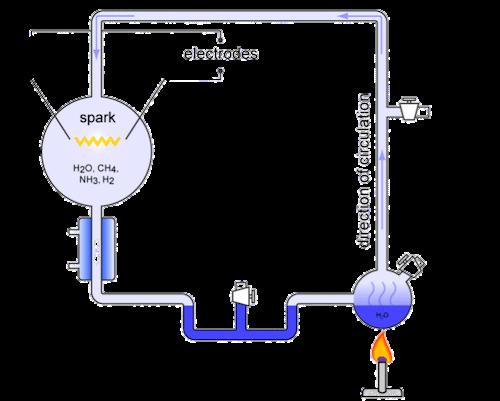
7 minute read
Feature: The origins of lifeVanessa Tsui
The origins of life
Vanessa
Advertisement
Tsui, SFC1explores the age-old question of where and how life came about. You go into the shower and this thought springs into your mind: "How did I come into existence?" At first the answer seems obvious to you: you were birthed from your mother. But what about before her? What starts the evolution of complex organisms, or even simple organic life itself? Scientists have been trying to answer this question for years. While not entirely dismissing the possibility of extra terrestrial origins, they might have come up with some theories in the past century.
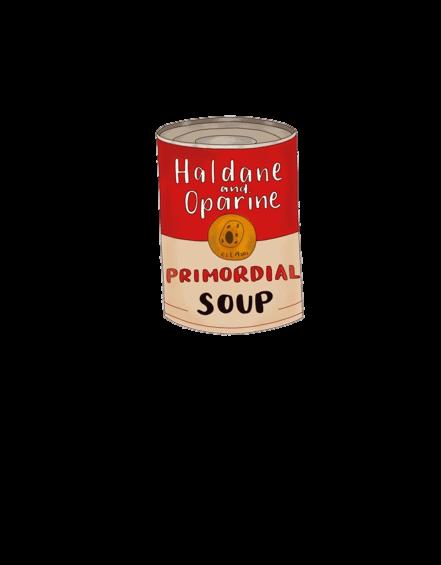
In the beginning...
In the 1920s, two scientists, J. B. S Haldane and Alexander Oparin theorised that life may come from a ‘ prebiotic soup’ or a primordial soup; they imagined the early ocean was rich with complex molecules produced by natural chemical reactions. In this soup, further reactions can take place, eventually producing living cells. This speculation actually dates back to 1871 when Charles Darwin wondered if life could have formed from chemicals "in some warm little pond" . It is thought that about
IllustrationbyReginaLo
4 billion years ago was the earliest possible time that the first life could emerge on Earth, a period called the habitability boundary. Fossil evidence shows that microbes existed about 3.7 billion years ago, known as the biosignature boundary. At some point between the habitability boundary and biosignature, non-life became life. This miraculous arising is what scientists call Abiogenesis. But what is life? At school, we learn MRS C GREN, the checklist for the characteristics of life of what we know today. The earliest life, however, is a lot simpler. The definition for life is so vague that many scientists refuse to bother with it. In 1944, however, Schrödinger defined life as ‘ a struggle against entropy, the persistent resistance to decay, the preservation of disequilibrium’ . That is to say, a thing is considered alive when it fights for survival, and it also means that life will constantly evolve to better adapt to survive.
‘A struggle against entropy, the persistent resistance to decay, the preservation of disequilibrium. ’ The soup of life
Going back to the primordial soup, Darwin’s and Oparin’s ideas were only speculation and not legitimate hypotheses since no one could find a way to test or observe them back then. In 1953, two other scientists called Stanley Miller and Harold Urey tried to replicate the formation of life by simulating early Earth conditions in the lab and carefully watching what happened. They designed a water apparatus to model the ancient ocean and gently boiled to mimic evaporation. Along with water vapour, they chose methane, hydrogen and ammonia as the gases in the atmosphere. As a source of energy, they added electrical sparks using 2 metal electrodes to simulate lightning. Their goal was not to recreate life, but to test the first step of Oparin’s model - can simple chemistry naturally give rise to the complex molecules of life? After running the experiment for a week, their ocean became brownish-black. Careful analysis revealed that many complex molecules indeed formed, such as amino acids (the building blocks of life) which were previously thought to only be produced inside our bodies. However, scientists later criticised this model because they couldn’t know for sure if the gases used by Miller really were the most common gases of the ancient earth. Because of this, many other simulations have since been done to show that the molecules of life can form in a wide range of environments, including hypothermal vents or on the earth with the help from the sun’s radiation.
The experimental set up for the Miller-Urey experiment. Image credit: "Miller and Urey's experiment,
What comes next?
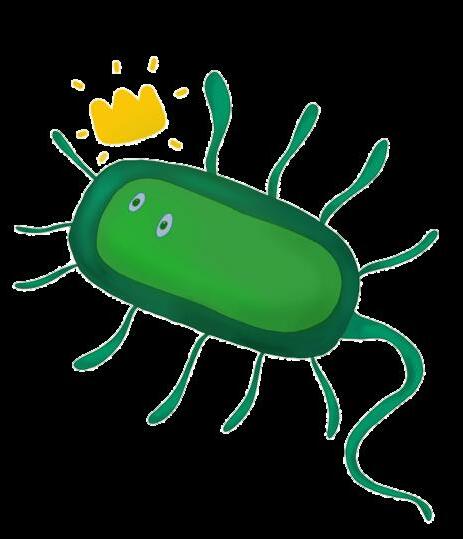
Despite the possible limitations of the model, organic molecules needed to somehow give rise to cells for the first life to appear. In 1977, a piece of the puzzle was uncovered that involved rocks, in particular, minerals. It was found that the amino acids assemble on the clay surfaces to form small proteins once the water has evaporated. It turns out that clay minerals have the ability to take up organic molecules, protect them against the UV radiation in addition to concentrating and catalysing their polymerisation. And so, some minerals could support the synthesis of large protein chains, a first step to protein synthesis. One of the necessities of a cell is their cell membrane as they require something to compartmentalise inner contents, keeping it from escaping into the surrounding waters. The solution to this is a membrane with lipid molecules. Since lipids do not mix well with water, how can they possibly surround the aqueous cell? It was found that those from egg yolk mixed with water spontaneously give rise to tiny spherical vesicles with lipids surrounding them. The two layers of lipids cleverly arranged themselves in a way that their backs were away from the water and the part of the molecule that tolerated water was in contact with it.
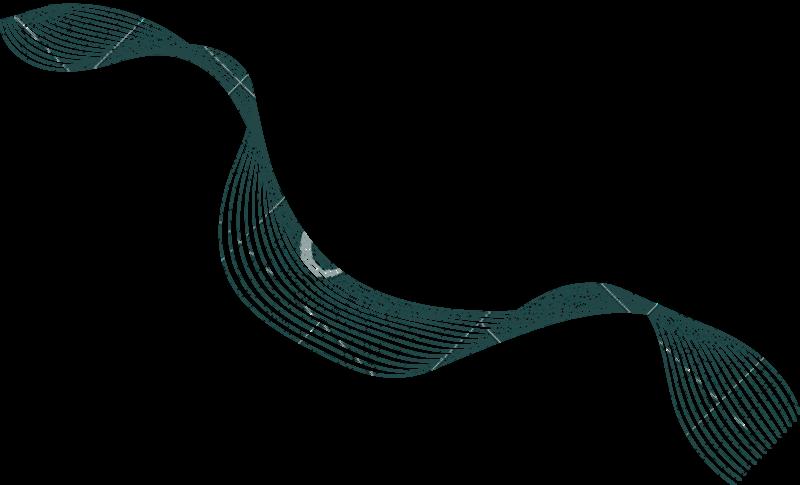
Hence, a membrane-bound vesicle is formed when clays are present and encourages lipid molecules to form membranes. These vesicles undergo multiple cycles of growth and division. If a vesicle also contains some material such as nucleic acid, water would move in by osmosis and the vesicle would fuse with empty vesicles, increasing its surface area. This would then stimulate it to divide into smaller vesicles. Later, natural selection would choose the best vesicle to be the primitive cell. If I were to ask you to describe our relationship as humans with bacteria, "partner in crime" would probably not be the first phrase to come to mind. Yet, bacteria are our ancestors. Without them, we could not have been the complex organisms we are, or have even come into existence. 3.5 billion years ago, the earth's atmosphere mainly consisted of nitrogen, carbon dioxide and methane, lacking the fundamental gas for us aerobics - oxygen. All oxygen is locked up in molecules such as water. Due to the absence of a protection ozone layer, the land was bathed in lethal levels of UV radiation so that the only shelter for life was underwater. The ocean was therefore populated with anaerobic microorganisms which did not require oxygen for growth, where they would have died or reacted negatively if exposed to large amounts. Until one day, somewhere around 2.5-3.5 billion years ago, a microbe evolved to photosynthesise. This very special microbe is the primitive cyanobacterium. This is the single cell organism that caused the first mass extinction on Earth and paved the way for complex life. The chlorophyll that evolved in their cells could harvest the energy from sunlight, turning carbon dioxide and water to oxygen and sugar which they used for energy. Photosynthesis gave these cyanobacteria a huge advantage over other species. They could now produce their own energy from an endless supply of raw ingredients. Their population skyrocketed, and they started polluting the atmosphere with a new waste product: oxygen. This was so toxic to the anaerobes that it caused an extinction of virtually all life on Earth, leading geologists to denote this as the Oxygen Catastrophe. Eventually, life adjusted as aerobic organisms evolved and increased, absorbing the excess oxygen until oxygen levels reached the 21% of air we know today. This event was so pivotal because it gave organisms the boost of energy they needed to diversify since aerobic respiration produced much more energy than anaerobic respiration. Cyanobacteria were swallowed by a larger microbe in the process of endosymbiosis, leading it to become a more complex organism with the combined abilities of both. It is thought that cyanobacteria are the ancestors of plant cells in this way. The endosymbiotic theory did not stop there… mitochondria originated as oxygen- breathing bacteria with their own DNA were somehow absorbed into an eukaryotic cell. Working together with their host cell and dividing inside them, this allow the evolution of a more complex organism providing them with the energy for growth and replication. And this is what makes life as we know today.
An example of micelle formation in a PEGylated Liposome, which shares a structure similar to a cell membrane (Muralidharan, 2014).
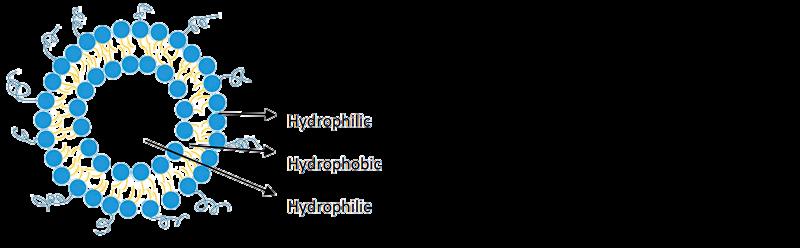