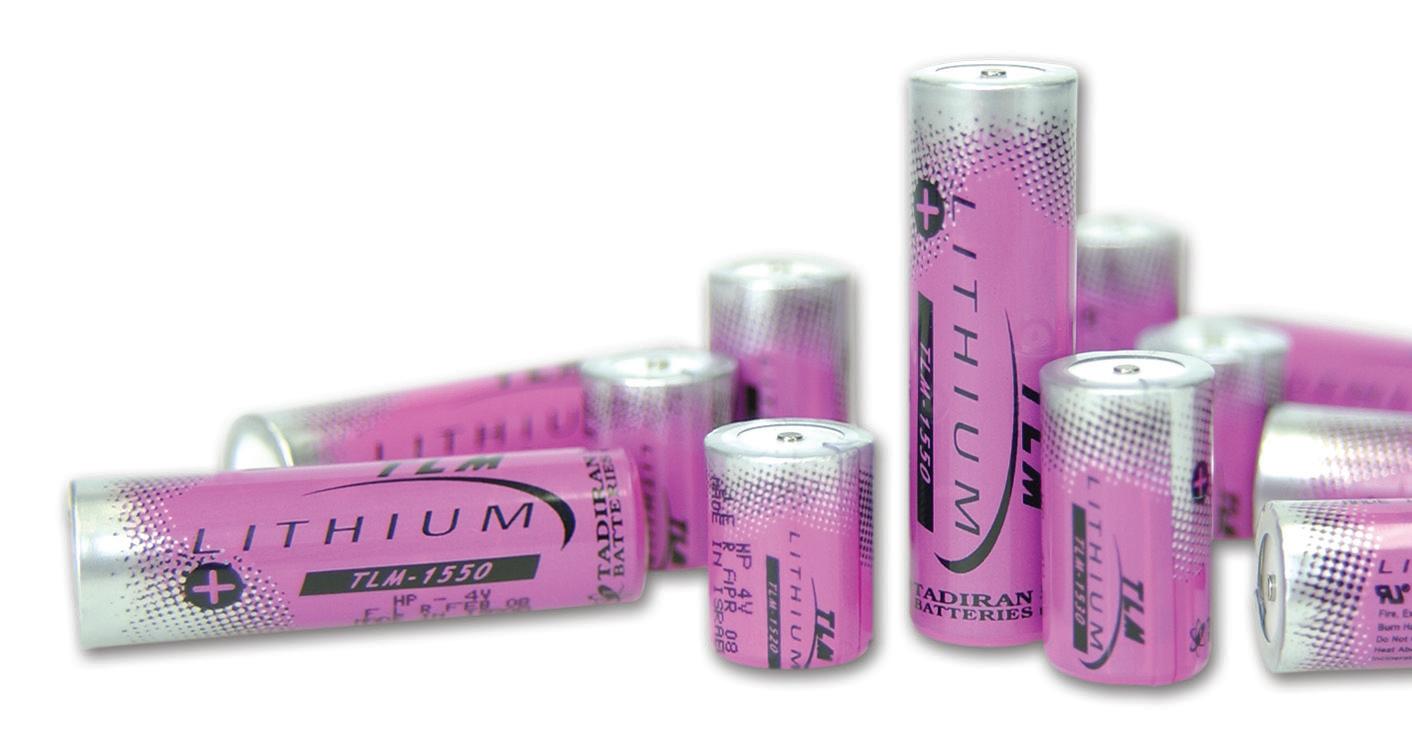
7 minute read
The 40-year battery pack
Sol Jacobs • Tadiran Batteries
Not all primary batteries offer the low annual self-discharge rate needed for the lengthy lifespan of remote wireless devices consuming microamp-level currents.

The vast majority of remote wireless devices are poweredby primary (non-rechargeable) batteries that range from consumergrade to industrial grade. Consumer-grade alkaline batteries deliverhigher discharge rates of energy, resulting in a short operatinglife. Also available are consumer-grade lithium batteries thatdeliver medium-to-high discharge rates of energy with short-tomediumoperating life, including iron disulfate (LiFeS 2), and lithiummanganese dioxide (LiMNO 2) chemistries.
Meanwhile, a growing number of industrial wireless sensors and devices are being designed to operate for decades without having to replace the battery. They typically require only a low rate of energy discharge and consume microamps of average current. Many of these devices sit in extreme environments and hard-to-access locations, often connected to the Industrial Internet of Things (IIoT).
Lithium-based batteries have high intrinsic negative potential, exceeding that of all other metals, with an operating current voltage (OCV) ranging from 2.7 to 3.6 V. Lithium batteries are also non-aqueous, with the absence of water enabling them to endure extreme temperatures without freezing.
Among all commercially available available chemistries, bobbintype lithium thionyl chloride (LiSOCl 2) cells are preferred for use in remote locations and extreme environments. Bobbin-type LiSOCl 2 batteries feature the highest capacity and highest energy density of any lithium chemistry, along with an extremely low annual self-discharge rate (less than 1% per year), enabling certain devices to operate for up to 40 years. Bobbin-type LiSOCl 2 chemistry also features the widest possible temperature range (-80 to 125°C), along with a glass-tometal hermetic seal that resists battery leakage. Common applications include AMR/AMI metering, M2M, SCADA, tank-level monitoring, asset tracking, and environmental sensors, to name a few.
All batteries experience some amount of self-discharge, where cell capacity gets depleted even when the battery is not connected to an external load. The ability to control passivation (basically, reduction of chemical reactivity) helps to lower selfdischarge: a quality unique to bobbin-type LiSOCl 2 batteries.
Passivation arises when a thin film of lithium chloride (LiCl) forms on the surface of the lithium anode, thus impeding the chemical reactions that result in battery self-discharge. When a load is placed on the cell, the passivation layer causes high initial resistance, resulting in a temporary drop in cell voltage until the discharge reaction slowly removes the passivation layer. The process repeats every time the load is removed.
Several variables can influence the passivation effect, including: the current capacity of the cell, the length of storage, storage temperature, discharge temperature, and prior discharge conditions, as partially discharging a cell and then removing the load can boost the amount of passivation relative to when the cell was new. Passivation can reduce a battery’s self-discharge rate, but too much of it can block energy flow.
Different bobbin-type LiSOCl 2 batteries can be designed with varying amounts of passivation and self-discharge. These cells can be designed with medium energy flow rates and higher self-discharge (lifespan of 10 years) or with lower flow rates and lower self-discharge to run a marathon (lifespan of up to 40 years).
Battery self-discharge rate is also affected by the quality of the raw materials and the method by which the battery is manufactured. For example, a lower-grade bobbin-type LiSOCl 2 battery can lose up to 3% of its normal capacity annually due to self-discharge, thus exhausting 30% of its initial capacity every 10 years, making 40-year battery life impossible. By contrast, the highest quality bobbin-type LiSOCl2 battery can feature a self-discharge rate of just 0.7% per year, thus retaining 93% of its original capacity after 10 years. A 40-year operating life is feasible.
A 40-year operating life is analogous to running a marathon. Distance is equivalent to the battery/device operating life. The energy necessary to run up an incline is equivalent to the rate of battery selfdischarge. The higher the self-discharge rate, the steeper the incline. A course with a lot of hills can have runners walking by the end of the race, and higher battery selfdischarge reduces the availability of useful power for device operation and thus reduces the operating life. Additionally, hurdles can be thought of as requiring energy pulses. The higher the hurdle (obstacle) the greater must be the pulse ability of the battery.
Certain applications, such as medical power tools and actuators, require a high rate of energy drain as well as high pulses. These applications draw average current measurable in amps, making them well suited for lithium metal oxide batteries. Flashlights, toys, and other consumer applications that require rates of discharge measurable in milliamps to amps may be best suited for alkaline, LiFeS 2 and LiMNO 2 batteries that can deliver medium pulses.
Many remote wireless sensors and other ultra-long-life, low-drain applications with average current measurable in microamps require the use of standard bobbin-type LiSOCl 2 batteries that can run marathons thanks to their extremely low self-discharge rates. But their low-rate design prevents these cells from delivering high pulses without modification. However, standard bobbin-type LiSOCl 2 batteries can be easily modified by adding a patented hybrid layer capacitor (HLC). The standard bobbin-type LiSOCl 2 cell delivers the low daily background current for a 40-year marathon while the HLC delivers periodic high pulses to power two-way wireless communications (steeple jumping). The patented HLC also features a special end-of-life voltage plateau that can be interpreted to deliver low-battery status alerts.
Consumer applications often use supercapacitors that deliver high pulses electrostatically rather than chemically. Supercapacitors are only selectively used in industrial applications due to performance limitations such as short-duration power, linear discharge qualities that prevent use of all the available energy, low capacity, low energy density, and high annual self-discharge rates (up to 60% per year). Supercapacitors linked in series also require the use of cell-balancing circuits, which adds expense and bulk and boosts their self-discharge rate.
SHORT-TERM TESTS DON’T SIMULATE LONG-TERM PERFORMANCE
Long-term battery performance cannot be easily duplicated using short-term test procedures, so specialized test methods must be employed. Here are some proven test methods:
Long-term laboratory testing – The ideal way to monitor battery self-discharge is to continually test batteries over time under various conditions, covering almost every possible scenario. Over time, the accumulated data points can be used to accurately predict performance based on cell size, temperature, load size, etc.
Accelerated testing - The Arrhenius equation--which is based on a two-fold increase of reaction rate for every 10°C rise in temperature--is often used to simulate long-term battery operation. Arrhenius tests are run at 72°C, equivalent to about 32 times the theoretical lifetime of battery at 22°C. However, short-term tests using the Arrhenius method tend to show inaccurate results.
Calorimeter testing. An extremely accurate test method is to measure the amount of actual heat energy lost using a state-of-the-art microcalorimeter, which can detect energy dissipation as low as 0.1 W.
Heat energy is generated three ways: entropy change, often referred to as reversible heat; cell over-protection, often referred to as irreversible heat; and chemical reactions, including self-discharge reactions that affect cell capacity, and side reactions that do not affect cell capacity.
Calorimeter testing can be especially useful for measuring losses in battery capacity that arise during long-term storage or from operation (including self-discharge), measurable using thermodynamic equations and cell voltage considerations. To ensure accuracy, the batteries must be stabilized for one year prior to testing, as first-year self-discharge tends to be higher than subsequent years.
Lithium titration. Lithium titration can be used to measure available cell capacity. The battery is cut open, and then titration is used to dissolve the remaining lithium to determine its volume. The higher the self-discharge rate, the less lithium will be found in in the cell. Lithium titration can be especially useful in special circumstances as when measuring the effects of extreme temperatures and/or prolonged high pulses.
Field results – Batteries working in the field offer the best from of validation for test results. Tadiran has its customers provide randomly sampled batteries taken from long-term deployments to verify the real-life impact of long-term exposure to extreme temperatures and to demonstrate how such conditions accelerate battery self-discharge. For example, batteries deployed in pioneering AMR/AMI devices from the 1980s exhibited significant amounts of unused capacity after 28 years in the field.
Another useful indicator of longterm battery performance is the number of Failures In Time (FITs), measurable in billions of device operating hours for devices in the field. Tadiran batteries have FIT rates ranging between 5 and 20 batteries per billion, extremely low compared to the industry average.
Every application has unique power requirements, so it is important to determine whether you need a sprinter (high discharge potential); a medium distance runner (moderate to high discharge rate with fairly low selfdischarge); or a marathoner (running with very low discharge for up to 40 years).
REFERENCES: Tadiran Batteries, www.tadiranbat.com