December - January 2023
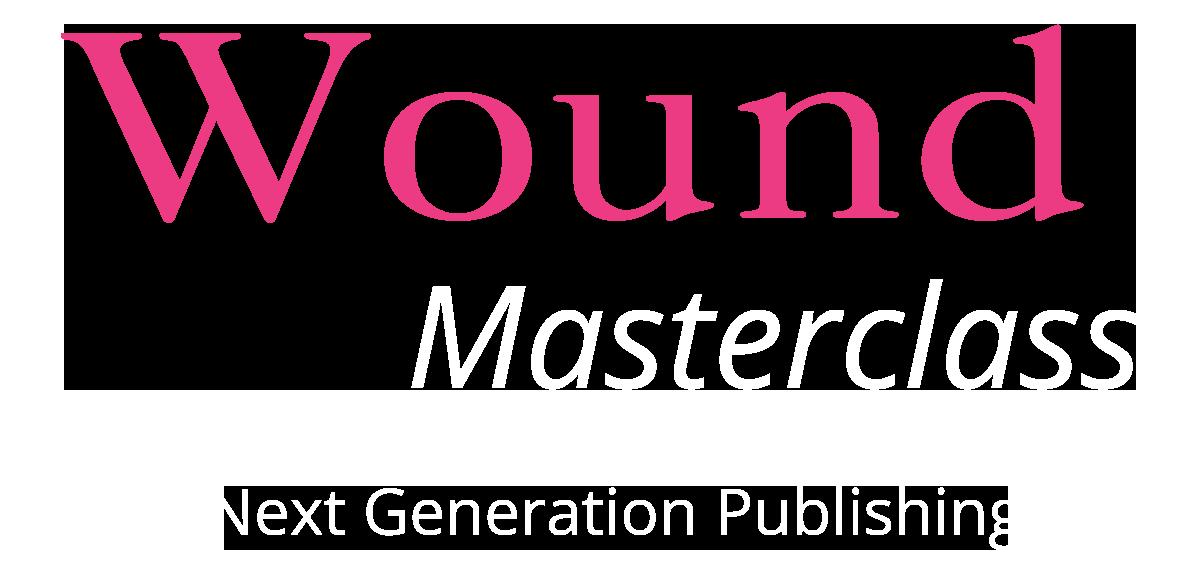
December - January 2023
Editorial Summary
This paper provides an overview of the future of three dimensional printed biofilm for in vitro and in vivo wound infection models. Biofilm is an essential component of understanding the mechanism of stalling of wound healing. Biofilm removal remains a contentious area of wound care. Very few antimicrobial rinses and dressings on the market have been developed to target biofilms as there still remains to be an established ‘ideal’ assay to study mature biofilms in a high throughput screen that is reproducible. We describe a novel 3D printed biofilm model that can be utilized in vitro as well as in vivo
Introduction
Chronic wounds are a significant financial burden ($25B) to our health care system.1-3 Chronic hard-to-heal wounds are also a significant burden to the quality of life (QoL) of the patients. One of the major risk factors associated with lower extremity amputations and patient mortality is infected wounds, that are often stalled in the inflammatory phase of wound healing.
Wound infections are resistant to antibiotics and antimicrobial therapies because the bacteria are deep in the wound bed in a polymicrobial biofilm with a dense layer of polysaccharides, proteins, nucleic acids, and lipids referred to as the exopolymeric substance, or EPS. The biofilm is resistant to mechanical shear, the microbes deep in the biofilm are senescent and are resistant to antibiotics.
Although there are countless antimicrobial wound care rinses and dressings on the market, many have limited efficacy for removing biofilms because these antimicrobials and antibiotics were developed using minimal inhibitory concentration (MIC) assays with planktonic bacteria, which are easier to kill compared to complex biofilms.4-7 In addition, very few of these products have been developed to target biofilms because there hasn’t been an ideal assay to study mature biofilms in a high throughput screen (HTS), that is reproducible and has a high signal to noise ratio.
Boston MA, United States
One of the best characterized ex vivo models to study biofilms was developed in Greg Schultz’s laboratory.8,9 This model system can generate mature biofilms that are more reflective of a hard to kill biofilm in a chronic wound, but the model system requires several replicates due to the high standard deviation. Although recent improvements in the sterilization of the explants with immersion in 70% ethanol followed by 10% bleach reduced this standard deviation to some extent, there is still quite a lot of variability from lot to lot of the porcine skin due to the potential contamination of the explants with B. subtilis spores.
Here we report the development of the first of a kind 3D printed
“To develop an assay for a drug high throughput screen for biofilms, we had to develop methods for measuring the integrity of the biofilms and the ratio of living to dead bacteria within the 3D biofilm.”
collagen impregnated polymicrobial mature biofilms that have the ideal properties of high signal to noise, and low standard deviation that make each replicate reproducible enough for microplate assay (96 and 384 well) formats for drug and antimicrobial assays.
biofilm formation and then rinsed with water prior to storage in a sterile 24 well plate at -80°C for further use.
Materials and Methods
Bacterial Strains and Reagents
Staphylococcus aureus (6538), Pseudomonas aeruginosa GFP (10145), and unlabeled P. aeruginosa (15442) were purchased from ATCC. Bacteria were grown overnight in tryptic soy broth (TSB) at 37°C prior to seeding into the collagen 3D printed biofilms. Shaved pig skin was obtained from Sierra for Medical Science.
Pig explants were sterilized as described by Yang et al. (2017), with the following modifications: The shaved porcine skin was sterilized with a combination of detergent (0.1% MACAT LHS) and a commercially available antimicrobial rinse (undiluted Oxivir 1) to reduce the starting bacterial bioburden. Following rinsing with water and air drying in the biosafety cabinet, the porcine explants were isolated with an 8 or 12 mm sterile biopsy punch. We then treated the biopsies with a 1M solution of CaCl2 for 20 minutes to mimic a calcium burn to improve
We used a Cellink 3D BioX Printer to print methylacrylated collagen (LifeLink 200) loaded with either GFP-Pseudomonas aeruginosa or Staphylococcus aureus and Pseudomonas aeruginosa and then photo-activated the collagen with lithium phenyl-2,4,6-trimethylbenzoyl phosphinate (LAP) dye and polymerized with a near-UV light source (365 nm). Briefly, we set up the Cellink 3D Bio X printer to print 12, 5 x 1 mm biofilm collagen disks at a pressure of 12 kPa, at a speed of 4 mm/sec, and an infiltration rate of 75%. An amber syringe containing a suspension of 2 ml of Lifelink 200 Collagen and a 250 μl of an overnight culture of each microbe was loaded into the printer in a dark room to avoid premature curing of the collagen with ambient light. After printing all the layers of the collagen-microbial disk, the UV light was utilized to cure the 3D print.
Following printing, the biofilm disks were exposed to natural light for 30 min to allow for complete curing and then incubated overnight at 37°C. The disks were then incubated for another 24 hours at 37°C in a proprietary biofilm binding buffer and were either used immediately or stored in tryptic soy broth with 2% sucrose for lyophilization followed by ambient storage or stored at -80°C for future use.
To develop an assay for a drug high throughput screen for biofilms, we had to develop methods for measuring the integrity of the biofilms and the ratio of living to dead bacteria within the 3D biofilm. Biofilms were imaged using a Keyence BZX-810 Fluorescence Microscope with DAPI, Texas Red, and GFP filter sets and a CFI
PLAN FLUOR DL 4X or 20x Nikon Lens. Biofilm integrity assays were performed by staining the poly-N-acetyl-glucosamine, (PNAG) with Alexa Fluor 488 labeled wheat germ agglutinin as describe by Skogman et al. (2006).10 For our Live/Dead Analysis of the bacteria in the biofilms, we utilized LIVE/ DEAD™ BacLight™ Bacterial Viability Kit which utilizes SYTO 9 to stain live cells with green fluorescence and propidium iodide (PI) to stain dead cells red.
We used a handheld 365nm UV light source and a digital phone camera to show the integrity of 3D printed GFP P. aeruginosa biofilms as shown in Figure 1.
Comparison of 3D Printed Biofilms vs. Pig Explant Biofilms
One of the inherent issues with the classical
and well recognized pig explant biofilms developed in Greg Schutz’s Lab is that each replicate has a high standard deviation requiring many replicates to achieve statistical significance. The second major inherent issue with the pig explant model is that it is often contaminated with Bacillus subtilis spores which makes each lot of porcine material less reproducible. We used P. aeruginosa labeled with GFP to compare the intensity and the standard deviation of biofilms formed in pig explants or the 3D printed collagen biofilms as shown in Figure 2.
Figure 4 illustrates the Live/Dead staining of the 3D printed biofilms at 20X magnification. Most of the bacteria in the biofilm stain is Green in the PBS control with a low intensity of dead bacteria in the Red image.
Figure 3 shows the diffuse PNAG fluorescence of the 3D printed biofilm at 20x magnification after incubation for 48 hours to form mature biofilms of S. aureus and P. aeruginosa and then incubated overnight in PBS.
To validate and test the efficacy of the 3D printed biofilm model, we treated the biofilms with chlorohexidine (CHG) or an essential oil (lemongrass (LG) and cinnamon (CN)) at 4% overnight at 37°C. Figure 5 shows that CHG had a profound reduction in the PNAG staining suggesting that it can affect the integrity of the biofilms. In contrast the PNAG staining was less affected by LG and CN.
Following imaging of the biofilms we were able to sonicate three replicates of each treatment group and determine the CFU level of the bacteria in the polymicrobial biofilms (P. aeruginosa and S. aureus). Figure 7 shows that the treatment groups of CHG, LG and CN reduced the bacterial bioburden of the 3D printed biofilms but did not kill all the bacteria suggesting that 3D printed biofilms are hard to kill and robust enough for a high throughput
screen.
In this paper, we describe the development of a standard HTS assay for mature polymicrobial biofilms using a state-of-the-art 3D Bioprinter to make consistent and reproducible replicates. The 3D printed mature polymicrobial biofilm have very high signal with limited standard deviation (<2%) among individual replicates. We are currently optimizing the lyophilization conditions so that plates can be shipped to collaborators for wound biofilm studies in vitro as well as in vivo
Our findings indicate that the 3D printed biofilms are robust and more reproducible than the pig explant model system described previously by Yang et al.1,2 The 3D printed biofilms can be stained with fluorescent probes to measure both the integrity of the biofilm
Disclosures
We have no conflicts of interest to disclose.
1. Sen CK, Roy S, Mathew-Steiner SS, Gordillo GM. Biofilm Management in Wound Care. Plast Reconstr Surg. 2021 Aug 1;148(2):275e-288e. doi: 10.1097/PRS.0000000000008142. PMID: 34398099; PMCID: PMC8439557.
2. Olsson M, Järbrink K, Divakar U, Bajpai R, Upton Z, Schmidtchen A, Car J. The humanistic and economic burden of chronic wounds: A systematic review. Wound Repair Regen. 2019 Jan;27(1):114-125. doi: 10.1111/wrr.12683. Epub 2018 Dec 2. PMID: 30362646.
3. Sen CK. Human Wounds and Its Burden: An Updated Compendium of Estimates. Adv Wound Care (New Rochelle). 2019 Feb 1;8(2):39-48. doi: 10.1089/wound.2019.0946. Epub 2019 Feb 13. PMID: 30809421; PMCID: PMC6389759.
4. Percival SL. Restoring balance: biofilms and wound dressings. J Wound Care. 2018 Feb 2;27(2):102-113. doi: 10.12968/jowc.2018.27.2.102. PMID: 29424642.
5. Daeschlein G. Antimicrobial and antiseptic strategies in wound management. Int Wound J. 2013 Dec;10 Suppl 1(Suppl 1):9-14. doi: 10.1111/iwj.12175. PMID: 24251838; PMCID: PMC7950476.
6. Clinton A, Carter T. Chronic Wound Biofilms: Pathogenesis and Potential Therapies. Lab
Med. 2015 Fall;46(4):277-84. doi: 10.1309/LMBNSWKUI4JPN7SO. PMID: 26489671.
7. Mancl KA, Kirsner RS, Ajdic D. Wound biofilms: lessons learned from oral biofilms. Wound Repair Regen. 2013 May-Jun;21(3):352-62. doi: 10.1111/wrr.12034. Epub 2013 Apr 1. PMID: 23551419; PMCID: PMC3648594.
8. Yang Q, Phillips PL, Sampson EM, Progulske-Fox A, Jin S, Antonelli P, Schultz GS. Development of a novel ex vivo porcine skin explant model for the assessment of mature bacterial biofilms. Wound Repair Regen. 2013 Sep-Oct;21(5):704-14.
(PNAG) or the live/dead staining which correlates well with the fold log reduction of the CFU. We are currently validating this model system in a Diabetic mouse model to show that 3D printed biofilms have utility both in vitro as well as in vivo