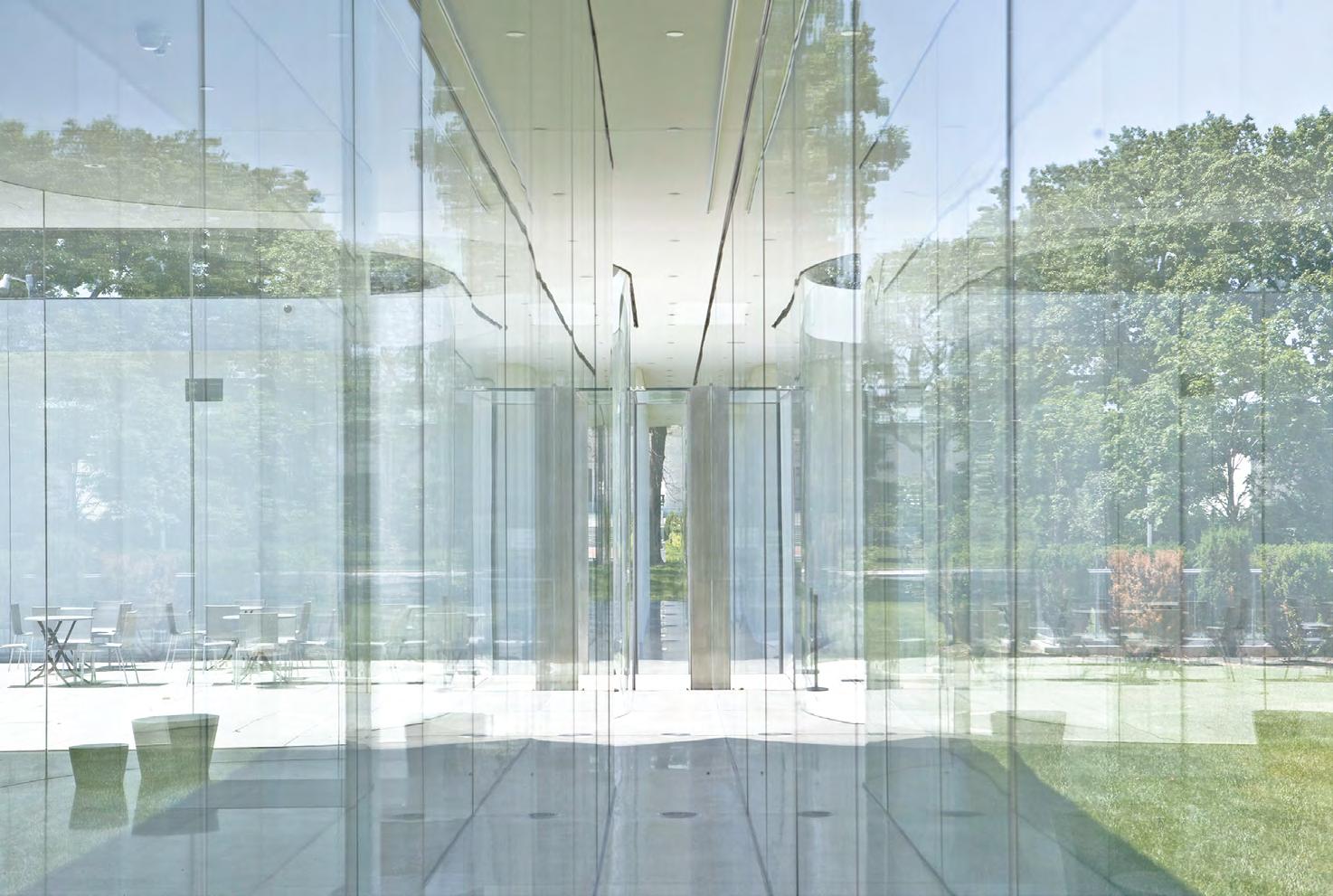
17 minute read
Air, light, and liquid in motion: blurred transparency of the Glass pavilion in Toledo
AKI ISHIDAA
In architecture, when glass is experienced in motion under changing light, the perception of it is not simply as transparent but is a specific kind of transparency that can be called blurred. As new technologies in glassmaking and engineering expand the range of effects and performance of clear glass, its transparency becomes increasingly multivalent and complex, making nuanced differences difficult to perceive. Toledo’s Glass Pavilion exemplifies these blurred transparencies, which result from several mutually dependent factors: innovative approaches to mechanical and structural engineering, balancing of light coming from multiple sources and directions, and an unusual spatial organization of glass-enclosed air plenums and rooms to create radical programmatic adjacencies and complex array of reflections.
A Virginia Tech School of Architecture + Design, USA.
KEYWORDS: TRANSPARENCY, GLASS ARCHITECTURE, SANAA
Introduction
The Glass Pavilion (2006) at the Toledo Museum of Art, designed by Kazuyo Sejima and Ryue Nishizawa of SANAA, presents a novel kind of transparency that results from layering of curved glass with volumes of air. Built with clear glass as the primary exterior walls and interior partitions, the Pavilion is simultaneously opaque and transparent in its construction and spatial organization. It is opaque in its concealment of steel structure which supports the transparent ribbons of massive glass. At the same time, it is remarkably transparent in exposing elements of architecture that are typically hidden from view, notably the glass plenum through which air circulates. Historically, glass and air have been intimately linked since the pre-industrial production of glass, in which exhalation of air from human lungs transformed molten glass into cylinders, which were then cut and flattened into sheets. Like the visual effects seen on blown glass, Toledo Glass Pavilion’s transparency is soft, watery, and intimately airy–in contrast to the hard, cold, machine-made impression often associated with glass. This softness is further underscored by its play of layered reflections seen on the surfaces of curved glass (Figure 1).
The Glass Pavilion is a carefully calibrated instrument of light, shadow, and reflection. Although clear glass is the predominant material, the building differs from the see-through transparency of modernism described as shadow-less glass box in Ludwig Hilberseimer’s 1929 essay Glasarchitektur. He writes that the Crystal Palace built in 1850 to 1851 “obliterated the old opposition of light and shadow, which had formed the properties of past architecture. It made a space of evenly distributed brightness; it created a room of shadowless light” (Hilberseimer, 1995, pp. 9-10). The Pavilion is also distinguished from the diffused, shadowy luminosity of translucent glass buildings that became widespread in the late 1990s following PostModernism. In contrast, the use of curved, massive monolithic glass in the Pavilion leads to an intensified transparency in which a clear material gives mutable readings that range from apparent depth to near-opacity.
In architecture, when glass is experienced in motion under changing light, the perception of it is not simply as transparent but is a specific kind of transparency that can be called blurred. As new technologies in glassmaking and engineering expand the range of effects and performance of clear glass, its transparency becomes increasingly multivalent and complex, making nuanced differences difficult to perceive. Toledo’s Glass Pavilion exemplifies these blurred transparencies, which result from several mutually dependent factors: innovative approaches to mechanical and structural engineering, balancing of light coming from multiple sources and directions, and an unusual spatial organization of glass-enclosed air plenums and rooms to create radical programmatic adjacencies and complex array of reflections.
Fig. 01 Layered reflections seen through the double-glazed buffer zone for air. The Glass Pavilion’s transparency is soft, watery, and intimately airy. I. Baan, 2007.
Glass bubbles drawn with a brush
Each individual room in the Pavilion is enclosed by a curving wall of lowiron, monolithic laminated glass panels three-quarters of an inch thick. “Our concept is to wrap each program [element] with a curved line drawn by a single stroke of [the] brush,” writes SANAA’s principal Ryue Nishizawa (Sejima et al., 2011, p. 23). The program elements include exhibition rooms for glass crafts, multi-purpose room, open-air courtyards, storage, and hot glass demonstration shops. In order to keep the main floor free of visual obstructions as much as possible, the mechanical rooms are located in the basement, and the cooling towers and pumps are off-site in a separate building (Lane, 2006). On the main floor, enclosing all bubble-like rooms is an exterior envelope of the same glass but at a greater thickness of one inch. Interior glass rooms float within the exterior envelope’s continuous glass skin, separated from each other by a thirty-inch air cavity (Figures 2). This zone of air mitigates temperature differences between the outdoor and the conditioned indoor spaces, as well as between the galleries and the shops with glass kilns. Normally, this temperature control is served by the air cavity found in standard double-glass facades, or the mechanical shafts and spaces concealed in opaque stud walls. This transparent cavity flows like
water through the building to make a contiguous air cushion, expanding and contracting as it turns rounded corners while maintaining a minimum egress clearance for people to pass through for maintenance.
Each of the 360 glass panels, measuring thirteen and one-half feet tall and weighing 1,300 to 1,500 pounds (Levine, 2012), were manufactured in Pilkington’s factory in Germany. They were shipped to Shenzhen, China where they were slumped (a process of shaping glass panels over mold at high temperature) and laminated with polyvinyl butyral (PVB) interlayer to fabricate the thick monolithic panels (Ra, 2017). Visible materials and structures other than glass are made inconspicuous. Slender steel columns of three and one-half inch to four and one-quarter inch diameter support spans as long as sixty feet and hold up a shallow roof of two-feet deep (Nordenson and Schneider, 2016). The bottom of the glass wall panels rests on recessed tracks in the concrete floor and are held in place laterally by another track concealed in the ceiling (Ra, 2017). The roof is designed to float over the glass and never bear on the glass panels (Oki, 2017).
At vertical joints, translucent structural silicone holds two sheets of glass together, which eliminates vertical mullions or channels. Since the glass panels are monolithic and not insulated glass units, the glass walls appear continuous and ribbon-like (Ra, 2017). The curved glass panels appear neither completely transparent nor opaque. The viewer sees the interior and exterior simultaneously, layered with reflections of the nearby trees, cars, and houses, overlapping them with the objects and world beyond and before the glass walls.
Whereas museums normally depend on opaque walls to exhibit artifacts, SANAA fittingly maximized transparency in a museum which exhibits glass objects and processes. The all-glass foyer physically separates, yet visually connects, the hot lab from the gallery; without this transparent thermal buffer, these two spaces with highly varied needs for temperature, moisture, and acoustic normally could not be next to each other unless separated by insulated opaque walls. From the glass-wrapped foyer between gallery and hot lab, a visitor can view glass sculptures and vessels, against, in the background, the molten glass shaped into solid objects in the adjacent hot lab (Figure 3). Multiple states of glass appear simultaneously superimposed upon each other, through a plan that situates thick monolithic glass in a radical configuration. The superimposition is also temporal. Glass objects spanning a period of 4,000 year, from a Mesopotamian glass bowl to Libby Glass Company’s punch bowls are viewed in a fluid transition from one enclosure to another.
Nishizawa’s remark that they enclosed each program with a single brush stroke suggests a space different from one drawn with a pen. Like western watercolor paintings, Japanese calligraphy and brush painting–sumi-e–achieves tonality by varying ink density with water. Less ink and more water
are used for faint or distant scenes, and the strokes are layered to depict depth. Moreover, unlike a pen with uniform line width, a brush stroke varies in width and transparency depending on the pressure place on the brush and the angle at which the brush glides across the paper. Like a brush stroke, the air cavity that surrounds each bubble-like enclosure in Toledo is contiguous and varied in width. Similar to multiple superimposed watery brush strokes with a faint hint of ink, transparent glass sheets suggest depth and even opacity when seen through multiple layers, creating a mutable palimpsest of images.
Engineering glass transparency
The Glass Pavilion took an exceptionally integrative approach to climate control in a nearly all-glass building, a type that is notoriously difficult to cool and heat efficiently. Questions of heating and cooling glass building challenged modern architects from the early days of glass buildings. Bruno Taut’s Glass Pavilion in Cologne and Paul Scheerbart’s book Glassarchitektur, both dated 1914, foreshadowed a future in glass buildings with double-glazed walls, including their capacity to solve glass’s critical weakness, thermal transfer. Like Taut, Scheerbart carried the conviction that technology was “the formative agent of the current culture” (Ersoy, 2015, p. 156). Tuat and Scheerbart similarly saw the need to experiment with glass architecture enabled by iron structure. In Scheerbart’s prophetic 1914 book Glasarchitektur, he contemplated double glass, looking far ahead of his time to speculate what glass architecture could be. He wrote, “As air is one of the worst conductors of heat, the double glass wall is an essential condition for all glass architecture. The walls can be a meter apart–or have an even greater space between” (Scheerbart, 1972, p. 42). Taut and Scheerbart’s imagination of two sheets of glass spaced about a meter apart bears a notable resemblance to the thirty-inch air space at the Toledo Glass Pavilion.
SANAA worked with Transsolar, a climate engineering firm founded in Stuttgart, Germany. Transsolar’s climate control strategies contributed a kind of material transparency unique to this program and geographic location. The Pavilion consists of three energy zones (Figure 4): the perimeter buffer zone, the hot glass demonstration areas, and the galleries with individual climate control requirements (Moe, 2008). The perimeter zone mediates spaces with variable climatic needs. Hydronic radiant heating and cooling in the floor and ceiling tempers the air in the first zone. This moderates the difference in temperatures between the indoor and outdoor glass surfaces, which in turn prevents condensation on the glass in both hot and cold weathers. Furthermore, the hot air generated in the hot glass demonstration spaces are recovered and re-directed through a hydronic floor slab in
order to heat other spaces in the building. The building redirects cool air collected from the galleries to cool the hot glass demonstration zone (Moe, 2008). Na Min Mike Ra of Front, the glass consultants on this building, notes recirculating the heat from the hot shop furnace, which is kept on 24/7, made an all-glass building possible in the cold climate of Toledo. Otherwise, the heating cost would have been prohibitively expensive (Ra, 2017).
SANAA’s design process treats engineering with equal importance as the other aspects of design. The design team regards technical matters, including the details that guide the construction process and placement of columns relative to the glass, as an integral aspect of visual appearance. The girders and joints, ductwork, and plumbing pipes reside within the twofoot depth of the roof. Vertical runs for the pipes and ducts are housed in the full-height opaque walls. As with many of SANAA’s buildings, such as the Naoshima Ferry Terminal (2006), with ultra-slender columns, these opaque walls must also accommodate lateral bracing. The slender columns are mostly solid steel, but some are hollow to run infrastructure and rainwater between the roof and floor (Nordenson and Schneider, 2016).
SANAA’s buildings are a feat of mechanical and structural engineering. They appear deceptively simple and a-tectonic by comparison to the structural expressions of Peter Rice, Jörg Schlaich, or Norman Foster. In SANAA’s work, the visible structure is minimized so the atmospheric effects become foregrounded. In their essay “Ocean of Air”, Cristina Diaz Moreno and Efren Garcia Grinda describe this phenomenon as “intensified perception in which the process of minimizing the object’s main features (form, materials, size) takes priority over those usually regarded as secondary” (Diaz Moreno and Garcia Grinda, 2004, p. 37).
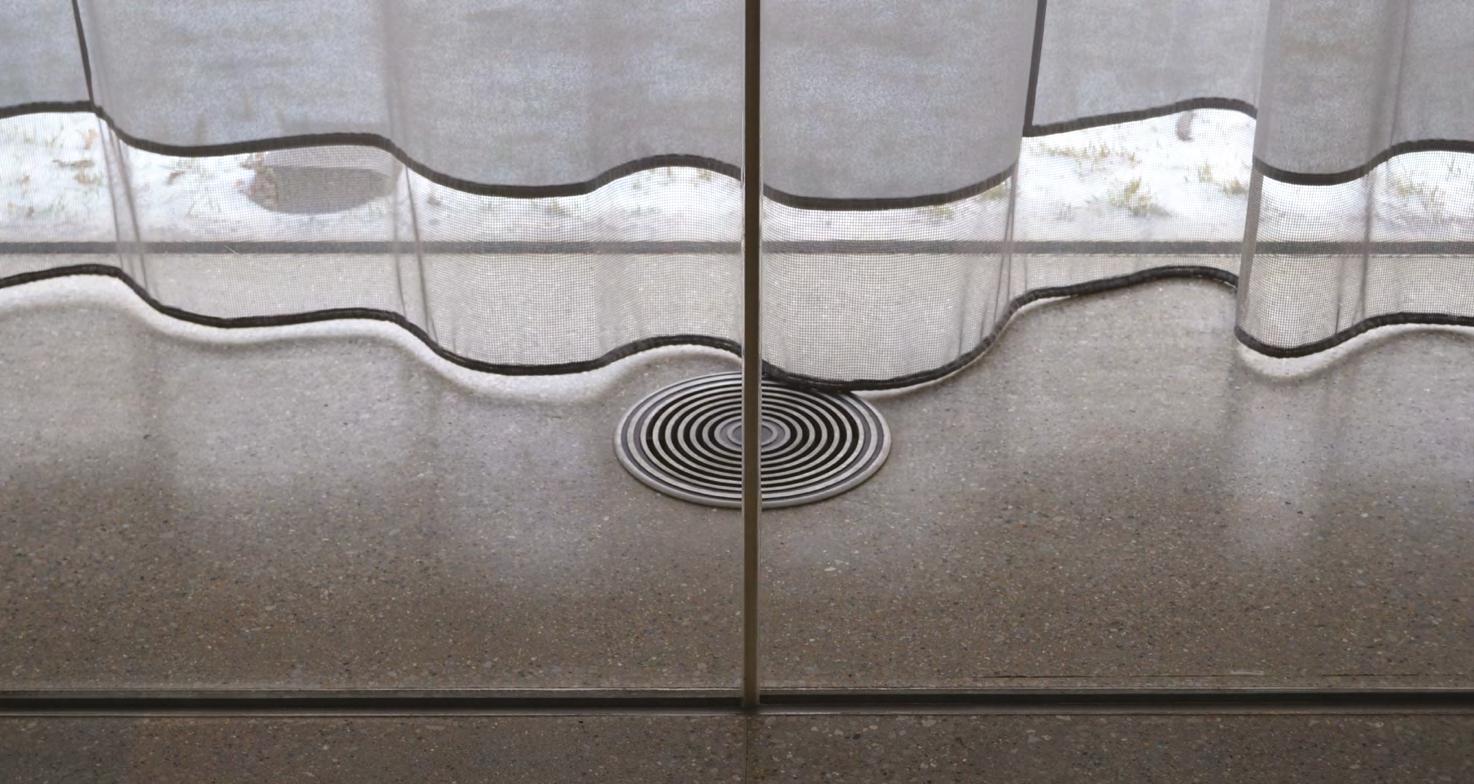
Fig. 02 Air circulates through the thirty-inch air cavity along the building’s perimeter. N. Coates, 2013.
Glass details and material finishes
At Toledo, SANAA took great care to make the glass construction details unobtrusive while amplifying its spatial effects. This was done through treatment of seams, specification of glass, edge finish, and strategic placement of joints relative to the columns. Exposed glass edges in contemporary buildings are often highly polished to make them prismatic, then arissed (chamfered by sixteenth of an inch). At Toledo, the edges at silicone joints were ground smooth but left unpolished (Ra, 2017) to draw the eye not to the material edges but to the effects on the expansive surfaces. The architects specified low-iron glass was to minimize the green tint of standard glass, and carefully tuned the reflective finishes (Oki, 2017). Even though the architects were interested in reflecting the site context on the glass walls, the effects differ from those of Two-Way Mirror by Dan Graham, for example. Oki recalled they considered coating the glass to reduce reflections but not to increase them. Furthermore, reflective metal finishes are minimized in the building. The slender steel columns and light fixtures are finished in matte white. Exposed metals are limited to the stainless-steel frames at the glass doors and the guardrails around exhibited objects, where the shine is reduced with a matte, directional brushed finish (Oki, 2017). The occasional slender columns of only 3.5 or 4.25-inch diameter are purposely placed off the regular 8’ x 8’ grid so that the curved glass and its optical effects, not the column grid, take priority (Nordenson and Schneider, 2016).
Balance of light and transparency
Sejima and Nishizawa’s presumably do not begin design with specific optical effects of the building. In speaking of their acrylic wall installation of 2011 at the Barcelona Pavilion, they said, “We try not to select options for which we can already imagine the outcome,” an attitude which Beatriz Colomina calls “an architecture of deliberately unclear vision” (Colomina, 2010, p. 29). On the one hand, SANAA’s project architect Toshihiro Oki affirms this was the case in Toledo. On the other, the building is an exquisite lens carefully and deliberately calibrated for optical effects. He says, “Glass has the ability to reflect the seasons, the colors, and the sky. That gives a different kind of atmosphere on any given day or season…That possibility is what they (Sejima and Nishizawa) were interested in. But that’s the unknown in a way…You have an idea, but until you see it built, you don’t know for sure” (Oki, 2017). On the one hand, effects are unknown, but on the other, the Pavilion is marked by precision and discipline characteristic of SANAA. The effects are not accidental.
The architects introduced ways to balance the light levels between inside and outside. At nighttime when electric light makes the interior brighter
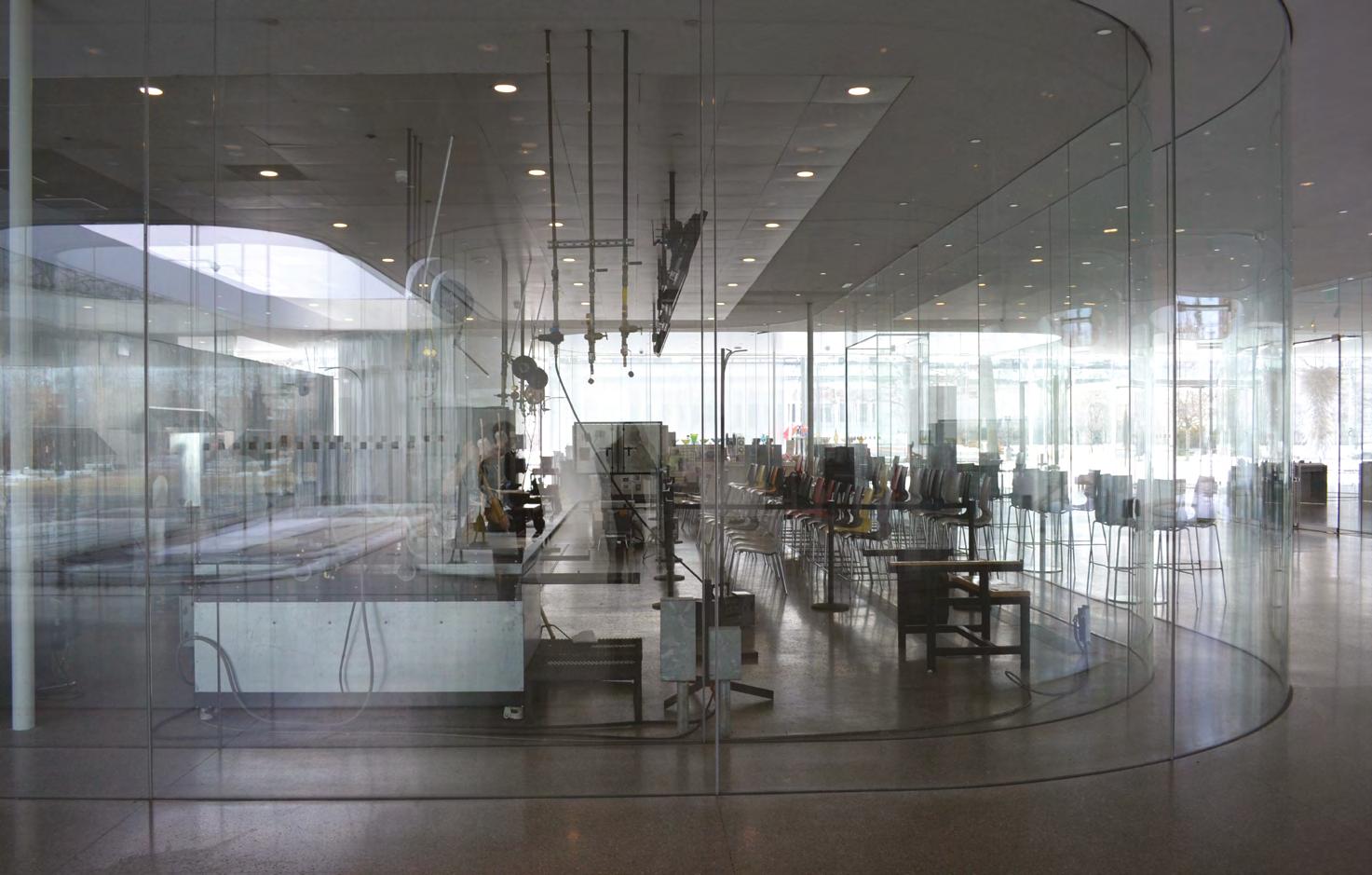
Fig. 03 From the glass-wrapped foyer between the gallery and the hot lab, a visitor can view glass sculptures and vessels, against the molten glass shaped into solid objects in the adjacent hot lab. N. Coates, 2013.
than the exterior, the vision of those inside the museum is dominated by the reflections of themselves and the interior objects. To counter this, the surrounding landscape is made artificially brighter to balance the light levels inside and out. This neutralizes the extreme differences and makes the glass appear less visible. Lighting the landscape from light poles reduced the interior reflections on the glass. Lighting designer Richard Kelly mastered this technique at Johnson’s Glass House, where the interior appears to be surrounded by walls of trees washed in spotlights (Drexler, 1993). By making the exterior brighter than the interior, a viewer inside can see through the glass without reflections obstructing the view. However, at Toledo, there are not sufficient vertical surfaces outside on which to project the light; the existing trees were too tall to be eye-level with people inside. Consequently, the interior appears brighter and more visible at night than during the day (Oki, 2017). The flickers of bright reflection spots on the glass play with the eyes to insinuate that there may be more glass rooms beyond a glass room, or perhaps it is a reflection of another.
In the daytime, the light in courtyards provides additional natural illumination for the interior to balance out the light levels. Oki also noted that a high contrast of light levels and constant views of reflections throughout the museum fatigue the eyes. The quiet, day-lit courtyards offer relief from the effects seen on glass (Oki, 2017). The natural light from the courts is filtered by curtains, which are coated with reflective aluminium flakes on the
exterior surface to both minimize solar gain and filter visible light. This effect amplifies the diaphanous qualities of the interior (Moe, 2008). The moveable curtains of the Toledo Pavilion respond to the varied light in building at different times of the year and day. Likewise, the transparency of glass fluctuates with the season, the diurnal cycle, and the observer’s position in relation to glass walls.
Clear glass intensified
Observing the glass at the Toledo Glass Pavilion uncover complex and intensified effects, as well as metaphysical readings of transparency. In the Pavilion, a curious organization of rooms in plan and material organization is coalesced with skilful lamination and bending of glass and a daring approach to structural and mechanical engineering. It couples technical innovations with SANAA’s intent to foreground the atmospheric effects over the structure by disrupting the conventional hierarchies between structure and partition,
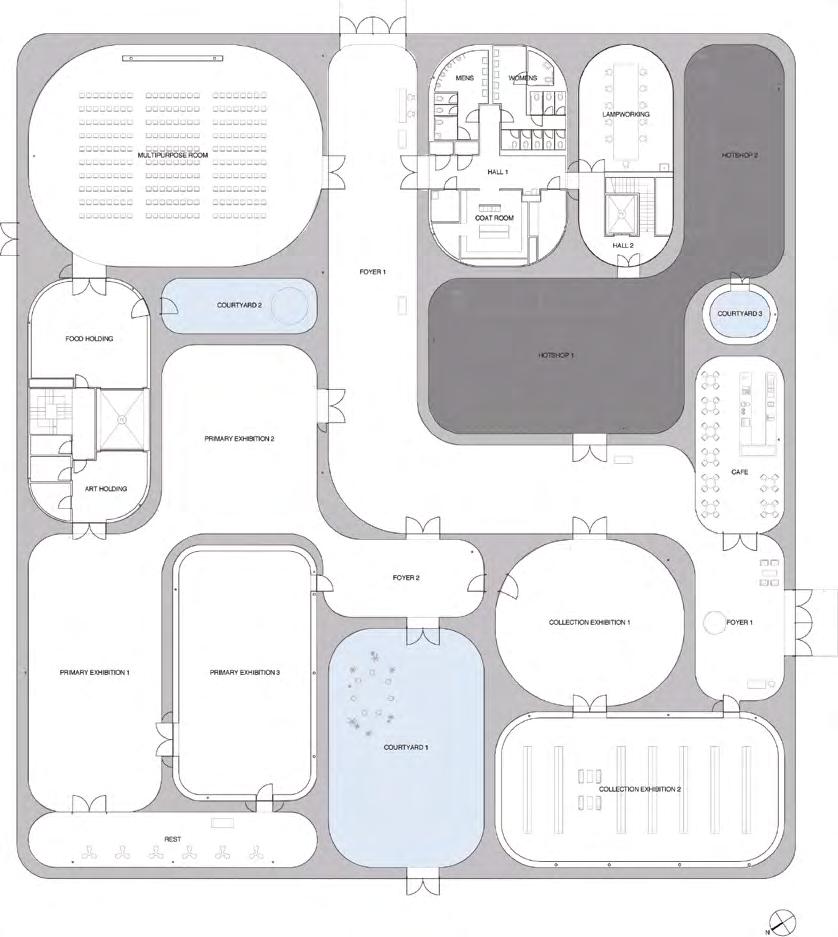
Fig. 04 Plan color coded to indicate three energy zones: perimeter buffer zone (light gray), hot glass demonstration area (dark gray), and galleries with individual climate control requirements (white). Plan courtesy of SANAA with color coding by author, 2006.
and concealment and exposure. From this approach emerges a different kind of intensified transparency of crystal-clear glass. In the design process, the architects discovered unanticipated programmatic adjacencies afforded by transparent glass. Bubble-like glass rooms are connected to yet isolated from each other by an interstitial airspace typically concealed in an opaque poche. The air cavity appears to fluctuate in opacity and thickness, like a stroke of a brush. The effect is soft, fluid, and watery, evoking return of glass to its non-rigid, liquid state, cushioned by air. The building is disciplined and precise in its execution, yet dynamic in sensual, intangible effects. In and around the pavilion, the glass continuously presents views of deep spaces beyond overlaid with reflections of locations far and near, through quick and slow motions. SANAA’s spatial organization of program, materials and light, combined with blurring of opaque-transparent hierarchy in structural and mechanical systems, result in a complex, intensified space of perception and a novel interpretation dynamic glass transparency.
References
Colomina, B. (2010). Undisturbed. In Costa, X. (ed.), SANAA: Kazuyo Sejima, Ryue Nishizawa. Barcelona: Actar, pp. 18-29. Diaz Moreno, C., Garcia Grinda, E. (2004). Ocean of Air. El Croquis. SANAA Sejima Nishizawa 1998-2004. Madrid: El Croquis, pp. 26-39. Drexler, A. (1993). The Architecture Opaque and Transparent. In Kipnis, J., Whitney, D. (ed.), Philip Johnson: The Glass House. New York: Pantheon, pp. 2-7. Ersoy, U. (2015). Glass, as Light as Air, as Deep as Water. In Mindrup, M. (ed.), Material Imagination: Reveries on Architecture and Matter. Burlington: Ashgate, pp. 155-167. Hilberseimer, L. (1995). Quoted. In Riley, T. (ed.), Light Construction. New York: Museum of Modern Art, 2004, pp. xx-yy. Lane, T. (2006). Polishing the Glass Pavilion (online). In http://www.toledoblade.com/Art/2006/06/04/Polishing-the-Glass-Pavilion.html (last accessed June 2022). Levine, S. L. (2012). Glass Pavilion (online). In https://sah-archipedia.org/buildings/OH-01-095-0097 (last accessed June 2022). Moe, K. (2008). Integrated Design in Contemporary Architecture. New York: Princeton Architectural Press. Nordenson, G., Schneider, B. (2016). Toledo Museum of Art Glass Pavilion. Structure Magazine (online). In http://www.structuremag.org/wp-content/uploads/2014/08/D-Spotlight-Nordenson-Feb-091.pdf (last accessed June 2022). Oki, T. (2017). Phone interview by author to Oki, T. (January 8, 2017). Former project architect at SANAA on the Glass Pavilion at the Toledo Museum of Art. Ra, N.M. (2017). Phone interview by author to Ra, N.M. (March 27, 2017). Principal in charge of the Glass Pavilion at glass consulting firm FRONT. Scheerbart, P. (1972). Glass architecture. In Sharp, D. (ed.), Glass architecture, by Paul Scheerbart; and Alpine architecture, by Bruno Taut. New York: Praeger, pp. xx-yy. Sejima, K., Futagawa, Y., Nishizawa, R. (2011). Kazuyo Sejima, Ryue Nishizawa 2006-2011. Tokyo: ADA Edita.