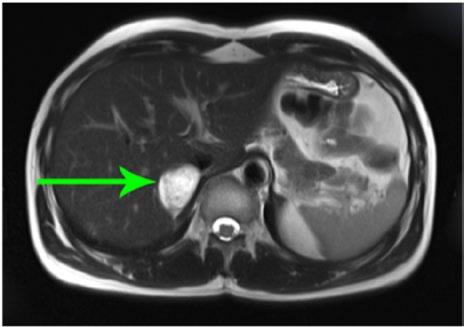
8 minute read
Genetics of Asthma Michelle Wong
PHEOCHROMOCYTOMA
MRI of abdomen. The arrow points to a tumour in the right
A pheochromocytoma is a catecholamine-secreting tumour, formed by chromaffin cells within the adrenal medulla. 90% are idiopathic, and the other 10% are related to other genetic disorders. The increase in epinephrine leads to alpha receptor activation, causing vasoconstriction, which increases blood pressure. The increase in norepinephrine activates beta-1 receptors (found in the heart) and beta-2 receptors (found in airway smooth muscles). The activation of beta-1 receptors leads to increased heart rate and stroke volume, putting one at risk of tachycardia. Beta-2 receptor activation leads to smooth muscle relaxation in the respiratory system. The Classic Triad of symptoms includes an episodic headache, palpitations and tachycardia, and diaphoresis. There are also other, less common, symptoms: orthostatic hypotension, weight loss, polyuria, often in conjunction with polydipsia, as well as paroxysmal hypertension. The onset of symptoms is often triggered by stress, anaesthesia, or labour.
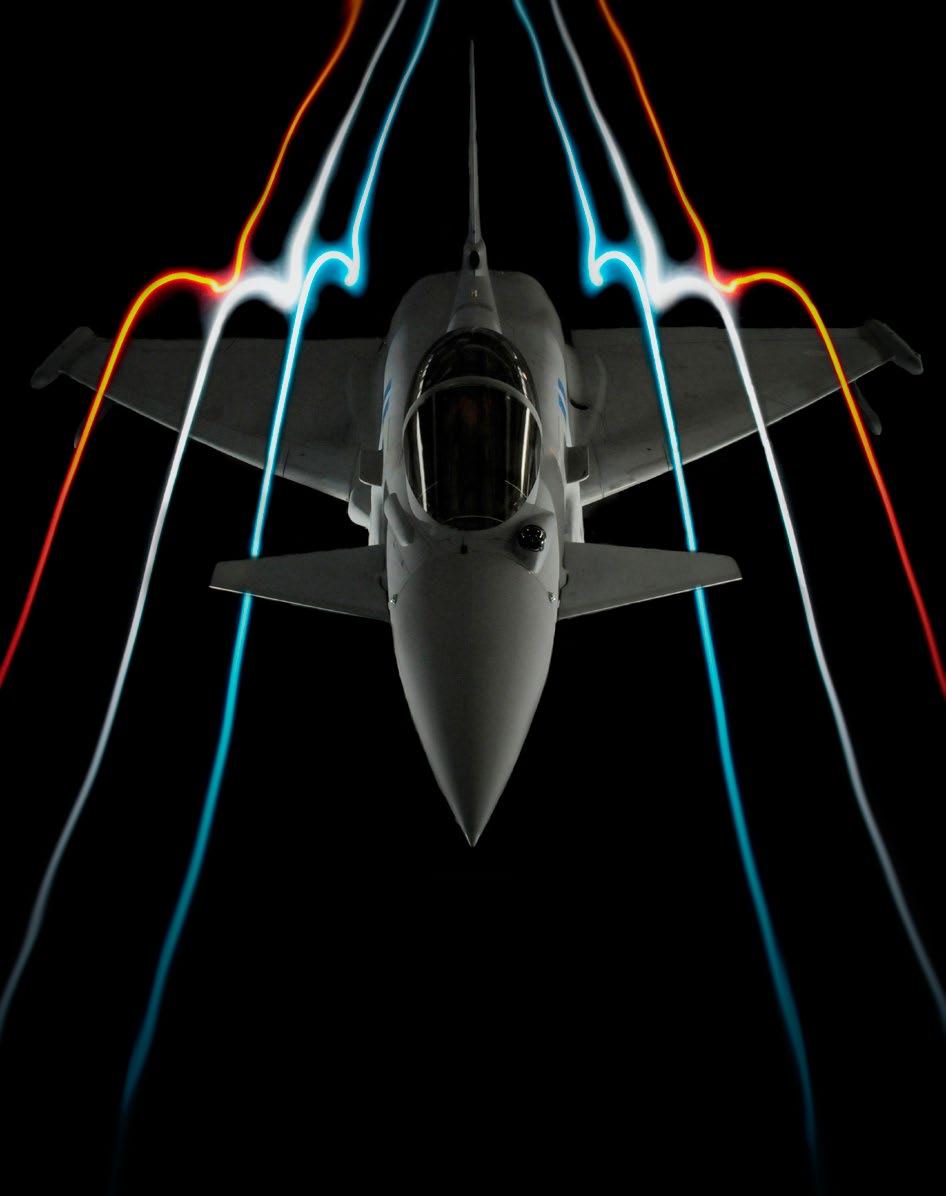
DEGRADATION OF THE CATECHOLAMINES
Dopamine is produced mostly in dopaminergic neurones, so degradation mostly occurs in the extracellular space. The initial step of this degradation is deamination by monoamine oxidase. The product here is DOPAL, a deaminated aldehyde intermediate. DOPAL lacks a beta-hydroxyl group, so binds more to aldehyde dehydrogenase (AD) than aldehyde reductase (AR), to form DOPAC. However, there is a pathway to DHMA via AR. The end product of dopamine degradation is Homovanillic Acid (HVA), which is produced when DOPAC undergoes o-methylation by catechol-o-methyltransferase (COMT). Epinephrine and norepinephrine are primarily synthesised in chromaffin cells in the adrenal medulla, or in sympathetic neurones. They are stored in vesicles and only released on activation of the neurone. However, some can leak, passively, into the neuronal cytoplasm. The initial degradation step of deamination can then occur by intracellular monoamine oxidase. The remaining steps of epinephrine and norepinephrine occur in the extracellular space. DOPEGAl (the product of this deamination) has a beta-hydroxyl group, and therefore binds more readily to AR than AD, to produce DHPG. As with dopamine, there is another pathway in this step, forming DOPET via AD. COMT is then used to form MHPG, which then forms MOPEGAL via AD. MOPEGAL is a shortlived intermediate in the degradation, so soon, via AD, becomes the end product: Vanillylmandelic acid (VMA). Norepinephrine also has an additional degradation pathway when it is first o-methylated by COMT to form normetanephrine, which, in turn, forms MOPEGAL via monoamine oxidase. The final step is the same: the formation of VMA via AD.
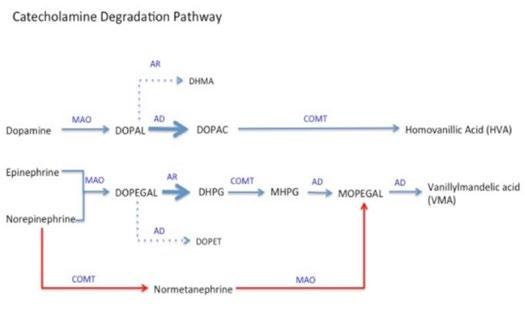
The Invisible Forces Aerodynamics
O-Teen Kwok
Aerodynamics is a branch of fluid mechanics concerned with predicting the forces and moments when an object interacts with moving air. Generally, aerodynamicists are interested in flows around airfoils - the cross-section of a wing, as well as the lift and drag produced.
HOW IS LIFT GENERATED?
The most popular ‘explanation’ of lift is Bernoulli’s principle. It states that if the pressure drops along a streamline, the velocity increases and vice versa, which is described mathematically by Bernoulli’s equation, one of the most well-known equations in fluid mechanics. Its most common form is written as:
The variables P is the static pressure, is the velocity, is the density, g is the acceleration due to gravity and h is the relative height. Bernoulli’s equation can be described as the conservation of energy for a flowing fluid, assuming a steady, incompressible and inviscid flow along a streamline. These assumptions are reasonable because air is considered to be incompressible in aerodynamics when they are flowing with the Mach number being less than 0.3 (~100m/s); whereas the effect of viscosity is only confined in a thin boundary layer around the surface of an airfoil, so it is largely negligible. Besides, the small change in height is negligible, so this can be simplified as:
Bernoulli’s principle tells us that as the velocity of air increases when it moves over the top of an airfoil, the pressure drops. As a result, the pressure gradient between the upper surface and the lower surface causes lift. However, the explanation overlooks the fact that Bernoulli’s equation applies only along a streamline and it is based on the initial assumption that air travels faster on top of an airfoil without explaining why this is so. Although Bernoulli’s principle is a fallacy, it is important to note that Bernoulli’s equation is used extensively by aerodynamicists, for instance, when calculating the freestream velocity in wind tunnels. A common misconception is the equal transit time theory which suggests that since an airfoil has a longer upper surface than the bottom, air molecules have a greater distance to travel compared to those that move under the airfoil. In order to meet up with other air molecules that started at the leading edge when t = 0, the air molecules going over the top surface must move faster. Similarly, by using Bernoulli’s equation, the lift can be explained by the pressure gradient. What’s wrong? Apart from the misuse of Bernoulli’s equation, another fundamental flaw of this theory is that the air travelling above does not get to the trailing edge at the same time as the air travelling below. If this were true, aerobatics aircrafts would not be able to fly upside down since they have symmetrical wings. In reality, the air above reaches the trailing edge before the air below as it has a significantly higher velocity. This can be observed at ease when pulses of streamlines consisting of smoke particles are fired at an airfoil shown in Figure 1. There are simply no physical laws that support the theory but frustratingly, this misleading idea is so widespread that it has even been taught in textbooks! Among all the different theories, the explanation using Newton’s third law is most applicable. Since air has mass and is redirected downwards by the airfoil, there must be an equal and opposite force acting on the airfoil. Therefore, no matter what shape the wings are, lift can be generated as long as there is a suitable angle of attack (how steep a wing is tilted) which turns the flow down. Nevertheless, this theory still fails to elucidate the lower pressure atop the wing. So, what is the correct theory? Surprisingly, there is no universal consensus on the theory of lift. Academics have been attempting to explain lift for years but they either fail to fully address the problem or lack statistical evidence. John D. Anderson, a leading authority in this field, gave a disappointing conclusion in an interview: “There is no simple oneliner answer to this”.
While the absence of a theory might seem scary, the technical side of this field has reached maturity and aerodynamicists are able to accurately predict flow behaviours using both numerical and analytical methods, leading to an array of complex applications.

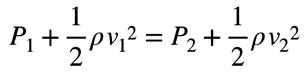
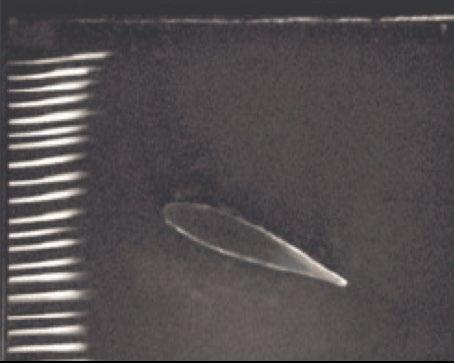
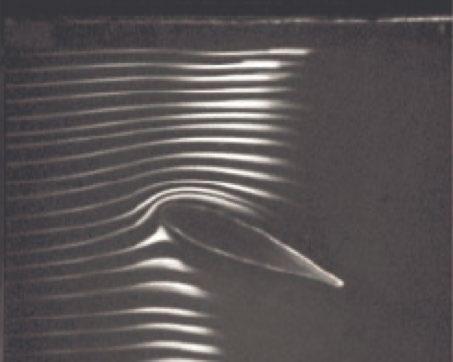
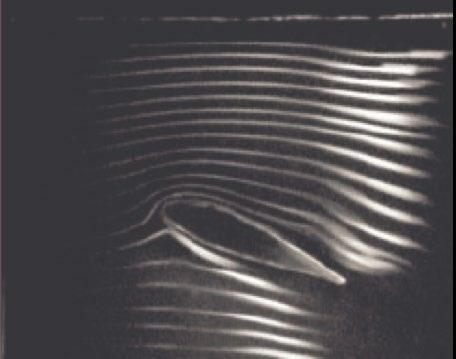
DRAG AND SEPARATION
Compared to lift, drag seems to be more intuitive as one could easily imagine the resistive force when walking in a pool. Drag forces are caused by two different sources, pressure and shear stress. Pressure p always acts normal to the body surface, whereas shear stress τ is the stress distribution that is tangential to the body surface. The drag force is the resultant of these two sources in the direction of the flow.
When air flows through an airfoil, a boundary layer is formed around the surface, where air travels slower as it gets closer to the surface due to friction forces. In addition, only above the boundary layer can the flow be regarded as inviscid. As fluid elements separate at the stagnation point, those which flow over the top accelerate. This acceleration must be caused by a pressure gradient known as the favourable pressure gradient. Beyond a certain point, the flow starts to retard and so the pressure in the flow direction
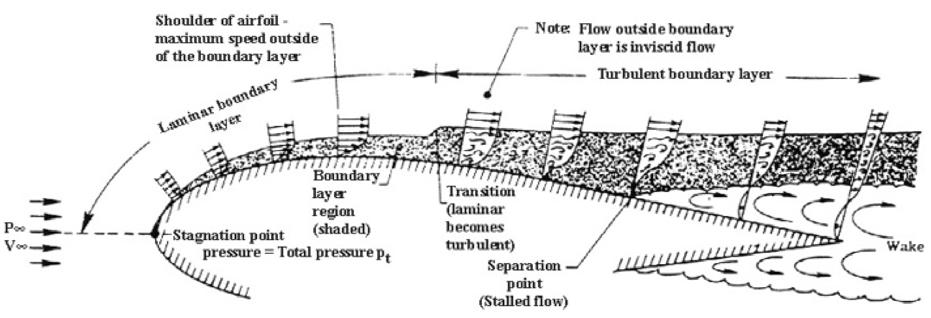
increases, pushing the fluid back. This increase in pressure is called the adverse pressure gradient. If it acts over an extended distance, the deceleration will eventually be great enough to reverse the direction of flow. Since the flow cannot travel backwards because of the oncoming air, the flow detaches from the boundary layer and a turbulent flow is produced around the surface instead, resulting in a flow separation as shown in figure 2. The turbulent flow produced increases the pressure and thus reduces the pressure gradient. This could lead to a significant loss of lift and a gain of drag when having a large angle of attack (wings are tilted up too much). In aviation, this is known as stall and could lead to catastrophic consequences; for example, causing plane crashes. In aerodynamics, laminar flow is the airflow in parallel layers, with no disruption between them. In contrast, turbulent flow is a flow regime characterised by chaotic property changes in which the airflow mixes across. To delay flow separation, we could promote turbulence since the turbulent boundary layer has a more rapid increase in velocity along the y-direction than that in a laminar boundary layer as shown in figure 3. The resulting higher kinetic energy of the turbulent boundary makes it less susceptible to the adverse pressure gradient and therefore, shifts the separation point downstream. For example, the dimples on a golf ball are designed to cause vortices which result in a turbulent flow. This delays flow separation and reduces the pressure drag so that the golf ball can travel farther.
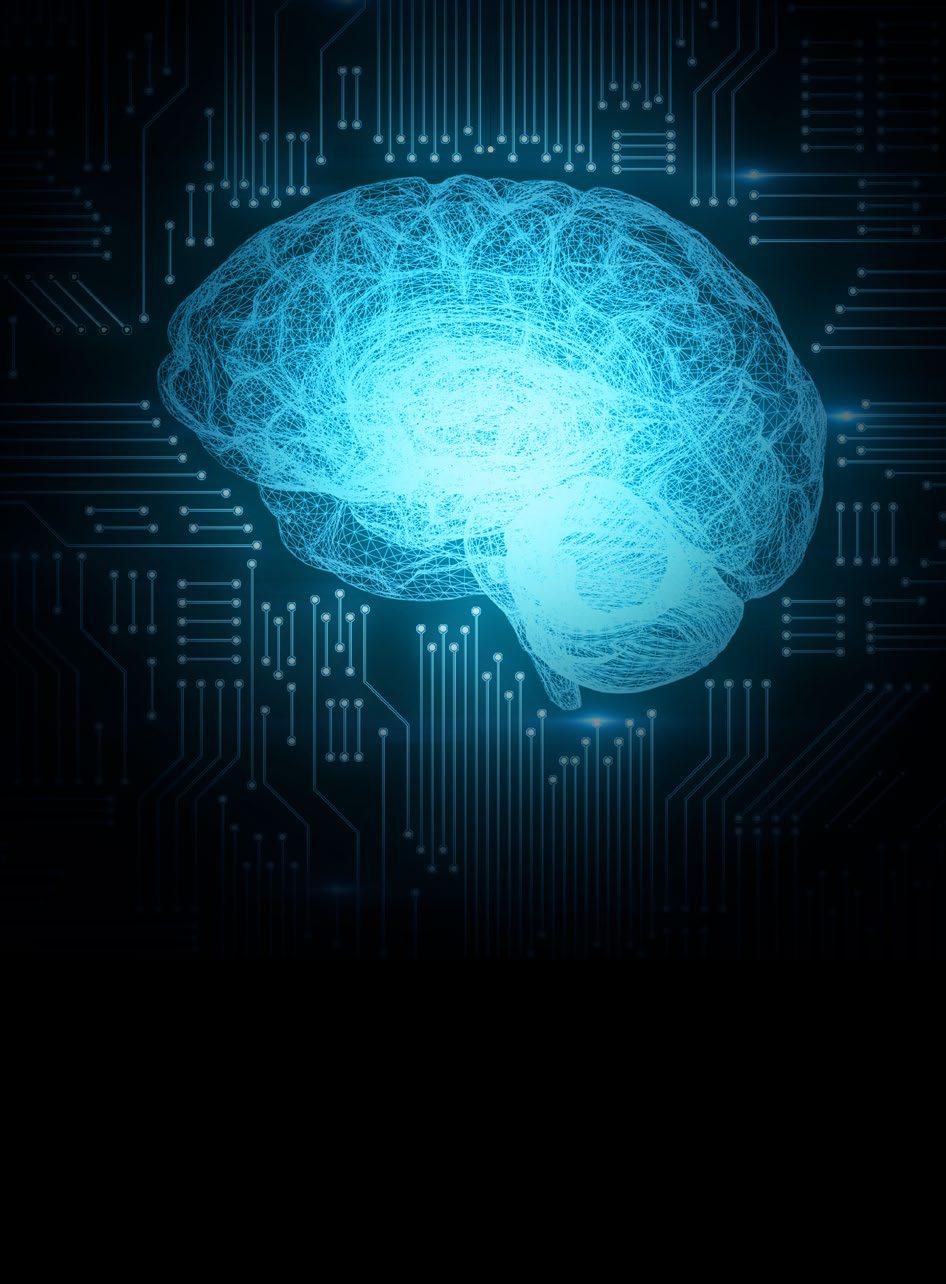
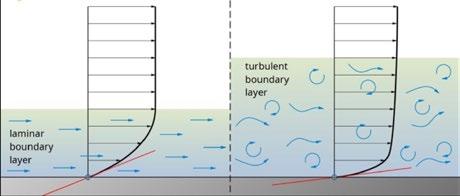
Figure 3. Velocity profiles in a laminar and turbulent boundary layer
Brain-Computer Interface
Chelsea Chen