DCQUANTUM
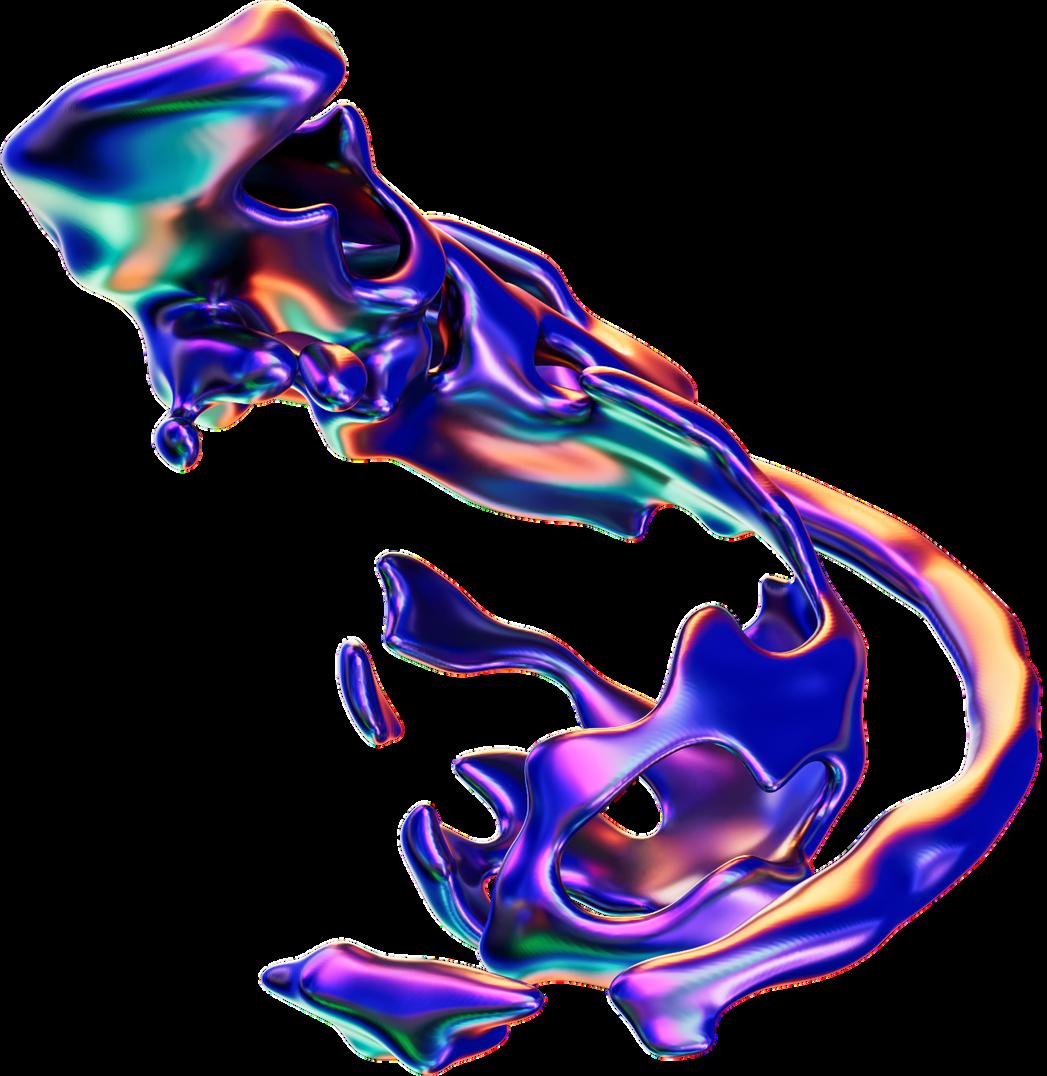
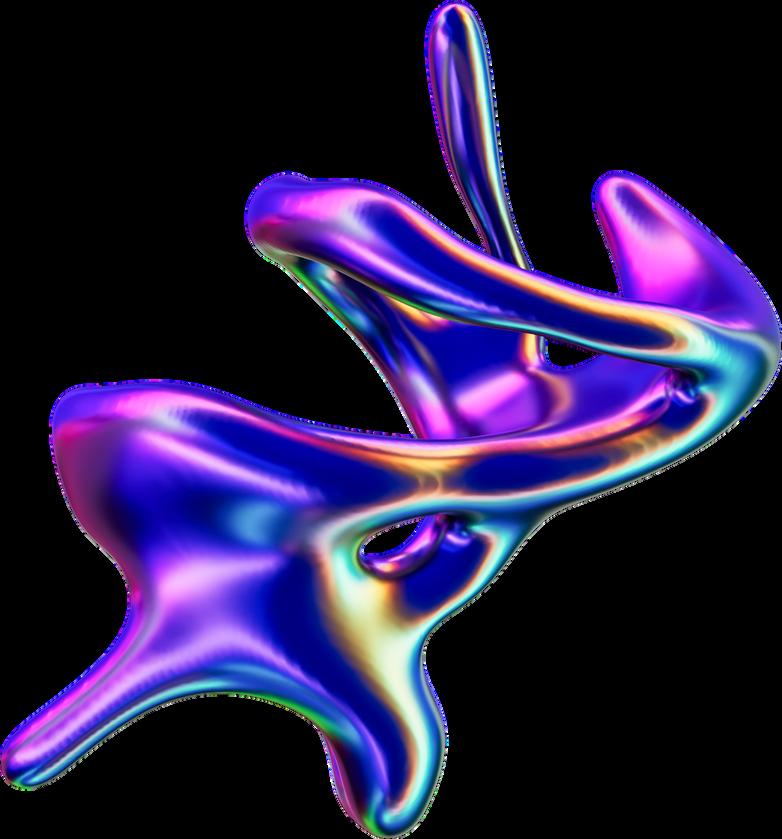
The concept of time travel has captivated human imagination for centuries, fueling countless works of fiction and inspiring endless philosophical debates. While time travel remains purely speculative in reality, exploring its physics can shed light on the fascinating principles that govern our understanding of time and space. In this article, we will delve into the physics of time travel, examining the theories, paradoxes, and possibilities that surround this fascinating subject.
In order to grasp the physics of time travel, we must first comprehend the concept of the space-time continuum. According to Einstein's theory of general relativity, space and time are inextricably linked, forming a fourdimensional fabric known as space-time. Objects with mass and energy, such as stars, planets, and even ourselves, warp this fabric, creating what we perceive as gravity.
One of the key tenets of the theory of relativity is time dilation, which asserts that time can be stretched or contracted depending on the relative motion between observers. This phenomenon has been confirmed by various experiments and is an essential component of understanding time travel.
When an object moves at high speeds or experiences strong gravitational fields, time slows down for it relative to a stationary observer. This effect is known as time dilation. As an object approaches the speed of light or enters an intense gravitational field, the difference in the passage of time becomes more pronounced. This discrepancy creates the possibility of traveling forward or backward in time, albeit in a limited sense.
When discussing time travel, the Grandfather Paradox often emerges as a paradoxical conundrum. It postulates that if one were to travel back in time and prevent their own birth or kill their own grandfather, a contradiction would arise. This paradox highlights the potential conflicts that arise when causality is violated, creating a loop in which events cause themselves. In physics, these loops are referred to as causal loops. The notion of causal loops challenges our understanding of cause and effect, introducing the possibility of selfcreated events that exist outside linear time. Resolving these paradoxes remains an open question in the physics of time travel, emphasizing the complexity and inherent limitations of our current understanding.
While the physics of time travel continues to intrigue scientists and theorists alike, it remains a subject of speculation rather than a reality. Concepts such as time dilation, wormholes, and causal loops form the foundation of our exploration, shedding light on the intricate interplay between space, time, . and the laws of physics. As we continue to unravel the mysteries of the universe, understanding time travel may offer insights into the nature of reality itself
By Arnav Singh 9JBEreferences:
Einstein, A. (1916). Relativity: The Special and General Theory.
Thorne, K. S. (1994). Black Holes and Time Warps: Einstein's Outrageous Legacy.
Hawking, S. W. (1998). A Brief History of Time.
Morris, M. S., & Thorne, K. S. (1988). Wormholes in spacetime and their use for interstellar travel: A tool for teaching general relativity. American Journal of Physics, Novikov I. D. (1991). Time machine and self-consistent evolution in problems with f-interaction. Physics-Uspekhi,
The furthest man has travelled through interstellar space is to the dark side of the moon. However, beyond this lies a sphere that has the potential for human colonisation by terraforming the atmosphere, a planet capable of supporting human life shortly. Our next goal is to reach the Red Planet, Mars. Today, when scientists observe the Martian surface, they see features resembling river valleys, basins, and streams, hinting that the planet hosted ecosystems. This discovery has piqued the curiosity of researchers, scientists, and engineers.
Terraforming is the process by which a celestial body is altered to be inhabitable for humans, making it more Earth-like. Mars has two main problems: the temperature and the lack of oxygen in the atmosphere. It has a temperature of -153°C-20°C, making it virtually impossible for humans to live on it. However, ironically, the process that is making Earth increasingly uninhabitable for humans has the potential to allow human life on Mars: the greenhouse effect. Mars contains a significant volume of frozen carbon dioxide, so by evaporating this greenhouse gas, we can increase the temperature of it, over time. This, on the other hand, would be quite costly as the power needed to achieve the desired heating is 27 terawatts, equivalent to firing the United States' most competent weapon, the B-53, every 20 minutes.
Another solution to the low temperatures is to purposefully ignite asteroids which would be intentionally directed to Mars. The asteroids' gravitational potential energy is transformed into heat rather than being created by nuclear fusion. The energy released into the system by burning up one asteroid with mass 1015 kg is comparable to 330,00 B-53 bombs, or 1.265x 10^22 J. Moreover, Mars has only 1% of oxygen in its atmosphere, (20% less than the atmosphere of earth). A solution for this would be to use plants, which would thrive in the carbon dioxide-rich environment and (soon to be) warm atmosphere. The plants would also be used to slowly convert carbon dioxide into oxygen, making it more inhabitable for humans.
The current technology used to power rockets uses a chemical engine. Chemical propulsion systems use the combustion of propellants to produce exhaust gases. These exhaust gases can be accelerated (by high temperatures and pressures) through the nozzle, thus providing thrust.
A CAD image of a nuclear thermal propulsion system (Picture by NASA)
A solution that can be extremely useful in achieving the thrust required to reach Mars, while carrying the mass of astronauts and supplies is the employment of nuclear thermal propulsion systems, because fuel rods made of nuclear substances are extremely energy-dense, therefore only require small masses of fuel to achieve the same velocity.
Nuclear thermal propulsion systems push a liquid propellent, usually hydrogen, through a reactor core. This results in the uranium nuclei splitting into two daughter nuclei (nuclear fission), releasing heat. The heat converts the propellant into gas, providing thrust for the rocket after it expands through a nozzle.
ncy of a propulsion system is easured in specific impulse (a ure of how fast the propellant shoots out of a rocket). The pecific impulse for hydrogen is around 900 s for a fuel temperature of 2800K, whereas other propellants such as H₂O (for a nuclear chemical propulsion system) ave a specific impulse of only 75 s at the same temperatures gher specific impulse requires l to travel the same distance). use the mass of the propellant ional to the acceleration that fore greater efficiency can be o travel further on less fuel.
Another promising method is to use antimatter fuels to power our rockets. We can annihilate the antimatter by colliding it with matter, which would result in the production of massive amounts of energy. The energy produced by an antimatter f l ll an be calculated using Einstein’s famous equation, E=mc^2, where E is the energy produced, m is the mass of antimatter and c is the speed of light.
Overall, work done by engineers and scientists can significantly accelerate the production of various technologies, which in turn can take us to Mars. Examples include implementing nuclear fusion-powered propulsion systems, in place of the currently used chemical engine systems. Once we reach Mars, we still must ensure that the environment is suitable enough to sustain human life.
Day C. Terraforming Mars. Physics Today. 2021 Jul 1;74(7):8-.
Vasileva I, Ivanova A, Alexandrov S. Terraforming Mars is not out of the question yet and microscopic algae could help. Trakia Journal of Sciences. 2019 Jan 1;17(1):9.
Zhang Y. Advanced nuclear power engine: A brief overview of gas core reactor for space exploration.
By Arush AgarwalInternational Journal of Advanced Nuclear Reactor Design and Technology. 2023 hta A. Antimatter as an Energy Source. , Carroll K, Nikicio A, de Weck O, Hoffman J. Multi-Objective system of a Mars atmospheric ISRU plant for oxygen production. In 2021
E Aerospace Conference (50100) 2021 Mar 6 (pp. 1-12). IEEE.
From the ancient travelers charting their course by the stars to the pioneers of aviation breaking the sound barrier, humanity has always been marked by a curiosity to explore and push boundaries. Today, our sights are set on the most significant frontier yet - the cosmos. The vast distances between star systems, however, present a unique challenge. How do we traverse such great cosmic distances within a human lifetime? This leads us to intriguing ideas like faster-than-light travel, warp drives, wormholes, and other theoretical concepts. These notions stretch our understanding and offer exciting possibilities for crossing astronomical distances to the furthest corners of the universe in the blink of an eye. In this article, we will journey to the edge of our current knowledge, exploring these fascinating ideas and their potential to unlock the stars for future generations.
"In the universe, nothing can travel faster than light."
The image depicts an Alcubierre warp drive bubble, which illustrates spatial constriction in front of the bubble and spatial enlargement at the rear.
Credit: NASA
This cosmic speed limit, dictated by Einstein's theory of relativity, presents a significant challenge to interstellar travel. Even at the speed of light, reaching the nearest star system, Proxima Centauri, would take over four years. But what if we could bend the rules? This is where the concept of warp speed comes into play. Instead of moving through space, the idea is to move space itself. This begins our journey with warp drives. The Alcubierre Drive, named after Mexican physicist Miguel Alcubierre, is a prime example of this concept. This theoretical model, derived from Einstein's s in general relativity, proposes a method for faster-than-light travel.
m involves a spacecraft staying stationary within a local region of flat
10 space, known as a warp bubble, while manipulating space itself to move.
This is achieved by contracting space in front of the spacecraft and expanding space behind it, like a surfer riding a wave.
The key to this concept is exotic matter, a hypothetical form of matter with a negative energy density and negative pressures. This exotic matter would be responsible for creating the warp bubble and enabling space-time to contract and expand, allowing any object in this bubble to ride the region. This is known as the "Alcubierre Metric". In the context of General Relativity, it allows a warp bubble to appear in a previously flat region of spacetime and move away, propelled to reach ‘ warp speed’. While we don't yet have concrete evidence of its existence is allowed within the laws of quantum physics, overy is a prerequisite for the reality of the Alcubierre Drive.
Beyond warp speed, another theoretical concept that offers substantial possibilities for faster-than-light travel, is wormholes. The "bridges" or "tunnels" through the fabric of space-time could link distant points in the universe, allowing for near-instantaneous travel between them. Formally known s Einstein-Rosen bridges, they are a solution to Einstein's field equations of general relativity. They are conceptualized as a tunnel with two ends, each at separate points in spacetime, at potentially great distances from each other. This could
warp speed, another theoretical concept that offers substantial ties for faster-than-light travel, is wormholes. The "bridges" or rough the fabric of space-time could link distant points in the r near-instantaneous travel between them. Formally known as Einstein-Rosen bridges, they are a solution to Einstein's field equations of general relativity. They are conceptualized as a tunnel with two ends, each at separate points in spacetime, at potentially great distances from each other. This could mean a pathway between different points in our universe, or even between different universes altogether. To take an example, if we use a two-dimensional sheet of paper, and mark two distant points on the sheet.
Bending the paper such that the two points touch, essentially creating a direct path between them, encapsulates the idea of a wormhole, where the sheet of paper is spacetime, and the two points are the locations. There are two main types of wormholes – non traversable wormholes, which are non-traversable due to their rapid collapse, and traversable wormholes. But what is a wormhole made from? In the case of traversable wormholes, exotic matter can be used to hold open the tunnel, similar to the exotic matter used in the Alcubierre drive. This exotic matter is used to counter the natural tendency of the wormhole to collapse. To illustrate, consider a mountain tunnel. Without support structures, the tunnel would collapse under the weight of the mountain. In the case of wormholes, the 'mountain' is the gravitational pull of the universe trying to close the wormhole, and the 'support structures' are made of exotic matter.
In this exploration of interstellar travel, we've delved into the intricacies of the Alcubierre drive, wormholes, and exotic matter, and are beginning to glimpse at the possibilities that lie ahead. Yet, there remains a lot of knowledge to uncover in the realm of interstellar travel. With the advent of modern science and technology, what was once science-fiction is gradually becoming a possibility.
Tachyon propulsion, miniature black hole propulsion, matter-antimatter annihilation engines, and quantum vacuum plasma thrusters, to name a few, are also intriguing possibilities within our reach. These ideas push the limits of our understanding, inviting us to envision a future where the stars are not just points of light in the night sky, but destinations waiting to be explored. As we stand at the threshold of the cosmos, we can dream of a future where the vastness of space is not a barrier, but a beckoning call to adventure.
Wikipediacontributors.Alcubierredrive.InWikipedia,TheFreeEncyclopedia. RetrievedJune2,2023,fromhttps://enwikipediaorg/wiki/Alcubierre drive Wikipediacontributors.Exoticmatter.InWikipedia,TheFreeEncyclopedia. RetrievedJune3,2023,fromhttps://enwikipediaorg/wiki/Exotic matter Yirka,B(2017,January3)AlcubierrewarpdriveremainsadreamPhysorg RetrievedJune3,2023,fromhttps://physorg/news/2017-01-alcubierrewarphtml,WikipediacontributorsWormholeInWikipedia,TheFree Encyclopedia.RetrievedJune12,2023,from https://enwikipediaorg/wiki/Wormhole Redd,N.T.(2017,January12).WhatAreWormholes?Space.com.RetrievedJune 16,2023,fromhttps://wwwspacecom/20881-wormholeshtml
Hamilton,AJS(nd)JourneyintoaSchwarzschildwormholeJILA-Universityof ColoradoBoulder&NISTRetrievedJune16,2023,from https://jilacoloradoedu/~ajsh/bh/schwwhtml
Warp Drives: Yirka, B (2017, January 3)
Alcubierre warp drive remains a dream Phys org: https://phys.org/news/2 017-01-alcubierrewarp html
Michael (n d ) Warp Drive Michael's Notebook: https://michaelnotebook. com/wn/warp drive html
Wormholes: O’Neill, D (2019, October 23). The Physics of Wormholes Medium: https://medium com/swlh /the-physics-ofwormholes-654facefd2ea Yale University (2021) Wormholes and Entanglement. Yale Physics Department: https://physics yale edu/s ites/default/files/files/W ormholesAndEntanglement 2021 pdf
Other:
Antimatterengines: https://ntrsnasagov/api/citations/20200 001904/downloads/20200001904.pdf
QuantumVacuumPlasmaThrusters: https://ntrsnasagov/api/citations/201400 13174/downloads/20140013174pdf
Tachyons: https://enwikipediaorg/wiki/Tachyon Blackholepropulsion: https://enwikipediaorg/wiki/Black hole s tarship
Medicine is the aspect of science through which we care for patients and manage their diagnosis, prognosis, prevention, treatment, and palliation of their injury or disease. Medicine has come a long way since 3000 B.C. when the first doctors are estimated to have existed. There have been many breakthroughs in the science behind medicine and the human anatomy; however, in recent years, perhaps the ost important breakthroughs have been technological.
nce is human intelligence demonstrated by machines. d systems have rapidly increased in number as well as he past decade. AI can also be used for medicine, and efits of AI in medicine consist of the following: more
y when giving prescriptions as well as in surgery, and ed efficiency of hospitals financially, which can allow more money to be spent on areas of medicine rather than being wasted. Improving patient outcomes is likely the most important reason that AI has started to be implemented at hospitals in some aces around the world. However, there are also some d to AI: the difficulty of integrating AI in the already anaging the AI, and hiring the world’s best coders to
n also cost a lot of money, as well as the risk of the AI being hacked, which could harm many lives
.
In addition, AI makes it possible to put all medical knowledge to use in any case. Properly designed AI has the potential to make our health care system more efficient and cheaper, ease the paperwork burden that has more and more doctors considering new careers, fill the gaping holes in access to quality care in the poorest places, and, among many other things, serve as an unblinking watchdog on the lookout for the medical error estimated 200,000 people and cost $1.9 billion a
Faster and more accurate diagnoses are anothe in medicine. COVID-19's detection by AI algor recent example of this. The University of Centr reported that the algorithm correctly detected of the time and negative cases 93% of the time. second opinion for physicians and help them d analysis is another way AI is used in health care be predicted how susceptible a patient is to cer
Nevertheless, AI works by learning from the data it is fed. The data the systems receive must be accurate. It is important to remember who is inputting the data. Are cultural biases being passed on to the system? If the system is faulty, it can misdiagnose. Codes of ethics must be followed when using AI systems to ensure harmonious interactions with humans. Audits and testing should also take place regularly. This can ensure the systems are unbiased.
Another potential disadvantage of integrating AI in medicine is the risk of leaking patient data. These systems will collect patient data, which also brings ethics into play. Protecting patients from this is integral to the safe use of AI in medicine. They need to be made aware that their information is being used to feed an AI algorithm and is not limited to doctors. Security breaches are less likely to manifest if proper protocols are in place.
One way AI is being used is with CRISPR-Cas9. It is an innovative technique that geneticists and medical researchers use to remove, add, or modify DNA sequences in the genome. In the laboratory, researchers regularly use CRISPR to alter genes in plants, bacteria, and animal models. CRISPR is being used at ersity of Pennsylvania to study the possibility of treating multiple eloma, a blood cancer. Sarcomas have been treated with the same technique.
that CRISPR-Cas9 is a highly useful tool and one that can play a g part in how we treat some diseases in the future. But how is AI playing part in CRISPR-Cas9? "The primary risk associated with PR-Cas9 technology is the potential for off-target genome editing effects. CRISPR/Cas9 technology can induce site-specific DNA ations in human DNA" (as stated by UCLA). However, "Machine ning models have been proven to produce the best results when it o predicting the degree of both guide-target interactions and offget effects for a given sgRNA. This can significantly speed up the ment of guide RNA for every region of human DNA." by Markus cle for Data Revenue. This means that the use of AI in CRISPRfficiency of the process and reduces the risk of the process which could save many lives and allow for the creation of new cures.
AI can have a major role in medicine in the future, in addition to the roles it already plays today. We have seen that AI can have many benefits in medicine, such as improving patient outcomes, which is the most important thing in medicine, and increasing the efficiency of medicine, which will dramatically improve finances as well as help doctors with prescriptions. However, these benefits outweigh the few disadvantages that creasing the role of AI in medicine. These disadvantages consist of of the difficulties in integrating AI with medicine, making the AI itself faulty as well well as the potential risks of data leaks and bias in the AI. Overall, it is clear that, despite despite the risks that AI poses, the continued integration of AI in medicine can be highly beneficial to everyone and help make medicine even more effective than it already is.
Daniel, M. (2016) Study suggests medical errors now third leading cause of death in the U.S.05/03/2016, Johns Hopkins Medicine, based in Baltimore, Maryland. Available at: https://www.hopkinsmedicine.o rg/news/media/releases/study s uggests medical errors now thi rd leading cause of death in t he us (Accessed: 10 June 2023).
Mills, T. (2021) Council post: The risks and benefits of AI in medicine, Forbes. Available at: https://www.forbes.com/sites/forbestechcouncil/2021/03/23/the-risks-andbenefits-of-ai-in-medicine/?sh=26e1f20658d8 (Accessed: 10 June 2023).
CB Insights (2021) What is CRISPR?, CB Insights Research. Available at: https://www.cbinsights.com/research/what-is-crispr/ (Accessed: 10 June 2023). (No date) Hazard communication: CRISPR/cas9 technology definitions. Available at: https://rsawa.research.ucla.edu/wp-content/uploads/hazardcommunication-crispr-cas9.pdf (Accessed: 10 June 2023).
Schmitt, M. (no date) Artificial Intelligence in medicine: the top 4 applications, RSS. Available at: https://www.datarevenue.com/enblog/artificial-intelligence-in-medicine (Accessed: 15 June 2023).
They argued that massive density fluctuations at the quantum level (as particles move randomly) in the early universe caused more mass to be compressed in certain areas, allowing PBHs to form. As the universe expanded and cooled, there was no longer enough energy and density in places for the matter to compress and collapse under its gravity to produce any more PBHs[4]. The existence of galaxies today implies that the early universe must have been inhomogeneous, proving the plausibility of this theory[4].
The development of cosmic inflation theory in the early 1980s proved the existence of quantum fluctuations in the density of the early universe[4] and proposed a period of rapid, exponential expansion of the universe, faster than the speed of light[5]. This solves many theoretical problems in our understanding of the universe:
Homogeneity: the universe looks the same in every direction (uniform/homogeneous), posited by Einstein's cosmological principle[6]. The cosmic microwave background (CMB) also suggests homogeneity by showing temperature fluctuations by less than 0.0001 kelvin throughout the universe[7].
Inflation solves this as the quantum fluctuations at the beginning of the universe became extremely small/diluted relative to the size of the universe as it expanded[6].
Flatness: the flatness problem states that the universe and space-time seem flat/3 dimensional from our perspective instead of curved/4 dimensional. Cosmic inflation suggests that the universe's curvature expanded to near flatness[6].
Although PBHs can exist, there is no evidence that they do. However, studies have shown that the existence of these black holes would answer many of the questions in astrophysics.
D a r k m a t t e r
PBHs could be the source of dark matter[8]. Dark matter has properties that are inconsistent with anything in the existing standard model of particle physics - and PBHs weren't formed from baryonic matter, making them good candidates for dark matter[2][9].
Supermassive black holes are millions or billions of solar masses and are too massive to be created by the collapse of one or several exploding stars. One theory is that PBHs created in the first second of our universe have been absorbing matter and growing, acting as seeds of supermassive black holes, but they can't explain the sheer number of supermassive black holes[8].
E x c e s s i n f r a r e d r a d i a t i o n
Astronomers have detected excess infrared radiation from various dim, distant sources across the universe[4]. According to a study at Yale by Priyamvada
Natarajan (professor in astrophysics at Yale) and her colleagues, a certain amount of PBHs with 1.4 solar masses could account for all dark matter in the universe and have provided the gravitational anchors required for the first galaxies to form. Their model also perfectly predicts the growth of PBHs due to the accretion of matter and pro produces the same infrared radiation signature[4].
Microlensing: Light rays from objects behind a PBH will pass it beyond the event horizon, causing light rays to converge (gravitational lensing). Therefore, PBHs would act as a lens and magnify stars, galaxies, and supernovas behind them with similar brightness. Detecting distant supernovas or planets using telescopes/cameras without loss in brightness could be evidence of PBHs[2].
Hawking Radiation: Black holes emit radiation at a rate inversely proportional to their mass. Therefore, lighter PBHs would release radiation at an extreme rate, and in the last moments of their life, they emit a gamma-ray burst/GRB[10]. Detecting lots of hawking radiation or GRBs would prove the existence of PBHs, as recent black holes will evaporate slowly and survive for a long time[2].
Gravitational waves: These are ripples formed in space-time by massive objects accelerating[11]. Gravitational waves can be produced by neutron stars or black holes circling one another as they are pulled in by their gravity, resulting in a kilonova or a merge of black holes.
eleasing ld prove er Space ht detect universe. tational his time PBHs[4].
Regardless of where or how and if we find them, PBHs could help astronomers learn about our universe.. Firstly, the discovery of PBHs could teach astrophysicists the nature of the early universe and explain what happened to produce what we see today[8]. Secondly, PBHs could serve as probes into galaxy evolution[8] since they can help us see older galaxies via microlensing[12]. They are also crucial in high-energy physics (HEP)[8]. HEP explores the nature of space and time, the characteristics of the forces governing the interactions of matter and energy, and the origins and properties of the elementary particles to explain how our universe became what it is today[13].
However, primordial black holes have still not been proven to exist and currently remain one of the great questions in astronomy rather than a tidy answer.
Types of black holes [cited 10 May 2023]. Available from: https://universe.nasa.gov/black-
1. holes/types/#:~:text=Primordial-,Primordial,t imes%20more%20than%20the%20Sun's.
2. Primordial black hole [cited 10 May 2023].
AAvailable from: https://kurzgesagt.fandom.co m/wiki/Primordial black hol e#:~:text=A%20primordial%20 black%20hole%20is,the%20size %20of%20a%20proton.
3. Pam Weintraub. How primordial black holes might explain dark matter, 16 August 2022 [cited 12 May 2023]. Available from: https://aeon.co/essays/h ow-primordial-blackholes-might-explaindark-matter
4. Ian Randall. Concerning primordial black holes, 18 May 2022 [cited 12 May 2023]. Available from:
https://physicsworld.com/a/concerning-primordial-blackholes/#:~:text=Black%20holes%20formed%20much%20later,e ra%2C%20things%20would%20be%20different.
image credits: nasa
5. Britt Griswold, Dr. Edward J. Wollack. Cosmic inflation, 16 April 2010 [cited 12 May 2023]. Available from: https://wmap.gsfc.nasa.gov/universe /bb cosmo infl.html#:~:text=The%2 0Inflation%20Theory%20proposes%2 0a,relatively%20gradually%20through out%20its%20history.
7. Britt Griswold, Dr. Edward J. Wollack. Cosmic fluctuation, 20 August 2014 [cited 13 May 2023]. Available from: https://wmap.gsfc.na sa.gov/universe/bb c osmo fluct.html
9. J.M. Overduin, P.S. Wesson. Dark Matter and Background Light, 10 July 2004, [cited 13 May 2023]. Available from: https://arxiv.org/ abs/astroph/0407207
11. What are Gravitational Waves? [cited 14 May 2023].
Available from: https://www.ligo.c altech.edu/page/w hat-are-gw
Opening the Doors to a Mysterious Reality, 29 June 2020 [cited 13 May 2023]. Available from:
6. Steven Gimbel. The Big Bang Theory: https://www.wondriumdaily.com /the-big-bang-theory-openingthe-doors-to-a-mysteriousreality/#:~:text=Magnetic%20mon opoles%20refer%20to%20the,have %20been%20found%20so%20far.
8. Alison Klesman. What are primordial black holes?
10 July 2019 [cited 13 May 2023]. Available from: https://astronomy.com/news/2019/07/primordialblack-holes
10. Paul Sutter. Do black holes explode? 19 September 2022 [cited 14 May 2023]. Available from: https://www.livescience.com/can-a-blackholeexplode#:~:text=In%20the%20last%20mome nts%20of,high%2Denergy%20radiation%20a nd%20particles.
12. Galaxies, [cited 14 May 2023]. Available from: https://universe.nasa.gov/galaxi es/evolution/
13. High energy physics, [cited 14 May 2023]. Available from: https://physics.illinois.edu/researc h/groups-and-centers/highenergy-physics
Image credits: Nasa
by Arjun VermaThe body is a complex system, much like an engine, which requires continuous maintenance of conditions. Even the most minuscule of variations can have fatal effects. Surprisingly, everyday actions like eating cheese or drinking coffee are putting a burden on our bodies to immediately react and restore conditions to equilibrium.
pH is a measure of the acidity or alkalinity of a substance, and it is determined using a scale ranging from 0 to 14. Foods with higher acidic content generally have lower pHs. For example, dairy foods and meat are known to be acidic due to amino acids which contain sulphur (Adams, no date). Similarly, citrus fruits like lemon, contain citric acid. On the opposite end of the scale, green superfoods such as celery and spinach are alkaline, helping the body restore its natural pH (Lawler et al., 2022).
So how does chemistry determine the pH of a food? pH stands for the ‘potential of hydrogen’ (Walsh, 2019). It is the presence of protons, also known as H+ ions which make something acidic (Science, 2021). When food is metabolised by the body’s digestive system, a chemical residue called ash is formed. This ash interacts with bodily fluids, such as water, resulting in either an acidic or alkaline environment (Lillis, 2018). Naturally, substances like citric acid, hand off protons more readily, to produce a higher concentration of H+ ions in the ash solution (Science, 2021). This, in turn, lowers pH. Whereas spinach releases more OH- ions which shifts the balance towards alkalinity.
The body must maintain a pH between 7.35 and 7.45 to achieve optimal efficiency and preserve physiological health. This delicate equilibrium is achieved by carefully balancing the concentrations of H+ and OH- ions. When the pH falls below 7.35, it enters a state of acidosis, and pHs above 7.45 lead to alkalosis (Adams, no date).
The effect of severe acidosis on the body is detrimental, given that pH is a fundamental factor for creating an environment suitable for enzymes. Extremely high acidity can denature enzymes by changing the shape of their active sites and inhibiting their function. Enzymes are the key to life, so their dysfunction affects almost every reaction in our body.
Acidic foods can also erode our teeth! The enamel that coats and protects your teeth is gradually worn away by acids, leaving them more prone to bacterial infection and cavities. This also extends to the immune system, as bacteria and viruses thrive in acidic environments, so acidosis can lead to a higher susceptibility to fatigue and illness (Adams, no date). Furthermore, severe acidosis can also affect the central nervous system, causing confusion and drowsiness due to a lower pH
22 influencing neuron function and preventing normal brain activity.
image credits: polly on pinterestFortunately, our body has an innate ability to maintain its internal environment, known as homeostasis, meaning these symptoms are only present in extreme circumstances. Some mechanisms involve an acid-base reaction, in which the excess H+ ions are neutralised by alkaline chemicals, often the body’s own minerals. For example, calcium can be leached from the bones to neutralise acid. However, repeated use of calcium ions from bones can lead to osteoporosis, the weakening of their structure (Adams, no date).
People suffering from acidosis often have an elevated breathing rate, as an attempt to regulate pH. Respiratory compensation is when the body breaks down carbonic acid in the blood, into water and carbon dioxide. The CO2 is then exhaled, returning blood pH to normal levels. The kidneys, part of the renal system, play a vital role in maintaining the body’s acid-base balance. They work to remove H+ ions by producing slightly acidic urine, which can then be excreted from the body (Lillis, 2018).
So, what can you do to maintain your body’s balance? Firstly, eliminate unhealthy acidic foods from your diet, including sugar, food additives and processed food. Instead, replace them with healthier acidic options like fresh fruits and beans. It is also crucial that alkaline foods, like vegetables and seeds make up the majority of your diet. Furthermore, incorporating healthy lifestyle choices promotes overall well-being and aids the body’s detoxification process. For instance, exercise encourages the excretion of acidic chemicals from your body in sweat (Walsh, 2019).
B
In conclusion, acidic foods can have various effects all over the body, by disrupting the delicate pH balance which is essential for survival. By being mindful of our diet and understanding the impact of acidic foods, we can support our body’s natural balance and promote overall well-being
i b l i o g r a p h y
Adams, A. (no date) What effect do acidic foods have on the body? | livestrong, LIVESTRONG.COM. Available at: https://www.livestrong.com/article/474880-what-effect-do-acidic-foods-have-on-the-body/ (Accessed: 10 June 2023).
Dietary acids and your teeth (no date) MouthHealthy. Available at: https://www.mouthhealthy.org/all-topics-az/dietary-acids-and-your-teeth (Accessed: 10 June 2023).
Lawler, M. et al. (2022) What is the alkaline diet? review, research, Food List, and more, EverydayHealth.com. Available at: https://www.everydayhealth.com/diet-and-nutrition/diet/comprehensive-review-alkaline-diet-whatit-how-it-works-what-eat/ (Accessed: 10 June 2023).
Lillis, C. (2018) Are acidic foods harmful to health?, Medical News Today. Available at: https://www.medicalnewstoday.com/articles/322557 (Accessed: 10 June 2023).
The pH Scale (2019) Scientists Say: pH. Science News Explorer. Available at: https://www snexplores org/article/scientists-say-ph (Accessed: 10 June 2023)
Science, C (2021) An introduction to the ph-value of foods (& acid/base reactions), FoodCrumbles Available at: https://foodcrumbles com/food-chemistry-basics-acidbase-reactions/ (Accessed: 10 June 2023)
By Lia Warren
While the Cold war is a topic many may associate with history, not science, both chemistry and physics are heavily entangled within it. Though the lower portion of the periodic table is often ignored throughout GCSE and A-Level studies, history does not overlook these elements and instead highlights the dangerous, unpredictable and controversial side of elements 92 to 94.
In late 1940 a group of scientists from the University of Berkeley, California, discovered the first sample of plutonium element 94. Using a 60-inch cyclotron (a machine that can accelerate charged particles to high energies) a sample of uranium, element 92, was bombarded by deuteron to produce plutonium. Deuteron, a nucleus of deuterium (an isotope of hydrogen) containing one proton and one neutron, turns uranium-238 into uranium239. Uranium-239 loses an electron to become neptunium-239, which again loses an electron to become plutonium-239. Plutonium-239 is the main isotope found in weapons-grade plutonium. This isotope is so powerful that the complete detonation of 1kg of it has the power to produce an explosion equivalent to over 10,000 tonnes of chemical explosives. Similarly, one of the isotopes of plutonium’s origin isotope (uranium -238) also has high explosive potential due to its instability allowing it to take part in chain reactions- uranium-235. This chain reaction is known as a fission reaction and was discovered by a group of German scientists in 1938, who unknowingly altered the course of history. A fission reaction occurs when a neutron collides with the nucleus of an isotope and causes it to decay into lighter nuclei whilst simultaneously releasing extremely high amounts of energy along with more neutrons. The neutrons released allow the fission reaction to self-sustain as they go on to collide with more
nuclei. Along with being explosive, elements 92 and 94 are also extremely harmful to humans as their radioactive properties can cause cancer and organ damage, especially when inhaled as they decay to emit alpha particles that get stuck in lung tissue. Therefore, both plutonium and uranium have the potential to produce great damage, which the Manhattan Project saw the culmination of.
24
image credits: historycollection comThe Manhattan Project was a research project of the U.S. government from the years 1942-45 that was responsible for the first atomic bombs. Initially, scientists had to figure out exactly how much plutonium and uranium were needed to successfully create a bomb; if there is too little, then the bomb would not work. However, there was a degree of urgency to get this calculation correct as any delay would mean that WW2 would go on for longer. After many hours of tedious number crunching and lab labour, the critical mass required for an explosion to occur (based on cross-sectional measurements which indicate the probability of a nuclear reaction taking place), as well as the correct tamper materials (which are required to force neutrons back into the core and increase explosion efficiency), were identified. At Los Alamos, this data was use to inform the production of the inner mechanisms for Fat Boy and Little Man, the bombs dropped on Japan on the 6th and 9th of August 1945.
Los Alamos, New Mexico saw the home of Project Y, the highly classified weapons laboratory directed by J. Robert Oppenheimer. The lab was so secret that it did not exist on any map and one mailbox served the entire town! Much of the work done at Los Alamos involved the processing of tamper material for the bomb, the purification of uranium-235 and plutonium, the elements used in each respective bomb, and most importantly, the design of each bomb.
The first bomb dropped, Little Boy, was a uranium bomb that utilised a gun-type design; this worked by firing two masses of uranium-235 at each other to produce a supercritical mass (the mass of a fissile material that is more than enough to sustain a nuclear chain reaction) in a time that is less than the time between the occurrence of spontaneous fissions. In other words, the design works by firing two masses of uranium-235 at each other extremely quickly to produce an explosion. After the supercritical mass is achieved, a chain of neutrons is introduced from the initiator that triggers the start of the chain reaction. The chain reaction continues to occur until eventually so much energy is released that the bomb blows apart. The neutrons released in the chemical reaction travel at a speed that is equivalent to roughly 10 million metres per second. This speed, combined with the distance the neutrons had to travel, indicates that the complete process of the bomb took roughly a microsecond.
The second bomb dropped, Fat Man, was a plutonium bomb that employed an implosion strategy. Initially, they intended to use a similar gun-type design as in Little Boy but soon discovered that this wouldn’t work because, during the assembly of the bomb, neutrons would be emitted from spontaneous fission leading to a premature chain reaction. Thus, there would have been an overall decrease in energy released when the bomb was dropped. Consequently, the scientists changed the design of the bomb to an implosion technique: this involved the compression of the plutonium sphere so the density significantly increased to the point where the mass could go supercritical, hence producing an explosion. Compression was achieved by surrounding the fission material with highly explosive material that, when ignited, caused compressional shockwaves to be transferred to the centre of the bomb or towards the fissile material.
By mid-1945, the first-ever atomic bombs were ready. On July 14th, 1945, Little Boy embarked on its journey to the island of Tinian in the Pacific, despite doubts that the bomb wouldn’t work. Due to the limited amount of weapons-grade uranium available, there was only enough for one bomb to be built. This meant that the scientists were unable to test the bomb, and instead relied on their confidence in the gun design.
However, testing the plutonium bomb was necessary to ensure that the implosion design was effective. 210 miles south of Los Alamos, Oppenheimer picked the ‘Trinity’ site to test their new weapon. Observation bunkers, electrical cables and miles of roads were built in an obscure corner of the Alamogordo Bombing Range to enable scientists to observe and measure the explosion’s symmetry and energy and, by early
At 5:29:45 on July 16th, 1945, The Gadget was detonated, entering humankind into the nuclear age. Following the success of the Trinity Test, Little Boy was dropped on Hiroshima on August 6th and Fat Man was dropped on Nagasaki on August 9th bringing an end to the war in the Pacific but embarking the USA into the Cold War. Decades later, the development of atomic bombs during WW2, has altered the course of history. Throughout the late 1900s, both the USA and USSR were involved in an arms race that saw the creation of the hydrogen bomb in the early 50s and the intercontinental ballistic missile in the late 50s. The introduction of nuclear weapons to modern warfare has shaped global superpowers since the 1940s and the effects of the first atomic bombs, both politically and socially, are still prevalent in society today. Unarguably, the disciplines of both chemistry and physics played a key part in the conclusion of WW2 and the development of the Cold War and history in general.
World Nuclear Society (April 2021) Plutonium. Available at: https://worldnuclear.org/information-library/nuclear-fuel-cycle/fuel-recycling/plutonium.aspx
(Accessed: 6th June 2023)
Grant, V (December 2021) Atomic Number 94. Available at: https://discover.lanl.gov/publications/national-security-science/2021winter/plutonium-timeline/ (Accessed: 6th June 2023)
Royal Society of Chemistry (no date) Plutonium- Element Information. Available at: https://www.rsc.org/periodictable/element/94/plutonium#:~:text=They%20produced%20it%20by%20bombarding,for m%20element%2094%20(plutonium) (Accessed: 6th June 2023)
Atomic Heritage Foundation (June 2014) Science Behind the Atom Bomb. Available at: https://ahf.nuclearmuseum.org/ahf/history/science-behind-atombomb/#:~:text=However%2C%20one%20neutron%20does%20collide,causes%20a%20nucle ar%20chain%20reaction. (Accessed: 6th June 2023)
Orano (no date) All About Plutonium. Available at: https://www.orano.group/en/unpacking-nuclear/all-aboutplutonium#:~:text=The%20answer%20is%20simple%3A%20it,239%20transforms%20into%2 0plutonium%2D239. (Accessed: 6th June 2023)
Atomic Archive (no date) The Manhattan Project: Making the Atomic Bomb. Available at: https://www.atomicarchive.com/history/manhattan-project/p4s29.html (Accessed: 8th June 2023)
Atomic Archive (no date) Little Boy: A Gun Type Bomb. Available at: https://www.atomicarchive.com/science/fission/little-boy.html (Accessed: 8th June 2023)
Atomic Archive (no date) Fat Man: Implosion-Type Bomb. Available at: https://www.atomicarchive.com/science/fission/fat-man.html (Accessed: 8th June 2023)
US Department of Energy (no date) Manhattan Project: The Trinity Test. Available at: https://www.osti.gov/opennet/manhattan-project-history/Events/1945/trinity.htm
(Accessed: 8th June 2023)
Atomic Archive (no date) National Museum of Nuclear Science & History- Gadget. Available at: https://www.atomicarchive.com/media/photographs/nuclearjourneys/nmnsh/nmnsh-21.html (Accessed: 8th June 2023)
Freudenrich, C and Kiger, P.J. (March 2021) How Nuclear Bombs Work. Available at: https://www.atomicarchive.com/media/photographs/nuclear-journeys/nmnsh/nmnsh21.html (Accessed: 10th June 2023)
Atomic Archive (no date) Trinity Test- Jumbo. Available at: https://www.atomicarchive.com/history/trinity/jumbo.html (Accessed: 10th June 2023)
By Katie MeldrumKnowledge on the fundamental building blocks of the universe has been a priority for physicists, an idea that is coveted. In the quest to understand how things have come to be, scientists have made a plethora of remarkable discoveries, of which the “God particle” must rank highly. In 1964, Peter Higgs, Robert Brout and François Englert first theorised this particle, to explain why certain particles have a mass, thus creating the theory of this particle, which is of the Higgs field (a fundamental field that gives mass to other elementary particles, such as quarks). Yet the existence of this essential particle in particle physics was only confirmed 48 years later.
It took nearly 50 years to discover, and nearly 2 years since LHC started highenergy collisions for the Higgs boson’s existence to be confirmed, but why is that? Well, it is due to 2 main reasons: the mass of the Higgs boson (nearly 120 times that of a proton) and the lifespan of the particle (1/10²² seconds). The latter problem provides most difficulties that need to be overcome. The particle can only be proven by being generated in laboratories because it decays into other particles soon in nature. Due to this reason, the Higgs boson must be created by the LHC, not discovered.
For any particles to be produced, energy is required. Knowing Einstein’s mass-energy equivalence equation of E = mc ² , we know that any object with mass has energy (mass can be thought of as a form of energy). So, using the several different particle accelerators in the LHC, scientists were able to collide these particles that had vast quantities of kinetic energy many times, to produce 1 massive particle.
Particle accelerators are necessary in this step. They contain 2 vacuum pipes with a beam of protons contained within each pipe. These proton beams are accelerated, and gain kinetic energy, such that the protons themselves act as the energy-providing medium for the collision between the 2 beams. The energy in both proton beams then is available to ‘create’ mass. Every time there is a collision, a different result will be produced, which is another reason as to why the discovery of the Higgs boson took so long; physicists knew the probability of the Higgs boson being predicted at collisions when the proton beams were travelling at the specific velocities required to create the Higgs boson, but there was no guarantee that the desired particle would be created.
Since the Higgs boson has such a short lifetime, the advanced particle detectors in the LHC cannot detect the particle itself, so instead are aiming to detect what the Higgs boson decays into: 2 photons (a small particle that make up waves of electromagnetic radiation, with no mass or charge). The only issue is that other collisions also produce photons, so the presence of photons itself is not conclusive evidence to show that a Higgs boson is produced.
In order to determine the cause of the photons’ origin, a series of calculations are required. Assuming that the particle is stationary during the collision, the energy of the entire system can be expressed as E = mc2, where m represents the original particle's mass and c denotes the speed of light. This can be replaced by the equation m1c^2 + m2c^2 + Ek1 + Ek2 after the collision. It is important to note that this energy remains constant since the system under consideration is closed. That is to say, the combined energy of each proton’s internal and kinetic energy, which are both travelling in opposite directions at the same speed. However, as photons are thought to be massless, the sum of both of the photons' kinetic energies is all that is present (Ek1 + Ek2). Furthermore, the energy is equally split between the 2 photons, so each photon has the energy ½mc2. This means that the mass of the Higgs has been ‘turned into’ the energies of both photons; we can use this the other way, to find the mass of the particle and check to see if it matches that of the Higgs boson. However, this only applies when the particle in question is stationary; in most instances, the particle is moving, which calls for a lot more complex mathematics to determine if the Higgs’ decay causes these photons. Now it may seem as if the discovery of the Higgs boson is obvious: we only need to examine the collisions, find those that produce 2 photons and calculate whether this satisfies the Higgs boson’s mass. Yet, there is another issue to keep in mind: that the mass of the Higgs boson was originally unknown, so we could not keep testing with the hypothesised mass to see if 2 photons were produced from the Higgs’ decay – there was no original hypothesised mass.
While this does not cover the entire process of the Higgs boson discovery, there are some key major reasons as to why it took so long to discover - initially, it is not so much ‘discovered’ as produced. Even then, once the Higgs boson has been produced, there are many obstacles in the way that prevent us from being 100% sure about the existence of the Higgs boson, or if it is merely another particle.
CERN (2018) The Large Hadron Collider [Cited May 26, 2023], Available at: https://home.cern/science/accelerators/large-hadron-collider
CERN (2020) The Higgs Discovery Explained [Video] [Cited May 28,2023], Available at: https://www.youtube.com/watch?v=so2nCu2Jkbc&list=PLAk9e5KQYEqvdBn fSMsuVPt-qOBhEEv&index=1
CERN (2022) How did we discover the Higgs boson? [Cited May 28, 2023], Available at: https://home.cern/science/physics/higgs-boson/how
C. Sutton (2015) Higgs boson [Cited June 3, 2023], Available at: https://www.britannica.com/science/Higgs-boson
B. Greene (2013) How the Higgs Boson Was Found [Cited June 4, 2023], Available at: https://www.smithsonianmag.com/science-nature/how-the-higgsboson-was-found-4723520/
By Raghav JasujaHave you ever wondered why identical twins have the exact same genetic composition but still look and act slightly different? The answer concerns epigenetics. But what does the term epigenetics actually mean? If we look at the etymology of this word, the Greek root ‘epi’ means upon and the term ‘genetics’ refers to the genome of an organism. Hence, epigenetics is the study of how the environment can cause changes that act upon the expression of the genome, without altering the genetic code itself.
Epigenetics controls the activation of genes through three different machineries: DNA methylation, histone modification, and non-coding RNA. These epigenetic machineries can be affected by environmental factors like smoking, air pollution, electromagnetic radiation, diet, and exposure to metals and organic pollutants. In this article, we will be focusing on the mechanism of DNA methylation and how it affects the development of dental illnesses. DNA methylation involves the addition of methyl groups to cytosine bases in a DNA molecule, usually at CpG sites (where a cytosine nucleotide is followed by a guanine nucleotide). This changes the DNA’s structure, and so prevents RNA Polymerase from binding to the promoter, inhibiting the process of transcription, and, hence repressing a gene.
Now let’s take a look at how epigenetics affects dental illnesses.
image credit: Jeanette Ramos on pinterest
Periodontitis is a disease where the gum becomes inflamed and infected, leading to damage in the soft tissue surrounding the teeth, which can cause pain, tooth loss, increased tooth mobility and gum recession.
Studies of periodontal tissue have shown that the methylation of specific genes is linked to the development of gum disease. For example, increased methylation of the PTGS2 gene has been observed in periodontitis patients, leading to reduced expression of the COX-2 protein. This protein is an enzyme involved in the synthesis of prostaglandins, which play a role in inflammation and immune responses. The repression of the COX-2 protein could cause a decreased inflammatory response in periodontitis, potentially exacerbating the disease.
In addition, the hypermethylation of the tumour necrosis factor (TNF) cytokine causes reduced TNF expression which has been linked to the development of chronic periodontitis. TNF is an important pro-inflammatory cytokine involved in the immune response and so its repression can contribute to the progression of periodontitis.
It is important to note that epigenetic modifications in proinflammatory cytokines have been linked to nutrition, oral bacteria, and smoking, showing how environmental factors can affect the expression of genes. O r a l C a n
Epigenetics is known to play a significant role in the development of cancers. Many studies have found that hypermethylation and histone hypoacetylation of tumour suppressor genes can cause the onset of cancer. This is because it prevents the transcription of tumour suppressor genes, preventing the production of proteins that can repress and regulate the cell cycle, increasing the chance of uncontrolled cell growth. The specific p53 gene which is responsible for inhibiting enzymes in the G1/S phase of interphase, preventing the replication of DNA, has been found to be hypermethylated in human oral squamous cell carcinoma.
In addition, oncogenes are responsible for coding for proteins that stimulate the transition between stages in the cell cycle. Hence, the hypomethylation of oncogenes can lead to excessive cell division, causing the formation of tumours in oral cancer.
In the US, about 36% of the population suffer from dental anxiety. It is a very common fear and can have a large impact on treatment outcomes, especially if the fear is extreme. Recently, there has been evidence to suggest that these fears can originate further and that there is a transgenerational transmission of dental anxiety due to epigenetics (as epigenetic traits are heritable). Could this mean dental fears potentially stem from the brutal history of dentistry, and have been passed on through generations? The link between epigenetics and dental phobia suggests that future treatments for dental anxiety may need to be focused on seeking epigenetic changes. Furthermore, studies of psychotherapy on the effect of stress hormones have shown that methylation of the GR gene (NR3C1) exon F1 promoter, analysed at pretreatment, was able to predict the treatment outcome. This is exciting news for dental practices as it suggests that in the future, epigenetic tests could be developed to predict whether patients with severe dental anxiety and behaviour problems are likely to respond to psychotherapy.
The field of epigenetic research in dentistry is still in its early stages and so there is limited information presently available about the topic. However, this article provides a small glimpse into what this field of science entails. Whilst currently there are no practical uses of epigenetics in dentistry, it is still very interesting to understand how DNA modification can affect the development of dental illnesses as well as behavioural problems. With the ever-growing nature of scientific discoveries, epigenetics could soon be used to assess a patient’s susceptibility to periodontal disease and dental caries or even to screen for enamel defects in the teeth.
Andia DC, de Oliveira NF, Casarin RC, Casati MZ, Line SR, de Souza AP. DNA methylation status of the IL8 gene promoter in aggressive periodontitis. J Periodontol 2010; 81: 1336– 1341.
Ari, Cherukuri and Namasivayam (2016) Epigenetics and Periodontitis: A Contemporary Review, National Library of Medicine. Available at: https://www.ncbi.nlm.nih.gov/pmc/articles/PMC5198474/#:~:text=Epigenetics%20in%20Pe riodontitis,primary%20epigenetic%20mechanisms%20in%20periodontitis. (Accessed: 07 June 2023).
Dentophobia (Fear of Dentists) (2022) Cleveland Clinic. Available at: https://my.clevelandclinic.org/health/diseases/22594-dentophobia-fear-ofdentists#:~:text=How%20common%20are%20dentophobia%20and,in%20females%20than%20i n%20males. n%20males. (Accessed: 06 June 2023).
Eki et al. (2012) Periodontal disease, Centers for Disease Control and Protection. Available at: https://www.cdc.gov/oralhealth/conditions/periodontaldisease.html#:~:text=In%20its%20early%20stage%2C%20called,loosen%20or%20even%20fall%2 0out (Accessed: 05 June 2023).
Epigenetics (n.d) Oxford Languages. Available at: https://languages.oup.com/googledictionary-en/ (Accessed: 05 June 2023).
Genomics & Precision Health (2022) Centres for Disease Control and Prevention. Available at:
https://www.cdc.gov/genomics/disease/epigenetics.htm#:~:text=Epigenetics%20is%20the%20 study%20of,body%20reads%20a%20DNA%20sequence. (Accessed: 07 June 2023).
Hyodo et al. (2022) The mutational spectrum in whole exon of p53 in oral squamous cell carcinoma and its clinical implications, Scientific Reports. Available at: https://www.nature.com/articles/s41598-022-25744-
8#:~:text=Mutations%20in%20p53%20are%20common,squamous%20cell%20carcinoma%20(O SCC). (Accessed: 06 June 2023).
Jurdzinksi, Potempa and Grabiec (2020) Epigenetic regulation of inflammation in periodontitis: cellular mechanisms and therapeutic potential, Biomed Central. Available at: https://clinicalepigeneticsjournal.biomedcentral.com/articles/10.1186/s13148-020-00982-7 (Accessed: 07 June 2023).
Khouly et al. (2020) The Role of DNA Methylation and Histone Modification in Periodontal Disease: A Systematic Review, National Library of Medicine. Available at: https://www.ncbi.nlm.nih.gov/pmc/articles/PMC7503325/ (Accessed: 07 June 2023).
Sokolowski and Boyce (2017) Biology of the Epigenome, Encyclopedia on Early Childhood Development. Available at: https://www.child-encyclopedia.com/epigenetics/accordingexperts/biology-
epigenome#:~:text=The%20word%20’epigenetics’%20consists%20of,code%20for%20proteins% 20(ncRNA). (Accessed: 05 June 2023).
Torano et al. (2016) The Impact of External Factors on the Epigenome: In Utero and over Lifetime, National Library of Medicine. Available at: https://www.ncbi.nlm.nih.gov/pmc/articles/PMC4887632/#:~:text=Epigenetic%20marks%20 can%20be%20affected,capable%20of%20changing%20epigenetic%20status. (Accessed: 08 June 2023).
Williams et al. (2014) Epigenetics: a new frontier in dentistry, Wiley Online Library. Available at: https://onlinelibrary.wiley.com/doi/full/10.1111/adj.12155#:~:text=In%20the%20shorter%20tim e%20frame,dental%20caries%20and%20periodontal%20disease. (Accessed: 08 June 2023).
By Ayesha Khan