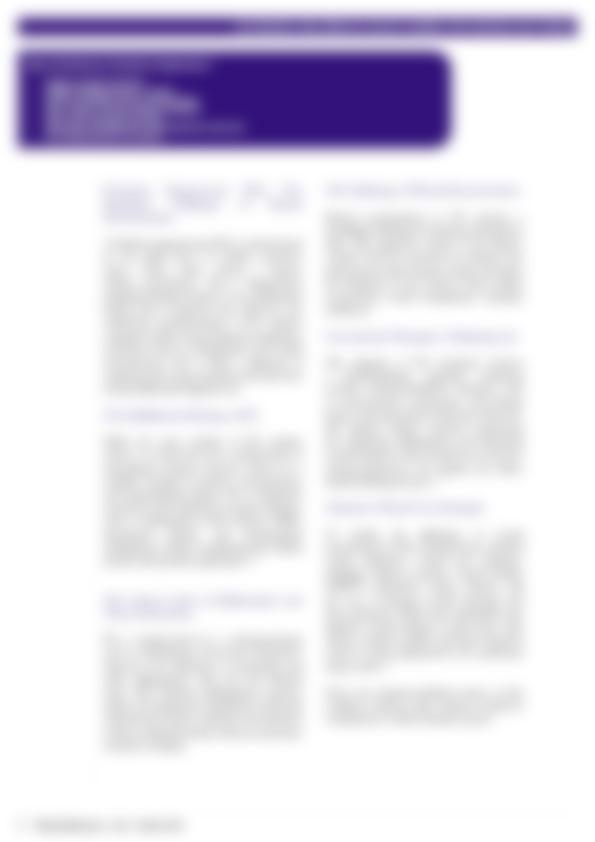
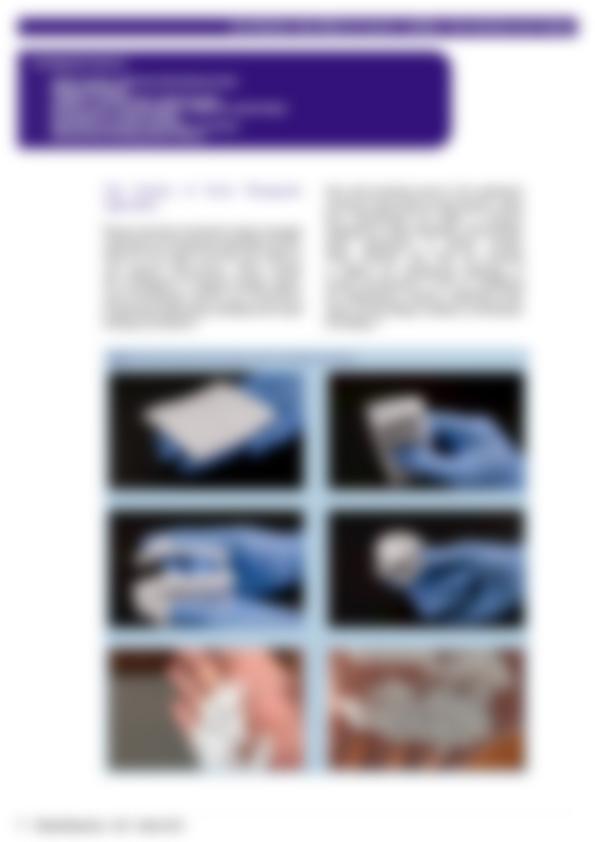
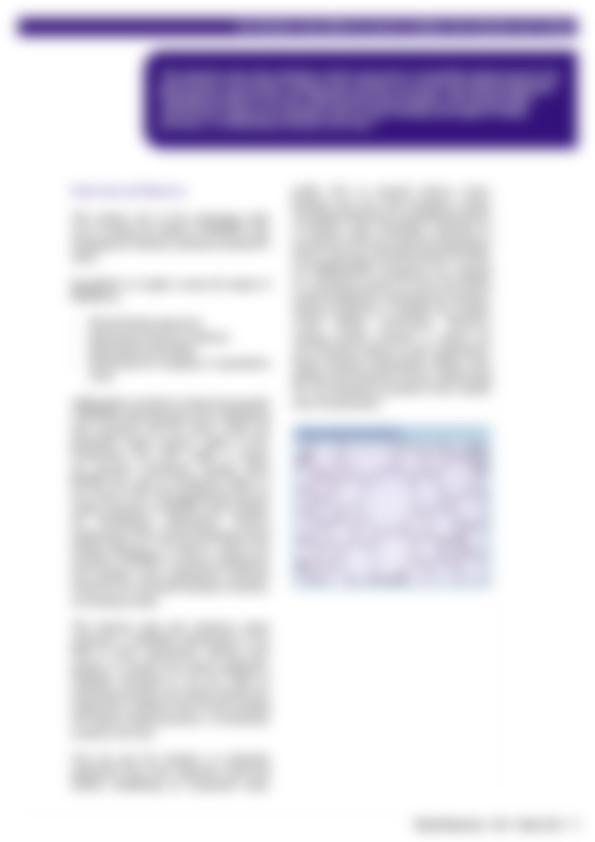
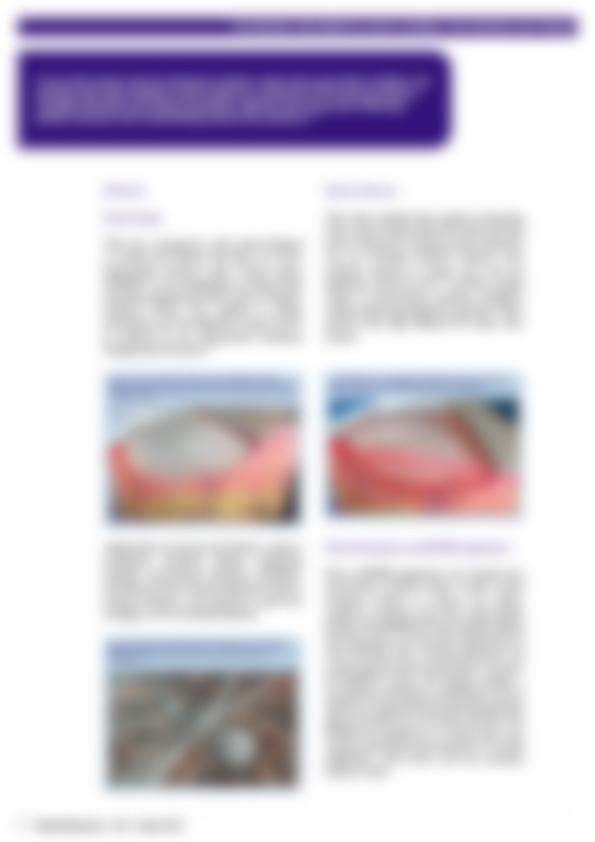
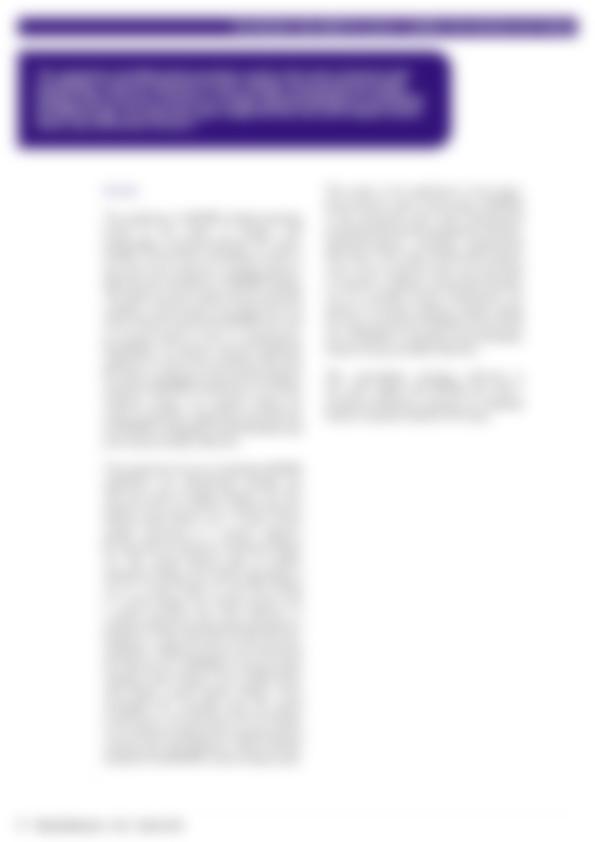
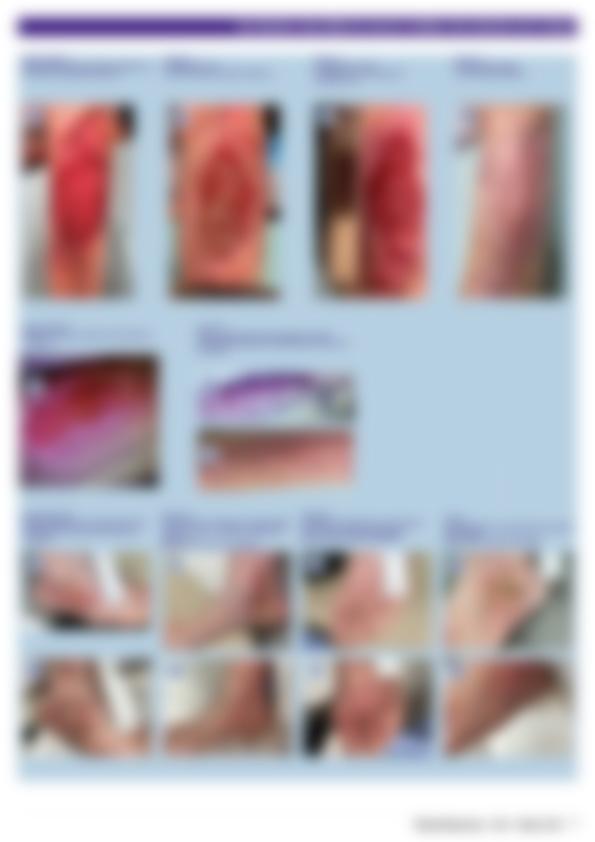

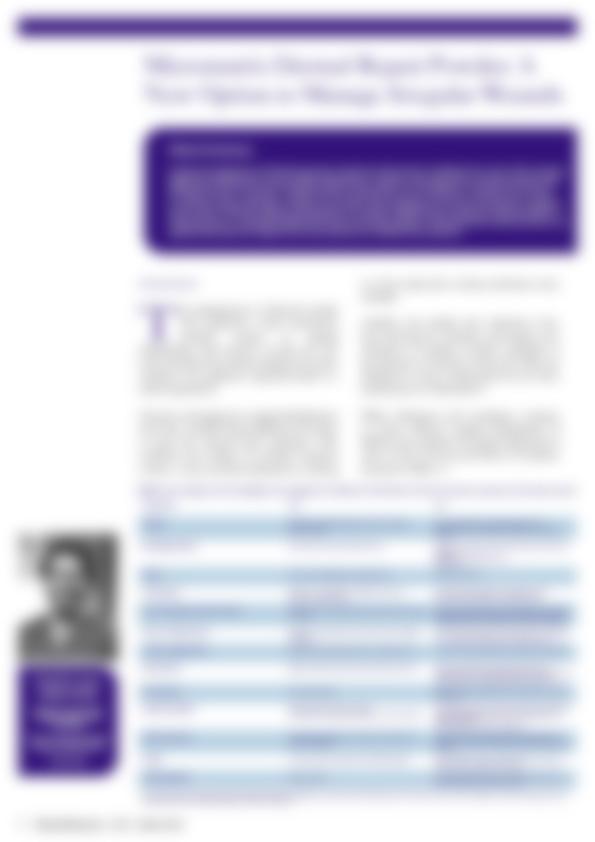
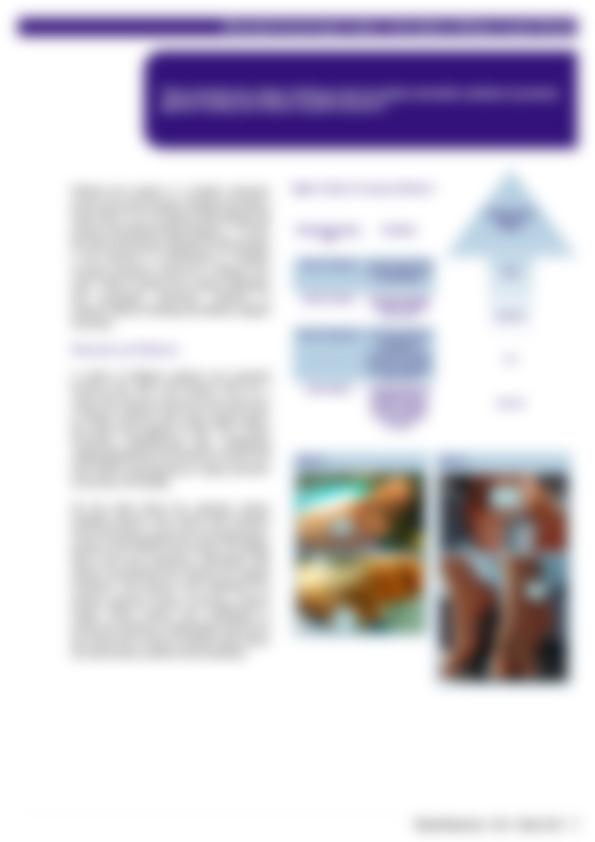
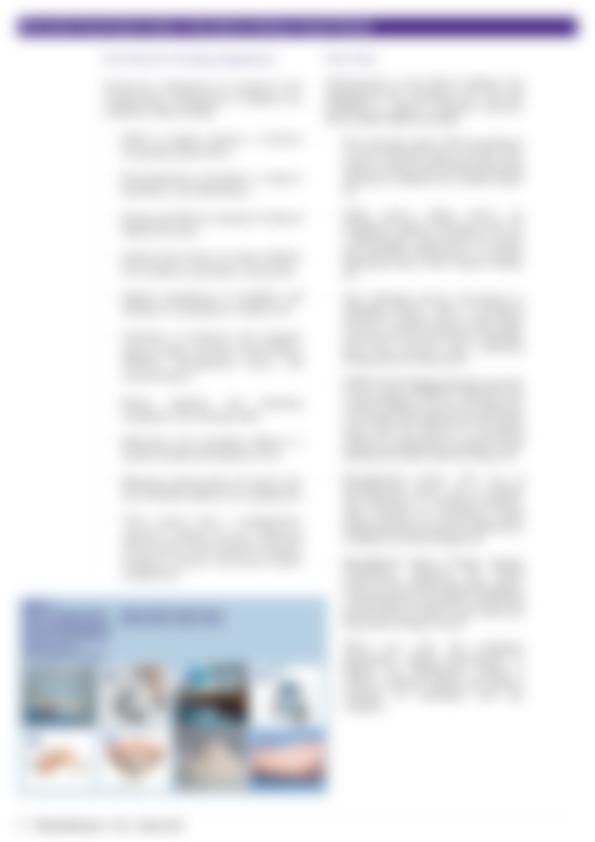
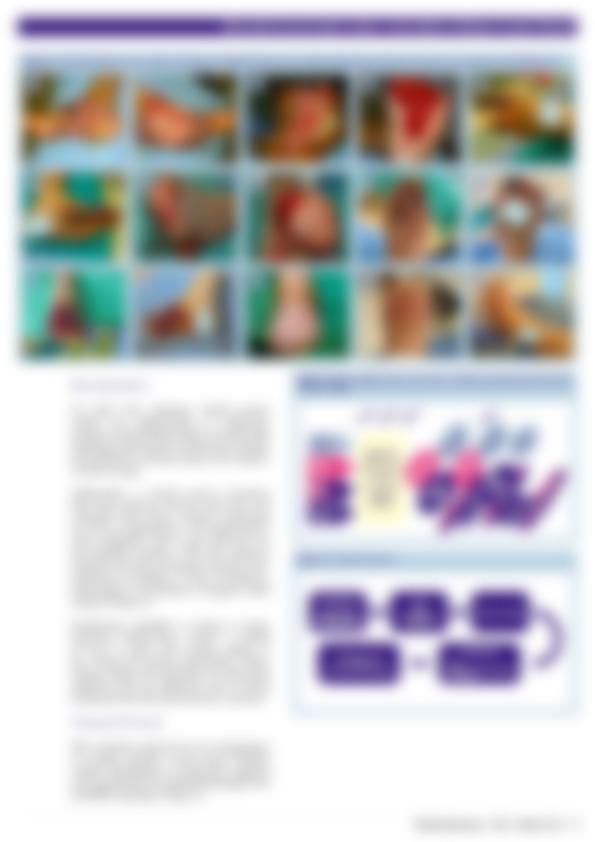
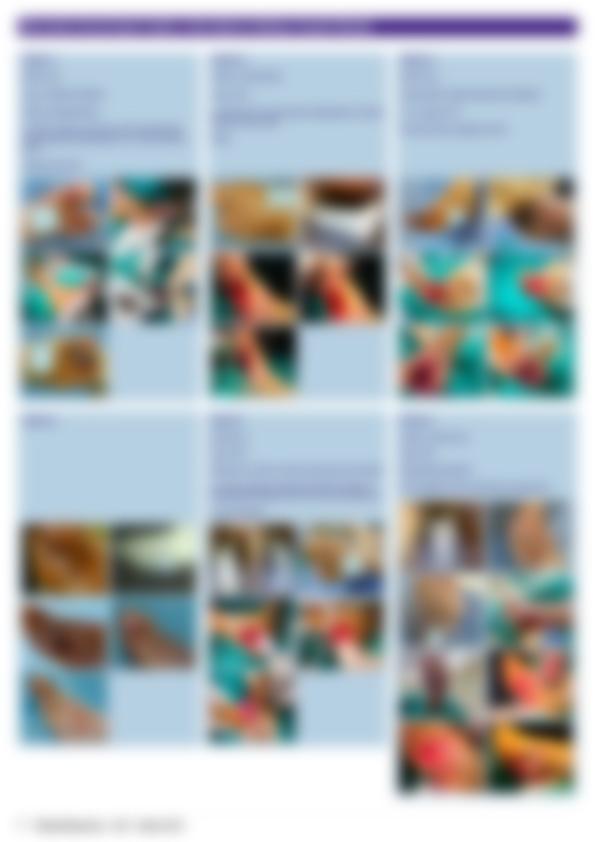
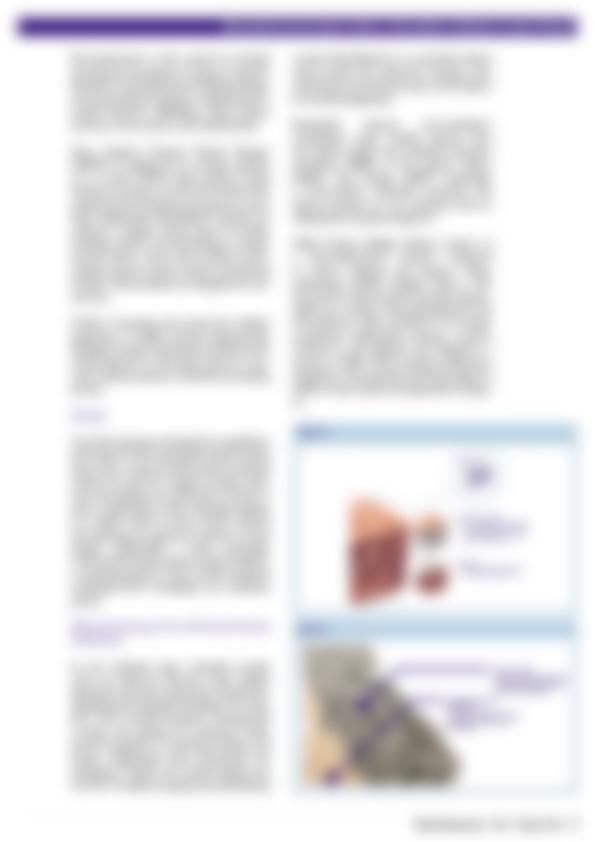
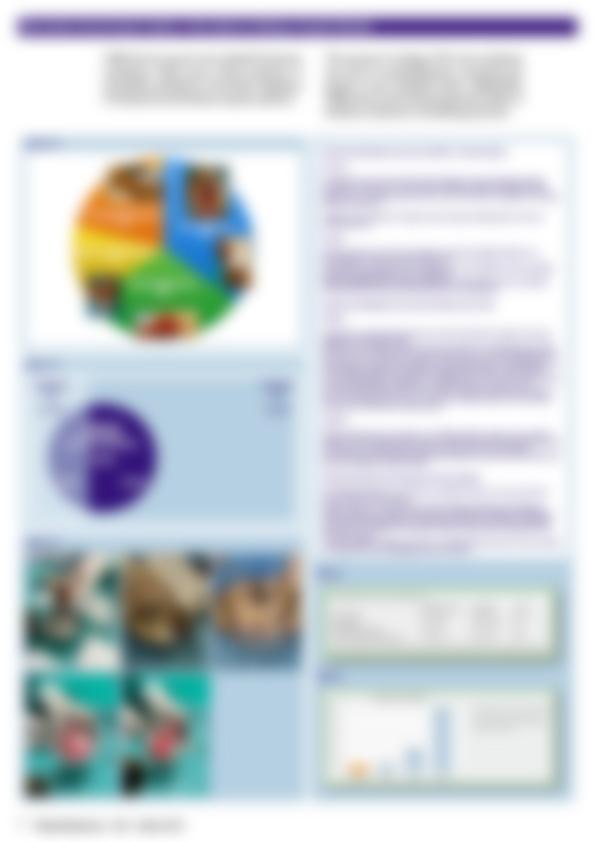
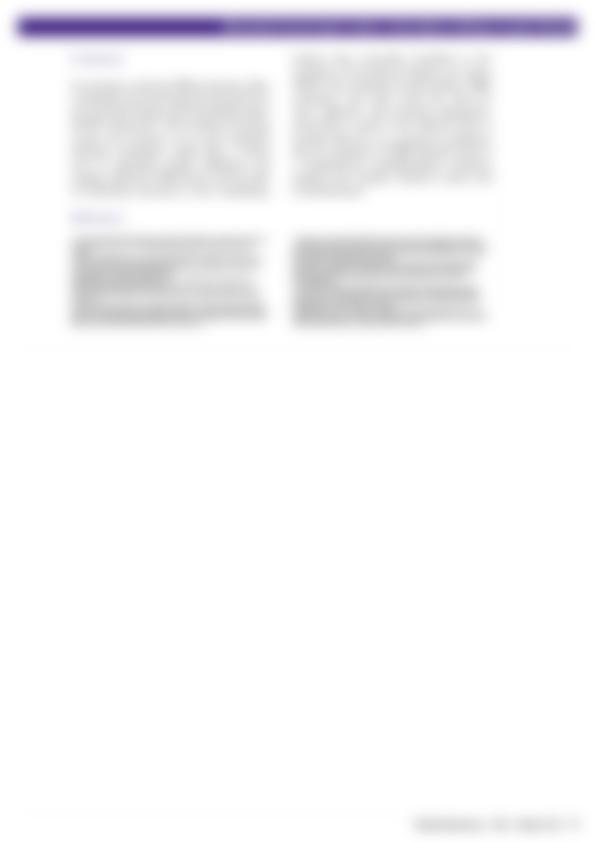
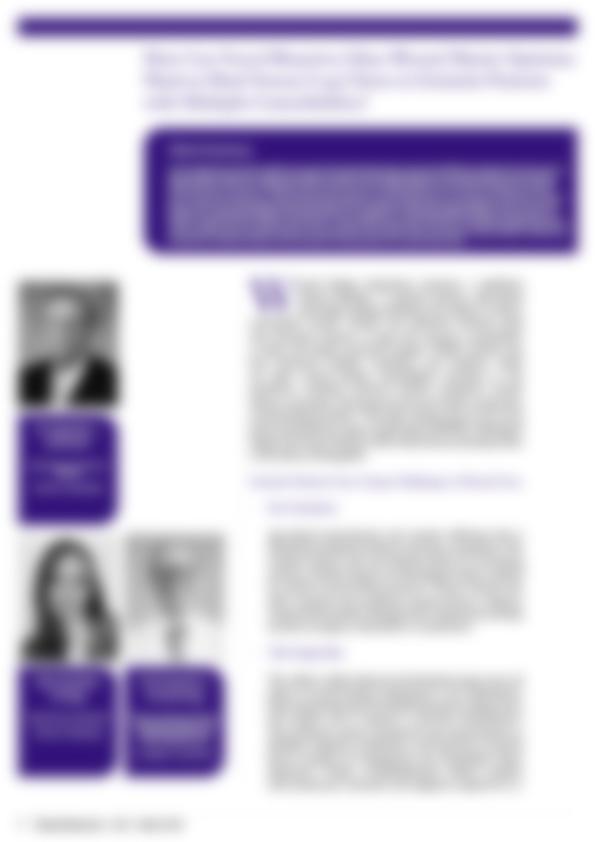
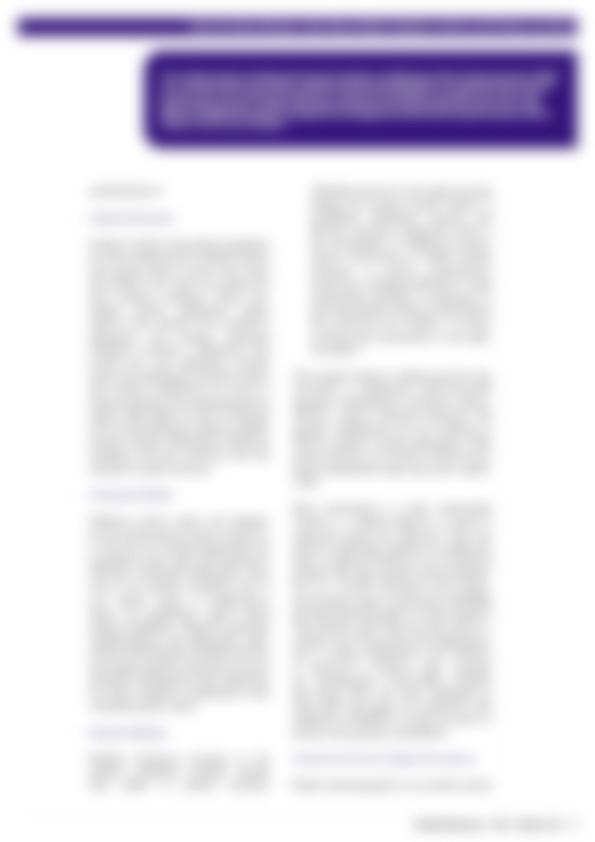
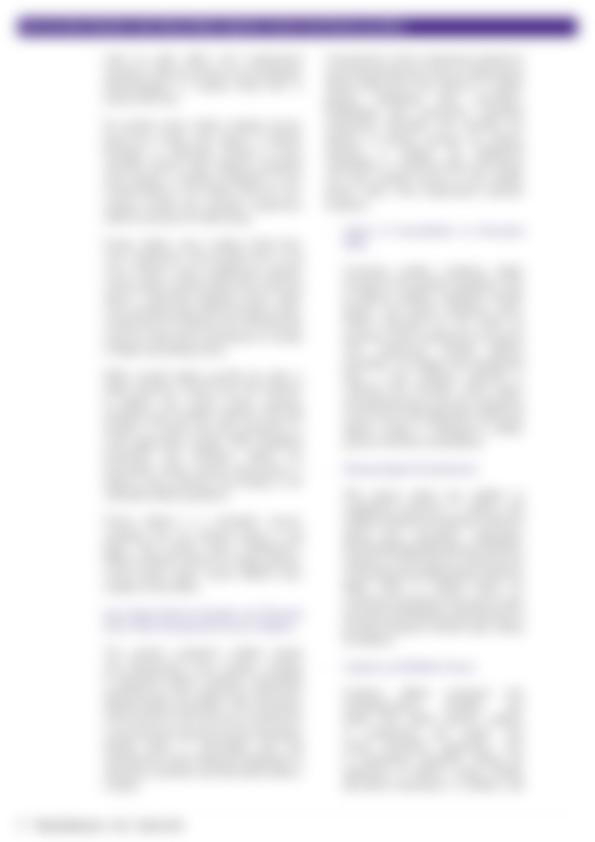
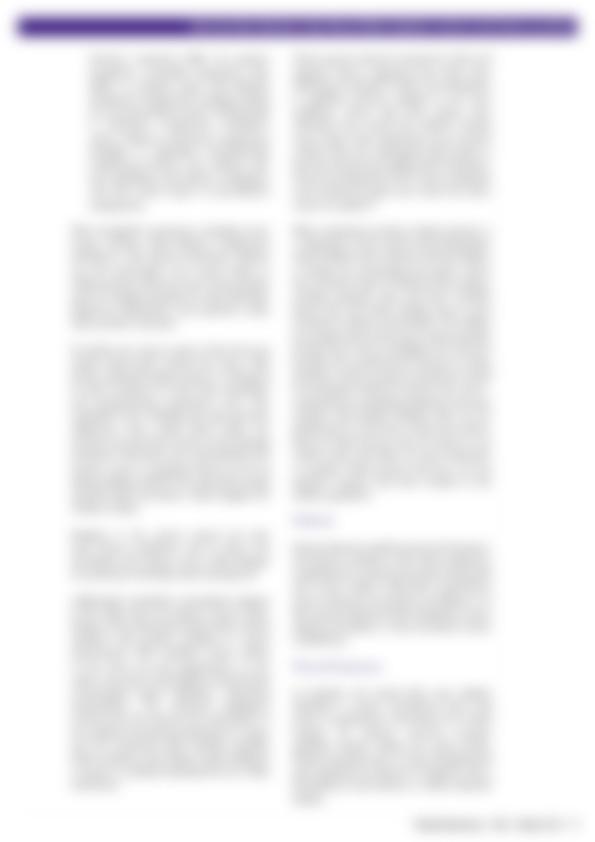
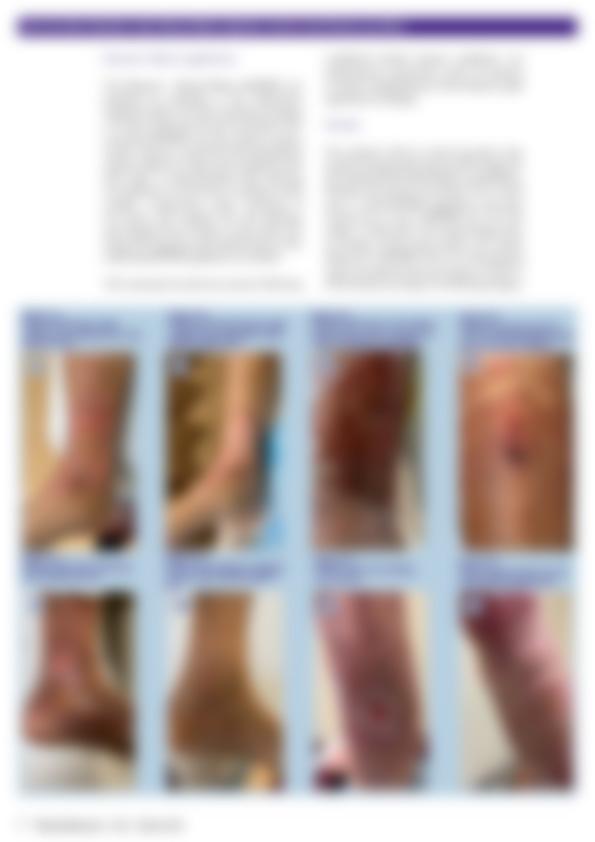
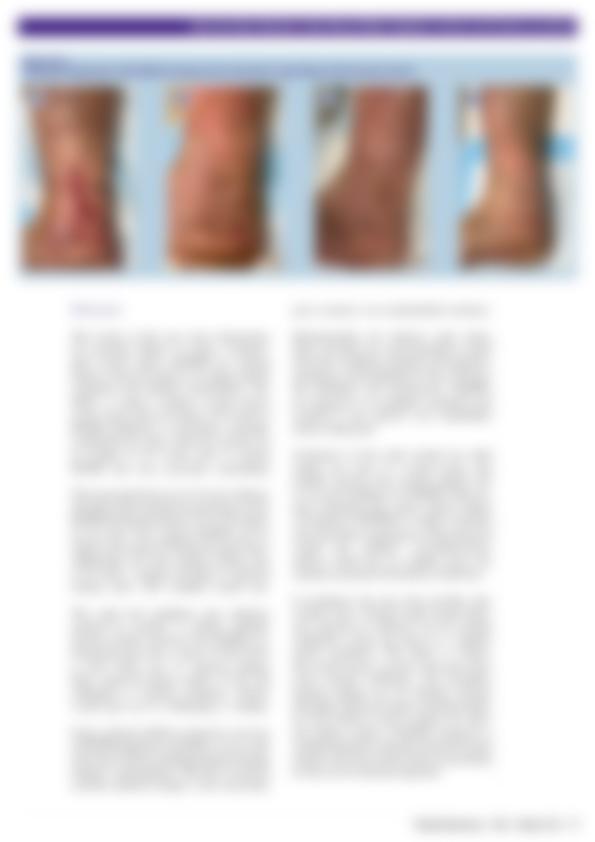
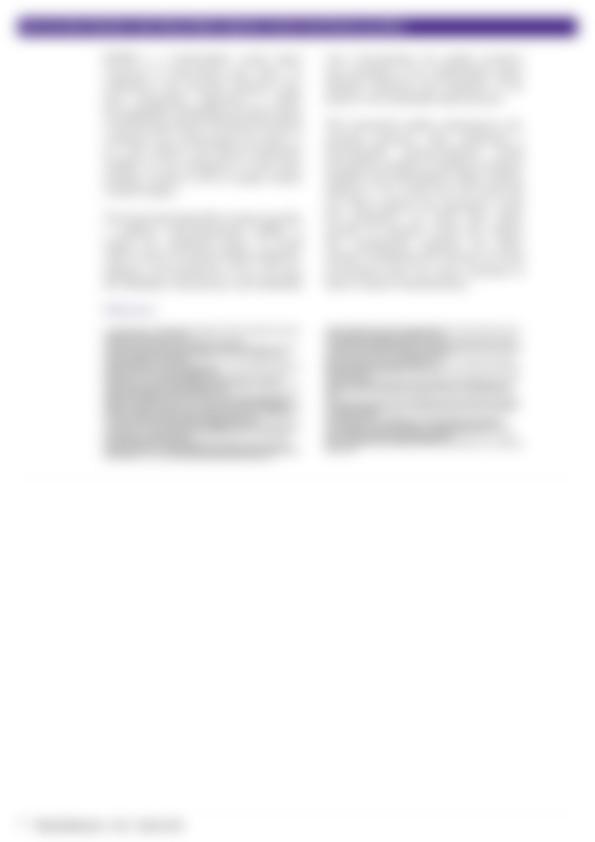
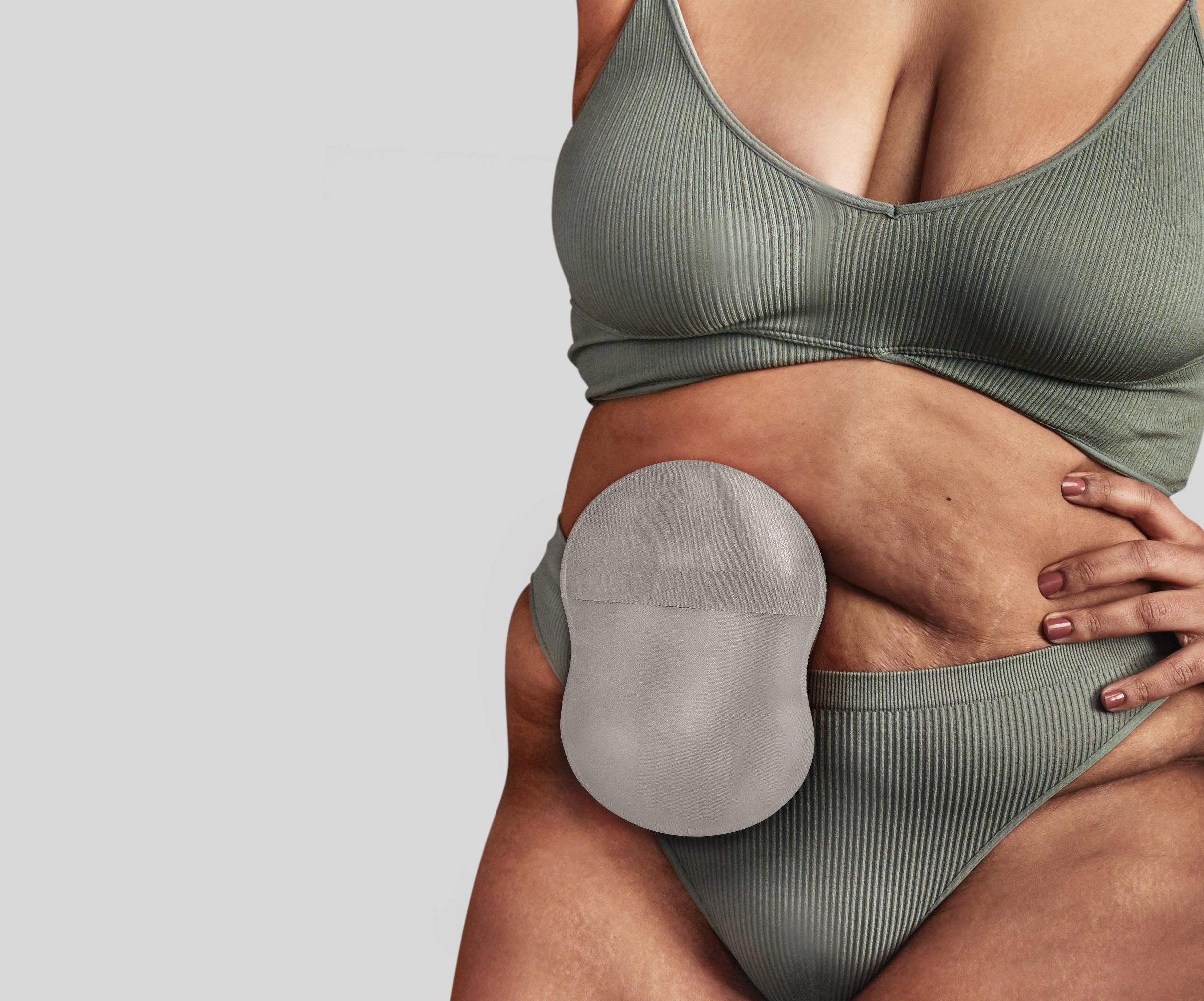










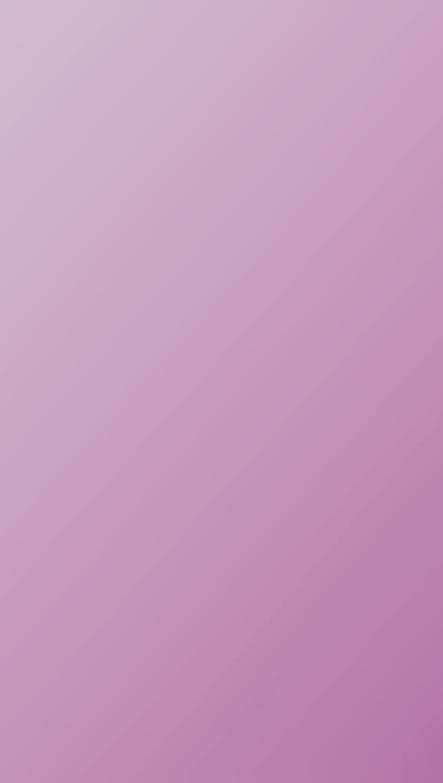
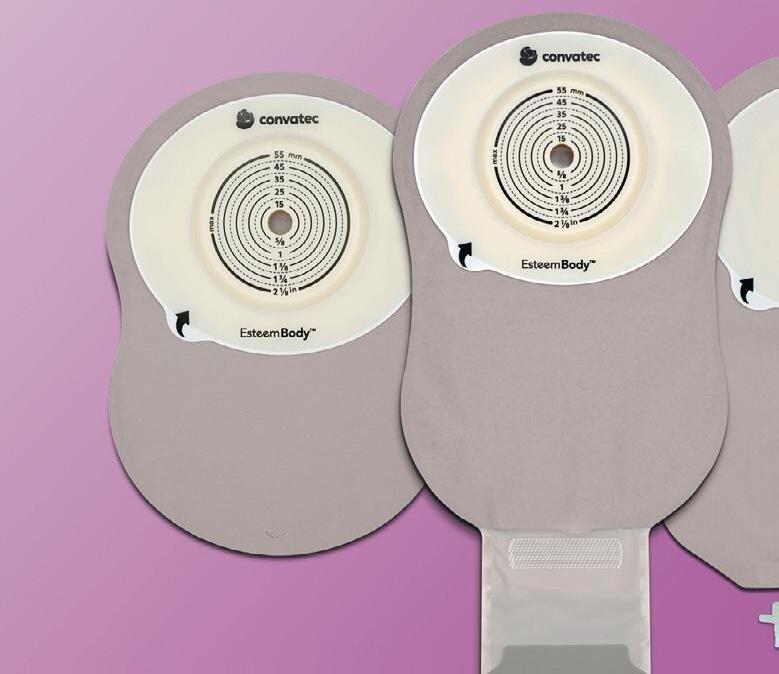
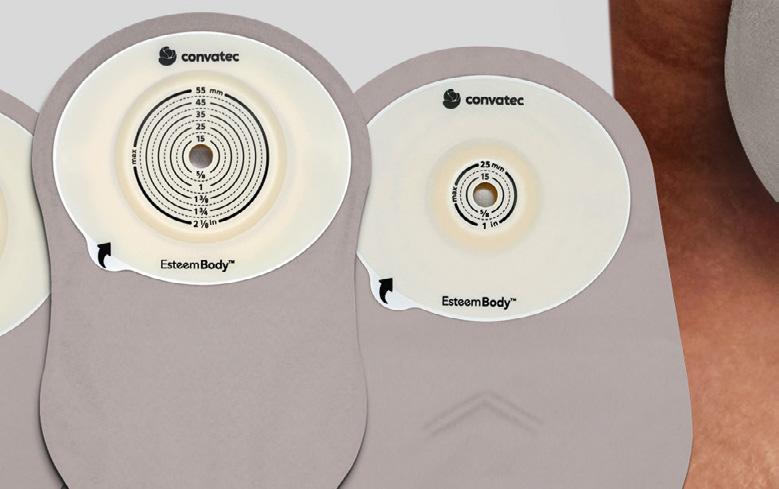
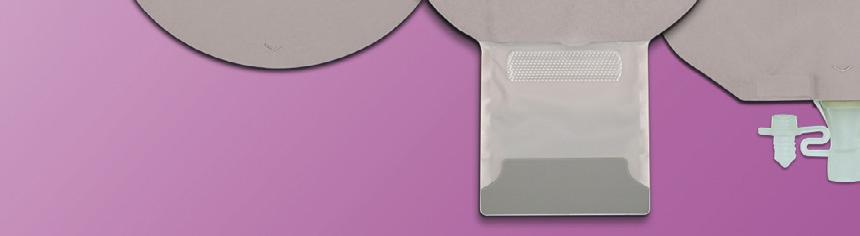
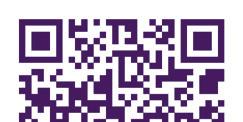
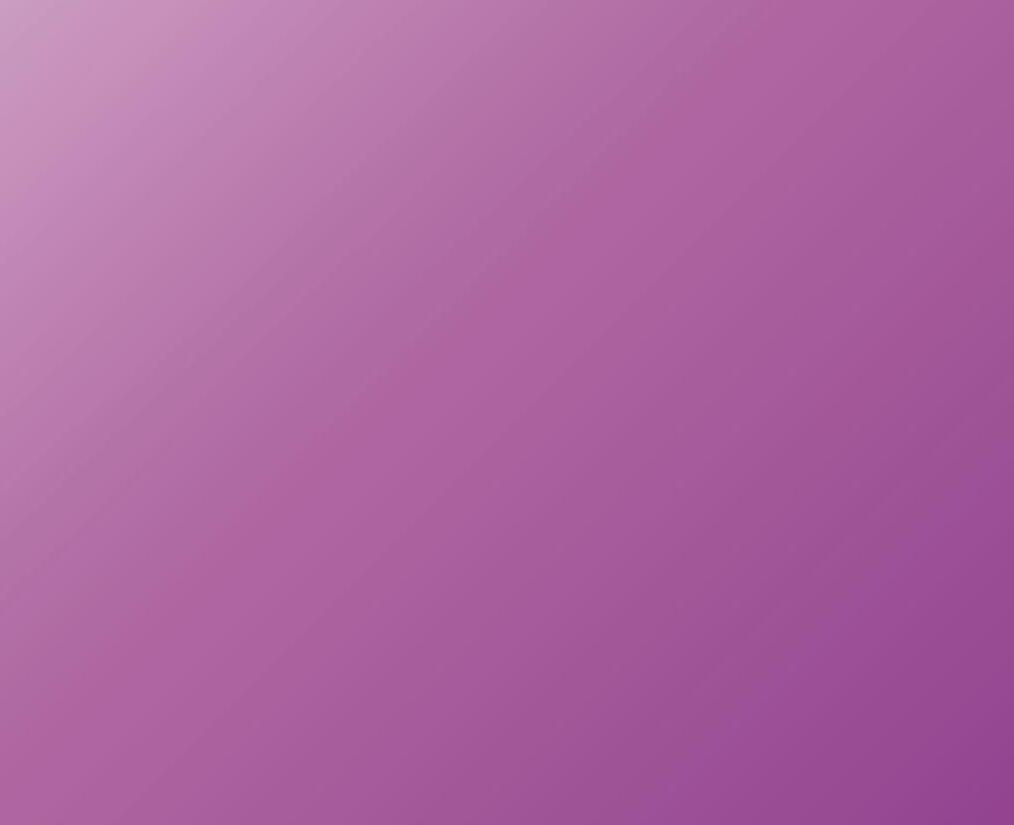
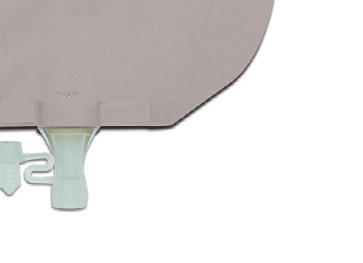
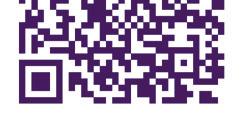
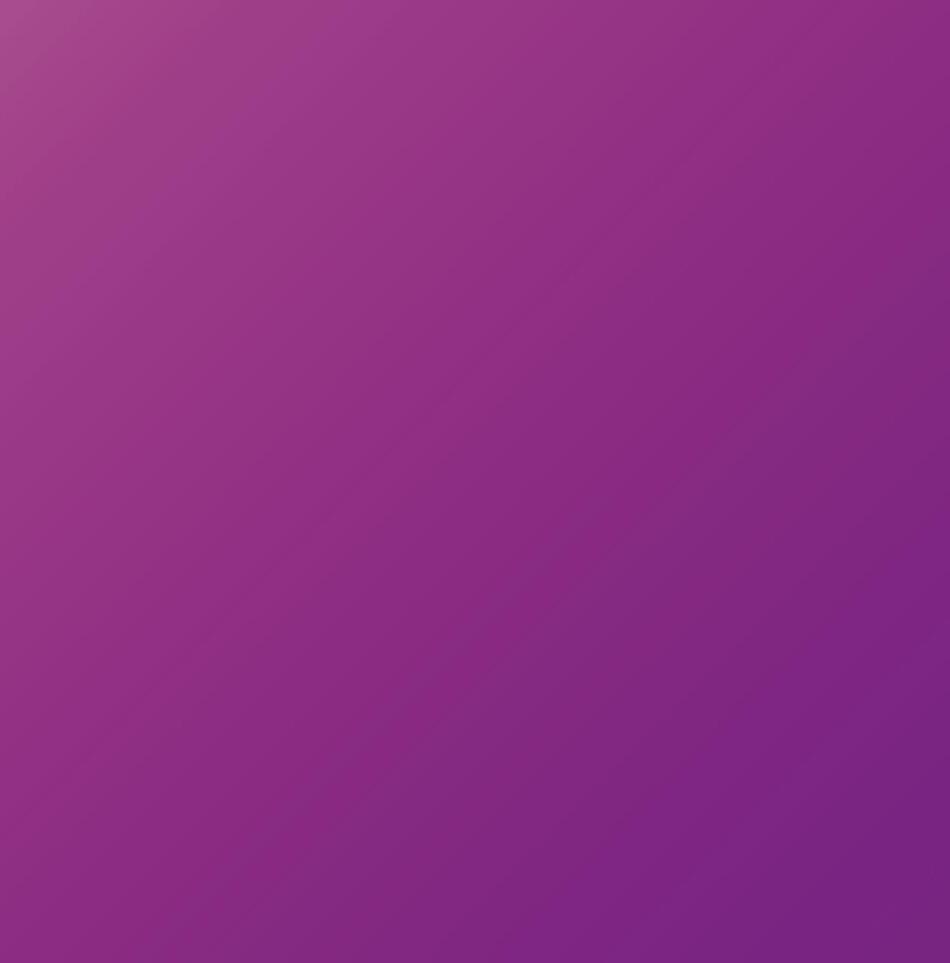
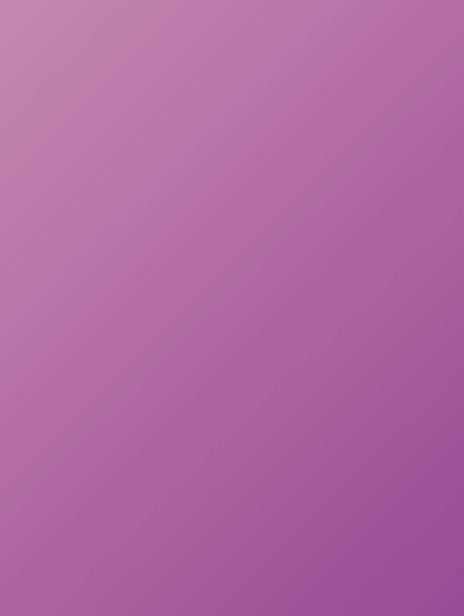
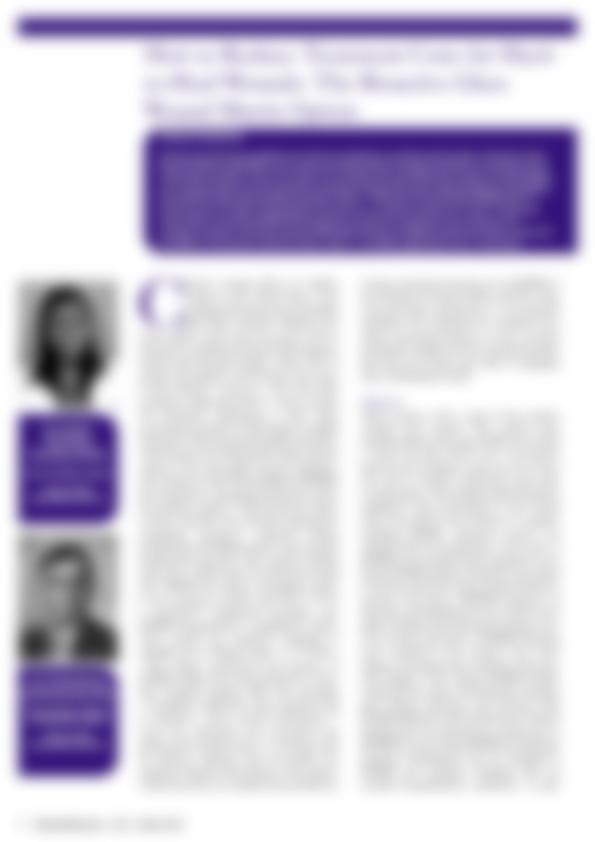
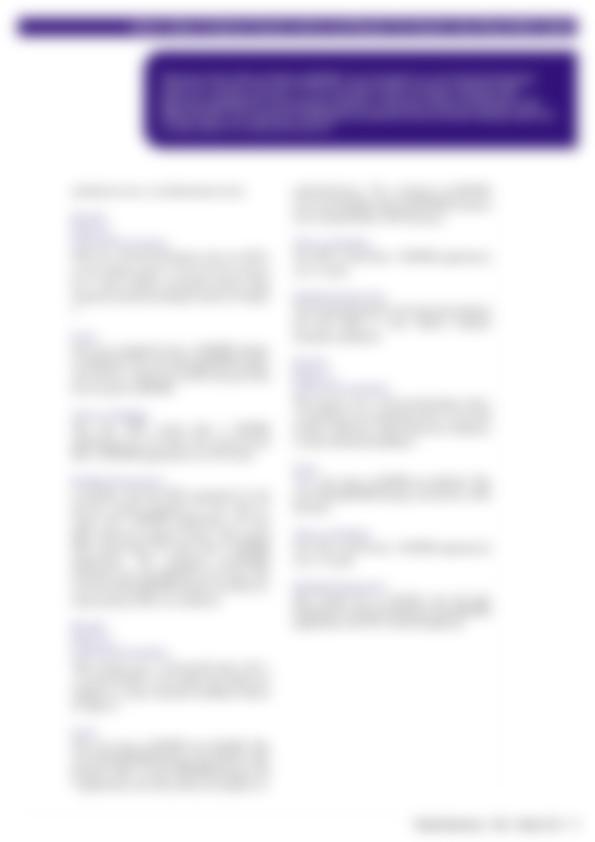
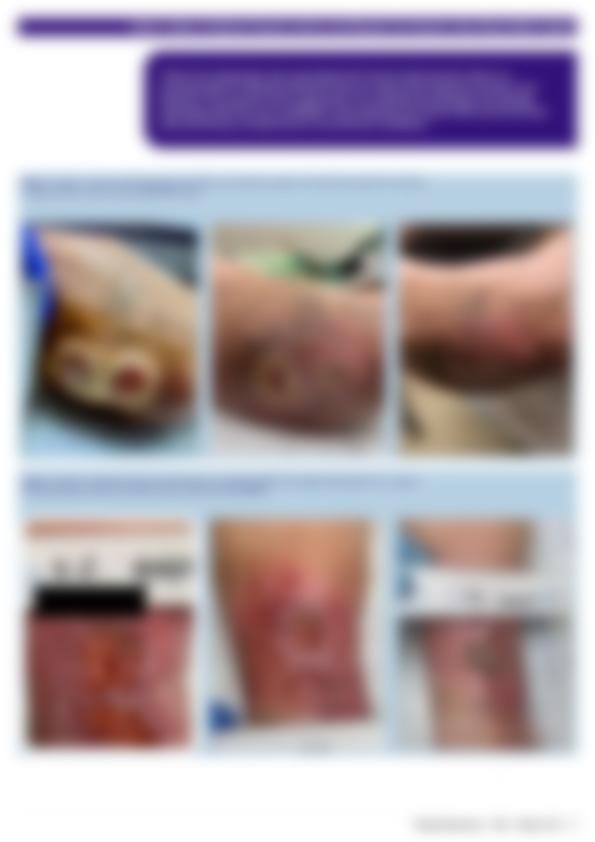
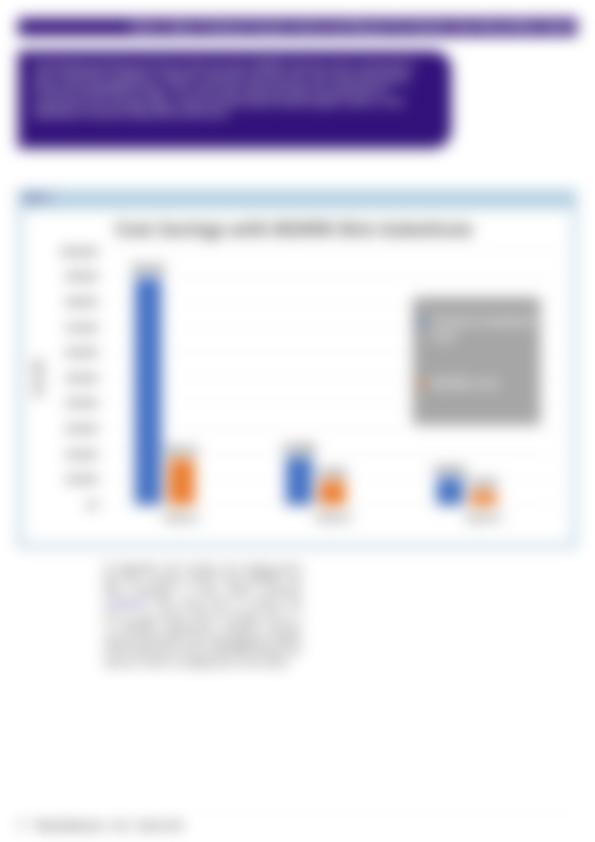
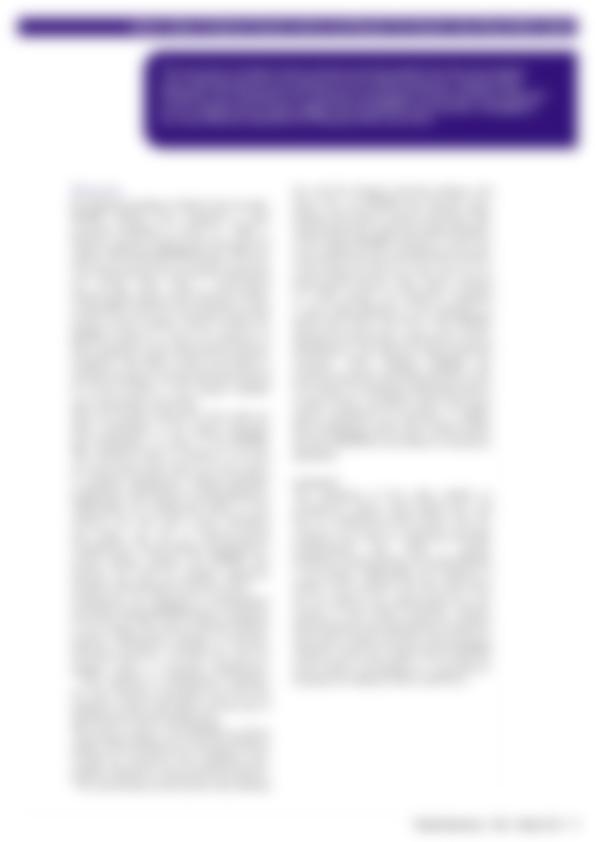
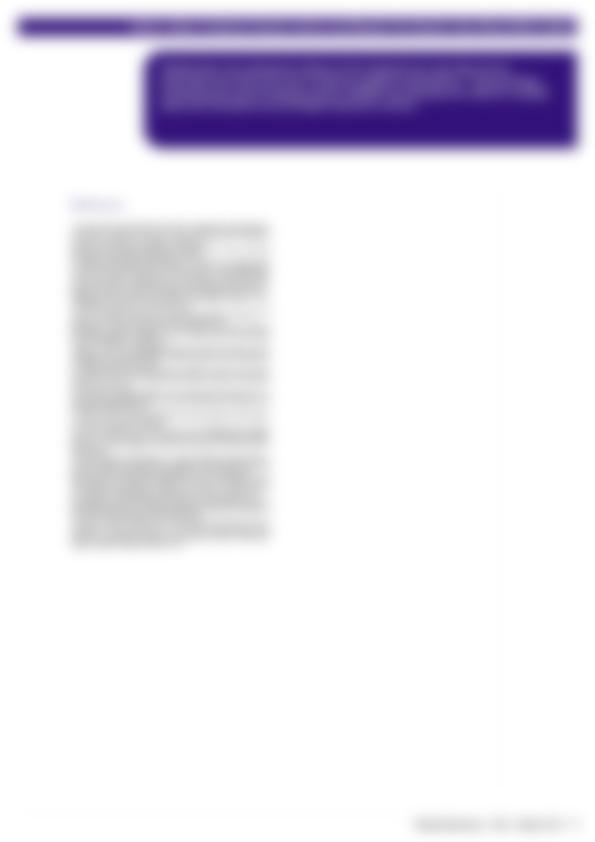
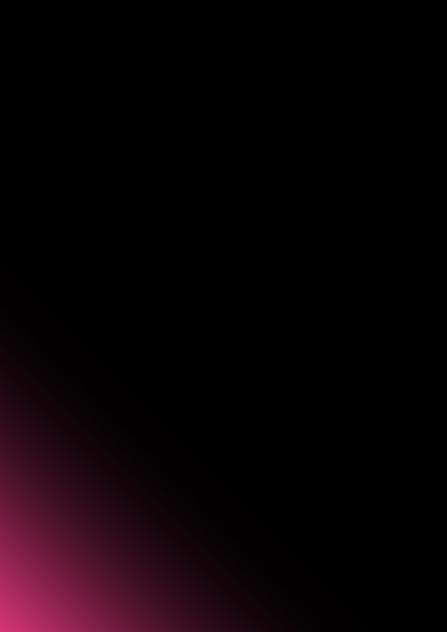
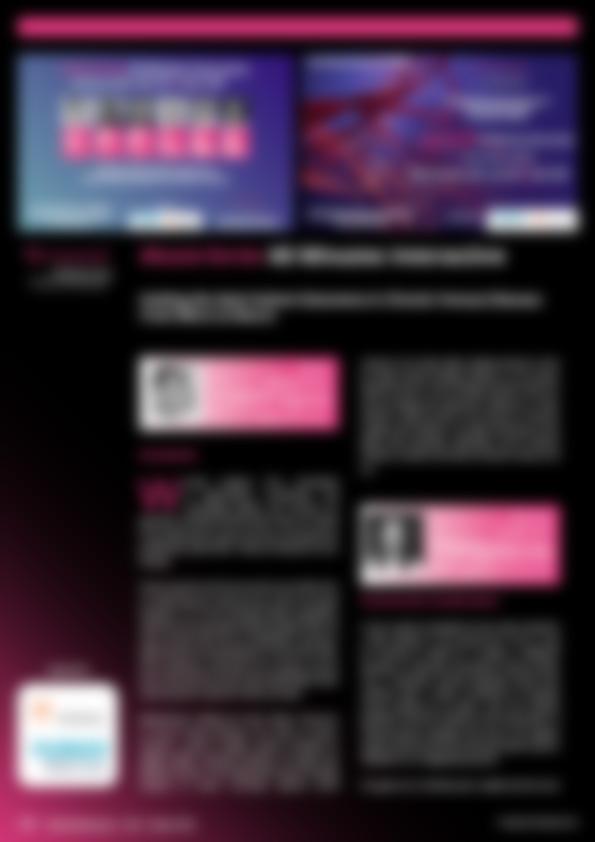
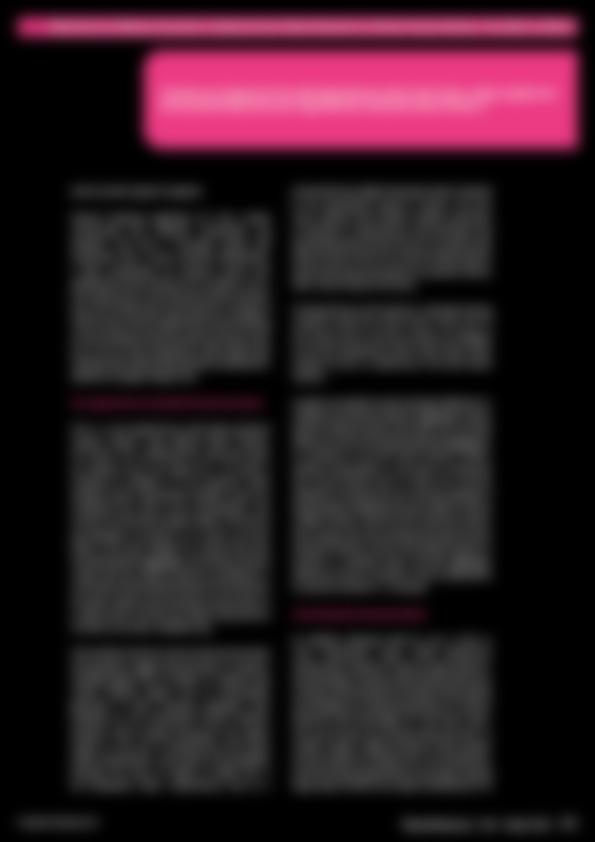
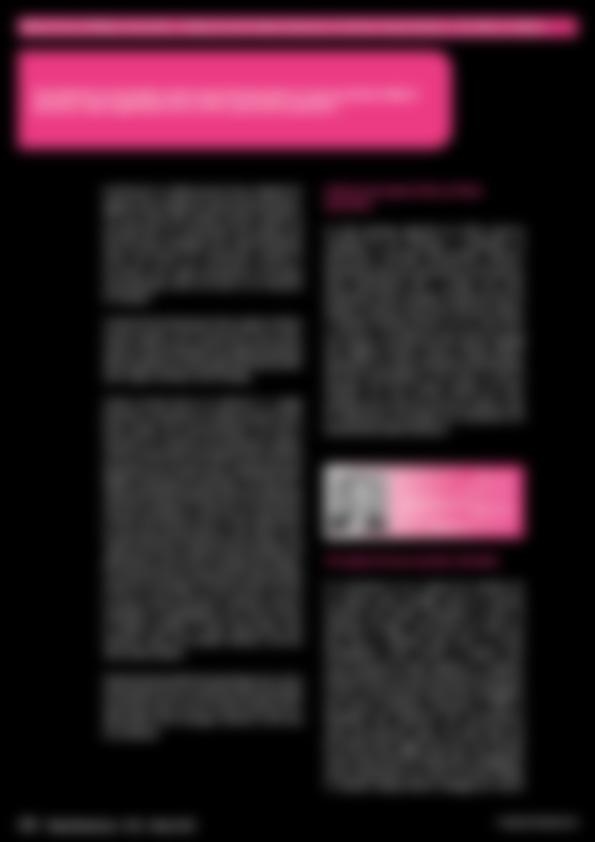
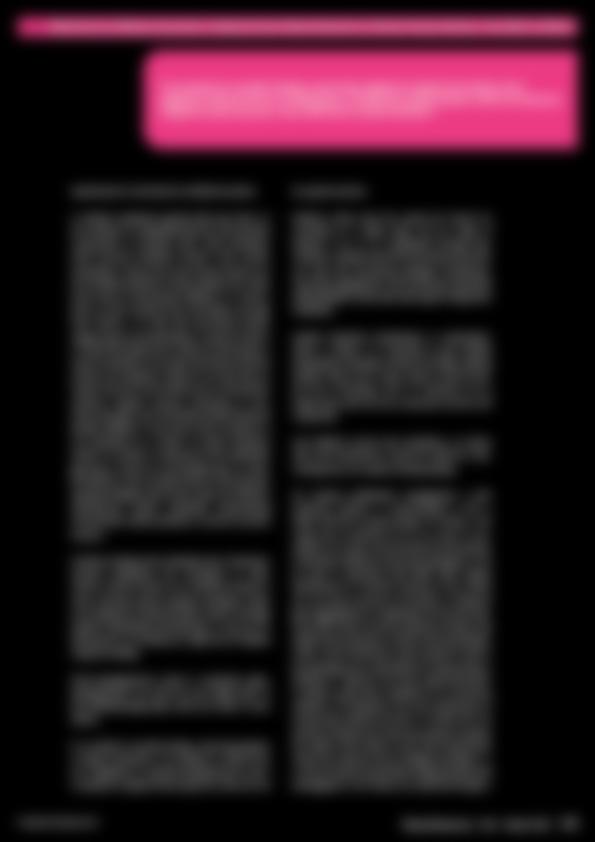
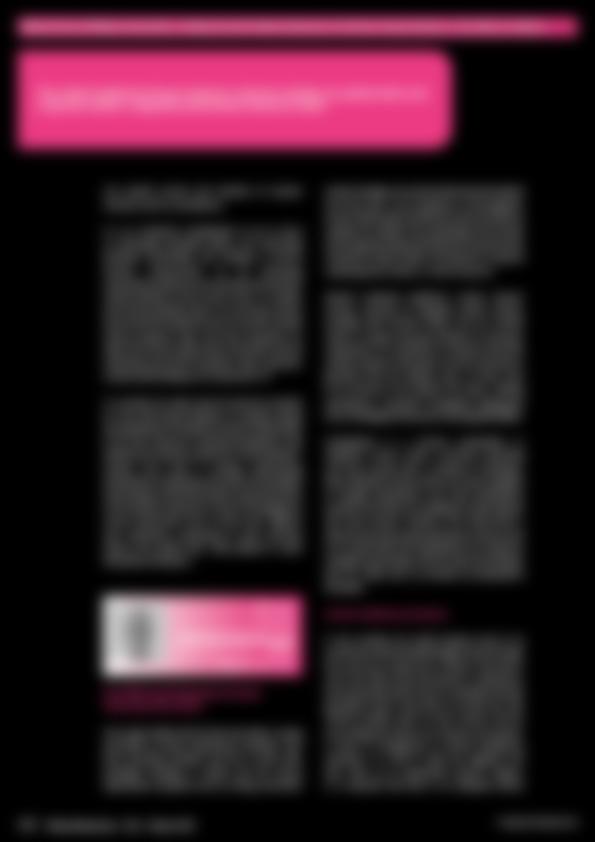
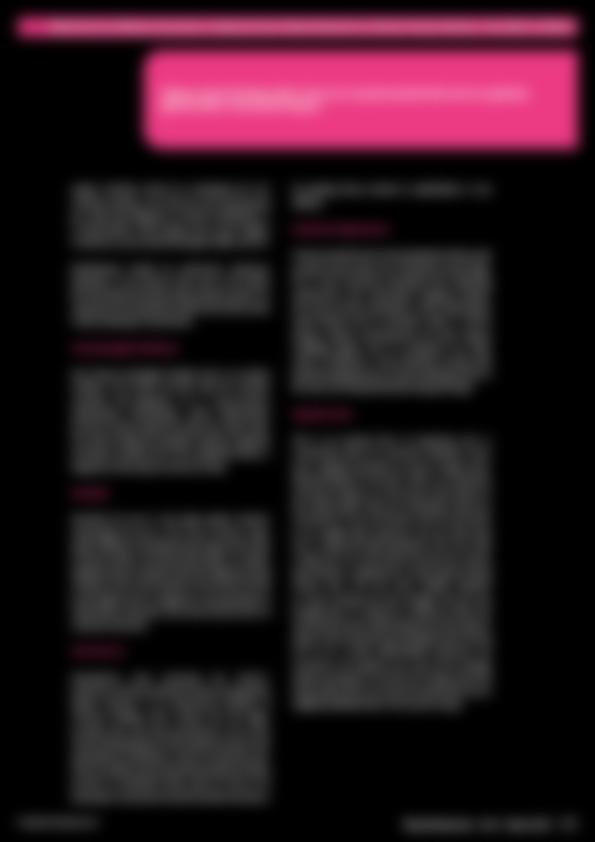
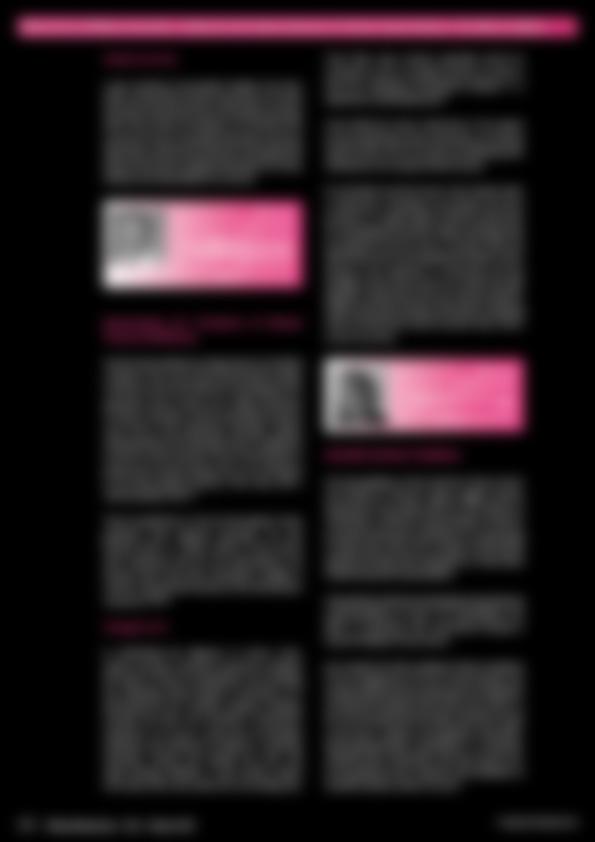
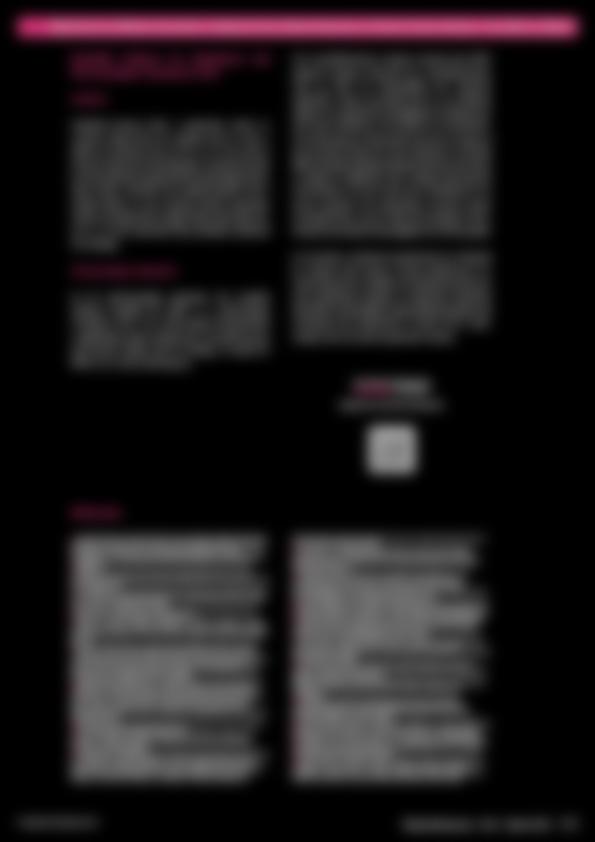
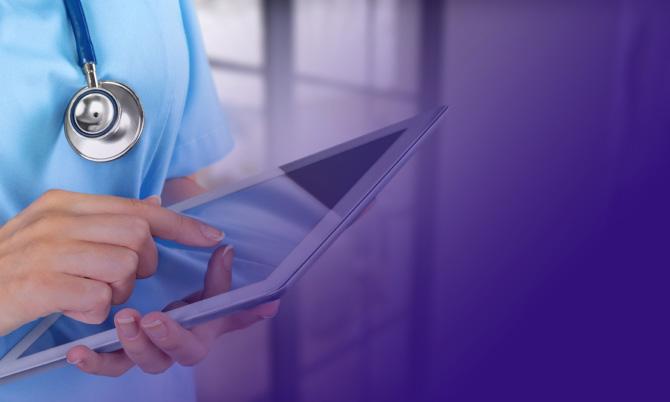
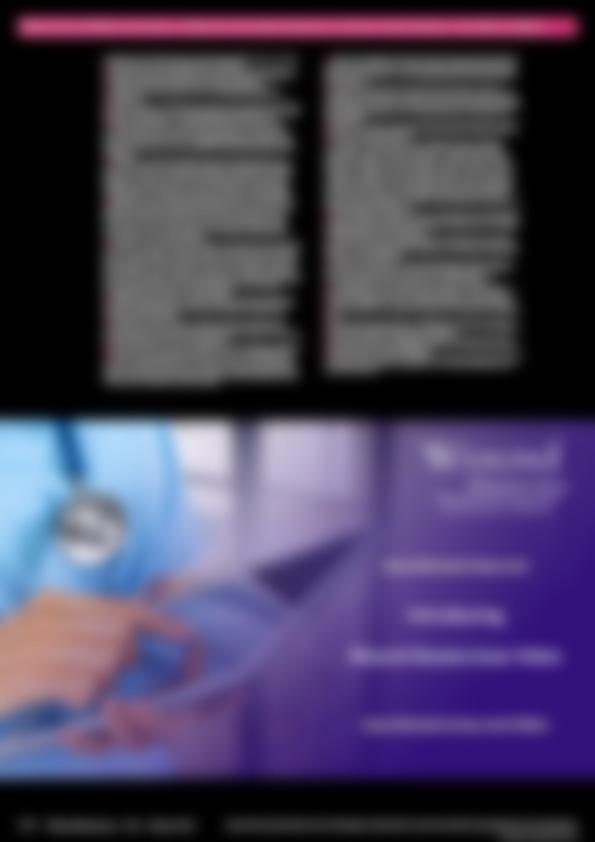
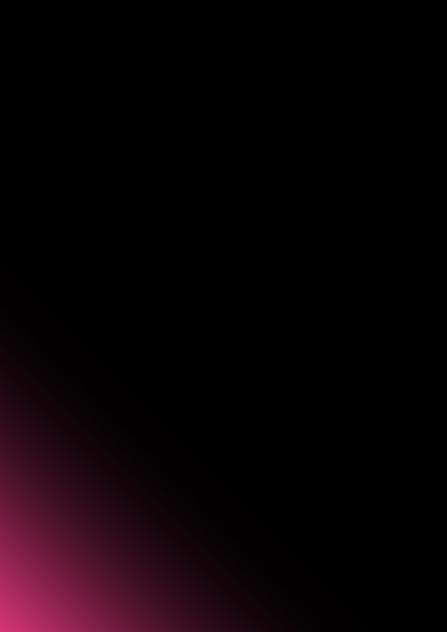
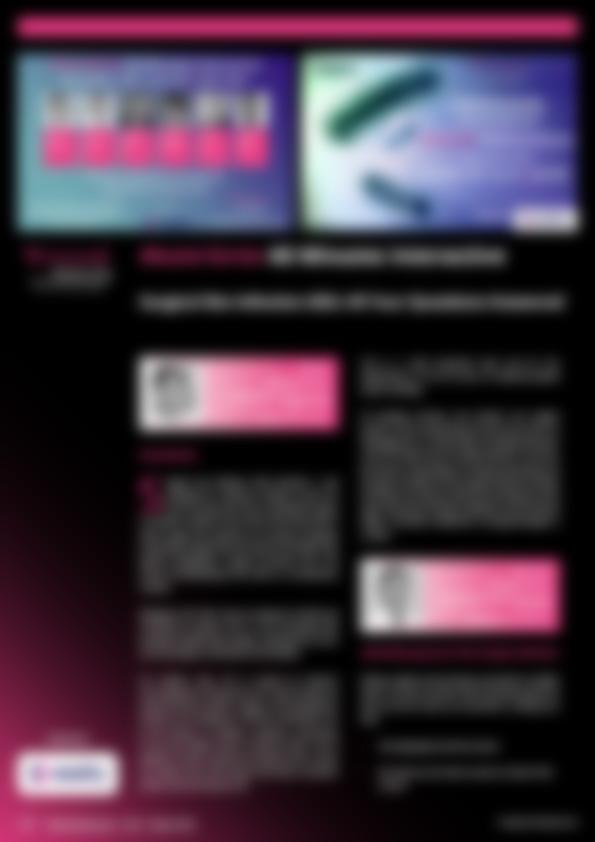
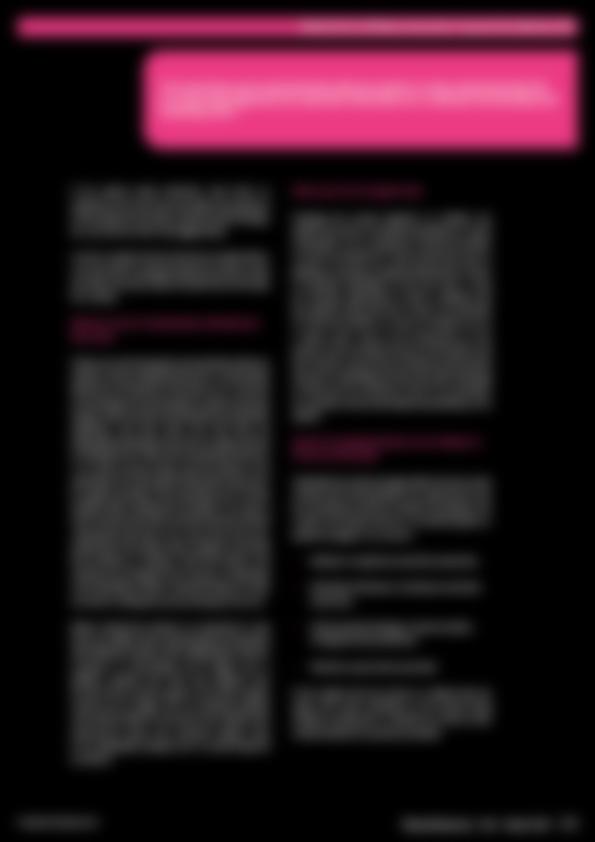
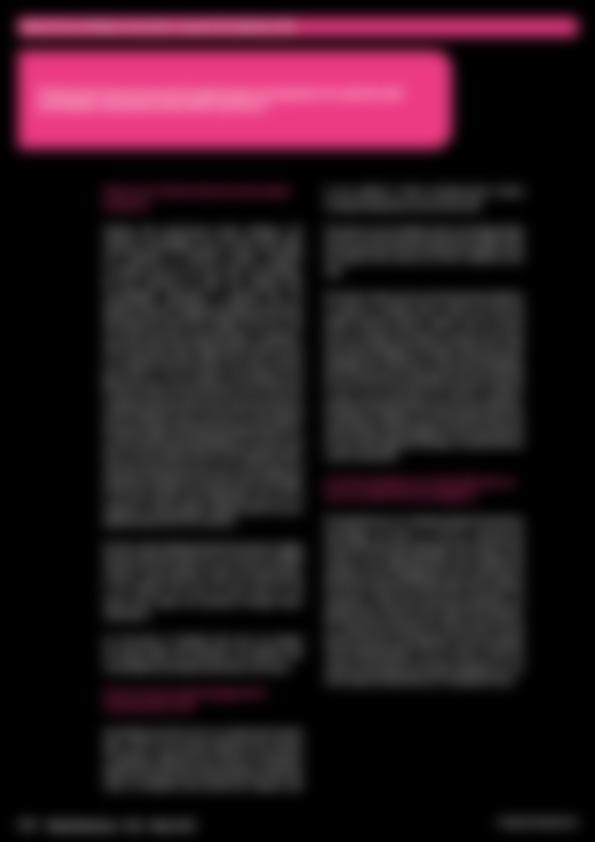
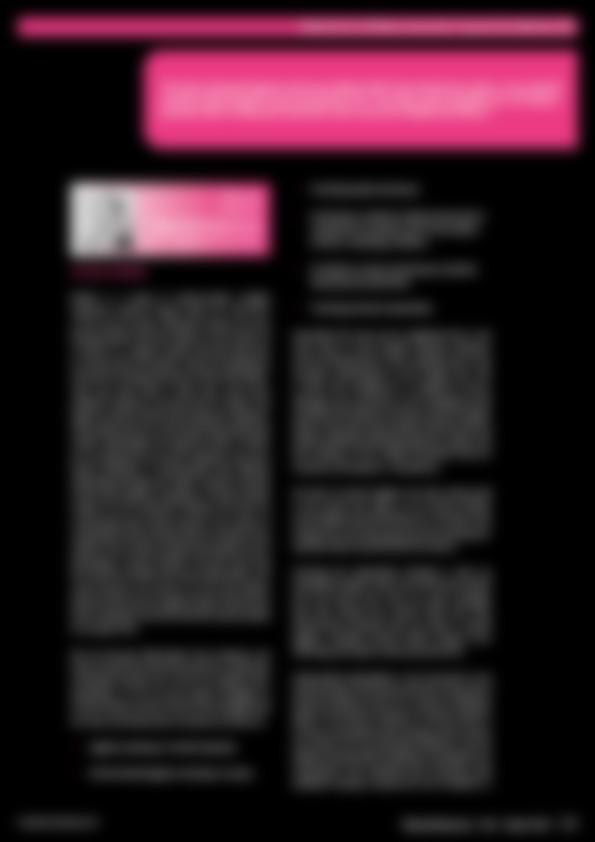
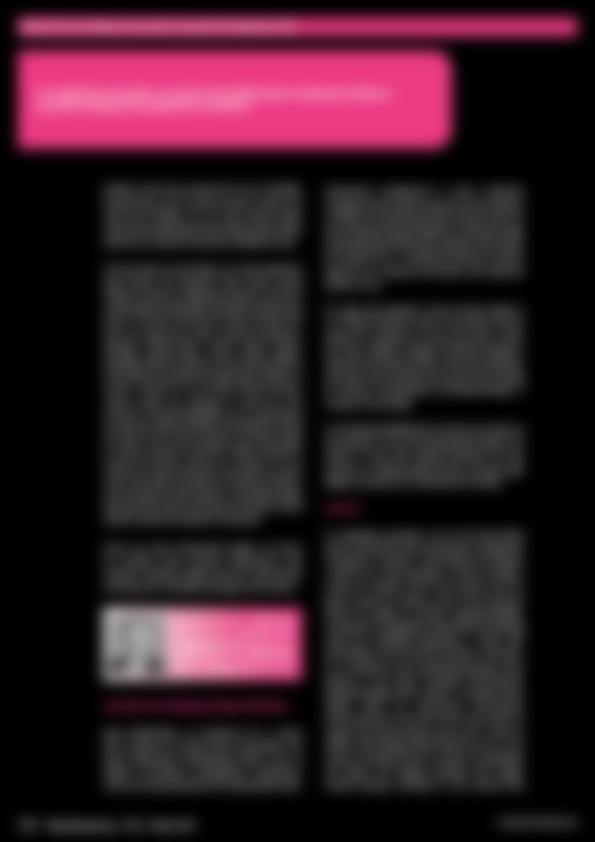
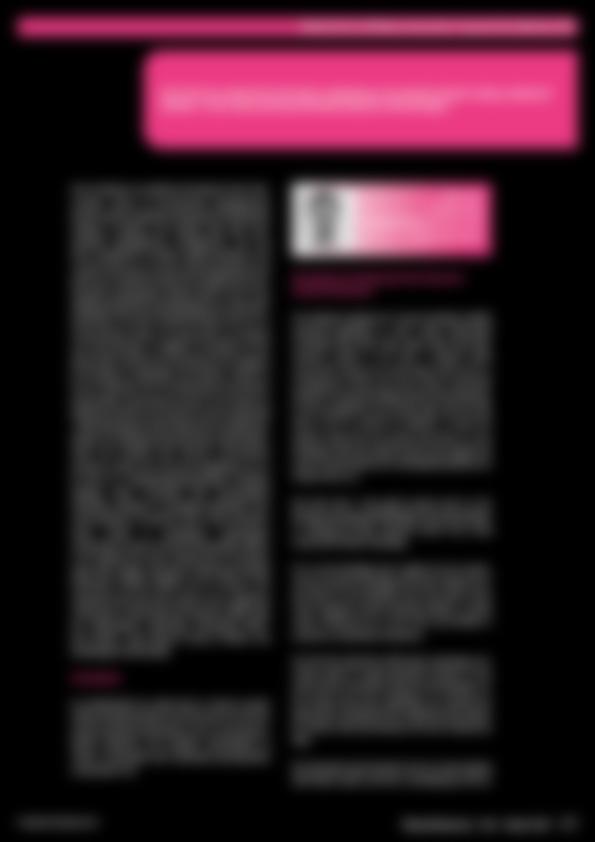
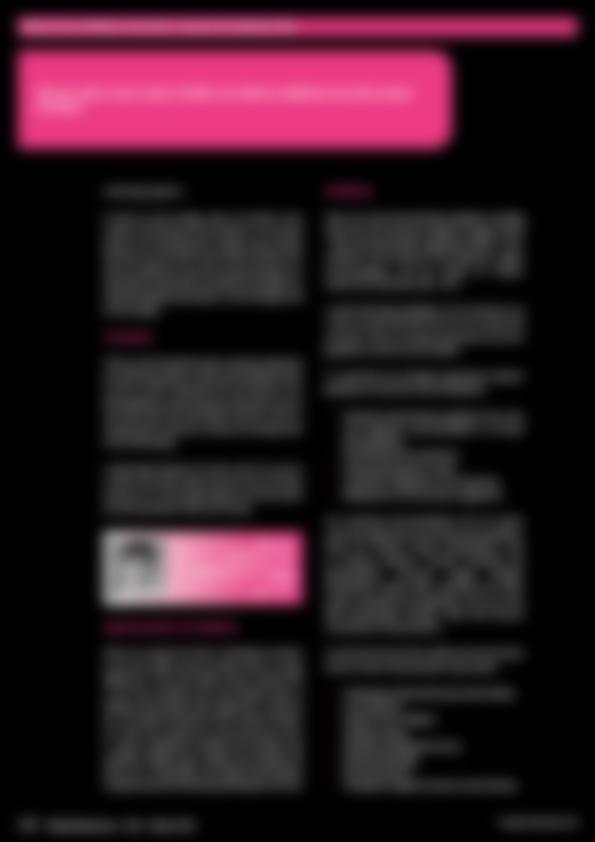
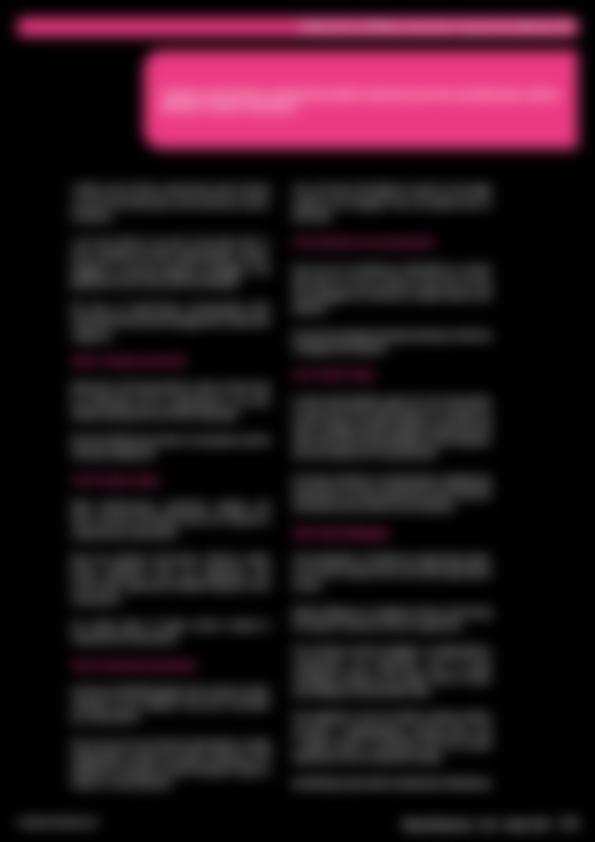

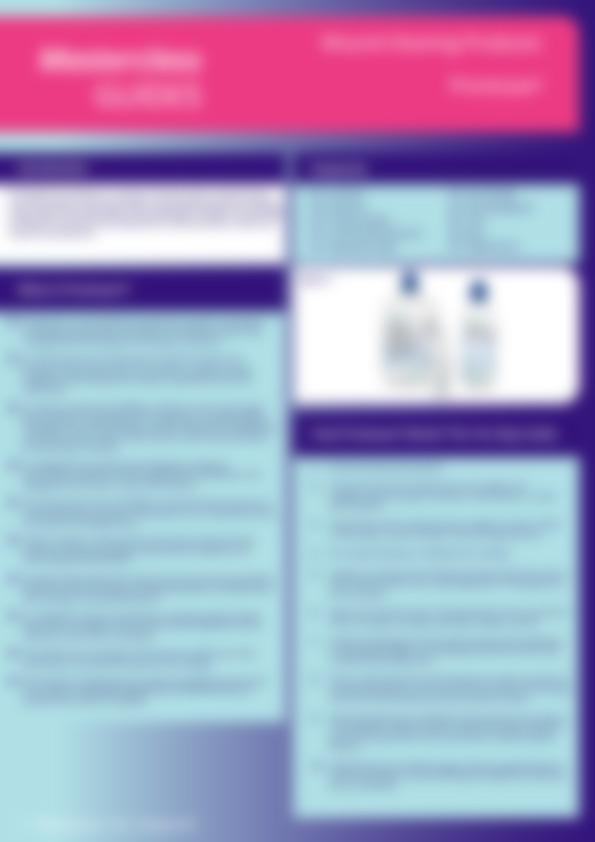
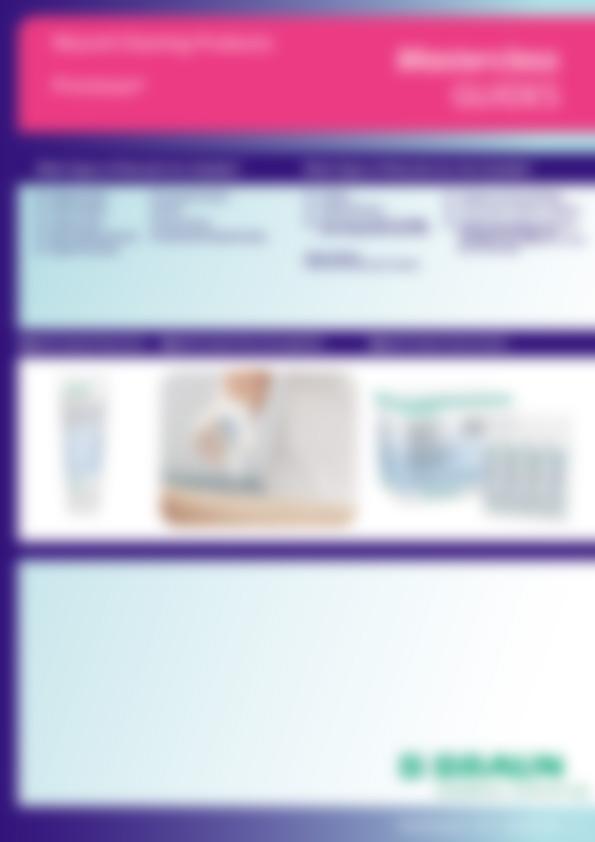
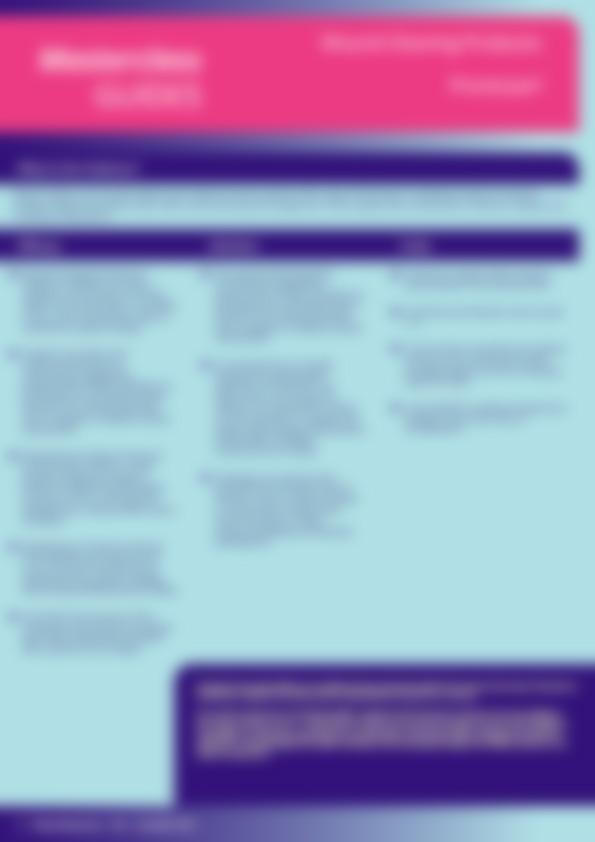
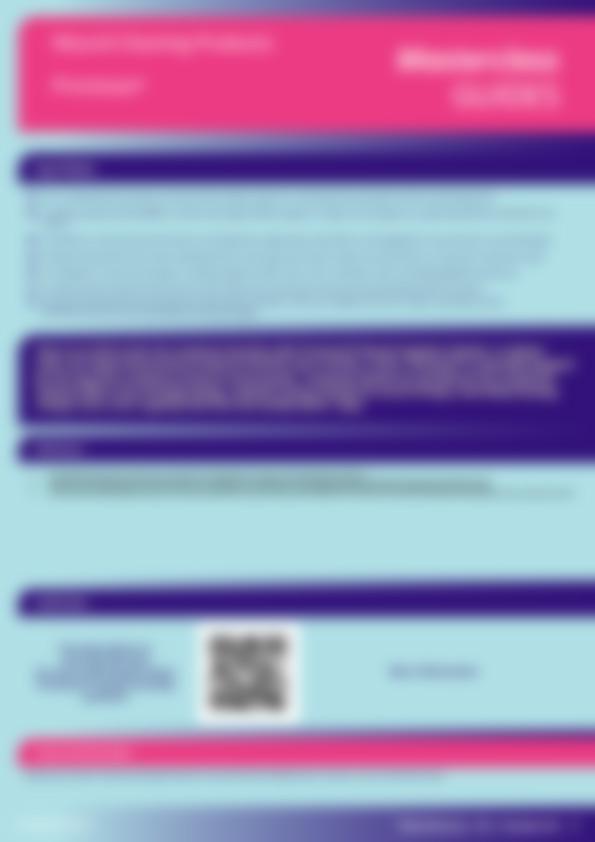
Bioactive Glass Wound Matrix
GUIDES
Introduction
This comprehensive masterclass guide offers an in-depth look at the Mirragen® Bioactive Glass Wound Matrix (BGWM) designed to support wound closure. Discover insights into the product’s unique composition, mechanisms of action, and clinical applications. Discover suitable wound types for its use, master the proper usage techniques through step-by-step instructions and video, ensuring maximum efficacy and patient comfort. An evidence-based mini case series showcases the transformative power of this cutting-edge wound care solution in real-world clinical settings.
What Is Mirragen® Bioactive Glass Wound Matrix?
■ Mirragen® Glass Wound Matrix is a flexible and formable skin substitute that easily adapts to any wound bed. Made from natural elements, the porous fiber and microsphere structure absorbs wound exudate to maintain moisture balance.
■ Solely composed of bioabsorbable fibers and particles. It is a flexible and moldable wound matrix that can be easily customized to fit the wound bed. The fiber structure of Mirragen® Glass Wound Matrix allows it to absorb fluid from the wound and facilitate natural wound healing.
■ The material is biocompatible and will eventually be fully absorbed at the wound site. This resorption process is initiated by the exposure of the material to fluid at the wound site.
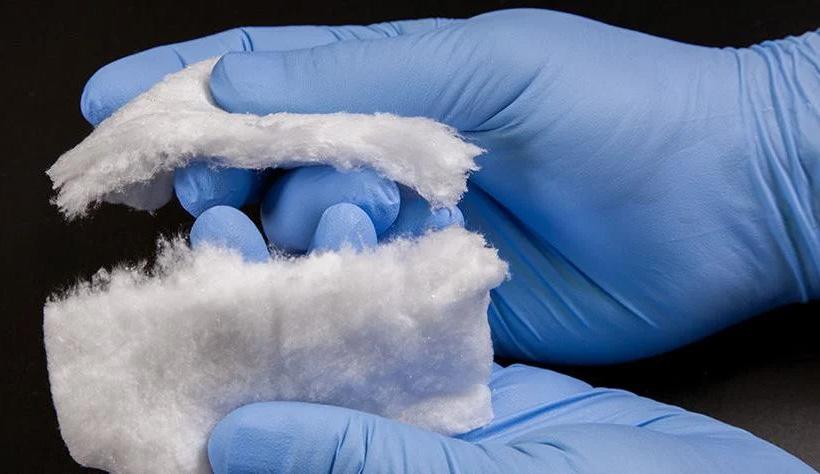
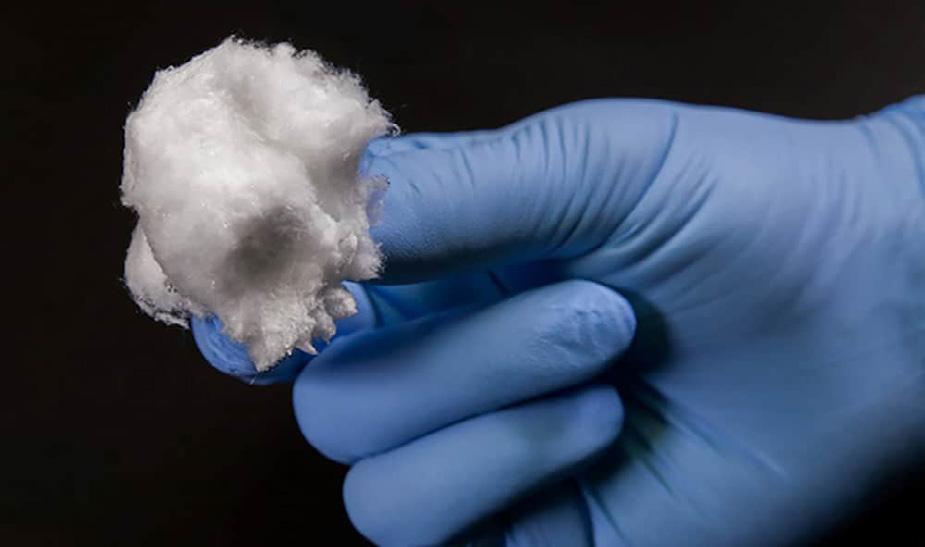
Keywords
■ Bioactive glass matrix
■ Wound
■ Wound care
■ Moisture Management
■ Wounds
■ Wound Healing
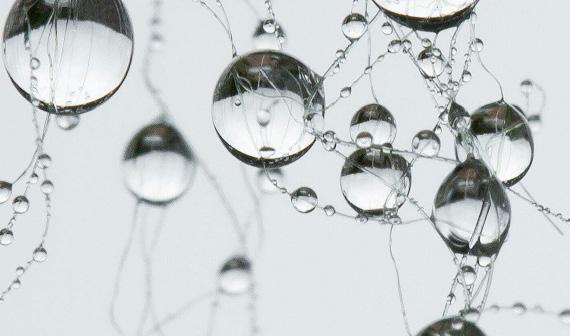
How to use Mirragen® Glass Wound Matrix
■ Mirragen® Glass Wound Matrix is sterile and aseptic techniques should be used.
■ Prepare the wound bed using standard wound cleaning and debridement procedures.
■ Dry the periwound area.
■ Cut or shape Mirragen® Glass Wound Matrix to fit the size of the defect, directly in the wound bed, ensuring full coverage.
■ If applying Mirragen® Glass Wound Matrix to a dry wound, moisten the wound bed and/or the material with sterile saline to aid in maintaining a moist wound environment.
■ Use appropriate fixation as determined by wound location, size and depth.
■ Add a non-adherent layer to secure Mirragen® Glass Wound Matrix to the wound bed.
■ Place a suitable secondary dressing over the Mirragen® Glass Wound Matrix to manage the wound environment and protect the wound area. The secondary dressing should completely cover the Mirragen® matrix.
■ Reapplication of Mirragen® and all secondary dressings should be conducted according to standard wound care practices every 3 to 7 days. Heavily exudating wounds may require more frequent dressing changes.
■ As healing occurs, new tissue may grow into Mirragen® Bioactive Wound Matrix. This is desirable, DO NOT forcibly remove or debride areas of Mirragen® that are embedded or adhered to the wound site, these are 100% bioabsorbable and will eventually be absorbed at the wound site.
■ Cut or shape Mirragen® Advanced Wound Matrix to fit the size of the wound bed. Place the material directly in the wound, ensuring the entire wound bed is covered.
Figure 2: Bioactive Glass: high purity raw materials are mixed and heated at extremely high temperatures
Figure 1: Mirragen® Glass Wound Matrix
Watch Video of Application
Bioactive Glass Wound Matrix
When Should I use Mirragen® ?
■ Venous ulcers
■ Pressure ulcers
■ Diabetic ulcers
■ Surgical wounds
■ Wound dehiscence
■ Trauma wounds
What are the Clinical Results So Far?
■ Calcitrant Wounds
■ Hard-to-Heal Wounds
■ Pyoderma Gangrenosum
The
The
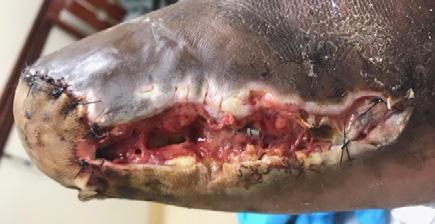
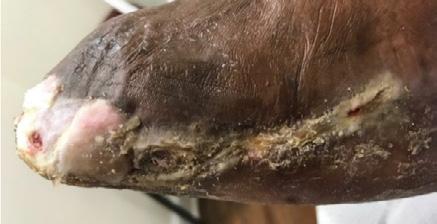
Mirragen® Bioactive Glass Wound Matrix was applied weekly to the wound bed and affixed using a non-adherent silicone dressing, then wrapped with gauze.
At weekly reviews, the patient returned for wound check and reapplication.
The wound was not disturbed on subsequent visits and did not require any additional debridement.
Mirragen® was reapplied at each visit and secured in similar fashion.
The wound progressed towards closure after only six applications of Mirragen® within 42 days
The clinician was extremely impressed with how effective Mirragen® was at providing the right environment to support the body’s ability to heal this wound, particularly after other failed treatments.
The provider found dressing changes were much quicker and simpler compared to other skin substitutes and was impressed with how easy it was to apply Mirragen® to the wound bed.
During
The
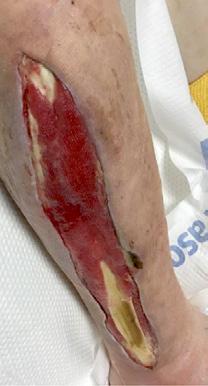
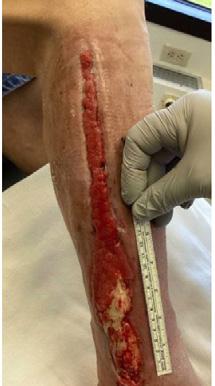
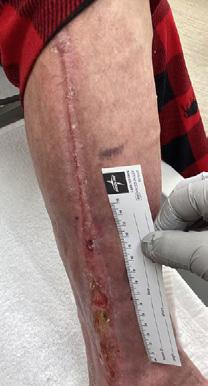
The patient also had the following comorbidities, which contributed to delayed healing: diabetes, renal failure on dialysis, stroke, and peripheral vascular disease resulting in amputation.
Initially the complex wound measured 29 cm x 4 cm 116 sq cm with desiccated necrotic tendon and fascia.
The wound, necrotic tissue and tendon were debrided, and Mirragen® Bioactive Glass Wound Matrix was applied to the wound bed
Weekly reviews performed and no additional debridement required.
Mirragen® was applied once per week and after 18 weeks of Mirragen®, this complex and hard-to-heal wound is closing
Measuring 3 cm x 0.5 cm, 1.5 sq cm the wound was noticeably clean and improving at week 18 and mirragen® was discontinued and standard of care was administered until the wound closed.
The provider was satisfied with the support Mirragen® provided to the body’s natural healing process and ease of placement wound bed.
The
The
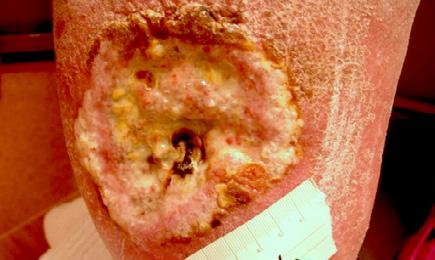
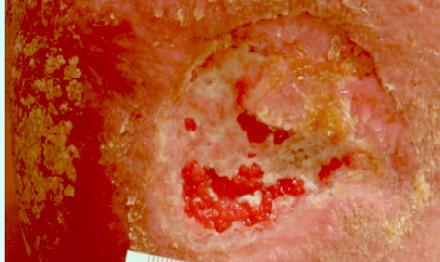
At week 1, the wound measured 8 cm x 8 cm x 2 cm, with a wound surface area of 64 sq cm
Mirragen® Bioactive Glass Wound Matrix ® was applied to the wound bed at weekly intervals.
At each visit, the wound was cleansed and debrided. Mirragen® was applied and covered with a non-adherent foam dressing.
Mirragen® was applied to the wound seven times over a 56 day period.
The wound progressed towards closure with seven applications of Mirragen® over 56 days.
The patient noticed early on that the odor from her wound had decreased significantly after just a couple of applications of Mirragen® and dressing changes were painless and comfortable.
This helped to ensure patient compliance with weekly visits to the clinic.
Mirragen®’s synthetic bioactive porous glass fibers, causes wicking of body fluids which aids in the placement of the product and once in the wound bed, mimics a fibrin clot, a well-documented healing process, allowing the body’s natural healing process to proceed.
was
contusion, from a offroad dirt bike accident with a delay in primary treatment of over 30 hours.
Pressure from the motorcycle caused compartment syndrome of the right leg
He underwent decompression surgery of his right leg. He was transferred intense physical rehab and continued wound care. The wound surface area was initially 112 sq cm
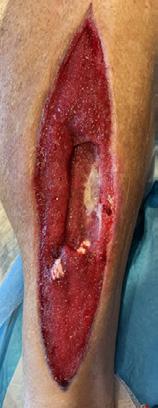
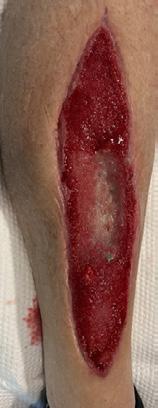
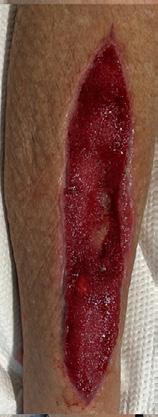
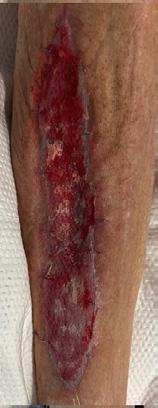
NPWT was used 3 times a week over exposed tendon with twice daily intensive physical therapy.
Mirragen® was used for tendon coverage and STSG was then performed.
Mirragen® was applied to the wound bed with contact layer and NPWT for 4 days post op.
Conversion to standard dressings occurred by Week 3 in this challenging wound resulting from acute spinal cord injury and multiple trauma to right lower extremity, due to healing progression.
All wounds, including both donor and skin graft sites, progressed towards closure in 3 weeks with 3 applications of mirragen® There was no evidence of scar contraction and there was improvement in the graft.
Mirragen® was selected because of its effectiveness at managing the wound environment, while supporting the body’s natural healing process and minimizing the need for multiple weekly dressing.
■ Atypical wounds
■ Undermined wounds
■ Skin graft donor sites
A 46-year-old
Past medical history included lower limb amputation due to PG. A non-healing wound was present for 6 years.
The dermatology team instigated steroids, STSG and a skin substitute, without success.
One further year of treatment with skin substitutes and multiple collagen products.
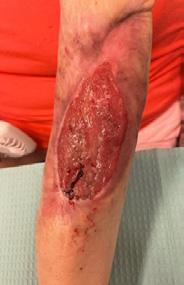
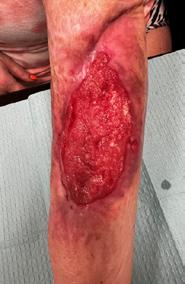
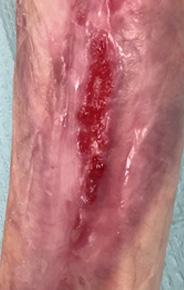
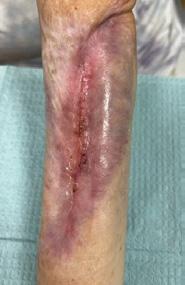
A plastic surgeon discussed other options including grafts or amputation.
Two weeks before scheduling the split thickness graft treatment commenced with Mirragen® Bioactive Glass Wound Matrix.
The wound was 8.2cm L x 5 cm W x 0.2 cm D, with a red, odorous, non-granulating, and painful wound bed and high exudate.
The wound was cleansed with normal saline, dried with gauze and Mirragen® was applied to the wound bed, covered with a nonadherent perforated dressing, and steristrips.
After the first two weeks, of Mirragen® the secondary dressing was decreased to one every 5 days, for exudate management.
A superabsorbent dressing with borders was applied
Wound progressed to closure at 18 weeks with applications of Mirragen® with a significant decrease in pain reported by patient.
The provider was able to clean the wound without pain and dressing changes were significantly faster
A significant decrease in product use because dressing changes reduced from 4 times a week to once a week.
On the last visit, the provider mentioned that the use of Mirragen® prevented an amputation on this patient.
woman with Pyoderma Gangrenosum (PG) of the right arm
Diabetic Ulcers Refractory Wounds
Venous Leg Ulcers
Surgical Wounds
Atypical Wounds
Bioactive Glass Wound Matrix Mirragen® Masterclass GUIDES
What
Is the Evidence?
The potential benefits and evidence supporting the use of Mirragen®, a novel bioactive glass wound matrix (BGWM) in the treatment of chronic, non-healing wounds is set out in the clinical evidence. The data, collected from various published retrospective case series, randomized controlled trials, and economic analyses, highlights the ability of BGWM to improve healing times, reduce costs, and streamline wound care practices.
Time to Healing Cost Reduction
■ In a randomized 12-week treatment after 2 weeks of screening, BGWM showed a favorable outcome in the treatment of patients with diabetic foot ulcers compared to standard of care (SOC) treatment.2
■ In a randomized trial of 40 patients receiving SOC or SOC plus BGWM for 12 weeks, a significantly higher proportion of wounds healed after 12 weeks of BGWM treatment at 70% compared to SOC alone (70% vs. 25%; adjusted P = .006).2
■ In a retrospective case series, five patients with 6 venous leg ulcers were treated all wounds healed after an average of 10.0 weeks (range: 3-27 weeks) during the use of BGWM, despite being present for an average of 15.1 weeks prior to initial BGWM application.7
■ A prospective pilot study involving three patients with a total of eight chronic, treatment-resistant pyoderma gangrenosum (PG) ulcers demonstrated that all previously non-healing wounds were returned to a healing trajectory following the introduction of BGWM therapy. 8
■ The application of BGWM resulted in the successful healing of all previously non-healing PG wounds, with patients experiencing significant pain reduction and no adverse events. 8
■ BGWM accelerates wound healing, with a wound closure rate of 6 weeks compared to 17 weeks with standard of care, reducing wound duration by 336 days. 2
■ In another case review, three patients with a mean age of 72 (range: 56-84) were treated for two surgical wounds and one radiation wound. All three wounds in this series had failed multiple previous advanced, costly therapies and surgeries with a mean wound duration of 13.2 months. Mean wound size volume at the start of BGWM was 1.9 cm³. All wounds healed after a mean of 8.1 weeks with the use of BGWM. 9
■ The result of a small case series suggests that BGWM has a success rate that exceeds the commonly reported industry standard of 50% and 70% of wounds healed; further, it could facilitate an earlier transfer of wound care patients into outpatient care, reduce healthcare costs, and improve quality of life. 10
■ Based on the physiologic properties of bioactive glasses at the wound interface, this innovative BGWM advanced wound matrix accelerates healing while minimizing the costs of care and improving outcomes.10
■ Mirragen® reduces time to heal by 336 days compared to standard of care. 12
■ Mirragen® has 2.8x faster closure rate than SOC12
■ In the case series of patients with venous leg ulcers, no systemic antibiotic therapy was required during the course of treatment with BGWM, suggesting a potential role for the matrix in managing wound bioburden and preventing secondary infections). This finding is noteworthy, given the concerns over antimicrobial resistance and the associated costs of antibiotic therapy. 7
■ The absence of systemic antibiotic therapy during the course of treatment with BGWM in patients with chronic PG ulcers highlights the potential role of the matrix in managing wound bioburden and preventing secondary infections, 8 which could lead to cost savings by avoiding the need for additional antibiotic treatment.
■ The ease of BGWM application and its ability to promote wound closure without requiring frequent dressing changes is advantageous from a clinical workflow perspective. 2 ,7 This streamlined approach to wound care may potentially reduce costs associated with frequent dressing changes and clinical visits.
■ Mirragen® results in 38% lower total costs of care over 40 weeks compared to standard of care 2,12
■ Mirragen® requires 53% fewer nursing/physician visits, contributing to a reduction in labor costs and enhanced cost-effectiveness 12
■ Mirragen® has 35% lower product costs due to a lower average application need, resulting in reduced overall product costs for healed wounds 12
■ Mirragen® leads to 78% lower unhealed wound costs over time, reducing long-term costs for unhealed wounds and saving an average of $8,377 per patient over 40 weeks 12
■ The estimated cost savings per patient between alternate advanced wound therapies and mirragen is $84,000 12
■ The total cost of care (TCOC) of using Mirragen®, compared to standard of care (SOC) for the treatment of diabetic foot ulcers over a 40 week period was modeled based on results from a 40 patient RCT 2,12
■ Results showed the TCOC for healed patients was $9,233 for Mirragen® versus $20,573 for SOC. The TCOC over the initial 12 week study was $6,925 per patient for Mirragen® and $5,143 for SOC. When including the cost for unhealed patients over 40 weeks, the total TCOC was $13,698 per patient for Mirragen® compared to $22,074 for SOC 12
■ The study concluded that use of Mirragen® for diabetic foot ulcers resulted in an estimated cost savings of $8,377 per patient over 40 weeks compared to standard of care. Mirragen® was found to be a cost-effective option for wound healing 2
■ An economic analysis found use of Mirragen® to be costeffective, with expected cost savings of $8,377 compared to standard care when evaluating total wound healing costs over 40 weeks. 2
■ Potential reduced need to utilise Negative Pressure Wound Therapy (NPWT) costing $119/ day. 12
■ A recent systematic review highlighted that the mean cost of treating diabetic foot ulcers (DFUs) surpasses $31,000, underscoring the economic burden associated with these wounds. 2
Frequency of Dressings
■ In a randomized controlled trial, Mirragen® doubled the wound area reduction as 12 weeks (79.4% vs. 36.5%) as compared to the standard of care (collagen alginate dressing)2
■ In the case series of patients with venous leg ulcers, compression wraps consisting of two layers were applied over the BGWM dressings and changed twice weekly at each study visit, along with inspection and reinforcement of the underlying BGWM application as needed. This protocol suggests that BGWM requires less frequent dressing changes compared to standard wound care practices. 7
■ The study involving patients with chronic PG ulcers mentioned that the BGWM was easy to apply and was used until complete wound closure was achieved for all ulcers 8, indicating a reduced need for frequent dressing changes.
■ The ability of BGWM to facilitate wound closure without requiring frequent dressing changes is mentioned as an advantage in the management of both venous leg ulcers and PG ulcers, 7 highlighting the potential of the matrix to streamline wound care and reduce the burden of frequent dressing changes on patients and healthcare providers.
■ These detailed findings, supported by the respective documents, underscore the potential benefits of using BGWM in the treatment of chronic, non-healing wounds, particularly in terms of accelerated healing times, cost reduction through decreased need for systemic antibiotics and frequent dressing changes, and a reduced frequency of dressing changes compared to standard wound care approaches.
Bioactive Glass Wound Matrix
Key Points
■ Mirragen® Bioactive Glass Wound Matrix mimic the microstructure of a fibrin
■ Mirragen® Bioactive Glass Wound Matrix is biocompatible with
and bioresorbable. ■ Mirragen® Bioactive Glass Wound Matrix is free of toxic or irritant particles
■ Bioactive Wound Glass fiber absorbs wound fluid to maintain moisture balance
■ Material covers the wound to support a clean environment
■ Mirragen® Bioactive Glass Wound Matrix dissolves over time, eliminating the need to disrupt healing by removing material
■ Fibrous structure allows for gaseous exchange at the wound site
■ Bioactive Wound Glass fiber can conform to any wound shape
■ Mirragen® Bioactive Glass Wound Matrix is synthetic, eliminating the concern over potential disease transmission unlike biologic or cellular tissue-based products
■ Material is stable at room temperature and requires no special storage conditions
■ Mirragen® Bioactive Glass Wound Matrix has a long shelf life of 5 years, and can be stored at room temperature
■ No preparation steps required
■ No tissue tracking required
“I have used Mirragen® on one of my most difficult cases where other skin substitutes did not work. I am impressed by Mirragen®, and I have not been impressed in a long time.” Dr Laurentin Perez
Biodegradable and bioabsorbable
Made with natural body elements, Mirragen® dissolves in physiological fluid, so there’s no need to remove it.
Easy to place
Because it’s flexible, easy to use, and will attach to a moist surface, Mirragen® is ideal for challenging wounds, including those that require tunneling or undermining.
Proven safe and effective
Mirragen® has shown great clinical results for a variety of wound types, from easy to heal to complex. In a randomized controlled trial, no adverse events were observed.
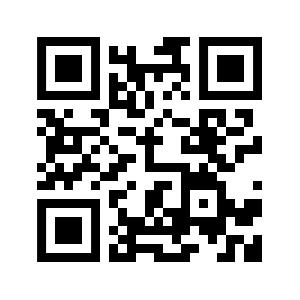
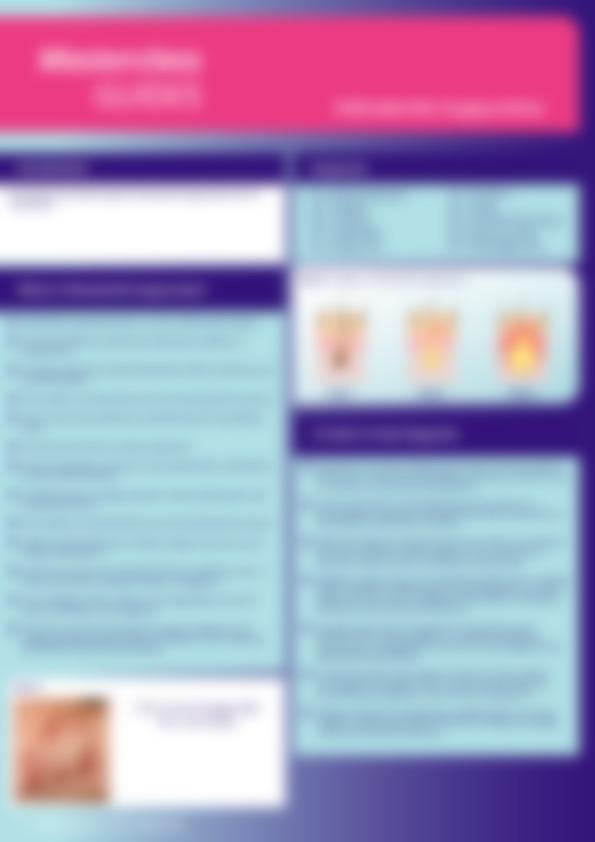
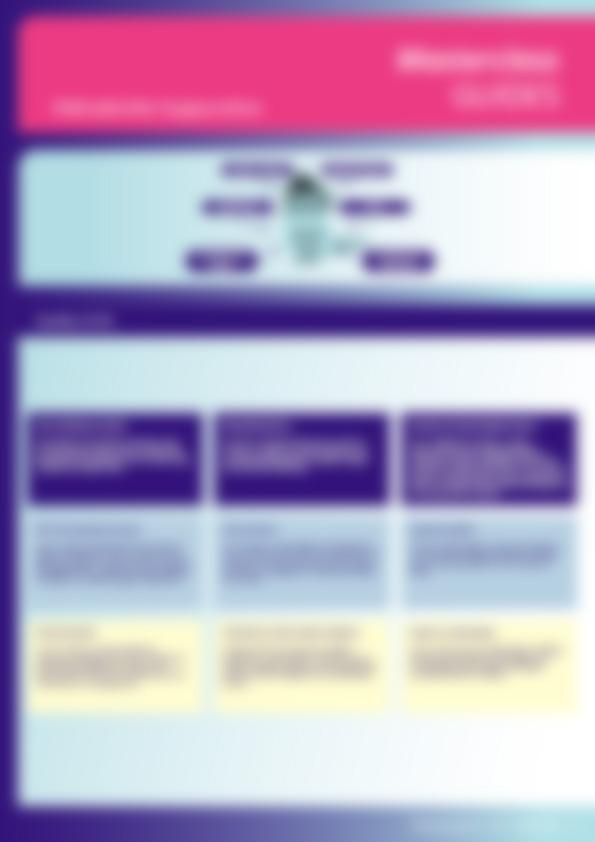
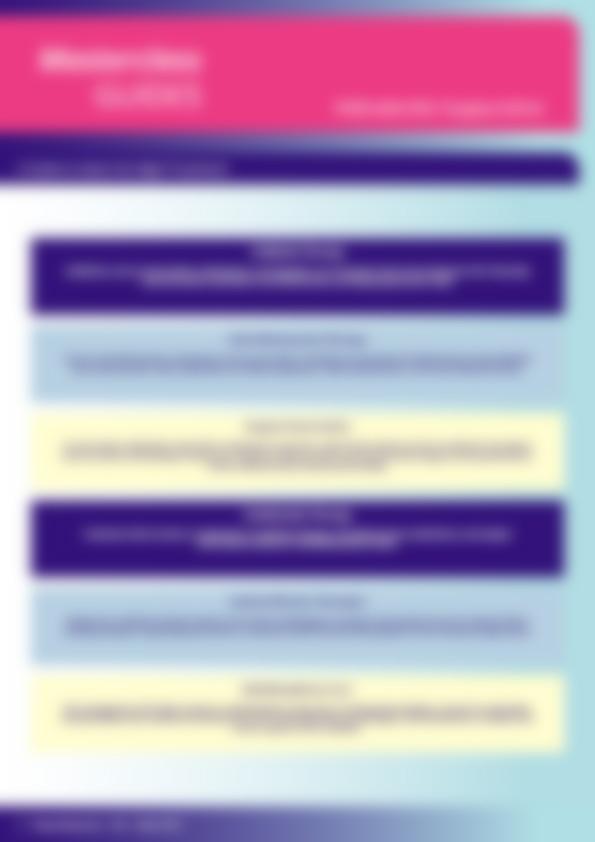
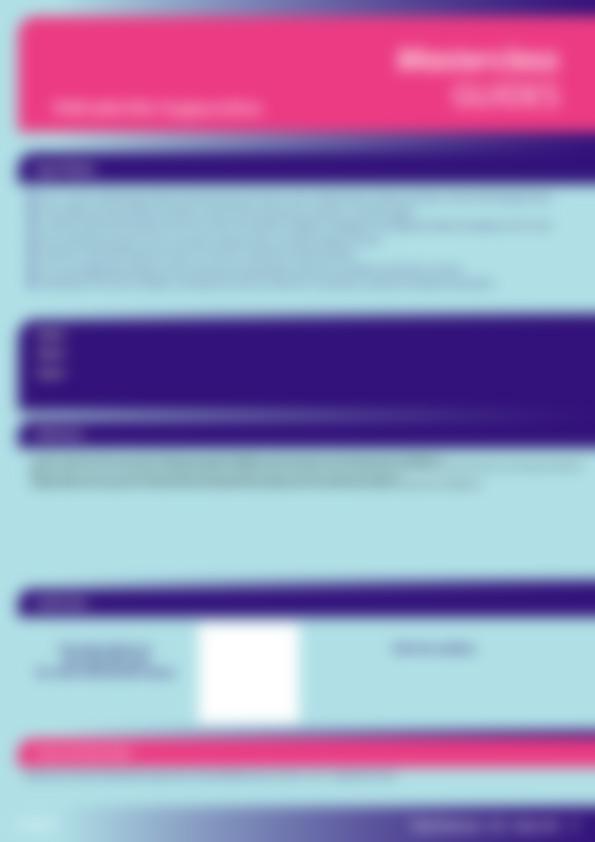
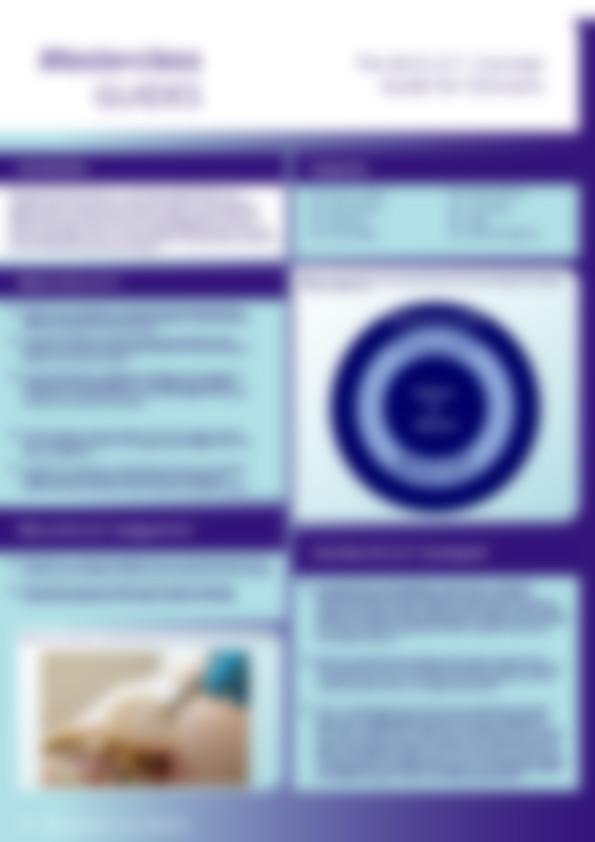
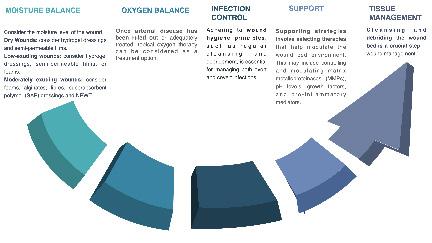
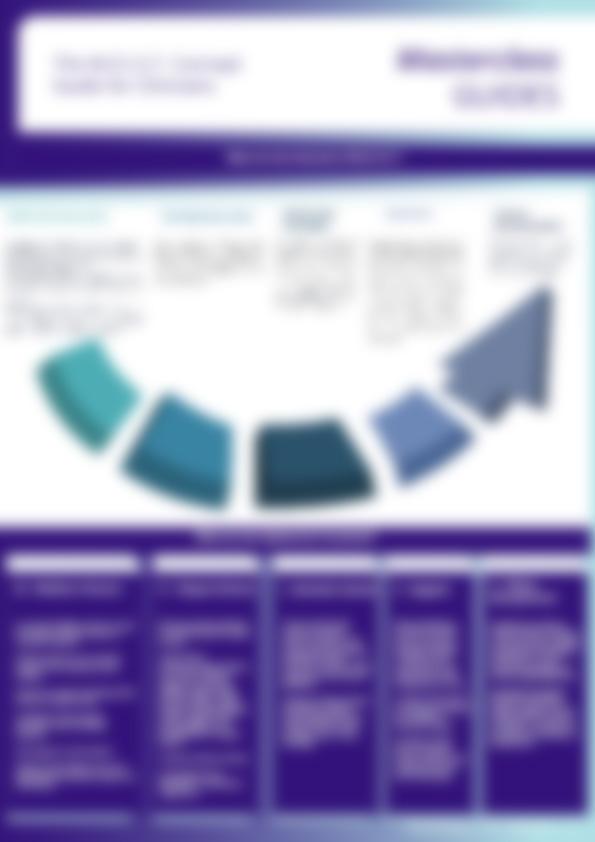
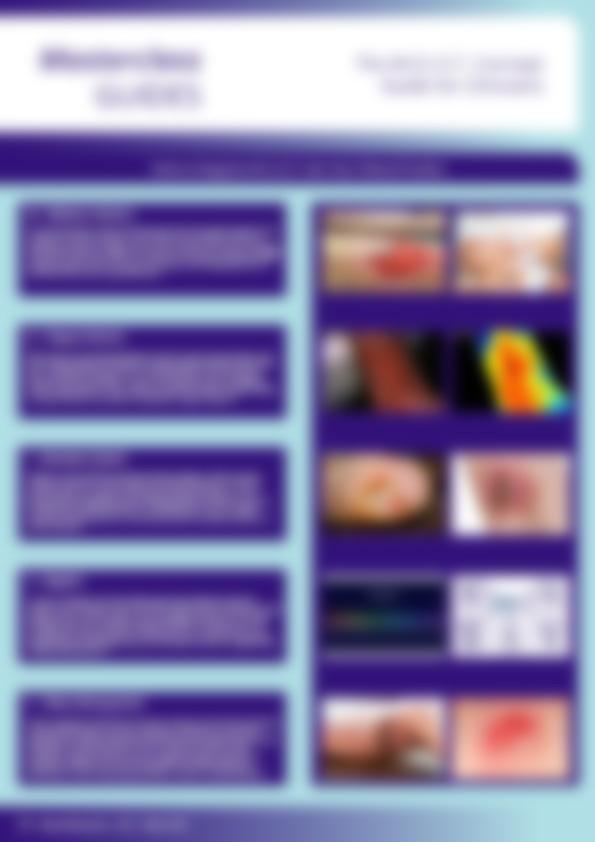
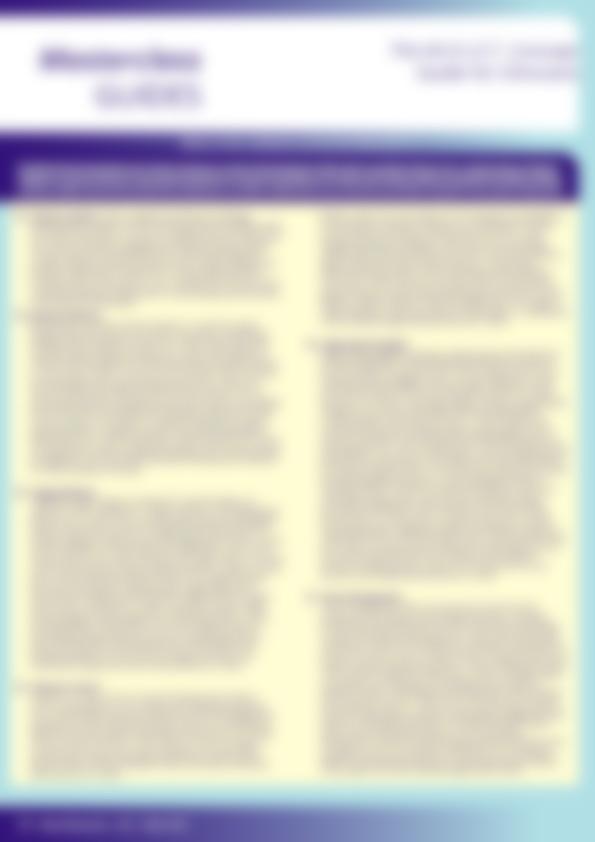
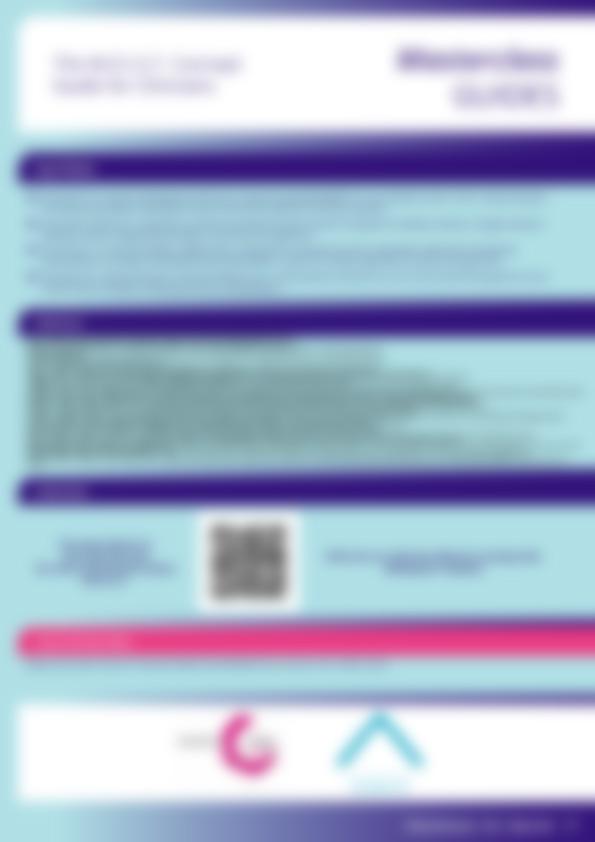
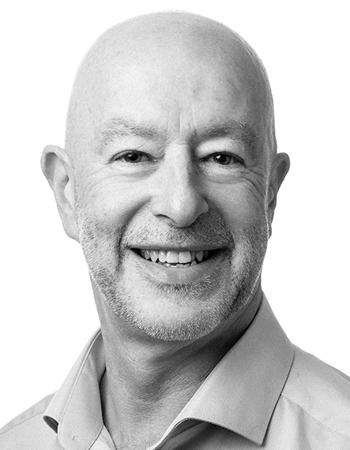
Bioelectric Stimulation, Electric Fields and the Current of Injury: Have We Been Overlooking One of the Most Important Coordinators of the Wound Healing Process?
Editorial Summary
This article presents a critical examination of bioelectric stimulation, electric fields, and the current of injury as potentially overlooked yet fundamental coordinators in wound healing. It elucidates the physiological basis of bioelectricity in cellular processes and its implications for clinical wound management.
The review explores the role of membrane potentials, transepithelial potentials, and bioelectric signaling in modulating key cellular behaviors crucial to healing, including proliferation, migration, and differentiation. It introduces the concept of the "skin battery" and how its disruption generates a "current of injury," which initiates and sustains the healing cascade.
Compelling evidence suggests that compromised bioelectric processes may underlie impaired healing in recalcitrant wounds, particularly in geriatric and diabetic populations. The article critically evaluates the efficacy of exogenous electrical stimulation therapy (EST), citing meta-analyses demonstrating significant improvements in healing rates for hard-to-heal wounds.
While acknowledging the limitations of earlier EST devices, the authors highlight recent advancements in microcurrent technology that offer more practical and integrable solutions for clinical application. They posit that incorporating bioelectric-based therapies could potentially address the increasing prevalence of chronic wounds more effectively than conventional approaches focused solely on biochemical mediators.
This comprehensive review advocates for a paradigm shift in wound care strategies, urging clinicians to consider bioelectric factors as essential components in the multifaceted approach to wound healing.
Introduction
Delve into any Wound Management textbook and you will learn all about the biochemical basis of wound healing - the complex interplay between growth factors, cytokines, proteases, cell membrane receptors and so on, which drive the wound healing process. However, scientists are becoming clearer that while these factors are indeed important stimulators, a much more fundamental coordinator of healing behaviours that is triggered by wounding is not even mentioned in most wound management texts.1 We are referring to bioelectricity.
Imagine that the growth factors and cytokines are the musicians in an orchestra, then bioelectricity is the conductor, making sure they are all co-ordinated and working in harmony to restore skin back to pre-wounded state.
The purpose of this document is to describe the physiological basis of bioelectricity and to explain the fundamental importance of bioelectrical fields and currents in the wound healing process.
Let’s Start With the Basics; The Membrane Potential in Cell Biology
All higher organisms, including humans, are organised into organs, the basic units of which are cells. The membrane enveloping each cell separates the highly organised system inside the cell from the environment outside the cell and the composition of the fluid inside the cell is very different from the composition of the extracellular fluid. One key difference is that the levels of electrolytes, for example Ca2+, Na+, K+, Cl- ions, vary between the inside and outside of the cells.
Under normal conditions, more positively charged electrolytes (predominantly Na+ and K+ ions) are found outside the cell than inside the cell. In addition, proteins are generally negatively charged and these are retained inside the cell. The overall effect is that the cytoplasm inside the cell has an electrically negative charge compared with the surrounding extracellular fluid. The difference in this electrical charge on either side of the membrane is called the membrane potential and measures 40-80mV.2 Essentially, there is a potential difference between the inside and the outside of the cell meaning that each cell can act as a tiny battery that stores ‘bioelectricity’ (Figure 1).
Dr Robin Martin
Freelance Scientific Consultant, Robin Martin PhD Scientific Consulting
Foggathorpe, United Kingdom
“The membrane potential has a purpose; when something happens to disrupt it, this drives a movement of electrically charged ions, which then stimulate the cell to change its cellular processes and behaviours.”
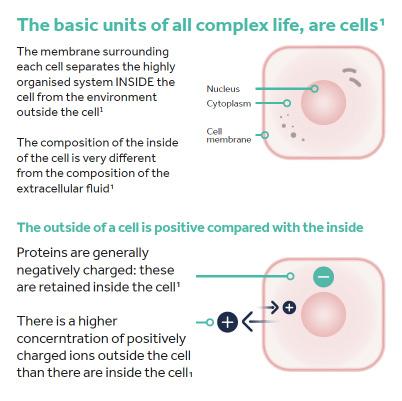
How Bioelectric Signalling Works
The membrane potential has a purpose; when something happens to disrupt it, this drives a movement of electrically charged ions, which then stimulate the cell to change its cellular processes and behaviours. We most often think about this happening in the context of nerve cells being stimulated to fire off a nerve impulse, but in reality these kinds of events happen in all cells in the human body.
Disruption to the membrane potential can happen in at least three different ways. Firstly, ion channels in the membrane can open, allowing ions to flood in or out, changing the charge across the cell membrane. Secondly, structures called gap junctions that connect neighbouring cells can open or close, either transmitting or blocking changes from one cell into its neighbours. Thirdly, minor physical damage to an individual cell’s membrane can allow diffusion of molecules from the inside or outside of the cell (Figure 2).2
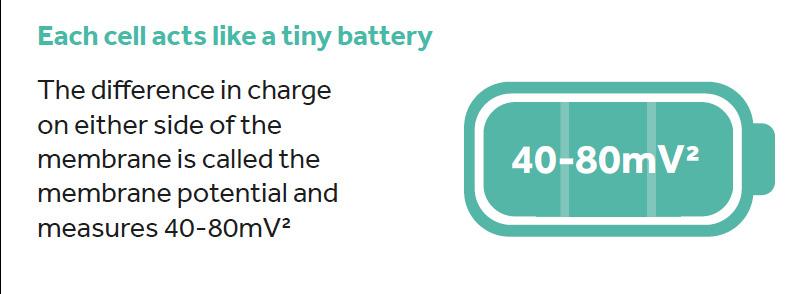
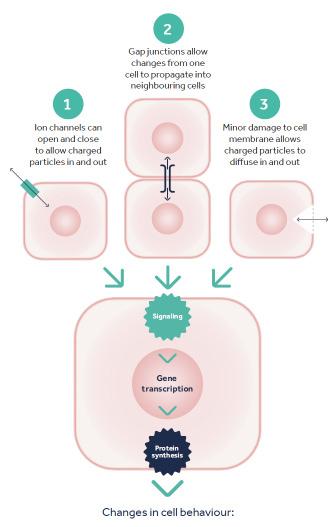
Figure 1: Membrane potential. All living cells, including human cells, have a different composition inside and outside their cell membranes. Differences in the concentration of charged molecules (like ions and proteins) between the inside and the outside of the cell generates a membrane potential. This is in effect a difference in electrical charge on either side of the cell membrane, which allows each cell to act as a tiny battery.
Figure 2: How disrupting the membrane potential can drive bioelectric signalling. Bioelectric signalling can be initiated in 3 main ways, all of which can result in signalling events inside the cell which trigger gene transcription, protein synthesis and essentially activate a range of cell behaviours.
“The electrical properties of epithelia are hardly ever mentioned in textbooks. Yet these properties are very important for organ function.” 1
In all of these scenarios, the membrane potential of the cell is suddenly changed by a movement of charged ions and proteins across the cell membrane. This change can trigger a wide variety of cellular responses and is collectively termed bioelectric signalling. When the membrane potential is disrupted, and the biochemical composition of the cytoplasm changes, receptors inside the cells respond to these changes by initiating gene expression and the synthesis of proteins through gene transcription and translation. Such cell activation can change the behaviour of a cell. Some changes triggered by bioelectric signalling include: cell proliferation, cell migration, cell differentiation and cell directional polarisation, among others. These are all cellular behaviours that are important drivers of the healing process.2
Transepithelial Potential and the ‘Skin Battery’
In the previous section we described how bioelectricity is created by the potential difference between different compartments, for example inside and outside the cell. But bioelectricity can also be observed across different compartments of tissue for example the outermost (apical) and innermost (basolateral) surfaces of epithelial tissues. Transepithelial potential has been measured in skin, gut epithelia, kidney and cornea.3
In the skin, the transdermal potential is commonly referred to as the ‘skin battery’. Intact skin has a transdermal potential of 15 to 50mV with the inside being positively charged relative to the outside (Figure 3A).4,5 The transepidermal potential is generated in the keratinocyte layers of the epithelium because of a net movement of sodium ions (Na+, positively charged) from the apical side of the cell (the outermost edge) through the cell, to the basolateral (innermost) edge.1,4,6 All cells have sodium channels, potassium channels and sodium-potassium pumps, and in most
cells these structures are randomly positioned around the perimeter of the cell. However, in keratinocytes that make up the epidermis, they are polarised with most sodium channels being localized to the apical (outermost) membrane, most potassium channels being found in the basolateral membrane (innermost layer of keratinocytes) and most sodium-potassium pumps also localised along the basal side where they pump sodium ions out of the cell in the direction of the dermis.1,4,6
The positioning of the sodium ions themselves is in constant flux; once they have been removed from the cell at the basolateral side, they try to diffuse down their concentration gradient towards the apical edge, however gap junctions that tightly bind keratinocytes to their neighbours, prevent free diffusion.6 The positively charged ions at the basolateral side are not able to diffuse away which leads to there being a trans-epidermal potential (Figure 3B).
Figure 3: How transdermal potential is generated in human skin. Figure 3A. Human skin is electrically charged with a relatively positive charge on the basolateral side of the epidermis (next to the dermis). This electrical charge has led to the skin being described as a battery. Figure 3B represents a layer of within the epithelium. Sodium channels on the apical side of the keratinocytes pump sodium into the cell. Sodium-potassium pumps situated on the basolateral side of the cell then eject the sodium from the cell. The sodium is not able to diffuse back to the apical side of the cell because it is blocked by the presence of tight junctions that tightly link keratinocytes to their neighbours and forms an impermeable barrier. Therefore, the basolateral side has a higher concentration of sodium ions and their positive charge generates a transmembrane potential that is positive on the basolateral side and negative on the apical side.
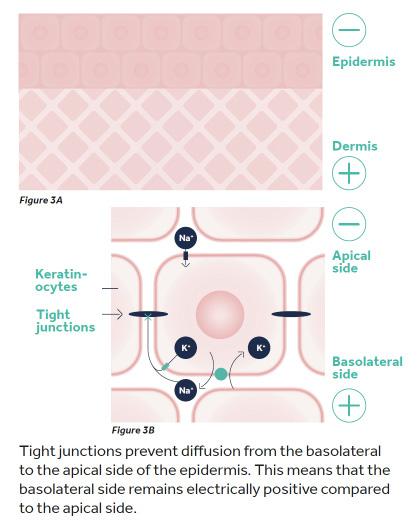
“All cells in tissue at the edge of the wound are exposed to this current of injury which stimulates their activity for several days after wounding. In normal wound healing, this wound-induced electrical field persists until the migrating epithelium reseals the wound and re-establishes tight junctions which create a uniformly high electrical resistance, at which point the wound-induced electrical field drops to zero and the transcutaneous potential, the skin battery, is restored.” 4-6
The ‘Current of Injury’
While intact skin acts as a battery of potential bioelectrical energy, what happens when the skin is wounded? Scientists have known the answer to this question for well over a century, since, in 1843, the scientist Dubois-Reymond first used a galvanometer on his own cut finger and identified that wounds had a measurable electric current.5 This has since been named the ‘current of injury’.
Wounding the epithelial sheet creates a hole that breaches the high electrical resistance established by the tight junctions between the cells, and this short-circuits the epithelium, in the local vicinity of the wound. The transdermal potential therefore drops to zero at the wound. However, because normal ion transport continues in unwounded epithelial cells beyond the wound edge, the transcutaneous potential difference remains at normal values around 2mm back from the wound.6 It is this gradient of electrical potential, 0 mV at the short-circuited wound bed, 15-50 mV a short distance away in unwounded tissue, that establishes a laterally oriented electrical field and drives a steady
bioelectric flow of current (Figure 4). This is termed the ‘current of injury’ and, in healthy individuals, is around 140mV per mm .4-6
All cells in tissue at the edge of the wound are exposed to this current of injury which stimulates their activity for several days after wounding. In normal wound healing, this wound-induced electrical field persists until the migrating epithelium reseals the wound and re-establishes tight junctions which create a uniformly high electrical resistance, at which point the wound-induced electrical field drops to zero and the transcutaneous potential, the skin battery, is restored.4-6
So, as the wound heals, the current of injury progressively reduces. The current of injury will also gradually decrease if the wound is left open to dry.7 Maintaining a moist wound environment has been recognised for decades as being vital for healing. Given that electrolytes are soluble and need fluid to diffuse, it is highly likely that moist wound healing is essential for the current of injury to exist. Seeing moist wound healing in the context of the movement of electrolytes and the current of injury, provides a new way
Figure 4: The ‘current of injury’. On wounding, there is an immediate, collapse of the transepithelial potential at the site of the wound (to 0mV) caused by loss of an intact epithelium. In adjacent unwounded areas, the ion transport properties of the epithelium remain intact and Na+ continues to be transported inwards towards the basolateral side of the epithelium (narrow black arrows) and prevented from diffusing to the apical side by the presence of intact gap junctions. However, there is no barrier to prevent ions from nearby unaffected areas to flow laterally towards the centre of the wound (blue arrows). This uncontrolled movement of ions towards the centre of the wound can be measured at around 50mV and is known as the current of injury. Adapted from Tyler et al (2017).2
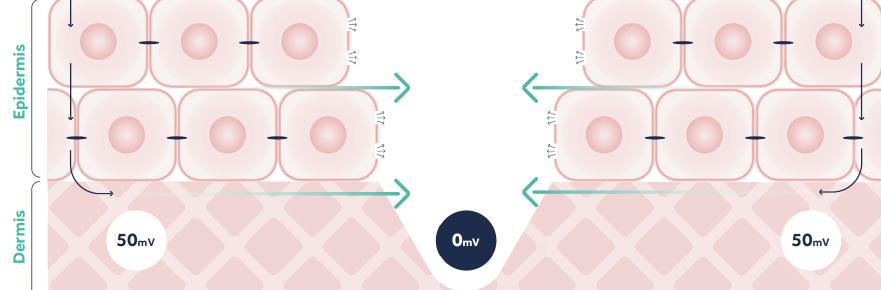
“Given
that electrolytes are soluble and need fluid to diffuse, it is highly likely that moist wound healing is essential for the current of injury to exist.”
of appreciating this basic tenet of wound management.
How Normal Wound Healing Processes Are Reliant on the Current of Injury
Many of the fundamental cell behaviours essential to the healing process are stimulated by bioelectric signalling, as evidenced in numerous scientific studies (Figure 5):1,4,6,8,9
• Proliferation: many cell types need to proliferate for normal wound healing to progress including white blood cells (essential in the inflammatory phase), keratinocytes (for re-epithelialisation), endothelial cells (to make new blood vessels essential for granulation tissue formation) and fibroblasts in the dermis (required to deposit replacement ECM). Bioelectric signalling stimulates proliferation in all of these cell types.1,6
Migration: Bioelectric signalling, through cell directional polarisation, is believed to instruct the newly proliferated cells in
which direction to migrate, so, for example, keratinocytes know to migrate towards, not away from the centre of the wound.1,4,6,9
Cell differentiation This process is essential in later stages of healing, in particular during the late proliferative stage and throughout the remodelling phase. New keratinocytes need to differentiate to form the layers of the epidermis. Fibroblasts differentiate during the phases of healing as their role changes. During the early proliferative phase fibroblasts are stimulated to rapidly produce ECM which is deposited quickly to close the wound. During the remodelling phase fibroblasts become differentiated into myofibroblasts, which remodel this newly deposited ECM into tissue more like normal dermis. Electric fields have also been shown to coordinate these cell maturation events.8
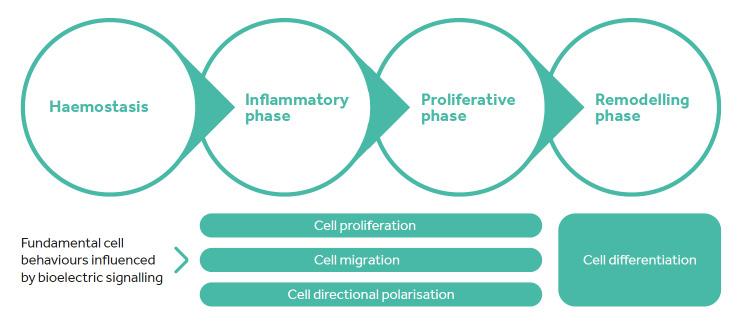
Figure 5: The cellular processes in wound healing that are reliant on the current of injury.1,4,6,8
“Not all wounds heal normally and hard-to-heal wounds which remain unhealed for at least 12-months represents some 30% of all wounds treated annually (UK data).” 11
Several studies have been conducted where researchers have used drugs to enhance or decrease ion transport, and therefore the current of injury, in wound healing models. They have found a clear association between the current of injury and the rate of healing – the lower the current, the slower the rate of healing.10 Given that the movement of charged ions is the way in which bioelectricity is ‘delivered’, these studies also demonstrate the central role of bioelectrical signalling on wound healing events.
There is Some Evidence That the Skin Battery and the Current of Injury Are Reduced in Some People
Not all wounds heal normally and hard-to-heal wounds which remain unhealed for at least 12-months represents some 30% of all wounds treated annually (UK data).11
Some of the main risks of hard-to-heal wounds include older age, diabetes, poor nutrition and ischaemia. It has been suggested that older people and those with diabetes have reduced or compromised transepidermal potential and a lower current of injury.5,12,13 Research in a volunteer study has shown that the current of injury is 48% lower in older people (aged 65+) than in younger people (aged 18-25). There were no differences between male and female. This suggests that the skin battery in older people is less efficient than in younger people.5 Other studies have shown that the skin battery (in intact skin) is lower in people with diabetes than in age matched controls, also suggesting that the skin battery is less powerful in people with diabetes, than in people with no diabetes.14 Studies in the cornea, an epithelium that has much in common with the skin and is often used as a model for cutaneous wound healing, have also shown that diabetes reduces the current of injury.15
It’s thought that having a compromised current of injury makes the wound healing process less
efficient – the conductor of the orchestra is not doing the job properly - and this is likely to be one of the reasons why hard-to-heal wounds are more common in these groups of people. More research is needed to fully understand how these factors interplay but it is possible that a compromised current of injury could reasonably be considered a barrier to healing.
In People With Compromised Skin Battery and Compromised Current of Injury, Application of EST at Physiologically Relevant Levels May Be Beneficial
For those people with a hard-to-heal wound, who may have a compromised current of injury, there is a promising treatment to promote healing. Electrical stimulation therapy (EST) is a highly evidenced treatment type in the field of hard-to-heal wounds with numerous meta-analyses of randomised controlled trials (of which 3 are described below).16-18 The rationale for applying EST is that by replacing the lost endogenous current it can ‘normalise’ the healing process. Many experts suggest that microcurrent EST may resemble a natural electric field or electric current, enhancing the patient’s own endogenous wound healing processes.2,19
One key meta-analysis focussed on hard-to-heal wounds treated with microcurrent devices. This meta-analysis found that wounds treated with microcurrent devices alongside standard care, reduced significantly more in size and healed significantly faster.17 A separate meta-analysis published by the Cochrane group, but focused on pressure ulcers, showed that compared with standard care alone, wounds treated with electrical stimulation wound therapy are almost twice as likely to heal and have a 4.6% increase per week in the rate of wound healing.20
Aside from stimulating healing, microcurrent electrical stimulation wound therapy has also been shown to significantly reduce wound pain,
starting after only a few days of treatment.21 We know that wound pain is often linked with inflammation, so it may be that the effect of microcurrent EST on pain is related to its ability to dampen down macrophage-mediated inflammatory processes.22
In the past, EST devices designed for use in wound management were expensive, very large and awkward to use. Also, most of these devices weren’t able to be used along-side standard care and this made it difficult to incorporate this potentially beneficial technology into everyday wound care routines. However, a new and innovative device, Accel-Heal Solo, is now available to enable use of this technology in frontline practice.
Conclusion
The burden of hard-to-heal wounds is enormous and is growing,11 despite the efforts of dedicated clinicians and scientists alike. The current approaches aren’t working well enough, and we need to do something different. Over the past several decades, much of the research and innovation in wound management has focussed on understanding and modulating the biochemical mediators of the wound healing process, the growth factors and cytokines, without much success.
However, running in parallel, a compelling body of clinical evidence supporting the use of exogenous EST to promote healing in hard-toheal wounds has been steadily growing. What scientists now know about the fundamental role of bioelectricity in coordinating the wound healing process, fits perfectly with this body of evidence and underpins the clinical benefit of applying EST to hard-to-heal wounds. Although in the past these EST devices were difficult to adopt in frontline clinical practice, that is no longer the case and innovative new devices that deliver microcurrent EST are now available that are easily incorporated into existing wound care practice and routines. These microcurrent devices are subsensory and inherently safe, and together with the substantial underlying science and evidence base, this should provide clinicians with the confidence to use in their everyday practice.
Of course, what every healthcare professional wants is to help their patients to achieve respite and to recover from their painful, hardto-heal wounds. We believe that microcurrent EST may help to achieve this goal. In the words of one patient with a painful VLU of 5 months duration, after starting treatment with microcurrent EST* his “leg started to heal and [he] started to get [his] life back”.23 And that is what we want for all our patients!
*Accel-Heal, Accel-Heal Technologies Limited, Hever, Kent, UK.
References
1. Nuccitelli, R. A role for endogenous electric fields in wound healing. Curr Top Dev Biol 58, 1-26 (2003). https://doi.org:10.1016/s0070-2153(03)58001-2
2. Tyler, S. E. B. Nature’s Electric Potential: A Systematic Review of the Role of Bioelectricity in Wound Healing and Regenerative Processes in Animals, Humans, and Plants. Front Physiol 8, 627 (2017). https://doi.org:10.3389/fphys.2017.00627
3. Chifflet, S. & Hernández, J. A. The plasma membrane potential and the organization of the actin cytoskeleton of epithelial cells. Int J Cell Biol 2012, 121424 (2012). https://doi. org:10.1155/2012/121424
4. Martin-Granados, C. & McCaig, C. D. Harnessing the Electric Spark of Life to Cure Skin Wounds. Adv Wound Care (New Rochelle) 3, 127-138 (2014). https://doi.org:10.1089/ wound.2013.0451
5. Nuccitelli, R. et al. The electric field near human skin wounds declines with age and provides a noninvasive indicator of wound healing. Wound Repair Regen 19, 645-655 (2011). https://doi.org:10.1111/j.1524-475X.2011.00723.x
6. McCaig, C. D., Rajnicek, A. M., Song, B. & Zhao, M. Controlling cell behavior electrically: current views and future potential. Physiol Rev 85, 943-978 (2005). https://doi.org:10.1152/ physrev.00020.2004
7. Cheng K et al. An occlusve dressing can sustain natural electric potential of wounds. J Invest Dermatol 104, 662 (1995).
8. Yang, J. et al. Bioelectric fields coordinate wound contraction and re-epithelialization process to accelerate wound healing via promoting myofibroblast transformation. Bioelectrochemistry 148, 108247 (2022). https://doi.org:10.1016/j.bioelechem.2022.108247
9. Zhao, M., Rolandi, M. & Isseroff, R. R. Bioelectric Signaling: Role of Bioelectricity in Directional Cell Migration in Wound Healing. Cold Spring Harb Perspect Biol 14 (2022). https:// doi.org:10.1101/cshperspect.a041236
10. Reid, B. & Zhao, M. The Electrical Response to Injury: Molecular Mechanisms and Wound Healing. Adv Wound Care (New Rochelle) 3, 184-201 (2014). https://doi.org:10.1089/ wound.2013.0442
11. Guest, J. F., Fuller, G. W. & Vowden, P. Cohort study evaluating the burden of wounds to the UK’s National Health Service in 2017/2018: update from 2012/2013. BMJ Open 10, e045253 (2020). https://doi.org:10.1136/bmjopen-2020-045253
12. Kloth, L. C. Electrical Stimulation Technologies for Wound Healing. Adv Wound Care (New Rochelle) 3, 81-90 (2014). https://doi.org:10.1089/wound.2013.0459
13. Fraccalvieri, M., Salomone, M., Zingarelli, E. M., Rivarossa, F. & Bruschi, S. Electrical stimulation for difficult wounds: only an alternative procedure? Int Wound J 12, 669-673 (2015). https://doi.org:10.1111/iwj.12194
14. Ionescu-Tîrgovişte, C. et al. Study of the cutaneous electric potentials and the perception threshold to an electric stimulus in diabetic patients with and without clinical neuropathy. Med Interne 23, 213-222 (1985).
15. Shen, Y. et al. Diabetic cornea wounds produce significantly weaker electric signals that may contribute to impaired healing. Sci Rep 6, 26525 (2016). https://doi.org:10.1038/srep26525
16. Rabbani, M., Rahman, E., Powner, M. B. & Triantis, I. F. Making Sense of Electrical Stimulation: A Meta-analysis for Wound Healing. Ann Biomed Eng (2023). https://doi.org:10.1007/ s10439-023-03371-2
17. Avendaño-Coy, J. et al. Electrical microcurrent stimulation therapy for wound healing: A meta-analysis of randomized clinical trials. J Tissue Viability 31, 268-277 (2022). https://doi. org:10.1016/j.jtv.2021.12.002
18. Girgis, B., Carvalho, D. & Duarte, J. A. The effect of high-voltage monophasic pulsed current on diabetic ulcers and their potential pathophysiologic factors: A systematic review and meta-analysis. Wound Repair Regen 31, 171-186 (2023). https://doi.org:10.1111/wrr.13063
19. Balakatounis, K. C. & Angoules, A. G. Low-intensity electrical stimulation in wound healing: review of the efficacy of externally applied currents resembling the current of injury. Eplasty 8, e28 (2008).
20. Arora, M. et al. Electrical stimulation for treating pressure ulcers. Cochrane Database Syst Rev 1, Cd012196 (2020). https://doi.org:10.1002/14651858.CD012196.pub2
21. M. Moon, D. Hazel & Hawes., L. Reduction in wound pain associated with electrical stimulation therapy results in a corresponding decrease in analgesic consumption: an evaluation of patients with painful leg ulcers. Presented at Wounds UK, Harrogate, Nov 2023. (2023).
22. Lallyett, C. et al. Changes in S100 Proteins Identified in Healthy Skin following Electrical Stimulation: Relevance for Wound Healing. Adv Skin Wound Care 31, 322-327 (2018). https:// doi.org:10.1097/01.Asw.0000533722.06780.03
23. N. Turner & Ovens., L. Clinical outcome results and quality of life improvements using electroceutical treatment - Patient perspectives. Poster presented at EWMA 2017. (2017).