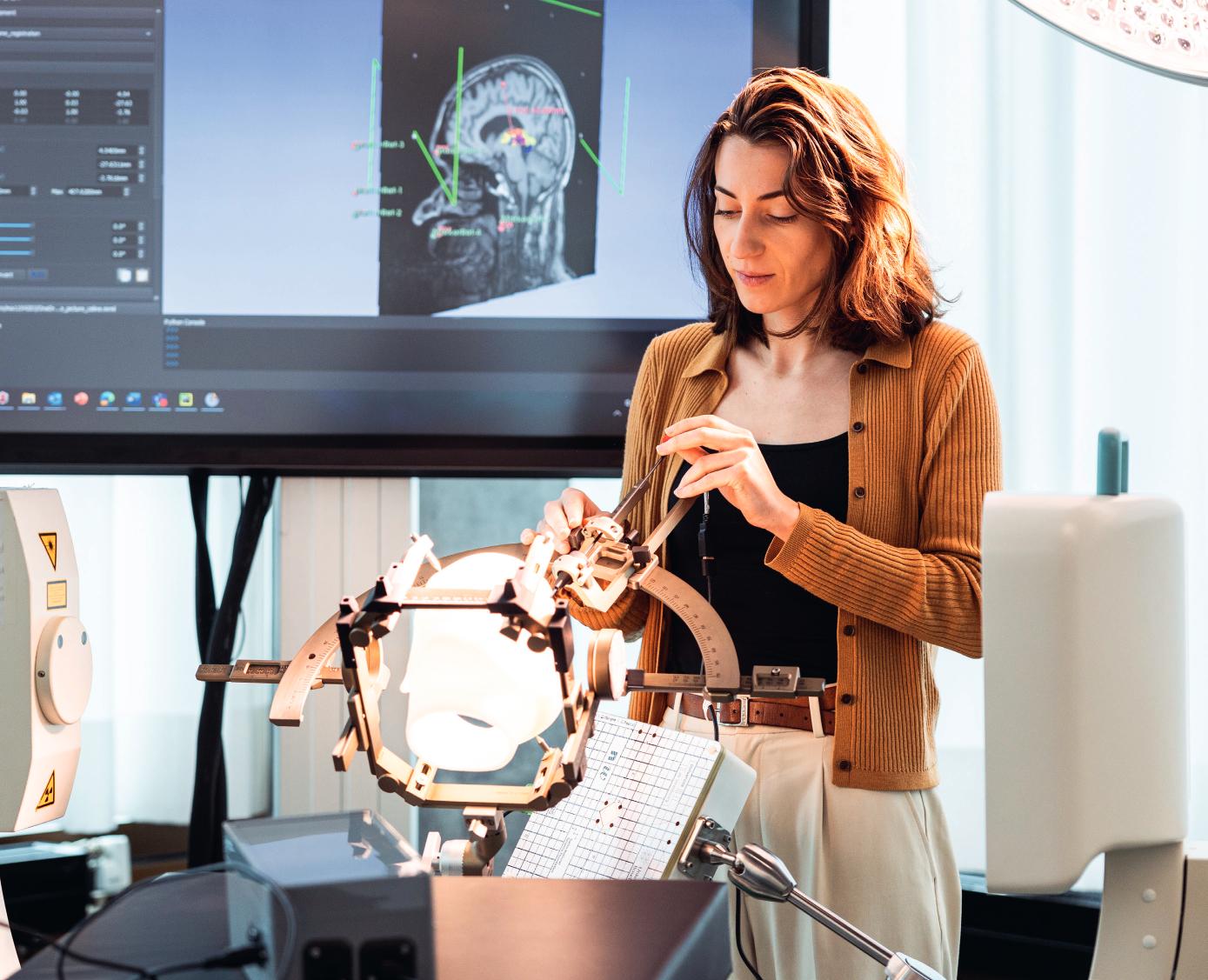
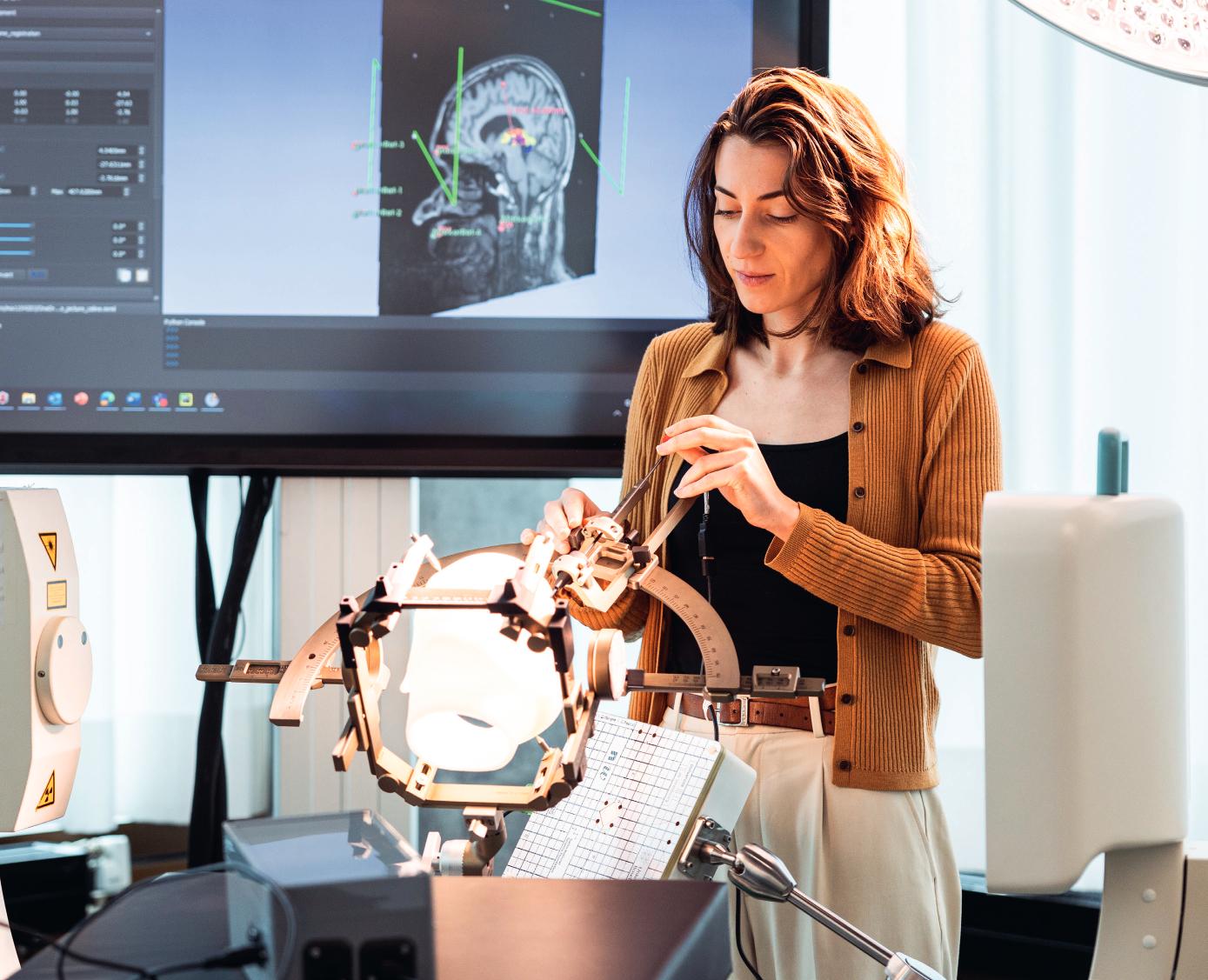
InconversationwithFalkoSchlottig
Since2022,theFHNWSchoolofLifeScienceshasfocused ondeepeningitscorescientificdisciplines,implementingdigital toolsandrenewingitscommitmenttoappliedresearchthatmattersto industryandgovernment.Prof.Dr.FalkoSchlottig,DirectoroftheFHNW SchoolofLifeSciences,commentsonkeyaspectsoftheschool’s strategyandvisionfor2035.
Collaborationisakeydriverofinnovation
Prof.Dr.Schlottig,whatroledoescollaboration playattheFHNWSchoolofLifeSciences?
UniversitiesofAppliedSciencesplayauniqueand importantroleinSwitzerland’seducationand economy.First,wecontributeresearchexpertise andcutting-edgefacilitiestoprojectswiththe privatesectorandgovernmentagencies,helping themaddresschallengesandanswerquestions. Second,thesecollaborativeresearchprojects offerundergraduateandpostgraduatestudents excitingopportunitiestoworkonrealworld situationsandneeds.WeadaptourBScandMSc programmeseachyear,toreflectchangeswesee inindustry.Thesuccessofourschoolisbuiltona dynamicinterplaybetweenresearchandeducation. Workingwithothershelpsusexcel.By selectingtherightpartnerstocollaboratewith, wearemoreinnovativethanwhenweworksolo. Thisphilosophyalsoapplieswithinourschool. Ourfourinstitutesworktogetheroncrossdisciplinaryprojects.Acollaborativeapproachis neededtosolvethechallengesofourtime.
Technologyfocus
Whicheducationalandscientificareashas theFHNWSchoolofLifeSciencesidentifiedas apriority?
Ourschoolismovingforwardindifferentareas. Wealwaysaimtokeeppacewithdevelopmentsin academiaandindustry.
Digitaltechnologiesarenowintegratedintoall ourBScprogrammes.We’reimplementinga combinationoflifesciencesandartificialintelligence,datascience,digitaltwins,automationand roboticsacrossourresearchareas,anddefining howstaffandstudentseffectivelyandethically workwithnewtools.Futurehealthisapriority topicidentifiedbytheFHNWthatourschoolcan makeimportantcontributionsto.
TheFHNWSchoolofLifeSciencesismoving forwardinappliedquantumcomputing.We’veestablishedanewresearchgroupinthisfield,andare workingcloselywithQuantumBaseltobring appliedresearchproblemstothetable seepage42).
Sustainablechemistryisgrowing,witha newBScprogrammeindevelopment.Several ofourresearchprojectscontributetotheFHNW’s zeroemissionpriorityandtoEUprojects.Grassrootseffortsinitiatedbytheschool’sresearchers areunderwaytomakeourlaboperations moresustainable.
It’scriticaltotakeastepforwardinapplying biobasedtechnologiesandothergreenmethods forproducingbasicchemicals.Thisisaneed sharedacrosstheindustrythatwillrequireusto beambitiousandworktogether.
Furthermore,theFHNWSchoolofLife Sciencesisstrengtheningitsactivitiesinbiotech, medtech,medinformatics,pharmatech,cell biologyanddiagnostics.
Internationalisation
WhatistheFHNWSchoolofLifeSciences‘local andinternationalpositioning?
TheFHNWSchoolofLifeSciencesoffersawealth ofinternationalstudyprogrammesandopportunitiestostudents.Beingwellconnectedtothe internationalenvironmentisourresponsibility. Manymultinationalcompaniesareestablishedin Switzerlandanddobusinessaroundtheworld. OurMScprogrammesinparticularneedto preparestudentsforthiscontext.Inparallel, UniversitiesofAppliedSciencesareattheservice oflocalbusinesses,citiesandcantons.Ourstaff andstudentsdevelopmanyvaluablebusiness relationshipsattheregionallevel.
NegotiationsforSwitzerland’sreassociationto HorizonEuropeareprogressingwell.Alreadyin 2024,researchersinSwitzerlandwereableto
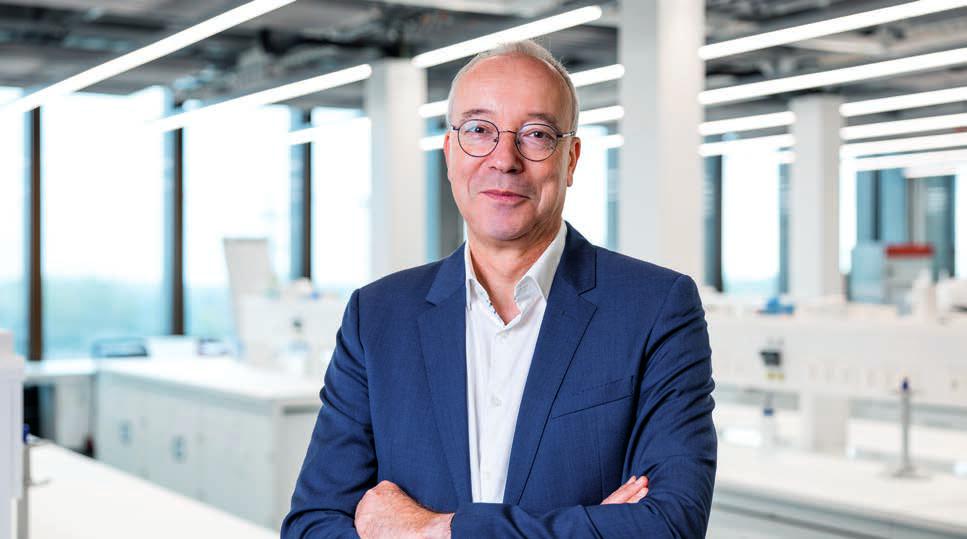
Prof.Dr.FalkoSchlottigleadstheFHNWSchoolofLifeSciences.
participateintheERCAdvancedGrantscallas beneficiaries.In2025,Swissresearcherscan participateinthecallsfortheERCStartingGrant, SynergyGrantandConsolidatorGrant.Theseare allpositivesignalsthatthefullreassociationof SwitzerlandtoHorizonEuropeisaroundthe corner.Inthemeantime,ourresearchershave foundnewwaysofmakingmeaningfulcontributionstoEuropeanresearchprojects,severalof whichareshowcasedinthisbrochure.
Challengesfacingus
Thepandemicfollowedbytwowarshaveledto difficultconditionsaroundtheworld.Howhasthe FHNWSchoolofLifeSciencesfeltthisimpact? Forthemostpart,weareinaveryprivileged positionasamemberofaworld-leadinglife sciencesclusterhereinBasel.Likeothersinthe field,wewereaffectedbychangesinthepriceof energy,chemicalsandgases,aswellasother
strainsonthesupplychain.
Weareconfrontedbyuncertaintiesand changesinindustry,financeandsociety.That’s whyweaimtodevelopcriticalthinkinganda senseofself-responsibilityinourstudents. Listening,askingquestionsinordertopropose relevantsolutionsandnotbeingafraidtomakea change.Recognizingthatourresearchisimportantandhasthepotentialtomakeadifferencein theworld.
Peopleatthecentre
WhatistheFHNWSchoolofLifeSciences‘ visionfor2035?
Inadditiontothecollaborative,scientificand internationalaspectswe’vetalkedabout,akey elementisputtingpeopleatthecentre.It’sour staff’senthusiasmandideasthatdriveusforward. We’vebeenluckytoaddseveralnewteamleaders thatbringindustryexperienceandnetworkswith
“Inourcommunities, withindustry,for patientsandwith researchpeers,the peopleoftheFHNW SchoolofLifeSciences aredrivinginnovation forward.”
FalkoSchlottig
them.Ourgenderandculturaldiversityare increasing,andthisbringsagreaterrangeof perspectivestoourwork.
Harnessingourpotentialmeansempowering staff.Providingfreedomtooperateandtakingthe timetolistentoeachother.Askingourselves honestlywherewecanhavethemostimpactand settingprioritiestogetherhelpsusstayfocused. Asweembracenewworkanditsflexibility,we recognisethevalueofface-to-faceconversations andbeingpresentforourcolleaguesandclients. Inourcommunities,withindustry,forpatientsand withresearchpeers,thepeopleoftheFHNW SchoolofLifeSciencesaredrivinginnovation forward.
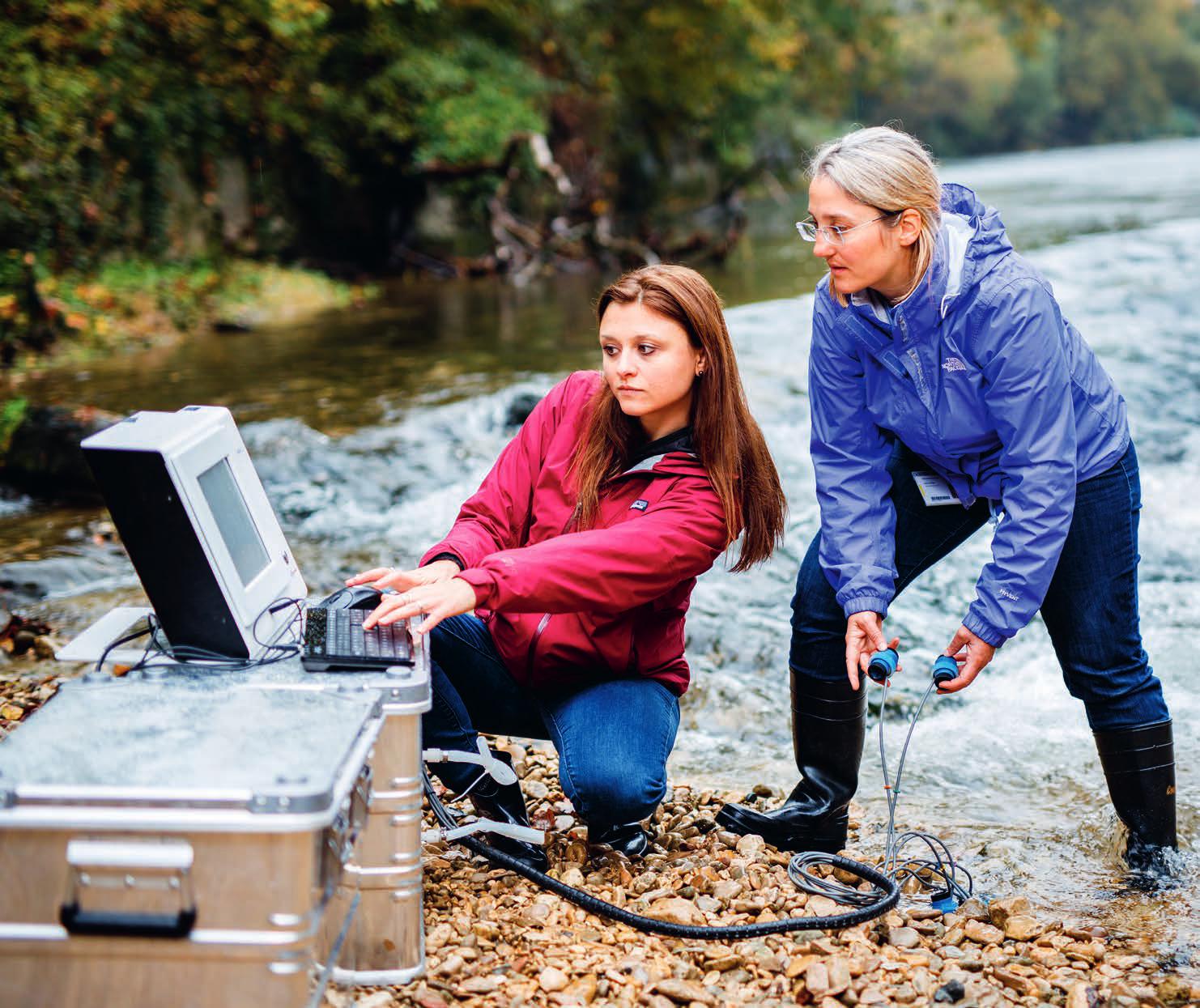
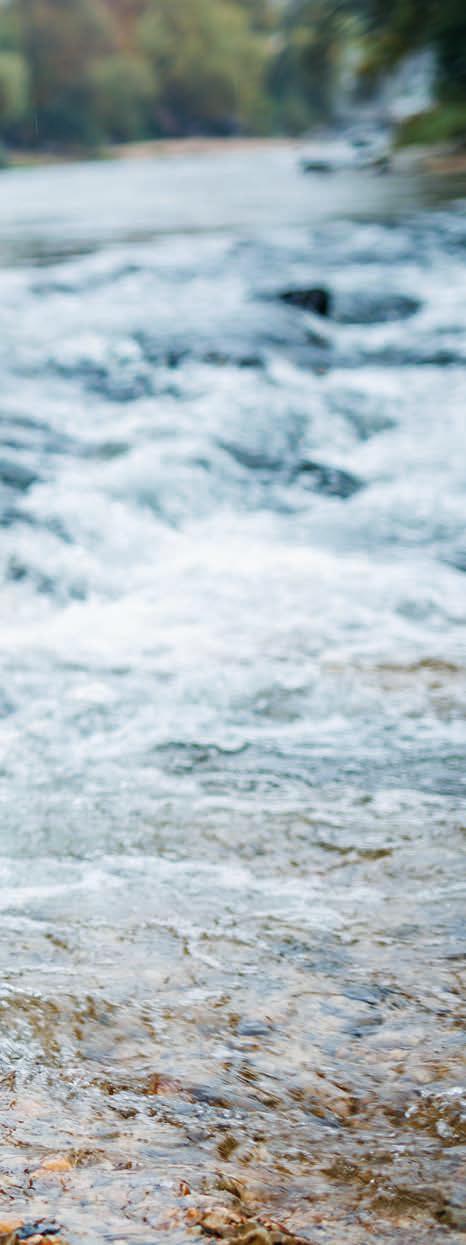
Inour communities
Ourworldisfacingunprecedented environmental,agriculturalandenergy challenges.TheFHNWSchoolof LifeSciencesactivelycontributesto developingsolutionsatthemunicipal, cantonal,nationalandinternational leveltofosterahighqualityoflife inourcommunities.
Waterexpertiseatthe serviceofourcommunities
Accesstocleandrinkingwaterisinequitableworldwide.About2 billionpeopleliveinwater-stressedcountries.Climatechangeand populationgrowthareputtingpressureonanalreadycritical situation.Waterresourcesarealsounderstrainfromtheagriculture industry,whichusesanestimated70%ofallfreshwaterworldwide.
Switzerland’sexpertiseinwaterconservation,managementand treatmentisinternationallyrecognised.Municipalandcantonal governmentsaswellasindustryarecontributingtotechnological advancesinunderstandingwastewaterandoptimizingitstreatment.
AttheInstituteforEcopreneurship,threeresearchgroupsare makingsignificantcontributionstolocal,nationalandglobalneeds forcleanwater.
Minimisingcombinedseweroverflow
MichaelThomann’steamisusingonlinesensors andmodellingtoexploitexistingwastewatercollection andtreatmentinfrastructure.
Incombinedsewersystems,rainwaterandwastewaterfromhouseholdsarecollectedtogether andmaketheirwaytowastewatertreatment plants.Heavyrainfallcancausethesecanalsto overflowbeforethewaterhasbeentreated.
Tocounterthis,Prof.Dr.MichaelThomann’s teamisparticipatingintheEuropeanproject StopUP,whichaimstoreducepollutioninreceivingwaters.Theteamisworkingcloselywith cantonBasel-Landschaft’sOfficeforIndustrial Operations(AIB),whichisresponsibleforsewers, wastewatertreatmentplants(WWTP)andlandfills.
AlthoughAIBmonitorstheamountofstormwaterdischargedfromtheirsewers,littleisknown aboutitsqualityandthusthepollutionload releasedintotheenvironmentduringcombined seweroverflowspills.Sewernetworksandrunoff dynamicsaresocomplexthatrepresentative samplingandlabanalysisarechallengingto implementineverydayoperation.
“Onlinesensorscancollectsufficientdata pointstocapturethedynamicsofevents.We aimtocorrelateparametersmeasuredwithour sensorswithtoxicologicallyrelevantpollutants suchasheavymetalsorcertainorganiccompounds”explainsMichaelThomann.Firstsamplingcampaignsandchemicalanalysesshowed promisingresults.Ecotoxicologytestingis
plannedin2024tofurtherunderstandenvironmentalimpactsofuntreatedwetweatherrunoff.
TheprojectalignswiththeSwissconcept tominimisetheecologicalimpactonreceiving watersthroughintegratedmanagementofthe sewernetworkandWWTP,includingoptimalutilisationofthehydraulicandbiochemicalcapacityof theWWTP.TheWWTPBirsusessequencingbatch reactorstotreatwastewater.Duringdryweather, thesereactorsexecutefullcleaningcycles.The plantswitchesintowetweathermodewhen heavyrainfallisexpected,sothereactorscan partiallytreatagreaterquantityofwater.
“Ourhypothesisisthatit’sprobablybeneficial toperformashortertreatment,evenifthat treatmentisabitlesseffective,thanhaving untreatedwaterspillover”saysMichaelThomann. “Modellingwillhelpusunderstandhowwecould furtherincreasetheinflowtotheWWTPduring rainyweatherwithoutundulycompromising treatmentefficiency.”
“Waterqualityinformationiskeyinanintegrated managementofsewernetworks,wastewater treatmentplantsandreceivingwaters”says GerhardKoch,HeadofTechnologyandDeputy HeadofAIBBirs.“TheFHNWhelpsusunderstand thisaspectbetterandtakeitintoaccountinour plantoperation.”
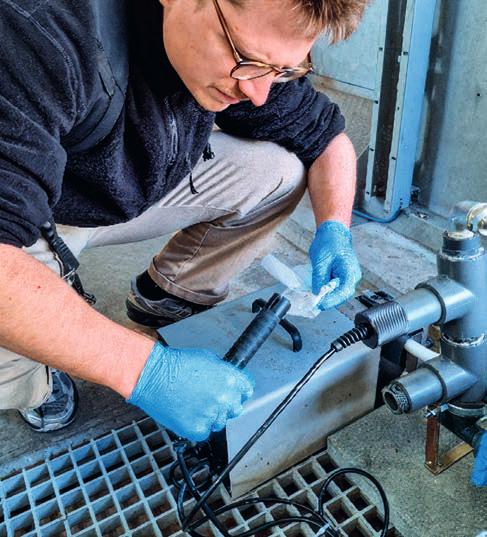
ResearchassociateBartoszKaweckicleanssensorsinstalled atthewastewatertreatmentplantBirs.
Communities: KantonBasel Landschaft;Europe Watersystem: Municipalwastewater Partners: 11partners Funding: EUHorizon101060428,SBFI22.00128
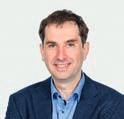
Prof.Dr.MichaelThomann Groupleader,Environmentaland watertechnologies +41612285334 michael.thomann@fhnw.ch
Howwelldoindustrialcompaniesknow theirwastewaters?
AtooldevelopedbyMiriamLanger’steamishelping industrybetteranswerthisquestion.
Themetal,pharmaceutical,chemical,cleaning andwastemanagementindustriesallneed tounderstandtheirwastewaterandcomplywith environmentalnorms.Whilechemicalanalyses canindicatethepresenceandconcentrationof targetedsubstances,theycannotpredictthe responsesoforganismsorcellstoacombination ofthesesubstances.Nordochemicalanalyses revealwhetherunknownsubstances,residues andmetabolitesarepresentiftheyarenot specificallysearchedfor.
Takinginspirationfromgapsidentifiedinan industryworkshop,Prof.Dr.MiriamLangerand ResearchAssociateDr.XeniaKlausfirstcollected nationalandinternationalknowledgeabout industrialwastewaterscreeningwithbioassays. Toanswerthechallengesthatcompaniesface, theycameupwiththeideatocreateapanelof biologicaltests.Theirtool,ABIScreen,combinesa time-efficientbiodegradationtestwithabattery ofrobust,broad-basedbioassays,including toxicitytothebacteriainthewastewatertreatmentplant,andeffectsonluminousbacteria, daphniaandalgae.Ifdesired,anAmes-testcan beincludedtodeterminewhethersubstancesin thewastewaterhavemutagenicpotential.
ABIScreeniscurrentlybeingdeployedinfive Swisscantons.Companiescollectsamplesof
theirwastewaterfromacombinedwaste streamorasub-streamofinterest.Theflasksare pickedupbytheInstituteforEcopreneurship andanalysedinitslabs,withthecostcoveredby theFederalOfficefortheEnvironmentandthe Swisscantonsinvolvedintheproject.Langer’s teamprovidesareadoutofkeyparametersin acomprehensiblereport,whichthecompanycan usetobothtreatandpreventproblems–orin manycases,confirmthesuccessoftheirwastewatertreatmentperformance.
Sincetheprojectbeganin2023,over50 companieshaveparticipated.Theyvaluethe informationtheyreceiveabouttheirwastewater, theconfidentialityoftheprocessandtheability totakemattersintotheirownhands.Somehave requestedfollow-upscreeningofsub-streamsso theycanzeroinonspecificissues.Thecontact betweenmembersofLanger’steamandindustry helpsstimulatedialogue.
“We’reveryhappywithwhatwe’veachieved” saysKlaus.“Companieshaverealisedthat performingbioassaysisnotaslong,complex orexpensiveastheymightthink,anditcomplementschemicalanalysisnicely.Theproject isencouragingthemtomeasuremoreoften andcheckthattheirtreatmentprocesses areworking.”
Industryclientsandgovernmentagencies agree.“Infuture,ABIScreencouldhelpusto identifycriticalsubstancesinourwastewater moreeasilyandeliminatethematsourcewherever possible”saysChristineWegmannfrom dsm-firmenich.
“Thecombinationofdegradabilitytestsand bioassaysinABIScreenenablesaholistic characterisationofaspecificwastewaterin additiontochemicalanalysesandsimultaneous evaluationofthecompany’sownwastewater treatment.Tracingtoxicandnon-biodegradable substancesinwastewaterbacktotheirsource helpscompaniestofindoutabouttherequired state-of-the-arttechniquesandtherefore representsaddedvalue”saysSaskiaZimmermann-Steffens,WaterDivision,FederalOfficefor theEnvironment.
Inthenextphaseoftheproject,Langer’steam willpublishanonymousbiotestresultsina databaseaccessibletoparticipatingcompanies, allowingthemtocomparemetricswithintheir industrysector.Thedatabaseisexpectedtogo livein2025,withadetailedanalysisofcombined resultsby2026.
Asmorecompaniesseevalueinthesetests andthevolumeoftestingincreases,theFHNW SchoolofLifeSciencesplanstotransferthe
knowhowtoprivatesectorlabs,helpingthem expandtheirserviceofferings.
“Thisprojecttrulyillustratesthevalueofthe FHNW’sappliedresearchexpertiseforSwissindustryandgovernment,andisanactivecontributiontofuturegoodwaterquality”saysLanger.
Community: Switzerland(CantonsAG,BL,GE,VD,ZH)
Watersystem: Industrialwastewater Financing/Support: FederalOfficeforthe Environment(FOEN)Switzerland,CantonsofArgau, Basellandschaft,Geneva,VaudandZurich Partners: VerbandSchweizerAbwasser und Gewässerschutzfachleute
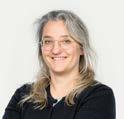
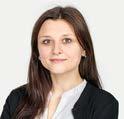
Prof.Dr.MiriamLanger
Groupleader,Ecotoxicology +41612285883 miriam.langer@fhnw.ch
Dr.XeniaKlaus
Scientificassociate,Ecotoxicology +41612285635 xenia.klaus@fhnw.ch
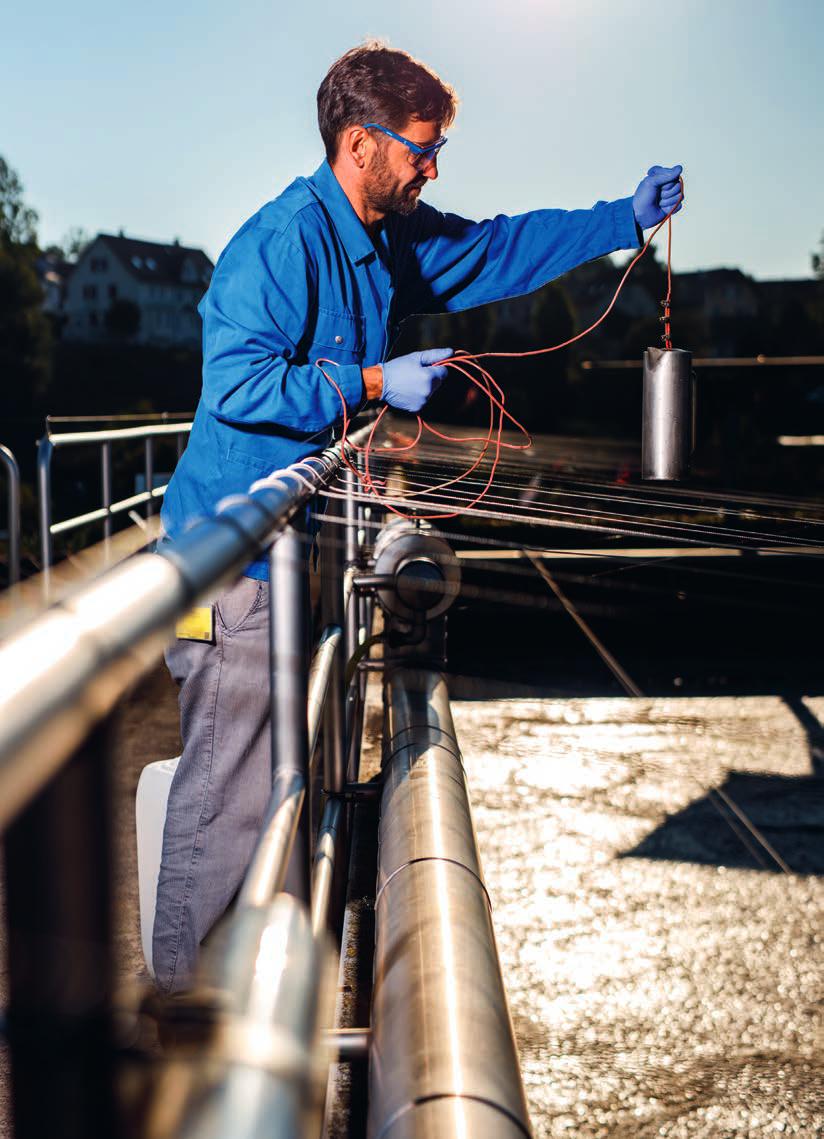
TechnicalspecialistPatrikEckerttakeswastewatersamples fortheFHNWInstituteforEcopreneurship.
Asystemicapproachtosafewater
MarynaPeterandherprojectteamarepreventingdisease throughhandwashingtechnology,behaviouralscience, effectivemanagementandlong-termrelationships
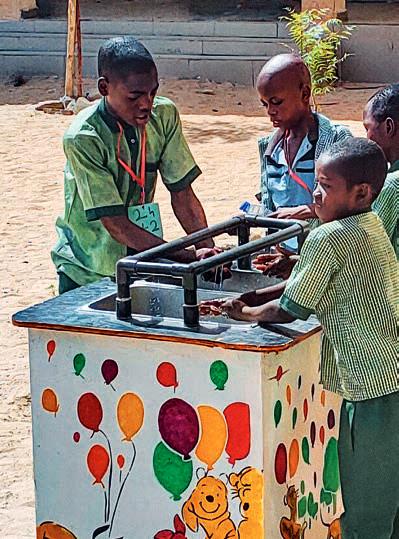
Over45%ofprimaryhealthcarefacilitiesand50% ofschoolsinlow-andmiddle-incomecountriesdo nothaveaccesstohandwashingfacilities,basic hygieneandwatersupplysystemsnecessaryto preventdiseasetransmission.Inresponsetothis challenge,Dr.MarynaPeterandherteamatthe InstituteforEcopreneurshipansweredacallfrom theSwissAgencyforDevelopmentandCooperationwithaprojectcalledHands4Health.Theprojectproposesanapproachtosupportwater,sanitationandhygiene(WASH)systemsthatreduce diarrhoeaamongchildrenatschoolsandprevent transmissionofinfectionsinhealthcarefacilities. Comingfromabackgroundinenvironmental engineering,Peteristheinventorofagravitydrivenmembranetechnologythatfilterswater andremovespathogenicmicroorganisms.Itcan beusedfortreatingdrinkingwater,butalsoto recyclewater.Gravit’eausystemsusethis technologyforhandwashing,reusingthesame
waterforseveralweeksbeforeitisreplaced.By providingasourceofwaterforhandwashingonly, thetechnologyhelpsconservedrinkingwater, whichisimportantduringemergenciesandin water-scarceareas.Peter’snot-for-profitassociationGravit’eauoffersthedesignunderopen accesstocommunities,wholearntoproducethe systemthemselveswiththesupportofassociationmembers.WhenPeterjoinedtheFHNW SchoolofLifeSciences,shenotonlybroughtthis technologicallegacywithher,butalsothevision todeployitusingasystemicapproach.
TheHands4Healthprojectsheleadsjointly withherWASHteamisactiveinMali,BurkinaFaso, NigeriaandPalestine.TheconsortiumiscomposedofresearchersfromtheFHNW,theSwiss TropicalandPublicHealthInstitute,ÉcolePolytechniqueFédéraledeLausanneandthe PalestinianPolytechnicUniversity.Alongside themarenon-governmentalorganisationsTerre
deshommes,CesviandSkatFoundation,aswell asprivatesectorpartnersRanasandMartin Systems.Theteamworkscloselywithlocal governmentsandcommunityrepresentatives, focusingonimprovingwater,sanitationand hygieneservicesatoff-gridschoolsandhealth carefacilitieslocatedinconflict-affectedareas.
Thefirststepisdevelopingasharedunderstandingofthecontext-specificchallenges anddefiningacommongoaltoworktoward. “Eachsituationisdifferent,andit’scrucialto engagelocalstakeholdersattheoutsetsothat wecanprioritisetheneedseffectivelyand sustainablyandunderstandthebarriers”says Peter.“NGOslikeourpartnersTerredeshommes andCesvihavelong-standingrelationshipswith communities,localgovernments,schoolsand healthcarefacilitiesandhelpusbuildtrust.”
Akeypartneroftheproject,Ranas,iscontributingtheirmodelofbehaviourchangeto
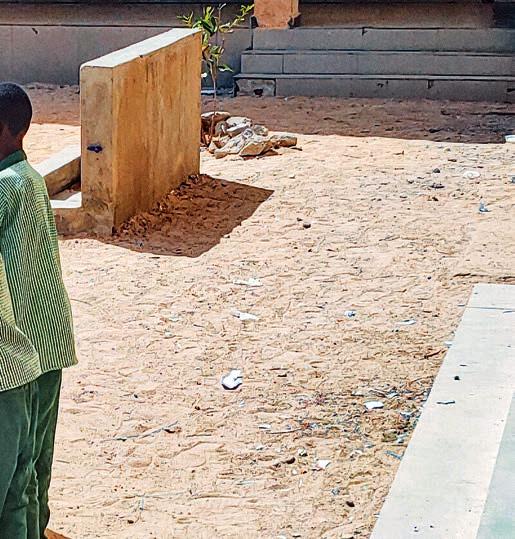
SchoolchildreninaprimaryschoolinMaiduguri,BornoState, NigeriauseaGravit`eauhandwashingsystem.
“Ourgoalisbroadpublic disseminationofasystemic approachtocommunities andNGOs,sotheycantest andimplementittoincrease sustainabilityandimpactof theirWASHprogrammes.”
MarynaPeter
understandmotivatorsforandbarrierstohandwashinginschoolchildrenandhealthcarefacility staff.Factorssuchasriskperceptions,attitudes, normsandvalues,abilitiesandself-regulationare importantdriversofbehaviour.Foreachcountry, behaviouralfactorsthatcanimproveconsistency ofhandwashingpracticeswereidentified.Correspondingbehaviourchangeactivitiescanthenbe selectedfromactivitycataloguesthatare preparedwithintheproject,specificallytargeted totheschools’andhealthcarefacilities’contexts.
Peter’steamiscontributingoverallproject leadershipaswellashardwareinterventions, monitoringtechnologiesandimpactevaluation. Theyareevaluatingsensorsforthehandwashing stationsthatmeasurewaterlevel,andsupporting NGOsandauthoritiesinestablishingeffective monitoringprocessesusingKobo based datacollectionappslinkedtodashboards.Finally, theyoffersimplehow-toguidesforplanning
improvementstowatersystems,maintaining watertanksandchlorinatingwater.
“Thisprojectisnotonlyaboutgettingscientific results”saysPeter.“Ourgoalisbroadpublic disseminationofasystemicapproachtocommunitiesandNGOs,sotheycantestandimplement ittoincreasethesustainabilityandimpactof theirWASHprogrammes.”
Since2021,Hands4Healthhasworkedin26 schoolsand24healthcarefacilities,withadditionalimplementationsplannedin24healthcare facilitiesand26schoolsbytheendof2024, reachingnearly100,000people.
Communities: HealthcarefacilitiesandschoolsinMali, BurkinaFaso,NigeriaandPalestine Waterandsanitationsystems: Recyclingwater forhandwashing;Chlorination;Rehabilitationof infrastructure;Behaviourchange;Digitalisedmonitoring andimpactevaluation
Partners: 10partners
Funding: SwissAgencyforDevelopmentand Cooperation Transform2020
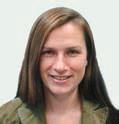
Dr.MarynaPeter Groupleader,Water,sanitation andhygiene(WASH) +41612285792 maryna.peter@fhnw.ch
Letthesunshinein
Ensuringsustainabilityofnew photovoltaicmaterials
Perovskites-Didyouknow?
Perovskiteisamineralcomposedofcalcium titaniumoxide.Itscrystalstructurehasinspired thedevelopmentofaclassofmaterials,also calledperovskites,whichfeaturetwopositively chargedionsandthreenegativelychargedions.
Perovskitesolarcells(PSCs containcompounds withtheperovskitestructure,mostoftenmethylammoniumleadhalides.Theyofferahighabsorptioncoefficientcoupledwithsimplicityandlow productioncost.IncorporationofcarbonelectrodesinPSCscanfurtherimprovetheirsustainability,usingfewercriticalrawmaterials.However, therearealsovoicesexpressingconcernsabout environmentalcompatibilityduetotheirlead content,whichislowbutpresent.
Perovskitesolarcellscanbeprintedasthin, flexiblefilmsforapplicationsinbuildings,vehicles andelectronicdevices.Intandemperovskitecells, aperovskitecellislayeredontopofatraditional siliconcell,whichsignificantlyboostsitsenergy conversion.PSCscanalsobeusedalonetopower smallvehiclessuchasdrones.Assolarpanelsize, locationandenergyneedsvary,PSCscanprovide newoptionstohelpdiversifythesolarmarket andmeetcleanenergytargets.
Netzeroby2050 thisgoalisattheheartofthe EU’sGreenDeal.Toachieveit,thedeploymentof solarphotovoltaictechnologiesmustbescaledup exponentially.Newmaterialsofferaleapforward inthecapacityofsolarpanelstoabsorband convertenergy,butit’simportantnottolosesight ofsustainabilityconsiderationsrelatingtotheir production,useandendoflife.
Dr.MarkusLenzandhisteamattheInstitute forEcopreneurshipareleadingthechargeininvestigatingthesustainabilityofnewphotovoltaic materials.Specializinginthecirculareconomyof metalsandminerals,theyareparticipatingintwo EUHorizonprojects,namelyNexusandPearl, toexamineifperovskitescanbesafelyusedinthe nextgenerationofsolarpanels.Inparallel,they areworkingonrecyclingperovskitessothatthey willnotbecomeawasteprobleminthefuture.
Perovskitecellscontainminimalamountsof lead,withaprotectivelayerofglassorplastic designedtocontainit.Lenz’steamisconducting outdoorleachingteststodetermineifthecellsare hermetic.Todothis,theyinstalledseveral perovskitepanelsontheroofoftheFHNWSchool ofLifeSciencesinMuttenzthatareexposedto real-lifeweatherconditions.Temperature,light, moistureandwindvarythroughouttheyear,and thepanelsaresubjecttomoreextremeweather
eventssuchashail.Metalquantificationtests performedonrainwatersamplescollectedfrom thepanelshaveshownverylowpredicted environmentalconcentrations,comparableto thosepermittedindrinkingwater.Withinthe Nexusproject,furtherfacilitiesarenowbeing installedinBolzanoandValenciatoensurethat perovskitescanbesafelyoperatedunder differentclimateconditions.“Wecurrentlysee littlereasontobeconcernedaboutthepossible environmentalimpactsofleadduringtheuse phase”saysLenz.
Butwhatifthecellweretobedamaged?To findout,Lenz’steamsimulatesmoreextreme weathereventsinacontrolledenvironment.Inthe Pearlproject,theyexposeperovskitemodulesto higherUVradiation,createdefects holes usinga laser,andlookatwhetherbacteriacoulddamage protectiveplasticlayers.
Andwhenthemodulesreachtheirendof life?Recoveryandrecyclingofcriticalmineralsis keytosolidifyinganindependentEuropean supplychainandensuringsustainabilityofsolar andbatterypower.Usingtheirexpertisein hydrometallicrecovery,Lenz’steamhasdemonstratedforthefirsttimehowwateralone issufficienttorecoverpureleadiodide(PbI2) fromperovskites.
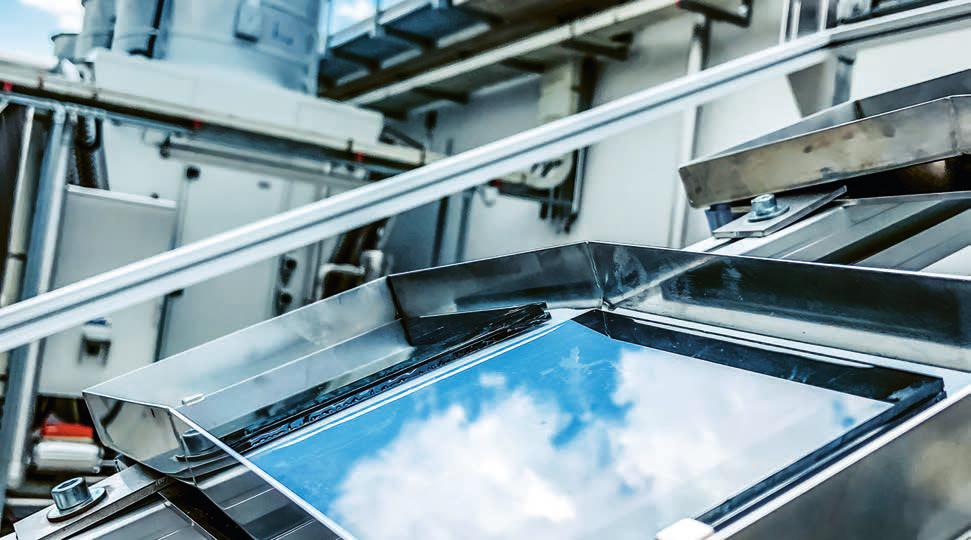
Lenz‘steamconductsoutdoorleachingteststoassessthesafetyofperovskite photovoltaicpanelsinstalledontheroofoftheFHNWCampusinMuttenz.
Therecoveredleadiodidecanbeusedto producenewperovskitematerials,whiletheold cellsarenolongerconsideredhazardouswaste,a win-winsituation.
InnovativesolarfirmsparticipatingintheNexus andPearlprojectsaregratefulfortheenvironmentalfatetestingandmaterialrecyclingdone byLenz’steam.
OxfordPV,whosetaworldrecordinenergy conversionof28.6%fortheircommercialsizedperovskite-on-silicontandemsolarcellin January2024,isworkingtowardatargetof>30% formodulepowerconversionefficiencyinthe Nexusproject.
SauleTechnologies,whoisparticipatingin thePearlproject,specialisesinprintingperovskite solarcellsonthin,flexiblesubstratesatlow temperatures.
“Inthepastdecade,perovskitePVhasgone fromalabcuriositytoapowerhousewiththe
potentialtodemocratisecleanenergy.AtSaule Technologies,werecognisetheurgencyofthe climatecrisisandarecommittedtoharnessing thistechnologyresponsibly,minimisingenvironmentalimpactsateverystageofdevelopment andproduction.ThroughthePEARLprojectwe aregainingcrucialinsights,ensuringasustainable futureforthecleanenergycomingfromour perovskitePVtechnology”saysDr.Konrad Wojciechowski,ChiefScienceOfficeratSaule.
“Ourroleisneithertoengageingreenwashing, nortogeneratealarmism”saysLenz.“Inthese projects,we’reprovidingscientificfactsthatcan helpmanagepossiblerisksofperovskites,determinetheirenvironmentalandsocialacceptabilityandfacilitatetheiradoption.Wealso hopeourmethodscontributetoscaling-upand broadlydeployingrecyclingprocessesfor criticalminerals.”
“We’reprovidingscientific factsthatcanhelpmanage possiblerisksofperovskites, determinetheirenvironmental andsociallyacceptability andfacilitatetheiradoption.”
MarkusLenz
Community: Europe
Financing/Support:
Nexus:HorizonEurope(GA101075330)andSERI (22.00314)
Pearl:HorizonEurope(GA101122283)andSERI (23.00383)
Partners:
Nexus:12partnersincludingOxfordPV Pearl:10partnersincludingSaule
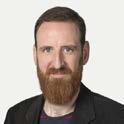
Dr.MarkusLenz
Groupleader,Appliedcircular economy +41612285686 markus.lenz@fhnw.ch
Inthemix
Combiningbiocharandcompostto rehabilitatedegradedsoils
Biochar-Didyouknow?
Biocharisaformofcharcoalobtainedbythermally decomposingbiomassattemperaturesbetween 400and700°Cwithoutoxygen,aprocess knownaspyrolysis.Over2500yearsago,Amazoniansusedasimilarprocessofsmouldering agriculturalwaste,bones,brokenpotteryand otherorganicmattertocreatehighlyfertileTerra preta,whichmeansdarkearthinPortuguese. Today,mostbiocharisproducedfromwoodyand grassybiowastes.
Pyrolysisofbiomassresultsnotonlyintheproductionofsolidbiocharbutalsoinbiofueland syngas.Plantsproducingbiocharmayusethe syngastheygeneratetofuelpyrolysis.
Biocharcanprovidestablecarboninsoilfor hundredstothousandsofyears.Itsporosityhelps soilretainwater,nutrientsandorganicmatter.It canalsoreducenitrousoxide(N2O)emissions duringcomposting,whichhelpscountergreenhousegasemissions.
Ifyouhaveavegetablepatchinyourgardenor flowersonyourbalcony,youknowthebenefitsof usingcomposttomaintainorincreasethequality ofyoursoil.Materialsaddedtosoiltoimprove itschemical,physicaland/orbiologicalproperties arecalledsoilamendments.Onesoilamendment generatingsubstantialinterestisbiochar,aform ofcharcoalobtainedfrombiomass.
IntheAgrocompositprojectconductedas partoftheEuropeanJointProgrammeonAgriculturalSoilManagement(EJPSOIL),scientists fromtheInstituteforEcopreneurshipandAgroscopearecollaboratingwithresearchersand practitionersinNorwayandHungarytocombine biocharwithcompostingofdifferenttypesof biowaste.Theyaretestingthepotentialof compositesoilamendmentstorehabilitate degradedsoils,increasenitrogenuseefficiency alongtheentirevaluechainandreducegreenhousegasemissions.
InSwitzerland,FHNWresearchersarecocompostingbiocharwithanaerobicallydigested greenwasteandtheorganicfractionofmunicipal solidwastefromananaerobicdigestionplant. InNorway,researchersarecombiningaquaculture biowastewithbiochar,whileinHungary,a mixtureofsewagesludgeandbiocharisbeing investigated.
First,theteamisproducingcompositesoil amendmentsfromdifferentbiowastesandwith varyingbiocharconcentrationsinthelab,and testingthesemixturesinpotexperimentson wheatandmaizeplants.Theyaremeasuringthe levelofnutrients,carbon,tracemetalsand polycyclicaromatichydrocarbon(PAH)inthe compositesthemselves,inthedifferenttypesof soilstheyareappliedtoandinthecrops.These resultswillbecomparedtopotsthatcontainno amendments,digestedwasteonly,biocharonly andtraditionalmineralfertilisers.
Next,theteamwillapplyitsfindingsoutdoors inlarge-scalefieldtests,toquantifythepotential ofthecompositesoilamendmentstoimprove degradedsoilsatspecificsites.Theywilldeterminewhichcompositeamendmentshelpenhance soilpropertiestosupportagroecologicalfunctions,includingincreasedcarboncontentand improvedwater-holdingcapacity.
“Fieldtestsarechallenging,withalarge numberofvariablesthatcan’tbefullycontrolled, buttheyareessentialtotranslatinglabresults intoapplication.Weareexcitedtogeneratenew insightsintobiowastevalorisationroutesfor healthieragroecosystemsinEuropethroughthe applicationofbiochar-compostcompositesin real-worldsituations”saysGross.
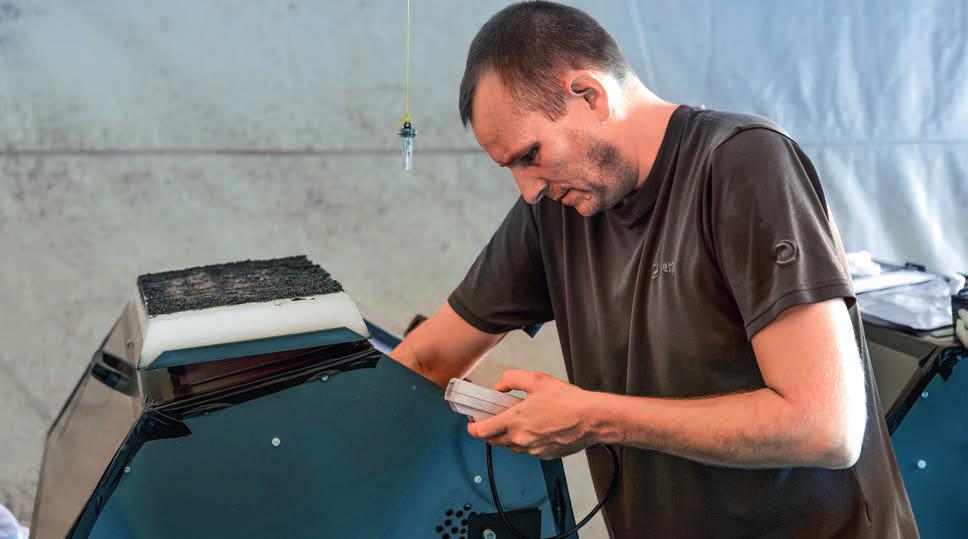
ThomasGrossusesadrumcompostertogenerateanewcompositesoilamendmentthatincludesbiochar, anaerobicallydigestedgreenwasteandtheorganicfractionofmunicipalsolidwaste.
Findingpromisingcompositesoilamendments isnottheonlyaim.Theprojectcollaborateswith severalfull-scalebiowastetreatmentfacilitiesand farmsinSwitzerlandandEuropetoexplore environmentallyandeconomicallysoundbiowaste valorisation.Toquantifytheenvironmental footprint,Gross’teamisconductingsubstance flowandlifecycleanalysistocalculateenvironmentalimpactsfromresourceuseandemissions alongthebiowastevaluechain.Combinedwith ecotoxicologicalassessmentsconductedbyother consortiumpartners,thiswillprovideasolidbasis todetermineiftherearerisksforsoilhealthand cropsafety,andwhethertheadditionofbiochar tocompostisjustifiedintermsofyield,environmentalimpactsandeconomiccosts.
Agriculturalandenvironmentalagencies requirethistypeofdatatoestablishregulations andprovidesoundguidancetofarmers.The SwissFederalOfficeoftheEnvironmentiscalling
formoreknowledgefromresearchbeforebiochar canberecommendedforuseinSwisssoils, particularlywithregardtotheeffectsonsoil organisms.Concernsregardingbiocharhavebeen formulatedinthefollowingfactsheet:Biocharin Swissagriculture-risksandopportunitiesforsoil andclimate[inGerman:Pflanzenkohleinder SchweizerLandwirtschaft RisikenundChancen fürBodenundKlima].
Attheintersectionofbiowastevalorisation, agricultureandbioenergy,thisprojectcanhelp generateknowledgeaboutthesafetyandefficacy ofcombiningbiocharwithcompost,andinthe longrun,contributetodrivingtheSwissbioeconomy.“Ultimately,wehopetohelpcloseresource cycles,reducethedependencyonnon-renewable fertilisersandprovidenewsolutionstorehabilitatedegradedsoils”saysThomasGross.
“Ultimately,wehopeto helpcloseresourcecycles, reducethedependency onnon-renewablefertilisers, andprovidenewsolutions torehabilitatedegradedsoils.” ThomasGross
Community: Switzerland,Europe
Financing/Support: SNSF31SL30_214524
Partners: Agroscope;BudapestUniversityofTechnology andEconomics;NorwegianInstituteofBioeconomy Research;InstituteforSoilSciencesofHungary
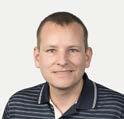
ThomasGross Researchassociate,Sustainable resourcesmanagement +41612285654 thomas.gross@fhnw.ch
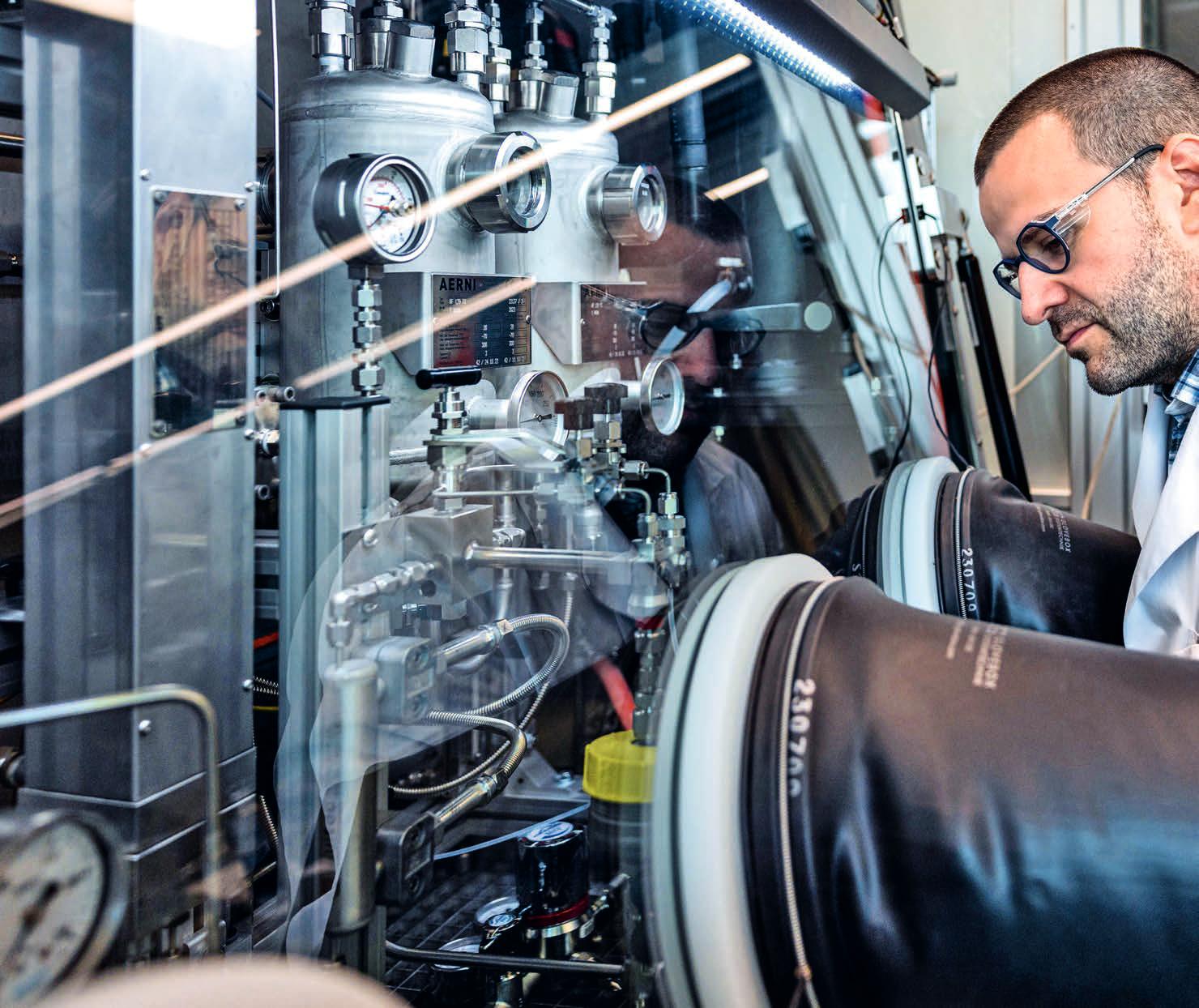
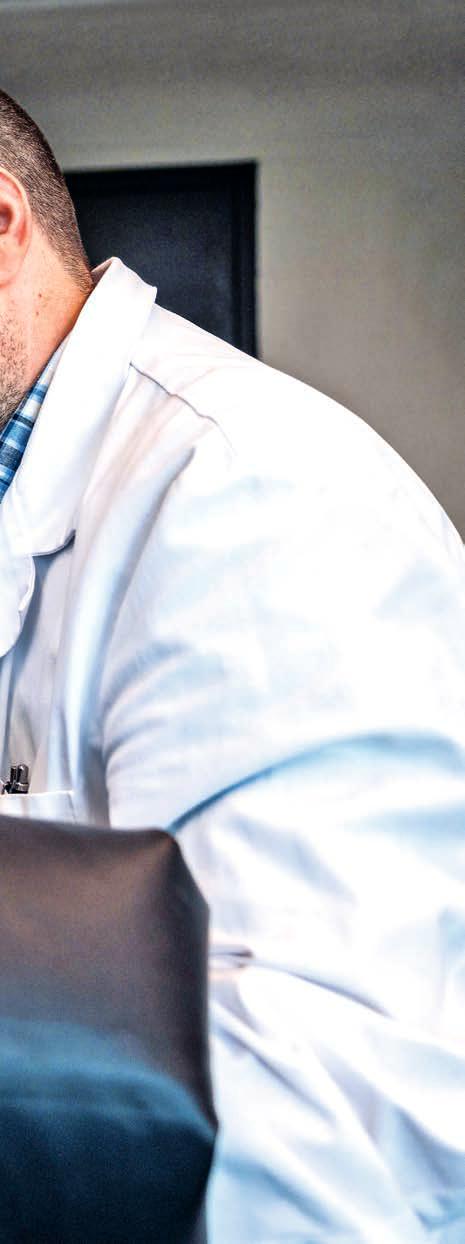
Trustedpartner forindustry
Swisscompaniesofallsizesseekus outasatrustedpartnerforapplied research.WithakeyroleintheBasel cluster,ourcompetencesandstateof-the-artfacilitiesareanassetforboth bigpharmaandSMEs.Innosuisse projectfinancingisavailableforfirms whocollaboratewithustobringtheir ideastowardthemarket.
Biologics–anewgeneration ofmedicinesthatrequire newsolutions
Biologicshaveestablishedtheirplaceasanewgeneration ofmedicinesthatprovidemoretargetedtreatmentoptions forcancer,autoimmunediseases,metabolicdiseases andgeneticdisorders.
Today,biologicmodalitiessuchasantibody-drugconjugates, bispecificproteinsandcellandgenetherapieshaveopened upevenmoreavenues,yettheypresentuniquechallenges inbiopharmaceuticalproduction.Meanwhile,someestablished biologicsaremovingfromclinicaltohomesettings,where patientscanadministerthemthemselves.Thiscreatesnovel considerationsforbiologicsstorage,injectionandalternative deliveryroutes.
TwoteamsattheInstituteforPharmaTechnologyandBiotechnologyarecollaboratingwithindustrytofindsolutionsto newchallengesacrosstheentirebiomanufacturingprocess, fromcelllinedevelopmenttoculture,purification,formulation, fill,finishandqualityassuranceoftechnicalandpreclinical trialmaterial.
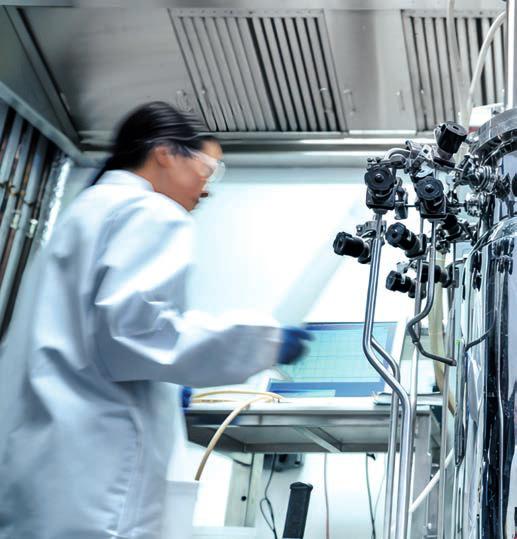
Cellculture
MammalianhostcelllinessuchasChineseHamster Ovary(CHO)orHumanEmbryonicKidney(HEK) cellsactaslivingfactoriestoproducebiologics. Understandingwhatishappeninginsidethe bioreactorduringdifferentstagesofcellcultureis keytoobtaininghighyields.
Ramanspectroscopy,includinganalysers, probesandsoftware,canenablereal-time analysisofnutrients,metabolites,productquality andcellviability,helpingmanufacturersgain controloftheprocessandmakedecisionsabout whentofeedtheculture.However,itswide-scale implementationiscurrentlylimitedbycalibration modeldevelopment,whichrequireslabour-intensiveandtime-consumingexperimentalsetups aswellascomplexITinfrastructure.
InanInnosuisseprojectcalledRamanReady, researchersfromtheInstituteforPharma TechnologyandBiotechnologyaredeveloping
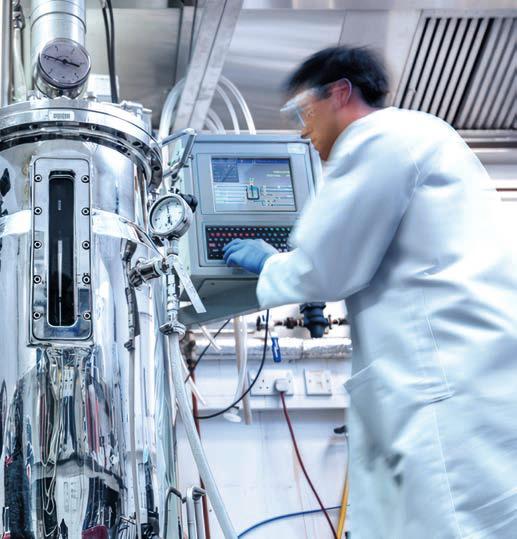
Endress+Hauser,aleadingproviderofRamansystems, isimplementingcalibrationmodelsdevelopedattheInstitute forPharmaTechnologyandBiotechnology.
ready-to-usecalibrationmodelsforRaman spectroscopyinbioprocesses.
“BycombiningRamantechnologywithmachine learningexpertise,weaimtocreateversatile andaccuratemodelsthatareapplicablefora widerangeofbiologicsandprocesses”saysProf. Dr.ThomasVilliger,GroupLeader,Bioprocess Technology.
Theproject’simplementationpartner,Endress+ Hauser,isaleadingproviderofRamansystems whobelievesintheimportanceofdelivering high-qualitysensorstoitsclients.
“Weareconfidentthattheworkwearedoing withtheFHNWwillhelpmakeRamansystemsmore accessibletoourclientsandbroadentheapplicationofthispowerfultechnology”saysLukasJegge, SeniorSalesEngineeratEndress+Hauser.
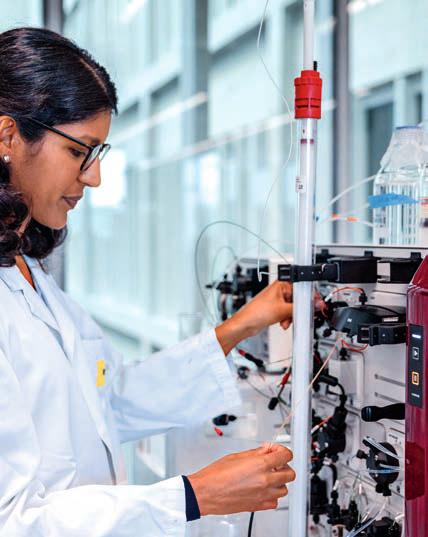
DoctoralresearcherSherinPanikulamperformschromatographytopurifybiologics,andanalyseswhetherhostcell proteinsremainafterpurification.
Purificationofantibodies
Afterproduction,biologicmodalitiessuchas antibodiesproducedfromahostcellneedtobe separatedfromtheculturemediuminwhichthey weregrown.Centrifugation,membranefiltration andproteinaffinitychromatographyarethemost commonstepstocaptureandpurifythetarget biologics.However,smallamountsofprotein impurities,knownashostcellproteins(HCPs,may remainafterchromatographyandcancause concernsforproductquality,safetyand/orefficacy.
Inalarge-scalestudyconductedwithNovartis, SherinPanikulamfromVilliger’steamanalysed 23biologicsafteraffinitychromatography.The goalwastofindoutwhattypesofHCPsremained andunderstandwhytheyhadbeencaptured alongwiththebiologics,tobetterguideprocess development.
Ofthe449HCPsidentified,mostwerecapturedalongwithfewerthan3biologics.However,
someHCPsremainedidentifiedinmanybiologics. Usingproteinnetworkanalysis,Panikulamshowed thatprotein-proteininteractionisanadditional mechanismforHCPco-elution,resultinginfailure toremovethembychromatography.
“Ourfindingsmayhelptoguidehostcellline development,forexampleknockdownorknockout oftheprimarybindingpartnerintheCHOcellline. Wealsohopethatnewpurificationstrategiescan bedevelopedtopreventcertainhost-cellproteins frombringingtheirfriendstothepartyandbeing capturedwithtargetbiologics”saysPanikulam.
“Nowadays,weneedabetterknowledgeof residualimpuritiesremaininginbiologics.These resultsarekeytounderstandinghowHCPs behaveduringthepurificationprocessofadrug, andsupportprocessdevelopmentincaseaproblematicHCPisspotted”saysNicolasLebesgue, PrincipalScientistinAnalyticalCharacterization atNovartis.
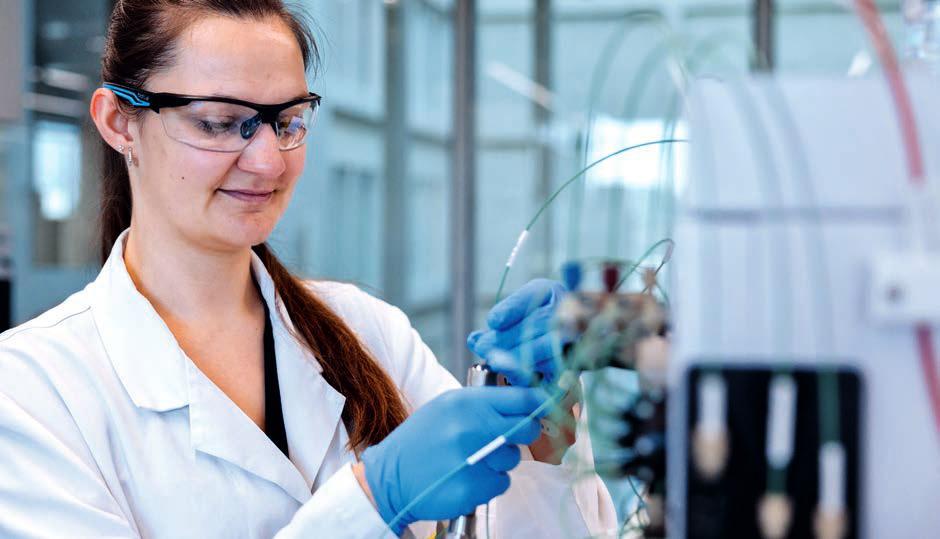
DoctoralresearcherJuliaMüllerdevelopsnewcontinuouspurificationstrategiesforviralvectorsusedingenetherapy.
Purificationofgenetherapeutics
Ingenetherapy,arepairDNAsequenceis packagedinsideaviralvector,alsocalleda capsid,thatdeliverstheDNAtothenucleusofa cell.Manufacturinggenetherapeuticsiscomplex andtimeconsuming,asthetraditionalproduction processrequiresthreeplasmids,growthofhost HEKcells,introductionofthethreeplasmidsinto HEKcells,productionbytheHEKcellsofviral capsidsthatcontainthetherapeuticDNAsequence,andseparationofcapsidsfromthe materialusedtoproducethem.
Notallviralvectorsproducedcontainthe therapeuticDNA.AfterproductioninHEKcells, about50-80%ofcapsidsareempty.Captureand polishingchromatographystepsareusedto obtain80-90%fullcapsidsandtoensuretherapeuticefficacy.Purificationprocessesforviral vectorsarenotaswellestablishedorstandardisedasthoseforantibodies,especiallyat
largerscale.Thisisacriticalbottleneckto makinggenetherapiesmoreaffordable andavailable.
InaprojectwithYMC,aleadingdeveloper ofchromatographicstationaryphasesand purificationequipment,JuliaMüllerfromVilliger’s labisworkingoncontinuouspurificationstrategiesforadeno associatedviralvectors(AAVs
“InsteadofcapturingAAVsonasinglecolumn andlosingsomeduringloading,weareusingtwin columnstotransfertheflow-throughfromthefirst columntothesecond,givingAAVsasecond chancetobecaptured”saysMüller.Afterwards, thefirstcolumnisemptiedandreintegratedinto theprocess,pickingupthebatonwhenthe secondcolumnisfull,andsoonuntileverything hasbeenpurified.Continuouspurificationnotonly capturesmoreAAVs italsouseslessmaterial andenergythanbatchpurificationandiseasierto characteriseforqualityassurance.
“Continuouschromatographyoffers greatpromiseforachievinghigher yieldsingenetherapymanufacturing,whileincreasingsustainability andreducingcosts.”
ThomasMüller-Späth,CEO,YMC
Similarly,duringpolishingtoseparatefull andemptyAAVs,thepurestfractionoffullAAVs centrecut isextractedfromthefirstcolumn, whilepartiallypuresidecutscontainingboth emptyandfullAAVsarerecycledonasecond column,resultinginincreasedyieldoffullAAVs withoutcompromisingpurity.
“TheresultsgeneratedbytheFHNWdemonstratethatcontinuouschromatographyoffers greatpromiseforachievinghigheryieldsingene therapymanufacturing,whileincreasingsustainabilityandreducingcosts”saysThomasMüllerSpäth,CEOofYMC.
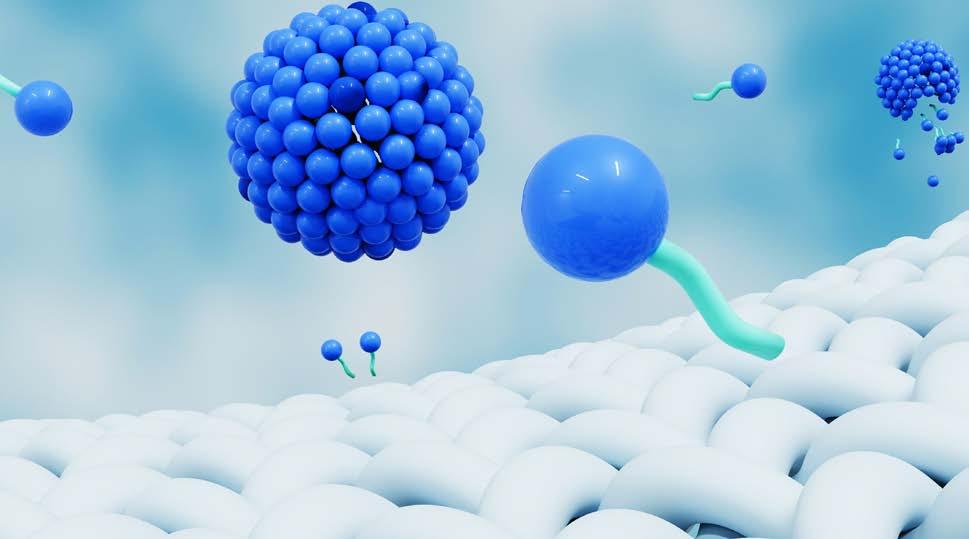
Surfactants,whichhaveahydrophilicheadandhydrophobictail,areusedinbiologicsformulationtopreventaggregation.
Biologicsdrugproductformulation
Biologicscanbecomedestabilisedbyconditions duringtransport,storage,andhandling,whichcan leadtolossofefficacyorunwantedimmune reactions.Withbiologicsadministrationmoving fromthedoctor’sofficetothehome,patientsare becomingpartofthecoldchainandsharing responsibilityforconsistentrefrigerationand properhandlingoftheirbiologics.
Topreventdamagetotheirstructure,biologics areformulatedwithsurfactants,amphiphilic moleculescomposedofahydrophilicheadanda hydrophobictail.Surfactantspreventbiologics aggregationbyblockingtheiradsorptionat air-waterinterfacesencounteredduringmanufacturing,storage,transportanduse.Onlythree surfactantsarecurrentlyusedincommercial biologicsformulation,andtheyfacechallenges whenitcomestodegradation.
Inaprojectconductedincollaborationwith
Novartis,Prof.Dr.OliverGemershausandhisteam helpedassess40newpotentialsurfactants, includingexistingmoleculesandnovelsurfactants synthesisedbyNovartis.Theyfirstexamined biophysicalpropertiesofthecandidates,followed bytheirabilitytopreventagitation-induced aggregationwhenformulatedwithdifferenttypes ofbiologicsincludingadisorderedprotein,a fragmentantigenbindingregion,monoclonal antibodiesandafusionprotein.Mostimportantly, thechemicalandenzymaticdegradationpropensityofthenewsurfactantswasinvestigated.
Theteamidentified2finaliststhatoffered comparableorbetterbiologicsstabilisationand significantlylesssurfactantdegradationcomparedtoexistingformulations.
“ItwascrucialtohavetheexpertiseofFNHWat ourdisposalwhenselectingtheabovealternative surfactants”saysKarolineBechtold Peters, DirectorScience&TechnologyatNovartis.
“Wewillcontinuetoexplorenewsurfactants together.”
CapabilitiesattheInstituteforPharmaTechnologyandBiotechnologyhavebeenbeneficialin twootherprojectswithNovartis:Microdownscale modelstoevaluatethefreeze-thawstabilityof biologicalagents;aswellasPhysicalassessment andmodelstoevaluatethesiliconeoildepletion activityofbiologicalagents.Bothareessentialfor productsinpre-filledsyringes.
“Earlydownscalemodelsandsimulationsenable speedtofirst-in-humanandhelpaccelerate thedevelopmenttimeline”saysBechtold-Peters.
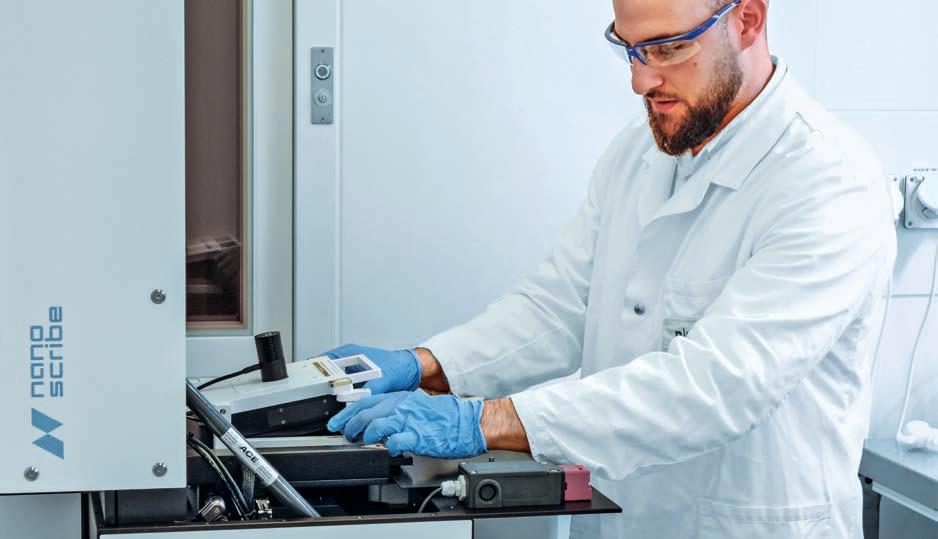
PhDstudentIliasAmaraproducesartificialreferenceparticlesusingtwophotopolymerisation.
Particlecharacterisation
Afterbiologicshavebeenproduced,purifiedand formulated,theyundergoqualityassuranceto ensuretheymeetestablishedstandardsforinjectabledrugformulations,includinglimitsofvisible andsubvisibleparticles.
Particlestypicallyrangefromnanometers tohundredsofmicrometers.Theymaybe extrinsictothenormalmanufacturingprocess,for examplecellulosefibersfromcleaningprocesses. Theycanalsobeintrinsic,havingemanatedfrom normalmanufacturingprocessesorthecontainer closuresystem,ortheymaybeinherent,originatingfromformulationexcipientsortheactive ingredient,forexampleexpectedaggregates orhazes.
Calibrationofinstrumentsusedforsizingand countingsuchparticlesisdonewithperfectly sphericalreferenceparticlesthatofferhigh opticalcontrast.Intrinsicproteinparticles,onthe
otherhand,poseachallengeforinstrumentsdue totheirirregularshapeandtranslucentnature.
Toaddressthis,Germershaus’groupfabricatedirregularlyshapedparticlesrepresentativeof subvisibleandvisibleparticles,inacollaboration projectwithRoche.Createdbytwophoto polymerisation(2PP)printing,theseartificial particlesmayserveasnewreferencematerial mimickingthemorphological,opticalandphysical propertiesofproteinparticles.
Intheirstudy,theycomparedthenew referencematerialtocurrentlyusedpolystyrene spheres,standardparticlesfromtheNational InstituteofStandardsandTechnologyandactual proteinparticlesgeneratedbyexposingmonoclonalantibodiestovariousstresses.
“Wearecurrentlyworkingtowardsbroad evaluationofthesuitabilityoftheprotein-like particlestandardswedevelopedwithstakeholdersinthepharmaceuticalindustry,withthe
ultimategoalofharmonisingsubvisibleandvisible proteinparticlecharacterisationacrosslaboratoriesandorganisations”saysGermershaus.
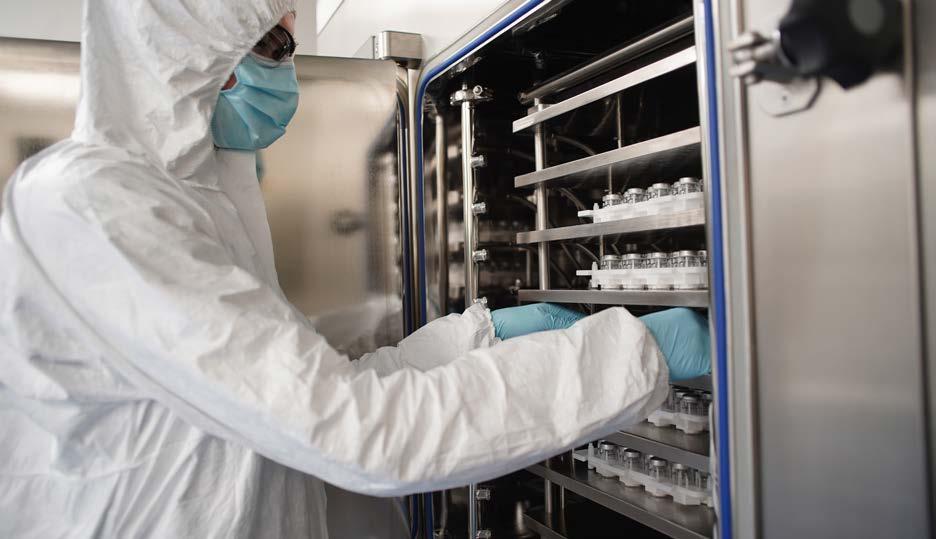
ScientificassistantYasminGretherconductstestsintheFHNW‘sProcessTechnologyCenter, wherecompaniescandevelopnewfillfinishformatsandprotocols.
Packagingandadministration
Oncebiologicshavebeenformulatedandcharacterised,theyundergofillfinish,whichinvolves transferringthebiologicdrugsubstancetoa sterilevialorsyringe,stoppering,inspectionand labelling.Somebiologicsarelyophilisedbefore stopperingtoincreasetheirstability.TheProcess TechnologyCenterattheFHNWCampusMuttenz featuresacleanroomwherecompaniescandevelopnewfillfinishprotocols,fillreferencesamples ormanufacturebiologicsforpreclinicaltrials.
Increasingly,biologicsarebecomingavailable forpatientstoadministerathomeusingpre-filled syringes,autoinjectorsorpatchpumps.These productsofferpatientsconvenienceandautonomy,andaredesignedtohelpovercomeneedle phobiaandincorrectadministration.
Pre-filledsyringescontaintherightdosage, andthepatientcancontroltheinjectionspeed.
Autoinjectorsaresemi-automatic,withabuttonto
starttheinjection,ahiddenneedleprotectedbya safetycoverandaudibleclicksthatindicatewhen theinjectioniscomplete.Patchpumpsandother wearabledevicesarethenewest,mostadvanced optionsforbiologicsself-administration.The latestdevicesfeatureinnovationstoreconstitute lyophilisedbiologicsandenabledeliveryoflarger volumesbysubcutaneousinfusion.
Germershaus’teamiscollaboratingwith companiesfromthepharma,packagingand devicesectorsfocusingonthedevelopmentof newinjectiondevices.Thesubcutaneousdelivery oflargervolumesiscurrentlyanimportanttopic. Studiesrangefrominvestigationofthecompatibilityofdevices e.g.patchpumps withbiologic drugproducts,tofillingofnovelpackaging formats e.g.cartridges underasepticconditions. Fillingcanbeperformedmanuallyorautomatically inbatchsizesfromsingledigitunitnumbersupto 1000unitsperhour.
Financing/Support: RamanReady:Innosuisse114.286IP-LS DevelopmentofaMobileDosingPumpforthe AdministrationofBiologics:25721.1PFLS-LS Partners: Endress+Hauser,Novartis,Roche, YMCChromaCon,SensileMedicalAG
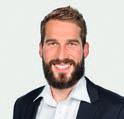
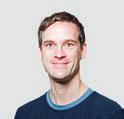
Prof.Dr.ThomasVilliger Groupleader,Bioprocesstechnology +41612285246
thomas.villiger@fhnw.ch
Prof.Dr.OliverGermershaus HeadofInstituteforPharma TechnologyandBiotechnology Groupleader,Formulations anddevicesforactivechemical andbiologicalsubstances +41612285526
oliver.germershaus@fhnw.ch
Overcomingfrontiersin brainmedicine
Ourbrainisprotectedbyalayeroftightlywovenendothelial cellsthatlinethebrain’sbloodvessels,calledtheblood-brain barrier.Thesecellsblockforeignagentssuchaspathogens fromenteringourbody’scommandcentre.Atthesametime, nutrientsthatthebrainneedsareallowedtopass,viaaprocess calledreceptormediatedtranscytosis(RMT).Receptorson thesurfaceofendothelialcellsrecogniseandbindtonutrients, andcarrythemintothebrain.
Thebarrieralsopreventssometherapeuticsfromreaching diseasetargetsinthebrain.Medicinesthatcansuccessfully enterthebrainincludeanesthetics,antidepressantsand antipsychotics.Agreatneedremainshoweverfortherapiesto treatAlzheimer’s,ALS,Parkinson’s,braincancersandmore. Biologicdrugscouldofferhope,butstrategiesmustbedevised tosneaktheselargemoleculespastthebarrierusingRMT.
TeamsattheInstituteofChemistryandBioanalyticsare workingonengineeringnanomedicinesthatcanenterthebrain, aswellasnewmodelsoftheblood-brainbarrierthatcanbe usedbypharmaceuticalcompaniestotestwhether medicinescancross.
Bighopesfornanomolecules
Nano-engineeredshieldforenzymereplacement therapycouldhelpitreachtargetsinthebrain
Enzymesareproteinsthatspeedupchemical reactionsincells.Theybreakdownfoodand toxinsinourdigestivesystemandliver,andhelp DNAunwindduringcelldivisionandreplication forexample.Beyondtheirroleinourbodies,their propertiesascatalystsmaketheminterestingasa greeneralternativetochemicalcatalystsfor chemicalreactions.
In2016,scientistsattheInstituteforChemistryandBioanalyticsdevelopedaninnovative methodtoengineerenzymesinsidenanoparticles, makingthemmoreresilientforapplicationsin biocatalysis.TheyspunoffthecompanyInofea, focusedonprovidingsolutionsforstable,active andpureenzymesforchemicalreactionsand productionprocesses.
Enzymescanalsoactastherapeutics,in caseswhenthebodyisnotproducingenoughon itsown.Buildingontheirenzymeengineering technology,Prof.Dr.PatrickShahgaldianandhis teamdevelopednano-architecturestoprotect sensitiveenzymetherapeuticsfromdegradation inthebody e.g.intestinaltract.Theteam designedanorganic-inorganichybridthatenables enzymestostayaliveandreachtheirtargetsin thegut.Thiscansignificantlyreducethepill burdenforpatients.Forthetherapeuticapplicationsoftheshieldingtechnology,Shahgaldian
spunoffPerseoPharmain2019.Thefirmis currentlyconductingpreclinicaltestingoffour digestivetherapies,aswellasinvestigatingifa similarapproachcouldbeusedtodelivera cocktailofenzymesthatpreventsmetabolic activityincancercells.
ThenextfrontierforShahgaldianandhisteam isanotherdifficultenvironmentformedicines:the brain.Thistime,they’reworkingonsolutionsfor lysosomalstoragediseases(LSD),alargegroup ofmetabolicdisorderscausedbyenzyme deficienciesinthelysosome.About50-70%of LSDsaffectthecentralnervoussystem.Enzyme replacementtherapiescanhelpaddresssome oftheseconditions,butunfortunatelytheydonot crosstheblood-brainbarrier.
Shahgaldian’steamisseekingtoovercome thisproblembydesigninganouterenzymeshield thatwillnotonlyprotecttheenzymes,butmore importantly,bindtoreceptorsontheblood-brain barriertoallowtransportacrossit.
“Bydecoratingtheenzymeswithstructures thatthebrain’sendothelialcellsrecognise andbindto,itmaybepossibletodeliverenzymes tothebrain.Wehopeourapproachwillcontributetonewtreatmentsforlysosomalstorage diseasesaffectingcentralnervoussystem targets”saysShahgaldian.
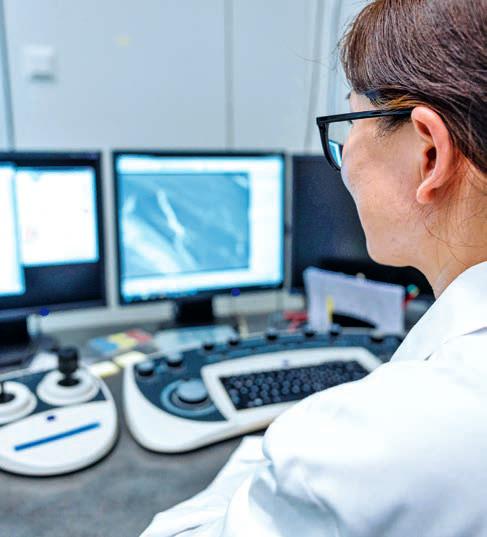
ResearchassociateCongyuWuperformsscanningelectron microscopytocharacterisenanomoleculesdesignedtobind totheblood-brainbarrier.
Anotherstrategythattheteammayexplorein thefutureisthedesignofnanoparticlesforintranasaladministration.Nosetobrainisapromising routethatavoidssomechallengesassociated withtheblood-brainbarrierandforwhichnanomoleculesareparticularlysuited.
Financing/Support: SwissNanoscienceInstitute Partners: PerseoPharma
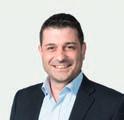
Prof.Dr.PatrickShahgaldian Groupleader,Molecular nanotechnology +41612285487 patrick.shahgaldian@fhnw.ch
Testingbrainmedicinesinthefastlane
New invitro modeloftheblood-brainbarrierhelps pharmaidentifypromisingcandidates
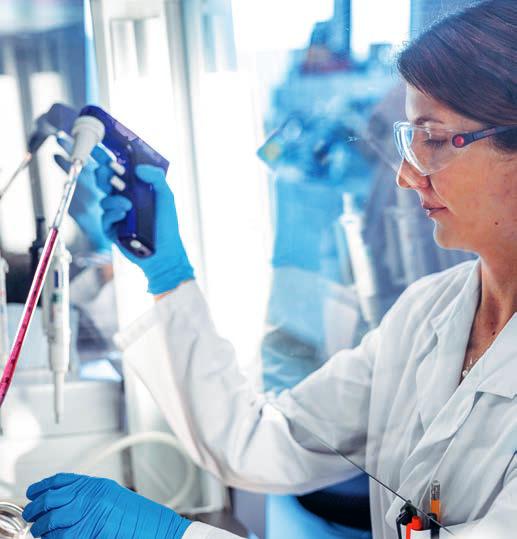
ResearchassociateFlorianaBurgioseedsbrainmicrovascular endothelialcellsinanOrganoPlate 3lane Mimetas tocreate amodeloftheblood-brainbarrier.
Theneedforbraintherapeuticsisgreat,andmany pharmaceuticalcompaniesaredevelopingdrugs. Totestthesenovelmedicines,clinicallyrelevant modelsthatmeasuretheirabilitytocrossthe blood-brainbarrierareneeded.
Invitro modelsarecellsgrowninsideaspecial devicethatmimicshowanorgan,orpartofit, functions.Prof.Dr.LauraSuter-Dick’steamatthe InstituteforChemistryandBioanalyticshasdeep expertiseindevelopinglivermodelstotestthe safetyofnewmedicines,whilereducingand replacingtheneedtouseanimalsforexample.
Backedbythisstrongfoundationinadvanced invitro modelsandnewapproachmethodologies (NAMs,postdoctoralresearcherDr.Floriana BurgiofromSuter-Dick’scellbiologygroup,in collaborationwithscientistsfromUCBPharma Belgium,setouttocreateamodelofthebloodbrainbarrierusinginducedpluripotentstem cells iPSC).Theseimmaturecellscanbedifferen-
tiatedintoanycelltype,makingthemversatile forresearch.
ThemodelimplementedbyDr.Burgiofeatures asinglecelltype:brainmicrovascularendothelial cells(BMEC)derivedfromiPSC.Shegrewthe modelinanOrganoPlate®(Mimetas andconductedteststoconfirmthatitmimicsbarrier tightness.Themodelfeaturesatopchannelwith endothelialcells,amiddlechannelwithextra-cellularmatrix,andanemptybottomchannel.Test moleculesareperfusedintothetopchannel; thosethatbindandpassarecollectedinthe bottomchannel.Thesystemiscompatiblewith automatedfluidhandlingandallowstheevaluationof40chipssimultaneously.
“WhentestedinourBBBmodel,weshowed thatamoleculewhichbindstothetransferrin receptorhasan11-foldincreaseincrossingthe barriercomparedtoacontrolmoleculethatdoes notbind”saysBurgio.“Thisestablishedthatour
modelexhibitsfunctionaltransferrinreceptormediateduptakeandtranscytosis.”
Theprojectwasconductedincollaboration withUCBPharmaBelgium,aleaderinmedicines forneurological,immuneandrarediseases.
“TheFHNW’smodeloftheBBBrepresentsa greatstepforwardtowardstestingtheabilityof potentialdrugcandidatestoenterthebrain.Inthe end,suchamodelcanimprovepreclinicaltesting offuturemedicinesfrombothanefficacybutalso asafetypointofview”saysRainerClass,AssociateDirectorof Invitro ADME,UCBPharma.
“Notonlydidthisprojectenableustodevelop amodelthatUCBPharmaBelgiumcanuseaspart oftheirdrugscreeningprocess;italsoallowedthe FHNWSchoolofLifeSciencestobreaknew groundindesigning invitro modelswithinduced pluripotentstemcells,propelledbythelatest microfluidictechnologies”saysgroupleaderProf. Dr.LauraSuterDick.
“TheFHNW’smodelofthe blood-brainbarrierrepresentsa greatstepforwardtowardstesting theabilityofpotentialdrug candidatestoenterthebrain.”
RainerClass,AssociateDirector ofInvitroADME,UCBPharma.
Partners: UCBPharmaBelgium
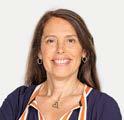
Prof.Dr.LauraSuter-Dick Groupleader,Cellbiologyand invitro toxicology
+41612285659
laura.suterdick@fhnw.ch
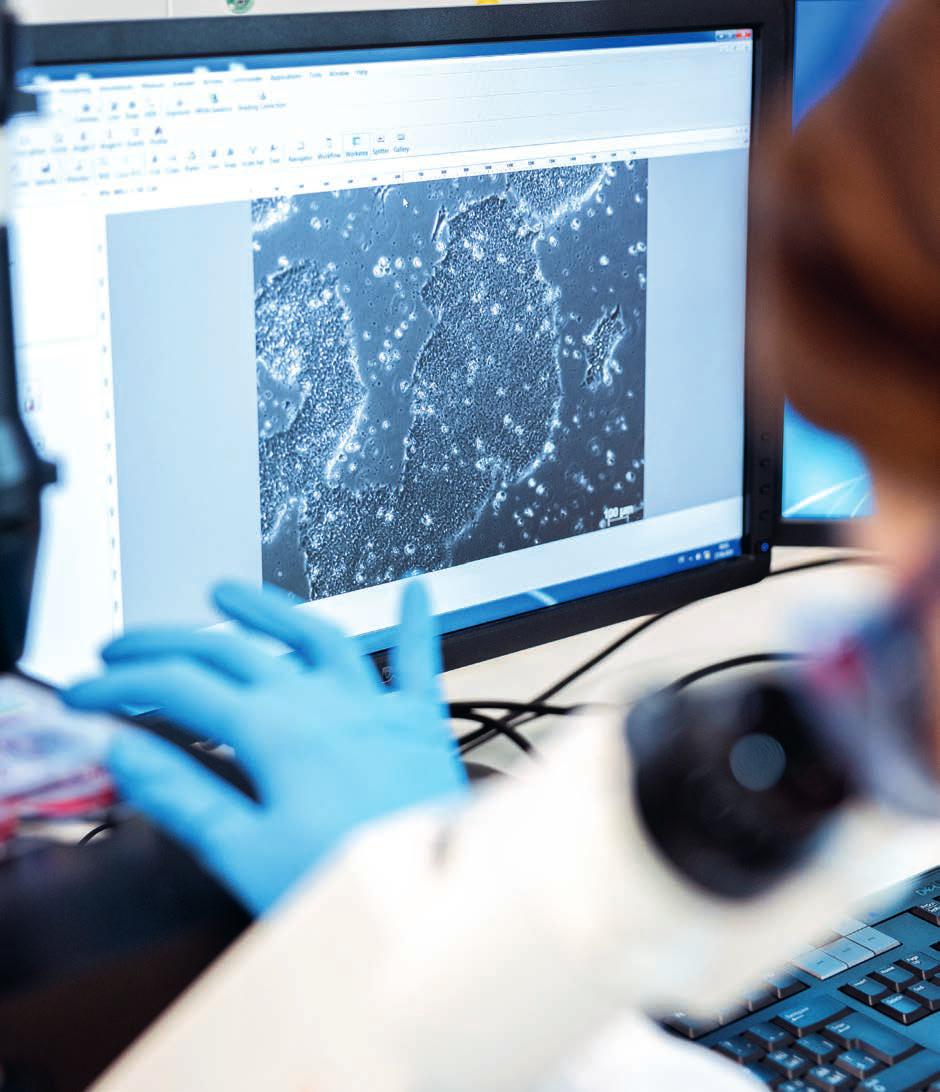
Burgioassessesiftheinducedpluripotentstemcellsaredenseenough tostartdifferentiationintobrainmicrovascularendothelialcells.
Safetyfirst!
AndreasZoggbringschemicalengineeringcompaniesand studentstogethertomakesharedadvancesinprocesssafety
Managingethoxylation–Didyouknow?
Ethoxylationisachemicalreactionofethylene oxidewithanalcohol,acidortriglycerideoil.The reactionisperformedtoproducesurfactants, whichareusedinlaundrydetergents,surface cleaners,cosmetics,agriculturalproducts,textiles andpaint.
Performingethoxylationasacontinuousreaction ismoresustainableandcost-effectivethan runningitasabatchreaction,buttheexplosive natureofethyleneoxiderequiresmasteryof reactionsafety.
Properlymanagingandscalinguphazardous continuousreactionsincludes: reactioncalorimetry:measuringheatreleased fromthereaction,determiningheatandgas removalrequirementstocontrolthereaction. thermaldecomposition:knowingthe temperatureatwhichasubstancechemically decomposes. explosionpressure:measuringthereaction’s pressuretodetermineitsriskofexplosion. pressurerelief:providingamechanisminthe reactortoreleaseexcesspressure.
Prof.Dr.AndreasZogghasapassionforprocess safety.HejoinedtheFHNWSchoolofLife Sciencesin2018afterservingasSafetyexpertat Novartis,andProcessdevelopmentchemistat F.Hoffmann LaRocheandCibaSCforseveral years.Nowheleadstheprocessdevelopment groupattheFHNW’sProcessTechnologyCenter. Heisfounderandpresidentoftheassociation Miniplant4.0.
“Threedriversofinnovationintoday’sSwiss chemicalindustryaresafety,sustainabilityand quality”saysZogg.“Ifyouaskme,safetyalways comesfirst!”
Manychemicalreactionsperformedto produceingredientsfordetergentsandcosmetics poseadangeroffireandexplosion.Transitioning frombatchorsemi-batchreactionstocontinuous flowchemistrycanprovidesafetyadvantages.
Second,ascompanieslooktoreducetheir energyconsumption,theyseetheopportunityto conductsomereactionsincontinuousmodeto avoidcostlyenergypeaks.Thisiscreatinga demandforcontinuousreactorsystemsdeployed atsmaller,decentralisedproductionsites.
Finally,asthepriceofchemicalsmanufacturedinSwitzerlandishigh,theirqualitymustbea differentiatingfactor.Scalingupflowchemistry helpspromotethefinechemicalssectornationally.
Zoggisaddressingallthreeelementsinan InnosuisseprojectcalledSimulation-basedsafety conceptforthescale-upofsafety-related demandingcontinuousprocesses,performedin collaborationwithMScstudentsfromtheFHNW SchoolofLifeSciencesandindustrypartners.
Zogg’steamiscontributingamodeltoscaleup acontinuousethoxylationreactionanddetermine whenanexplosionislikelytooccur.Theinitial modelisbuiltondatainscientificliteratureabout thermodynamicsandreactionkinetics,combined withsmall-scaledifferentialscanningcalorimetry (DSC)measurementscollectedintheFHNW’s ProcessTechnologyCenterexplosionreactor.
Todeterminewhentheethoxylationreactionis likelytocauseanexplosion,Zogg’steammodelled twoscenariosandperformedthemwithina uniqueexplosionreactor,usingtheresultingvapor liquidequilibriumdatatorefinetheirmodel. Althoughtheyhadmodelledamaximumexplosion pressureof12bar,thereactionwasperformedup to20barbeforeexploding.
Furthermore,Zogg’steamconceptualisedthe basicdesignofthecontinuousethoxylationplant, includingacomprehensiveriskanalysis(HAZOP).
Finally,theyperformedtheflowreactionina safetygloveboxthattheydesignedspecifically forthispurpose.Theboxprovidesanitrogen
“Theprocesssafetymodels developedbyFHNWareenabling safer,moreenergyefficient reactionsthatproducehighquality surfactants.Welookforwardto applyingthemodelstoscaleup productioninourfacilities”
RolandBorner,Schärer&SchläpferAG
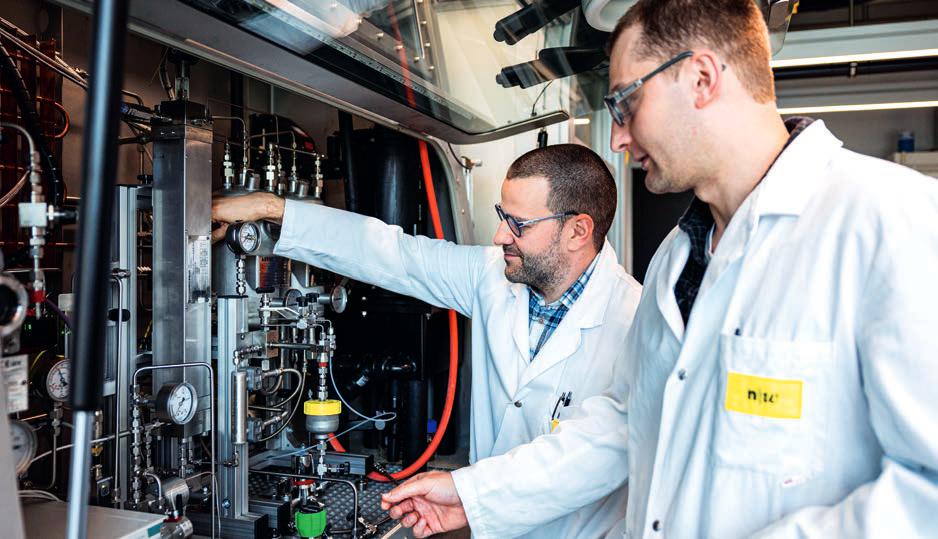
MasterstudentsThisZahndandBenediktBrönnimanngaindirectexperienceinmodelling andmanaginghazardousprocessesincollaborationwithindustrypartners.
atmosphere,monitorsoxygenandethyleneoxide levelsandfeaturesanintegratedsafetywasherto destroyethyleneoxidesteamleakage.
Fluitec,adesignerandmanufacturerof reactionsystems,iscontributingasmall-scale continuousreactioncalorimetertotheproject. Thisspecialiseddevice,whichsitsinsidethe safetyglovebox,measurestheheatreleased fromthereaction.Thereal-timedatacollected usingFluitec’sdeviceisusedtoadjusttheFHNW’s processmodel.
“Deployingourflowcalorimeterinanexperimentalsettingandcontributingtonewmodelsfor flowchemistryscale-upprovidesvaluableinsights abouthowthecalorimeterperformsatsmalland productionscale"sayssaysMarliesMoser,Fluitec.
TheFHNW’smodel,strengthenedbydata collectedbyFluitec’scalorimeter,willbeapplied bytheproject’simplementationpartner,Schärer &SchläpferAG,aproducerofsurfactantsfor
thepharmaandchemicalindustry.Thegoalisto enabledirectscale-upfromlabtoproduction volumes.
“Theprocesssafetymodelsdevelopedbythe FHNWareenablingsafer,moreenergyefficient reactionsthatproducehighqualitysurfactants. Welookforwardtoapplyingthemodelstoscale upproductioninourfacilities”saysRolandBorner, Schärer&SchläpferAG.
Inadditiontoindustrialinnovation,theproject provideshands-oneducationalopportunities.Two part-timeMScstudents,ThisZahndandBenedikt Brönnimann,areworkingontheInnosuisseproject whilecompletingtheirMaster'sinChemical Engineering.BScstudentsinchemicalengineeringalsocarryoutaportionofthework.The FHNW’sMiniplantfacility,includingitsunique explosionreactorfortestingthethermalprocess safetyofexplosivereactions,providesstudents withdirectexperienceinhazardousprocess
modellingandmanagement.
“EspeciallyforstudentsattheMaster'slevel,it enablesthemtodeepentheirknowledgein real-lifesettingsbeforetheygraduateandfully transitiontoindustry”saysZogg.“Atthesame time,itallowsustoadvanceknowledgeonhowto safelyscale-upflowchemistryprocessesto promotetheiradoptionbyindustry.”
Partners: Fluitec;Schärer&SchläpferAG
Financing/Support: Innosuisseproject62273.1IP-ENG

Prof.Dr.AndreasZogg
Groupleader,Processengineering andreactiontechnology +41612285825 andreas.zogg@fhnw.ch
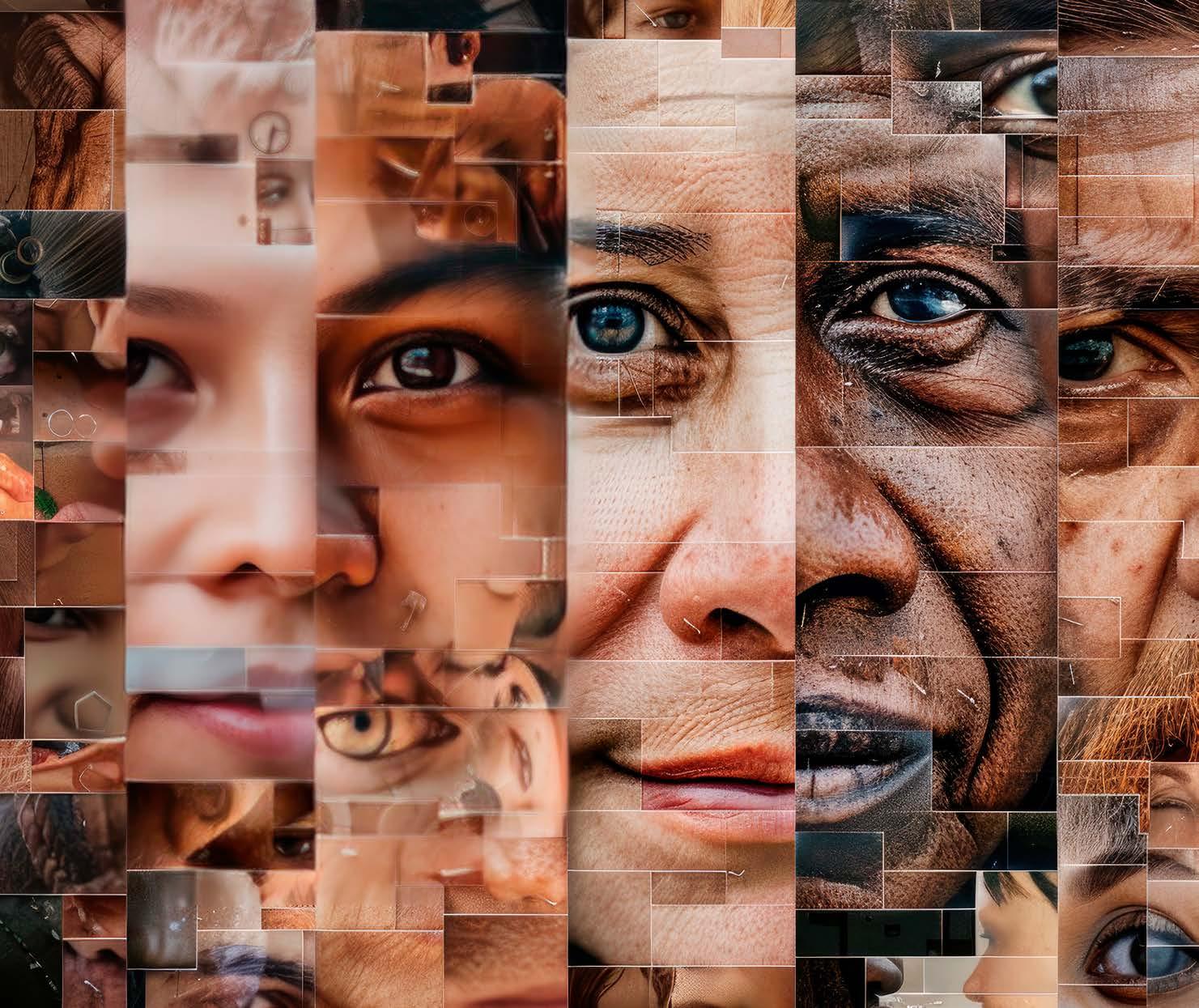
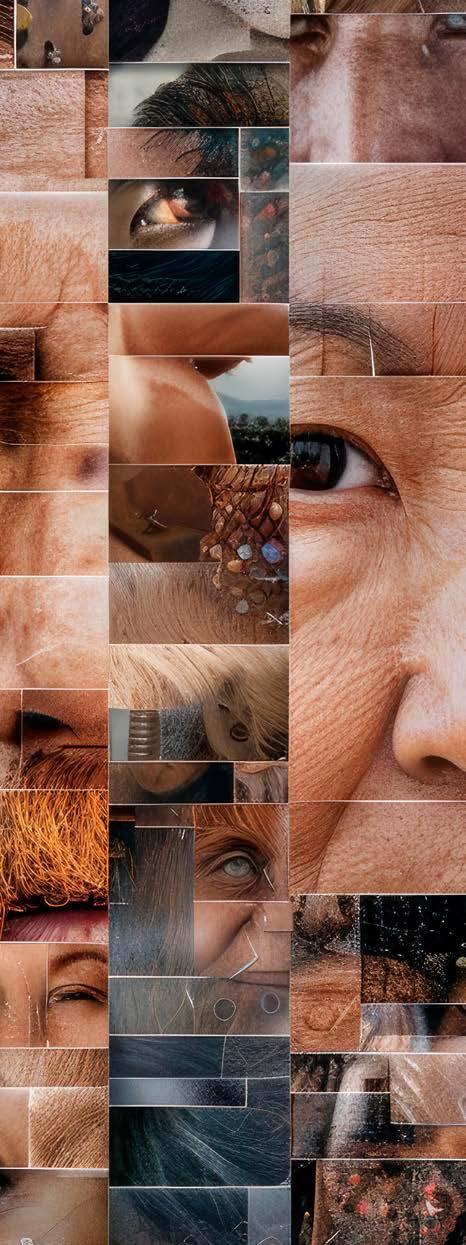
Progressfor patients
Healthcareisprogressingfromthe clinictothehome,andfromgeneralised treatmentstopersonalisedmedicine.
Adeeperunderstandingofthehuman body,diseaseandhealth,coupled withtechnologicalbreakthroughsand intelligentuseofdata,areproviding newoptionsforpatientsandmedical professionals.
Navigatingthebrain
Intelligentelectromagneticnavigationsystemforprecise implantationofdeepbrainstimulationelectrodes
Deepbrainstimulation-Didyouknow? Inthelate1980s,aFrenchbreakthroughin neurosurgery,deepbrainstimulation(DBS), openednewavenuesforthetreatmentofmovementdisorders.DBSinvolvestheimplantation ofelectrodesandtinyneurostimulatorsin thebrain.Thesedevicessendsignalstospecific targetsinthemotorregions,tohelpalleviate motorsymptoms.
DBSwasapprovedbytheFDAin1997forthe treatmentofParkison’sDiseaseandessential tremor.Itisnowalsousedtotreatdystonia,some obsessive-compulsivedisorders,epilepsy andchronicpain.Itmaybecombinedwithtaking medicines,orofferanalternativetothem.
Thesuccessofdeepbrainstimulation(DBS) dependsonprecision,bothinpositioningthe electrodesandstimulatingspecificbrainregions. Currently,pre-operativeimagingandstereotactic techniquesarethegoldstandardfortheinsertion ofDBSelectrodesintothebrain,buttheydonot offertrackingduringsurgery.
CélineVergne,whoiscompletingaPhDatthe InstituteforMedicalEngineeringandMedical InformaticswithajointenrolmentattheUniversitiesofBaselandStrasbourg,hasdevelopedan electromagneticnavigationsystemaimingatthe precisetrackingofelectrodesduringtheirimplantation.“Thechipintegratedintothestimulation device,coupledwithourvisualisationsystem,will allowsurgeonstoseetheangleandpositionofthe DBSelectrode”saysVergne.
Shefirstintegratedatinymagnetometerinto theelectrode.Lessthan1mmacross,themagnetic sensorcanbedetectedbygeneratinglowmagnetic fieldsandusingatrackingalgorithmtodetermine itsposition.Prof.Dr.JorisPascal’steamcontributedtothesensor’sdevelopment,whileProf.Dr. SimoneHemm’steamwasresponsibleforthesystemset-upandtestinginthesurgicalenvironment. IncollaborationwiththeUniversityofStrasbourg, Vergneisinvestigatingmachinelearningalgorithms tooptimisethesensor’strackingperformance.
Vergnethensetouttoensurethecompatibility ofherprototypewiththesurgicalenvironment.In theoperatinglabattheFHNWSchoolofLife SciencesinMuttenz,sherecreatedaDBSsurgical set-upusingaheadphantominsideastereotactic headset.Fortheelectromagneticnavigation system,calledManaDBS,sheaddedasystem controlunit,amagneticfieldgeneratoranda sensorinterfaceunitconnectedtotheDBSlead.
Electromagneticperturbationsfromthe magneticfieldcanthrowofftracking.Toaddress this,Vergneappliedanewtrackingprincipleto limitelectromagneticdisturbancesandensure correcttrackingoftheelectrode.
Implantationtrialsonaneggplantsimulating braintissuewereperformedinarecreatedDBS surgicalsetup.Thisgaveaninitialideaofthe sensor’sperformance.Thefinalstepbefore transfertotheclinicalenvironmentisuser-friendly softwaretovisualisetrackingintheoperatingroom.
ThesystemwillthenbetestedattheUniversity HospitalBaselduringasurgicaldressrehearsal. Thisenvironmentcansimulatethecompatibilityof thetechnologywiththeuniqueDBSoperatingtheatre,helpingtranslatetheprototypetotheclinic.
Ifsuccessful,ateamofneurosurgeonsatthe UniversityofBaselwilltestthetechnologyin patients.Ultimately,thesystemmaygothrough
medicaldeviceapprovals,ledbyaproducerof neurosurgicalornavigationinstruments.
“It’sveryexcitingtohavethreefieldscoming togetherinthisproject:electronics,computer scienceandneuroscience”saysVergne.“This multidisciplinaryapproachandcollaborationwith partnersaredrivinginnovationforward.”
“Thiselectromagneticnavigationsystemisa promisingtoolforthelocalisationoftheDBS electrodes.Wearehopefulthatitwillbeintegratedintothesurgicalprocedureandbringadvantagesforbothpatientsandmedicalteams”says Dr.EthanTaub,NeurosurgeonattheUniversity HospitalBaselandexternalexpertoftheproject.
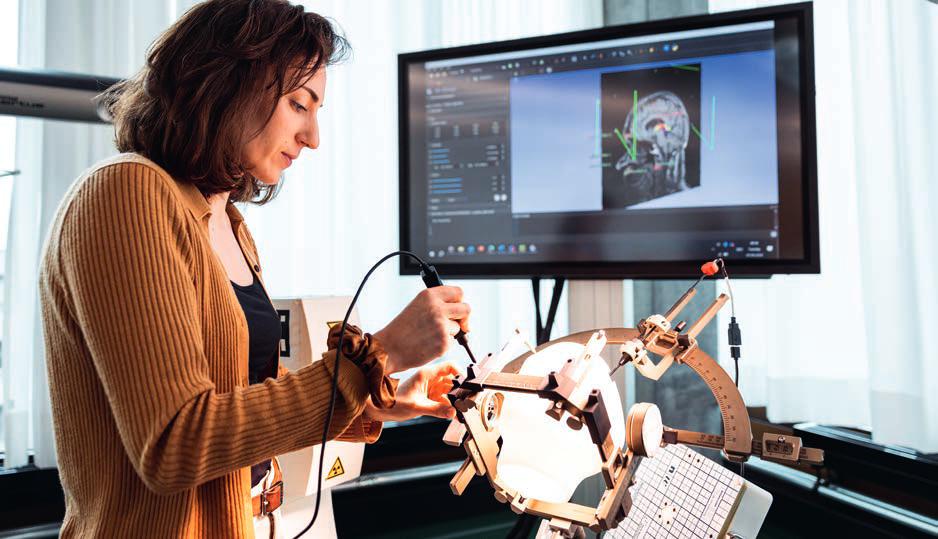
CélineVergnetestsherelectromagneticnavigationsystemforlocalisationofelectrodesin thebrain,usingaheadphantominsideastereotacticheadset.
Patients/TargetGroups: Parkinson’sdisease,essential tremor,dystonia,epilepsy,chronicpain
PartnersandPhDCo-Directors:
UniversityofBasel:Prof.Dr.med.RaphaelGuzman, Chairman,DepartmentofNeurosurgery
UniversitédeStrasbourg:Prof.Dr.MorganMadec, Simulationandelectronicssystems
Financing/Support: SNSFprojectgrant204448
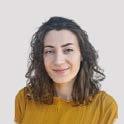
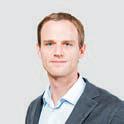
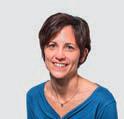
CélineVergne PhDstudent
Neuroengineeringandsensor systemsfordiagnosisandtherapy +41612285945
celine.vergne@fhnw.ch
Prof.Dr.JorisPascal Groupleader,Sensorsystemsfor diagnosisandtherapy +41612285647
joris.pascal@fhnw.ch
Prof.Dr.SimoneHemm-Ode Groupleader,Neuroengineering +41612285689
simone.hemm@fhnw.ch
Steppingup3Dprintingof orthopedicinsoles
DanielSeiler’slabisrevolutionizinghowpersonalised orthopedicinsolesaremade
Orthopaedicinsoleshelpredistributepressure andrelievesensitiveareasofthefeet,makingit morecomfortableforpeoplewithfootmisalignmentstowalkandperformdailyactivities.
Orthopaedicinsolesarealsoacrucialtoolfor patientswhosefootnerveshavelostsensitivity, whichcanunknowinglyleadtopressuresoresor evenamputation.Innon-medicalapplications, insolescanhelpincreasecomfortandstabilityfor adults,offersupporttopeoplewhospendalotof timeworkingontheirfeet,andboostperformance inrunningandothersports.
Eventothisday,personalisedinsolesare primarilyproducedusingcomputernumerical control(CNC)machinesandarefine tuned manually.Thistraditionalmethodofmillinginsoles isnotonlyenergy-intensivebutalsogeneratesa substantialamountofnoise,dustandchipwaste.
Theshifttoward3Dprintingofpersonalised insolescanreducewasteby95%.However,the keychallengeistheprinter’sabilitytomanage severaltypesofmaterialswithdifferenthardness levelsandproperties.
InanInnosuisseprojectperformedincollaborationwiththecompanyOrthopodoMalgaroli, DanielSeiler’slabattheInstituteforMedical EngineeringandMedicalInformaticshasdevelopedanautomateddigitalproductionprocess
processfor3Dprintingofpersonalisedorthopaedicinsoles.
“OrthopodoMalgaroliapproacheduswitha desiretomaketheirproductionprocessmoredigital,efficientandsustainable”saysSeiler.“This involvesnotonlyashifttoward3Dprinting processes,butalsonewmethodsinscanning patients’feetandarrivingattheoptimalinsole design.Weenvisionanend-to-endsolutionfrom footscanningtoinsoleoutputthatcanrevolutionisehowpersonalisedinsolesaremade.”
YvesLetzandRomanSantschifromSeiler’s teambeganbydesigningafuseddeposition modeling(FDM)3Dprintercapableofmanaging atleastfourdifferenttypesofsoftmaterialsthat canhelprelievepressure.Theyengineerednew printheadstoaccommodatematerialswith degreesofflexibilityrangingfrom60to90shore A.Theprintercanautomaticallyswitchmaterials andprintheadsbasedontheinsoledesignsit receives.Theseinnovationsenablethe3Dprinter toproduceinsolesthatmeetdiversecomfort andsupportrequirements.
Butlet’stakeastepback:howarepersonalisedinsoledesignscreatedinthefirstplace?A 3Dscanofthefootcombinedwithdynamicfoot pressuremeasurementsareusedwithclinical datasuchasvarus/valguslegsasinputforthe
insoledesign.Basedonthreedecadesofreal dataandexperiencefromOrthopodoMalgaroli, Seiler’steamisdevelopingartificialintelligence modelsandalgorithmsthatcanautomatically designcustomisedinsolesforpatients.
“Oursharedvisionisforpatientstocomeintoa clinicorastore,scantheirfeetandhavethe computercreatea3Dinsolemodel.Theinsolewill thenbeproducedbythe3Dprinter.Withinone hour,patientscanwalkoutwearingtheirnew custom-madeinsoles”saysSeiler.
Thinkingahead,thepartnershaveconceptualisedauser-friendlyinterfaceforthefootmeasurementandprintingplatformforpoint-of-care andpoint-of-salesettings.Astackabledesignwill accommodatemultipleprintersvertically,for clinicsthatproducemanyinsoleseachday.Finally, Seiler’steamisrefiningasecurecloudsolutionfor patientorcustomerdatastorageandprocessing.
“The3DprinterdevelopedbytheFHNWnow givesustheopportunitytoproduceorthopedic insolesquicklyandsustainably”saysMario Malgaroli,ownerofOrthopodoMalgaroli.“Inthe future,itcouldbeusednotonlyinouroffices,but alsoindoctors’offices,bypodiatristsand physiotherapists,andinshoeandsportsstores.” Seilerbelievesitcouldalsobeusedtotriage normalsupportrequirementsfrommedical
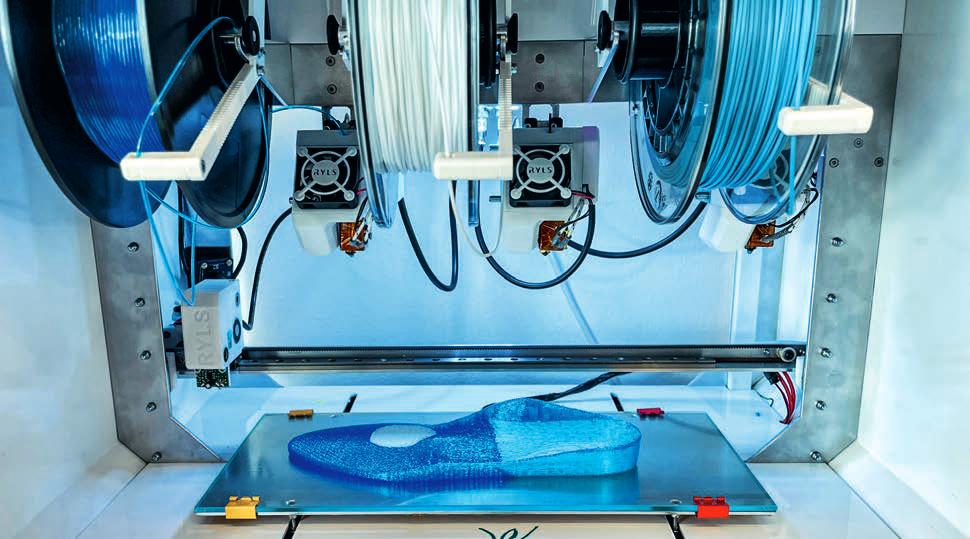
ResearchassociatesYvesLetzandRomanSantschidesignedafuseddepositionmodelling3Dprinter capableofmanaginguptofourdifferentsoftmaterialsrequiredforinsoleprinting.
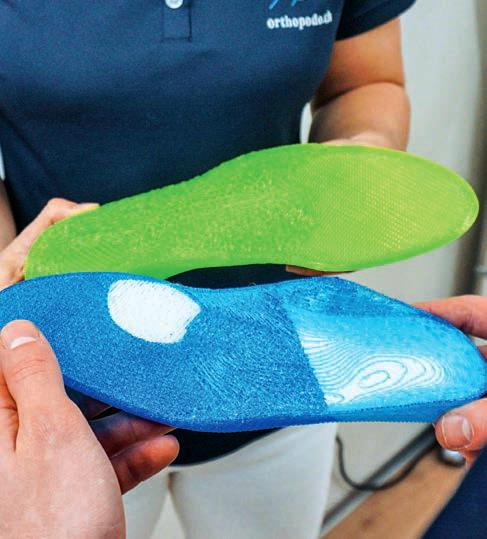
3Dprintingofpersonalizedinsoles canreducewastebyupto95%.
conditionsthatrequiretheexpertadviceof podiatrists,actingasadiagnosticdevice.
Thetransitiontoautomateddesignand3D printingofinsolesisaparadigmshiftfororthopedicfirms.“TheFHNWhashelpedOthopododerisk thetransitiontoManufacturing4.0bymaking advancedknowledgeaccessibleandworkablein practical,real-worldsituations”saysSeiler.
Inthenextstepoftheproject,atrialis plannedtovalidatetheeffectivenessandaccuracyoftheautomatedinsolemanufacturingprocess. Thiswillinvolvetestingthe3D-printedorthopaedicinsolesinreal-worldconditionstoensurethey meetpatientorcustomercomfortrequirements.
Seiler’sgroupisalsostartinganewproject financedbytheForschungsfondsAargautorefine thesoftmaterialfilamentsusedtoproducethe insolesandgivethemantimicrobialproperties.This workisbeingconductedincollaborationwiththe InstituteforChemistryandBioanalyticsand
theInstituteofPolymerEngineering.
Lastly,theteamisaimingtoreducethe amountofglueneededbetweenlayersofdifferentmaterialsbyintegratingtheglueintothe printingprocess.Theyarealsodesigningnew softmaterialsthatwillberecyclable,twofurther stepstowardincreasedsustainabilityof customisedinsoles.
Patients/TargetGroups: Footmisalignmentpatients, Adultswithreducedbalance,Standingoccupations,Sports Partners: OrthopodoMalgaroli
Financing/Support: InnosuisseProject107.955IP-LS
ForschungsfondsAargauProject20220930_17
ForschungsfondsAargauProject20240331_03
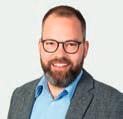
DanielSeiler Laboratoryleader, Medicaladditivemanufacturing +41612285848
daniel.seiler@fhnw.ch
Improvingwoundhealing afterC-sections
RetoWildhaber’steamhasdevelopedavisualisationtechnology tobetterilluminatecorrectivesurgeryaftercaesariansections
InSwitzerland,32%ofbabiesaredeliveredby caesareansection.Whilehospitalsareworkingto reducethenumberofproceduresthatarenot medicallynecessary,Switzerland’srateremains oneofthehighestinEurope.
Caesareansectionsposeseveralriskstothe mother,oneofwhichispoorhealingoftheuterine scar.Unknowntissuefactorscanleadtohampered woundhealingandgapsintheanterioruterinewall. Thesegaps,alsocalledniches,weakentheuterus inasubsequentpregnancy.Itcantake1-2years forwomentoobservepainandothercomplications fromimproperwoundhealing,whichmightrequire follow-upcorrectivesurgery.Chronicpainand multiplesurgicalinterventionscanpreventwomen fromperformingchildcaredutiesandreturningto theworkforce,andcanconsiderablyreducetheir qualityoflife.Ifthenichebreaksduringasubsequentpregnancy,therearesignificantrisksfor bothmotherandbaby.
SurgeonsattheUniversityHospitalZürich approachedProf.Dr.RetoWildhaberwiththeidea ofintegratingalightsourceintoauterinemanipulator,atoolusedduringgynaecologicalsurgery. Currentlaparoscopictoolsandcamerasystemsfor imagingtheuterusduringcorrectivesurgeryemit lightonlyoutsidetheuterus,andprovideanunclear viewofdifferentlayersoftissueinsidetheuterus.
Wildhaber,whospecialisesinsignalanalysis anddigitalbiomarkers,cameupwiththeideato useapulsinglightthatisinsertedintotheuterus throughthevaginaandcervix.Hesupportedthe developmentofthefirstprototypewhileworking attheBernerFachhochschule(BFH).In2021he joinedtheInstituteforMedicalEngineeringand MedicalInformaticsandcontinuedtoworkonthe projectincollaborationwithBFH,withwhomajoint patenthasnowbeenfiled.
First,Wildhaber’steamtackledthephysical challengesofrefiningtheprototype.Theyselected thematerialandprocesstoproducetheinstrument tipwherethepulsingredLEDlightsourceis housed:3Dprintedbiocompatiblepolymethyl methacrylate.Thetipsweresubjectedtomechanicalforcetestingtoensuretheycanbendduring surgerywithoutsnapping.
Tofacilitatesterilisation,theinstrumentcanbe disassembledintocomponentsandlaidflatinan autoclave.Theconicaldesignofonecomponent actsasa‘plug’forthecervix,stabilizingtheinstrumentandpreventinggasinjectedintotheabdomenduringsurgeryfromescapingtheuterus.
Tokeepthetipoftheinstrumentcooland preventdamagetouterinecelltissue,fibreoptic cablesareusedtotransmitlightfromthepulsing redLEDsintotheuterus.
Theteamthenputmathematicsintoaction, developingadigitalenhancementequationthat exploitsthedifferencebetweenthepulsinginterior lightemittedbytheprototype,andtheexterior lightemittedbylaparoscopictools,tocreatean imageoverlayonthesurgicalmonitoringscreen.
The2Daugmentedrealitywillhelpguide surgeonsbydisplayingtheboundaryofdifferent tissuesintheuterusandthelocationofthe surgicaltool.Itwillenablethemtoseetheniche andpreciselyremovescartissue.
Overall,theinstrumentprovidesanintrauterine lightsourcethatallowsdirectlocalisationofthe nichewhilestabilizingtheuterus,andiscompatiblewiththeDaVincirobotthatassiststhecorrectivesurgery.Thesmallcomputerrequiredto performthedigitalenhancementequationsand thescreenthatdisplaysthe2Dvisualisationcanbe easilyintegratedintoexistinglaparoscopicset-ups.
Thevisualisationtechnologyunderwenta proof-of-conceptpilotstudyinWildhaber’slabin thesummerof2024,whichwillbefollowedby clinicalstudiesattheDepartmentofGynecology attheUniversityHospitalZürichafterapprovalby Swissmedicandthelocalethicsauthorities.
“Webelievethatwomenwithlowerabdominal symptomsandwomenwithanichethatwishto conceiveasecondchildwillprofitfromthenew
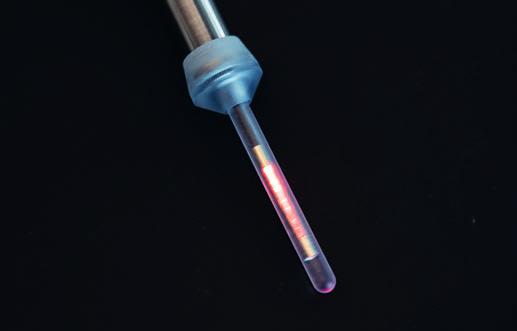
ApulsingredLEDlightinthetipoftheinstrumentisusedin digitalenhancementequationstogeneratea2Daugmented realityduringsurgery.
device”saysProf.Dr.med.CorneliaBetschart Meier.“Wearelookingforwardtoapplyingthe so-calledscarlightdeviceinafirstclinicaltrial.It willenhancethesurgicaltechniqueandweexpect itwillyieldagoodoutcomeformothersand children.”
“Thisdevicehasthepotentialtosimplifya medicallynecessaryprocedure,andmakeitsafer andmorereliable,thankstotoday’stechnology” saysWildhaber.
Ifclinicaltestsaresuccessful,aspin-off companyatUSZcouldbeformed,orthetechnologycouldbelicencedtoasurgicalorimaging instrumentsfirminordertobringittothemarket.
Patients/TargetGroups: Gynaecologicalhealth Partners: UniversityHospitalZürich, FachhochschuleBern
Financing/Support: Innosuisse(101.964IP-LS)
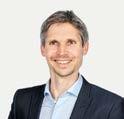
Prof.Dr.med.RetoWildhaber
Groupleader,Signalanalysisand digitalbiomarkers +41612286125 reto.wildhaber@fhnw.ch
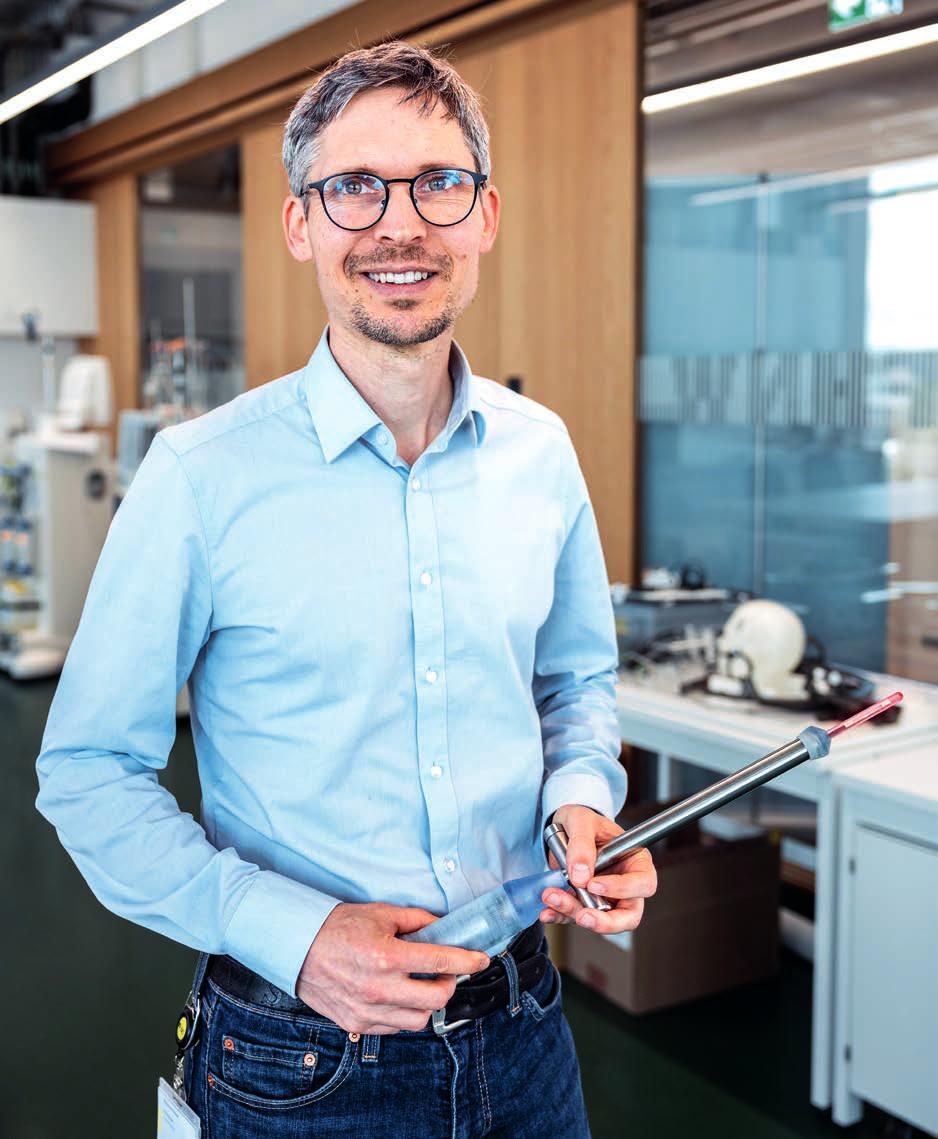
Intelligentdrugformulation
TwoteamsattheInstituteforPharmaTechnology andBiotechnologyareadvancingresearchonintelligent formulationoforaldrugs
Predictingsolubilityandformulation compositiontoreduceanimaltesting About80%ofexistingandneworaldrugsarepoorlysolubleinthegastrointestinaltract.Assessing theperformanceoforalformulationshaslargely reliedonanimaltesting,whichisincreasingdespite theEU’scommitmenttoreducinganimalsinresearch.
Tooffernewsolutionsandreduceanimaltesting,researchersattheInstituteforPharma TechnologyandBiotechnologyareparticipatingin theEUprojectInPharma.Theprojectaimsto developanend-to-endmodellingapproachtothe formulationofdrugsfororaladministration.
Prof.Dr.MartinKuentz,TeamLeaderinOral FormulationofChemicalDrugs,isleadingthe project’sworkpackage1,Computationaltoolsto developoptimaloraldrugformulations.Thegoal oftheworkpackageistofindsuitableexcipients andformulations,forexampleusingquantum chemicalmethodsandmolecularsimulation.
“Artificialintelligenceandmechanistic modellingareincreasinglyshapingthedevelopmentofdrugproducts”saysKuentz.
InPharmaactivelycontributestotraining13 early-stageresearchers,whoarecompletingtheir EuropeanIndustrialDoctoratesaspartofthe project.Kuentzissupervisingtheworkperformed bytwooftheseearly-stageresearchers.
OneofthemisShaidaPanbachi,whois collaboratingwiththefirmZentivaontestingthe industrialfeasibilityofnoveldeepeutecticsolvent formulations.Forthefirstpartoftheproject, Panbachiusedcomputationaltoolssuchas moleculardynamicssimulationstopredict physicalandchemicalpropertiesofdeepeutectic mixtures,whichshecompletedattheFHNW SchoolofLifeSciencesinMuttenz.
ThenPanbachitravelledtoPraguetoworkon capsulecompatibilityandprototypedevelopment withZentiva,aleadingmanufacturerofgeneric medicines.UnderthesupervisionofDr.Josef Beranek,Panbachiisstudyingthe invitro release propertiesofinnovativedeepeutecticformulations,whichbridgesovertoWorkpackage2led bytheUniversityofAthens.
ShaidaPanbachihasalreadypublishedafirst scientificpaper,whichcontributestotheoverarchingprojectobjective,topromoteformulation innovationusingcomputationaltoolsand invitro teststoreducepreclinicalanimalstudies.
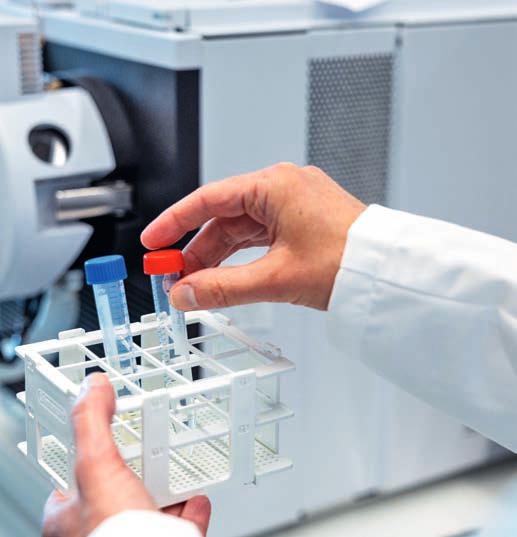
MartinKuentzandhisteamanalysenew liquidformulationschemically.
Financing/Support: EUHorizon2020InPharma955756 PartnersandBeneficiaries: 18organisations,including Zentiva
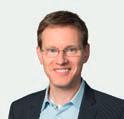
Prof.Dr.MartinKuentz
Groupleader,Oralformulationof chemicaldrugs +41612285642
martin.kuentz@fhnw.ch
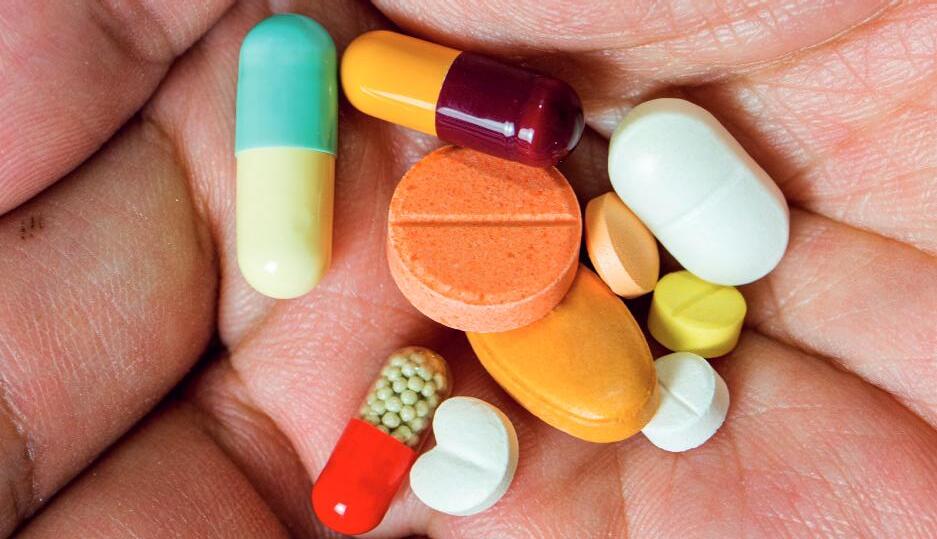
Aquarterofpeoplewhoare65andoldertakefiveormoremedicinesperday.Inthefuture,personalisedformulationsmade usingmicropelletswillhelpeasethepillburdenandmaketreatmentmoreeffective.
Precisionpharmaceuticals
Livinglongerisabenefitofmodernsociety,yetour lifestyleandageincreaseourdiseaseburden.A quarterofpeoplewhoare65andoldertakefiveor moremedicineseveryday,cocktailsthatsometimes haveunexpectedadverseeffectsorlittleefficacy.
Understandinghowwemetabolisedrugs individually drug geneinteraction,andhowthe drugsinteractwitheachother(drug-drug interaction,becomesincreasinglycomplexthe moremedicineswetake.
Across-disciplinaryteamfromthreeinstitutes iscollaboratingtodevelopprecisionpharmaceuticalsthatpredicttherightdosesoftherightdrugs attherighttimes.
Real-worlddrugcombinationsfromproton-pumpinhibitors,bloodthinners,anti-hypertensives,statins,painkillersandmorearetested inorgan-on-chiphuman invitro modelstostudy theirinteractionswhenabsorbedandmetabolised.
Thosemodelscomprisepatient-derivedcells withdifferentmetaboliserprofilestoreflectthe heterogeneityofourpopulation.
Feedingthisdatainto insilico modelsallows predictionofdosageadjustmentstoachievethe besttreatmentswiththefewestsideeffects.
Inthenextphaseoftheproject,theteamwill joinforceswithdrugmanufacturerstoworkon thenextgenerationofmedicinesthatcanbe individuallydosed.Insteadoffixed-dosetablets, drugswillbeformulatedinso-calledmicropellets, whichareeasytoswallowandperfectfor personaliseddosages.
“Imagine!Inthefuture,patientscangototheir pharmacy,andbasedontheirpharmaco-genetic profileandprescriptions,receiveprecision pharmaceuticalsthathavebeencustom-madefor them”envisionsProf.Dr.JohannesMosbacher, whoisleadingtheproject.Asitoncewasinthe past,pharmacistswouldbethosewhoprepare
themedicinesforpatients,thistimewithhelpof 3Dprintersormicropelletsortingmachines.
Patients/TargetGroups: Olderadults,peopletaking multiplemedicines
Partners: DOPPLSA,GlattGmbH
Financing/Support: StiftungFHNW,Innosuisse44386.1
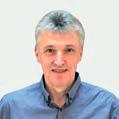
Prof.Dr.JohannesMosbacher Lecturer,InstituteforPharma TechnologyandBiotechnology +41612286149 johannes.mosbacher@fhnw.ch
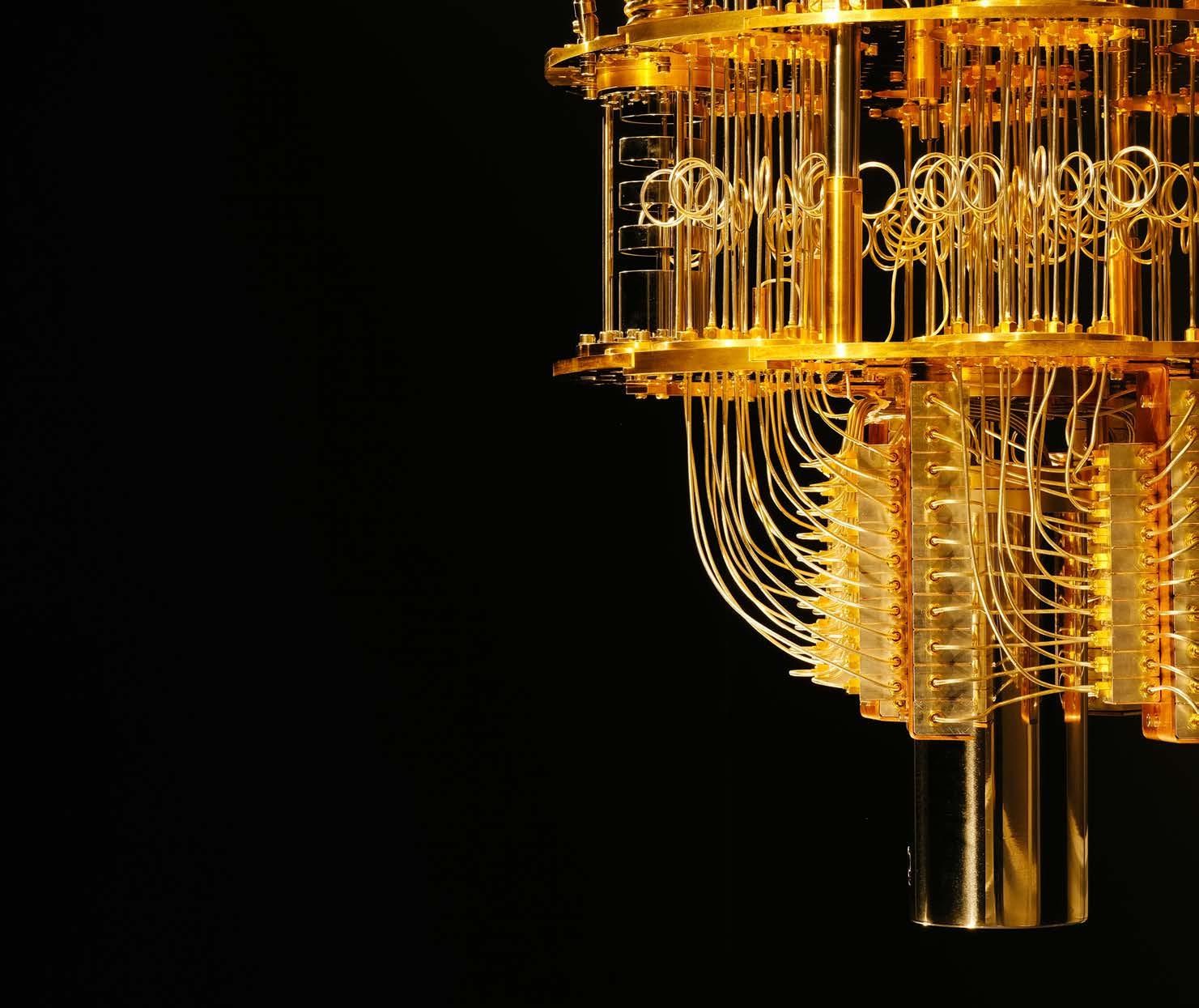
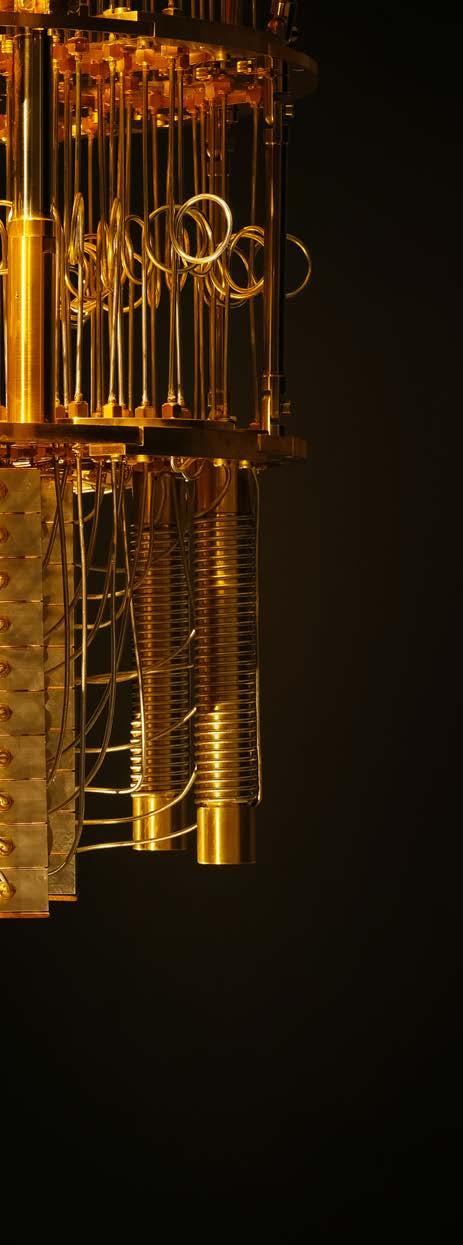
Technologyin focus
Artificialintelligence,datascience andquantumcomputingaredeveloping atanexponentialpace.Thisrapid evolutionisgeneratingopportunitiesto harness,aswellasresponsibilitiesto shoulder.Attheforefrontofthesechanges, theFHNWSchoolofLifeSciences providesscientificvisionandethical leadershiptodevelopthedigital technologiesofthefutureandapplythem toresearchquestionsinlifesciences.
Qubitsandbits withClémentJaverzac
In2022,theFHNWSchoolofLifeSciencesmadetheboldmove toestablisharesearchgroupfocusedonappliedquantumcomputing, becomingthefirstUniversityofAppliedSciencesinSwitzerlandto enterthequantumspace.LedbyClémentJaverzac,thegroupisona missiontoapplyquantumcomputingtolifesciences.
Itstartswitheducation
Prof.Dr.Javerzac,tellmewhat’shappening attheFHNWSchoolofLifeSciencesinquantum computing?
We’restartingwitheducation:makingstudents andfacultyquantumaware.Weheldafirstcourse intheMaster'sinMedicalInformaticsin2023, whichgavestudentsthecouragetotacklewhat canseemlikeacomplextechnology.In2024,a quantumcomputingmodulewasincludedinboth BScandMScprogrammes,andweheldasummer schoolattheFHNWonthistopic.
Youdon’tneedtobeamathematicianora physicisttotryquantumcomputing.Thereare user-friendlytoolsandpackagesliketheIBM QuantumComposerthatcanhelpyougetstarted. Whatyoudoneedisanunderstandingofwhich typesofproblemsquantumcomputingis goodatsolving.
Bringyourproblems
Howdoyouidentifytherightresearchquestions toapplyquantumto?
Workingalongsidelifescienceresearchersprovidesanexcellentopportunityforinterdisciplinary exchange.Iaskpeopletobringmetheirproblems! ButIdon’tguaranteethatquantumcansolve themall.
Oneapplicationweareworkingonissimulatingnuclearmagneticresonance(NMR)spectra thataretoocomplexforclassicalsupercomputers. Quantumalgorithmscanbeusedtodecodethe compositionofmatterfornewmaterials,for exampletoidentifythebeststructurefora materialthatcancaptureCO2
Quantumsensingismorematureandpromisingformedicalapplications,forexample,diagnosingfunctionallyrelevantcoronaryarterydisease fCAD).Theheart’stinymagneticfieldcanbe measuredwithquantumsensors.Togetherwith JorisPascalfromtheInstituteforMedicalEngineeringandMedicalInformatics,wearedevelopingamagneto cardiograph(MCG)asapoint of caredevicepoweredbyquantumsensors,in collaborationwithCSEMandtheUniversity HospitalBasel.
Thequantum-AIhandshake
Whattypesofproblemsshouldquantum computingstayoutof?
IncontrasttoAI,quantumcomputingisnot goodatsolvingproblemsthatinvolvebigdata, becauseitisslowtoloadit.Quantumisidealfor solvingcombinatorialproblemswherethereare fewvariablesintheinput,andmanypossibilitiesin theoutput.Thinksupplychainandlogistics,
schedulingshiftworkorDNAsequencing. Withhybridalgorithms,youcangetthebestof bothworlds:youcanaskAItosolveapartof theproblem
veryfast
,andquantumtheother
veryhard
Quantumcommunities
WhichquantumcomputersistheFHNWSchoolof LifeSciencescurrentlyaccessing?
Webookcomputationtimeonthebestinfrastructuresintheworld,includingIBM’squantum computersintheUnitedStates.Thisprivileged accessismadepossiblethroughourpartnership withQuantumBasel.
We’realsoexcitedaboutbuildingEuropean capacityinquantumcomputing.IonQisestablishingaEuropeanquantumdatacentreincollaborationwithQuantumBasel,consistingoftwo systemswith35and64algorithmicqubits respectively.ThiswillpositiontheBaselregionas oneofthemostpowerfulquantumcomputing centresintheworld.
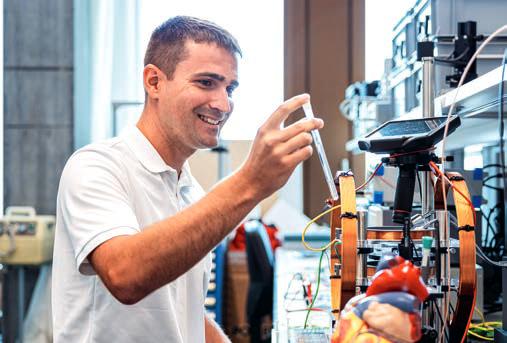
Javerzac‘steamdevelopsquantumsensorsfor newmedicaldevices.
Quantumforeveryone?
Classicalcomputersusedtotakeupentire roomsandbeusedbyspecialists.Nowtheyfit inourpocket.Doyouseeasimilartrajectory forquantumcomputers?
Idon’tthinkquantumcomputerswillreplace currentcomputers,becausedifferenttypesof processingunitshavedifferentfunctions.
Centralprocessingunits(CPUs makeupthe coreoftoday’slaptopsandmobiledevices.
Graphicprocessingunits(GPUs firstappeared forgraphicdesignandvideogameapplications, andarenowusedtotraindeeplearningmodels.
Tensorprocessingunits(TPUs anddata processingunits(DPUs arethenextgeneration ofprocessorsdesignedspecificallyforbigdata applicationsandcentres.
Quantumprocessingunits(QPUs arebestfor solvinghard,combinatorialproblemswithout replacingclassicalcomputing.
Beforequantumcomputerscanmakeittoprime time,weneedtoincreasetheirsize:notonlythe numberandqualityofqubits,butthenumberof operationstheycando,whichiscalledcircuit depth.TheNationalCenterofCompetencein Research:SpinQubitsinSilicon(NCCR-SPIN)is workingonalternativewaferscalequantumcircuitsthatcanbefabricatedbythesemiconductor
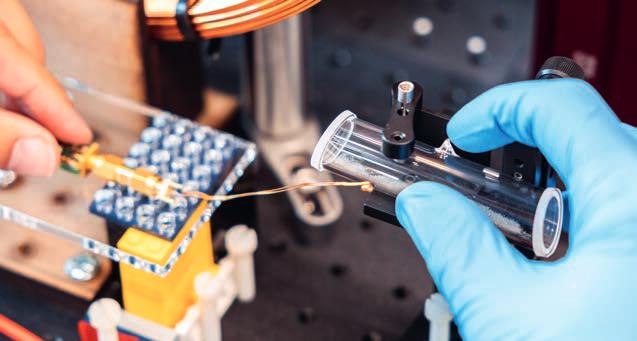
Atomicvaporcellscanbeusedtomeasuretinymagnetic fieldssuchastheoneemittedbythehumanheart.
industry.Scalingupfromqubitsinthedouble digitstothemillionswillbringusintoaneweraof quantumcomputing.
Quantumutility
Whatdoesthefutureofquantumcomputing looklike?
We’removingawayfromquantumsupremacy towardquantumutility–understandingwhere quantumcanmakeapracticaldifference.Atour HackLifeevents,companiesbringustheir problemsandwelookatavarietyofapproaches, includingAIandquantum,toseehowwecan solvethem.
Asthenextgenerationofappliedquantum expertsgraduatefromtheFHNWandotherorganisations,theywillmovetoindustryandbecome ambassadorsofthetechnology.I’mexcitedto seewherewestandinfiveyears,withthisnew cohortapplyingthetechnologytodrugdiscovery, materialsscienceandmedicaldevices.
Partners: uptownBasel,QuantumBasel,CSEM,University HospitalBasel,CreativeDestructionLab(CDL),NCCR Spin,SwissQuantumInitiative Financing: SNSF51NF40-180604,Innosuisse, NCCR-Spin,SCNAT.
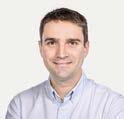
Prof.Dr.ClémentJaverzac Groupleader,Appliedquantum computing +41612285189 clement.javerzac@fhnw.ch
Spliceitup!
AbdullahKahramanisharnessinglongreadsequencing toidentifynewbiomarkersanddrugtargets
Sequentialimprovements
Prof.Dr.Kahraman,yourteamattheFHNW SchoolofLifeSciencesareanalysingpatternsin genesequencestounderstandandpredictdisease. Tellushowgenesequencinghasevolved. ThefirstgenerationofDNAsequencingbeganin 1977whenFredSangerpublishedhismethodof sequencingfragmentedDNAmolecules.Later,in 1987,itbecamepossibletoautomateSanger’s sequencingmethod,speedinguptheprocesstoa scalewherethesequencingoftheentirehuman genomecouldbeinitiated.Beforethefirstdraftof thehumangenomewasannouncedin2000,a secondgenerationofDNAsequencerswas developedthatcouldsequencemillionsoftiny DNAfragmentsinamassivelyparallelfashion. Now,thethird-generationofDNAsequencing machinescansequencesinglelongDNAfragmentsthat,incombinationwithsingle-celland locationinformation,cangiveusanunprecedentedviewofhowDNAandRNAmoleculesunderline humanbiologyanddiseases.
Alternativesplicesrevealbiomarkersofdisease
Whattypesofinformationcanbefoundusing long-readsequencing?
WhenRNAistranscribedfromDNA,non-coding regionsintheRNAsequencearecleavedoff
splicedout.Theremainingcodingregionsare combinedintodifferentmessengerRNAs.This cellularprocesscalledalternativesplicingisthe reasonwhythesmallnumberofgenesinourDNA cangenerateallthedifferentproteinsthatcells inourbodyneed.
Incancercells,alternativesplicingisoften broken.Asaresult,proteinsareproducedthat promotethesurvivalandgrowthoftumorcells. Withsecond-generationsequencing,accurately identifyingthecompletesequenceoflong transcriptshasalwaysbeenchallenging.However, long-readsequencingnowenablesthedeterminationoftheentiresequenceofindividual transcripts,providinguswithanunprecedented opportunitytoexplorethefulldiversityofRNA moleculesinnormalandcancercells.
IncollaborationwiththeFunctionalGenomics CenterinZurich,myteamusesthenewestsequencingtechnologiestostudytumourdevelopmentandtherapyresistanceincancerpatients. Wedevelopsoftware,machinelearninganddatabases,andintegratethenoveldatasetswithmutational,structuralandproteinexpressiondata.Our goalistoidentifypatternsthatcanpredictdisease progressionanddrugresponse,aimingtodetect cancersearlyenoughthatpatientscanbetreated withouttheirtumoursspreadingintometastases.
Formandfunction
WhataresomechallengesinanalysingRNA sequences?
Figuringoutifatranscriptisoformisadriverof cancerisnoteasy.Althoughlong-readsequencing allowsustoexaminethediversityofallcell transcripts,italsotendstorevealmanynoveltranscriptsforwhichnobiologicalfunctionisknown. Ourfirststudiessuggestthatmostofthese transcriptsaretechnicalartifactsortheresultof unfinishedsplicing.Understandingwhichtranscriptsarebiologicallyrelevantandwhichareonly artifactsisanimportantscientificquestionthat weaddressinmyresearchgroupusingour broadexpertiseinmachinelearningandomics dataanalysis.
Targetingtranscripts
Whattypesofdrugsortherapiescanbedevelopedtotargetdisruptedalternativesplicing?
Therearecurrentlytwotypesoftherapiesfor disruptedsplicing.Oneclassofdrugstargetsthe protein-RNAcomplexcalledsplicesome,whilethe otherbindstopathogenicRNAmoleculesto modifytheirsplicing.Splicesomeinhibitorsare approvedtherapiesforcancerpatientswhohave mutatedsplicesomegenes.Splicingmodifiers,in contrast,areantisenseRNAandsmallmolecule
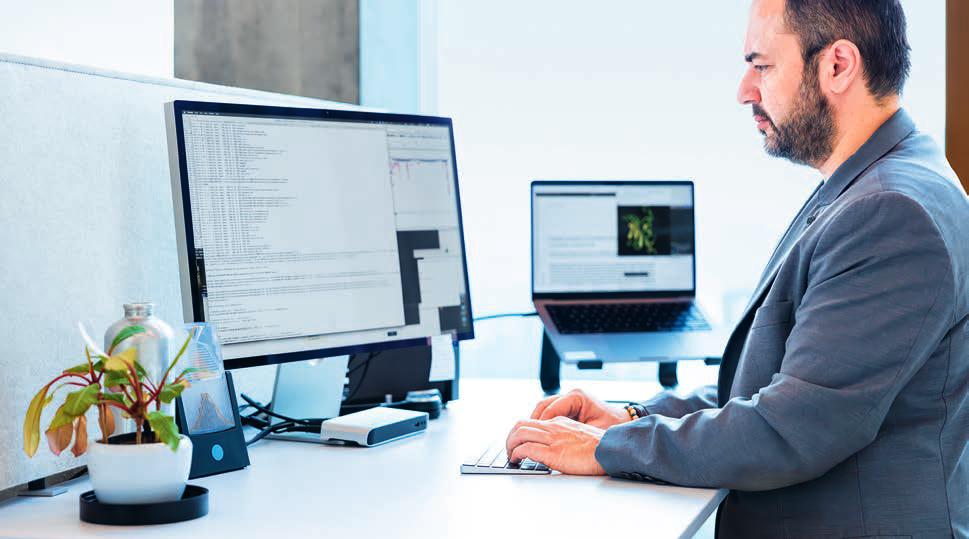
AbdullahKahramanusesmachinelearningandAI,aimingtodetectcancerbeforetumoursspreadintometastases.
drugsthattargetsplicingeventsinrarediseases, forexample,thedrugRisdiplambyRoche.It’sa smallmoleculethatcanactivatethegeneSMA2 byinducingtheinclusionofanexoninitsRNA, therebyrestoringmusclemotorfunctioninSpinal MuscularAtrophypatients.
Thenextfrontier:Wholeproteinsequencing Whichtechnologieswillshapethefutureofour geneticunderstandingofcancer?
Wecurrentlylackathoroughunderstandingof proteinsandproteincomplexesincancer.The problemwithcurrentproteomicsmethodsisthat theycanonlydetectsingleshortpeptidesfrom longproteinsequences.Ibelieve,therefore,that thenextfrontierwillbewholeproteinsequencing. Apromisingtechnologyinthisfieldisnanopore sequencing.Uncoveringentireproteinsequences willbeagamechangerfortargetdiscovery,not onlyasatooltovalidatealternativesplicing
identificationsbutalsotoassessproteinexpressionandregulatorymodificationsofproteins.
Atthesametime,machinelearningand artificialintelligencewillbecomefundamental technologiesforfuturecancertreatmentin hospitals.Cliniciansarealreadyusinglarge languagemodelstowritestructureddiagnostics reportsorautomaticallydetecttumorcellsin biopsyimages.Artificialintelligenceagentsin hospitalsareenablingthecollectionandintegrationofheterogeneousdatafrompatientsandwill helpcliniciansidentifythebesttherapypathfor theirpatients.Myteamisinvolvedindeveloping bothmachinelearningandAIagentsthrough collaborationsandcontributionstoflagshipgrant proposals.Wehopeourworkwillimprovethe treatmentjourneyofcancerpatientsinhospitals andcontributetofightingthisdevastatingdisease.
Partners: UniversityofZürichandUniversityHospital Zürich,FunctionalGenomicsCenterZürich,University HospitalBasel
Financing: KrebsligaZürich,EMDOGrant,SNFPractice toScience
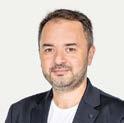
Prof.Dr.AbdullahKahraman Groupleader,Datasciencein lifesciences
+41612286223 abdullah.kahraman@fhnw.ch
Artificialintelligenceinthe ageofadisciplinarity
EnkelejdaMihoputsdifferentfieldstoworktodevelopnew medicines,diagnostics,andabetterunderstandingofthe health-diseasecontinuum
Developingnewmedicinesforunmet medicalneeds
Prof.Dr.Miho,therearemanywaystoapplyAIto researchinlifesciences.Whatisyourteamat theFHNWSchoolofLifeSciencesfocusingon? Oneofourappliedprojectsfortherapeuticsisthe designofbroadlyneutralizingantibodiesagainst theDenguevirus.Currently,thereisnomedicine totreatthevirus–existingtherapiesfocuson alleviatingsymptoms,whilecurrentvaccinesonly protectagainstsomeserotypes.
Todesignanantibody-basedtreatment,we investigatedtheB-cellimmuneresponseto Denguevirusantigensinmiceandhumans. Specifically,welookedatB-cellsinmurinebone marrow,whichiswherelong-termimmune memoryisheadquartered.Usinghigh-throughput single-cellsequencing,weobtained47.8million rawreadsofB-cellsandtheantibodysequences theyproducedafterexposuretoDengueantigens ofvariouscomplexity.
Thenwehadtomakesenseofthislarge-scale data.Weappliedmachinelearningtodetect patternsintheantibodyrepertoiresandsequencesagainstDengue.Butdothesefindingsapplyto humans?Todeterminethis,welookedatmemory B cells(MBC)fromhumanpatientsaffectedby acuteDengueVirus2.
Weconcludedthat,inbothmiceandhumans, exposuretoDenguegenerateslongCDR3sand Y-richmotifsintheantibodyrepertoire.Our hypothesisisthatthesefeaturescouldberesponsibleforquicklyactivatingmemoryB-cellsand generatingbroadlyneutralizingantibodiesthat bindtotheEproteinonthesurfaceofDengue viruses.
Notonlydidweidentifypromisingantibody sequencesforneutralisationofinfectionsbutwe alsogainednewknowledgeabouttheantigens. DenguewholeE-2complexantigengeneratesthe mostdiverseantibodyresponseandcould thereforebesuitableforthedevelopmentofnew vaccinesagainstthevirus.
Thenextstepofthisprojectwillinvolve humanizingantibodysequencesweisolatedin micesothattheycanbeusedaspotential therapeuticsforDengueinfectionandtoprevent severedisease.
Softwareasadiagnosticdevice
WhataboutDr.AI?Doyouseeabigrolefor AI-assisteddiagnosis?
IseeanincreasingpotentialinusingAItosupport precisediagnosisindiseasesthatcanbedifficult toidentifybecauseoftheiroverlappingand unspecificsymptoms,forexampleautoimmune
andrarediseases.Thisinvolvesunravellingwhich dataismostusefulfordiagnosingwhichdiseases, andfindingwaysofcombiningandstandardizing thatdata.Myteamisintegratingclinicaldatafrom electronichealthrecords,laboratoryvaluesand multi-omicsdata.Wehavedevelopednew integrationmethodsthatcandothis.
Thesecondelementinvolvesbenchmarking machinelearningmodelstodeterminehowwella givenmodelcanidentifydiseaseandwhichmodel isthemostaccurate.Wehaveenhancedour findingsfromrealpatientswithvirtualpatients–likeadigitaltwinofapatient.Wehaveworked withEuropeanhospitalsandmedicalexpertsfrom GermanyandFrance,andwehopetoapplythe findingstoimplementdigitalclinicaltrialsto improvenotonlydiagnosesbutalsotherapy regimenoutcomes.
Tobringsoftwareasadiagnosticdeviceto hospitalsandthemarket,wemaintaincloseties withSwissmedicandtheSwissNetworkfor DigitalMedicalRegulation.
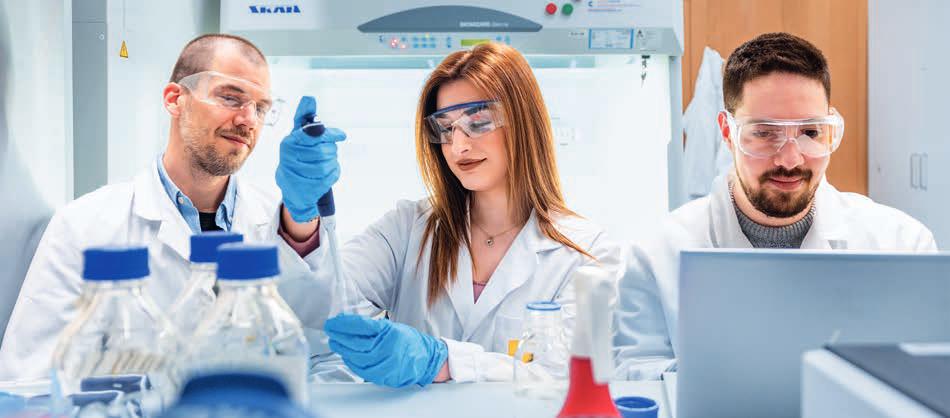
JetlindaKrasniqi,NicolasBopp,andJanKrutausemachinelearningtoextrapolateimmuneresponses,validatetheirworkin thelab,andfeedtheirresultsbackintocomputationalmodels,tobetterunderstanddiseaseandthebody’sresponse.
Listeningtothesecretsoftheimmunesystem Inbothexamplesyou’vegiven,itseemsthat understandingtheimmunesystem’sresponseto diseaseiskey.Whichresearchdirectionswill youtakeinthefuture?
Often,theimmunesystemrevealswhatis goingonbeforediseasestrikes.We’dliketomap outtheimmuneimprintofdiseases–acolossal task.I’minterestedinexploringandrevealingthe relationshipsbetweeninfection,cancerand immunediseases.
Todothis,myteamisstudyingmolecular dynamics.Thesearegiantnetworksthatfeature largeinputdata,whichAIiswellsuitedtohandle, butalsocomplexcombinatorialintermediate scenarios,forwhichquantumconceptsand quantumcomputingcouldhelp.Iexpectwewill useahybridapproachtouncoverthesecretsof howinfection,cancerandimmunediseases arerelated.
AIintheageofadisciplinarity
AIandlifesciencesarecomingtogether.Doyou consideryourselfaninterdisciplinaryresearcher?
Ithinkit’susefultousetheconceptofadisciplinaryinsteadofinterdisciplinary.Multiple disciplinesareevolvinginparallel.Somefields aremerging,whileothersaresplittingapart (e.g.theemergingbiochemistrybetweenbiology andchemistry.Myteamandmystudentsuse conceptsfromlifesciences,computerscience andbusinesstosolveaproblemathandby applyingprinciplesfromvariousandemerging disciplines.Soweareagnostictodisciplines inasense.
Thisappliesnotonlytoresearchprojectsbut alsototeaching,forexampleintheDigital TransformationinHealthcarecourseandevenin theHackLifehackathonwe’vebeenorganizing withProf.JaverzacattheFHNWSchoolofLife Sciencessince2022.Weaskorganisationsto presenttheirindustry-relevantchallenges,andwe useavarietyofapproachesincludingAI,MLand quantumcomputingtodevelopsolutionsthat shouldbepracticaltoimplementinabusiness context.
Andafinalwordabout‘hacking’–itusedto meanlearningtocode,nowitmeanslearning
toprompt.Largelanguagemodelshave changedtherulesofthegame.Themostfamous isChatGPTandtherearemanyothers.Using languagetoformulatepromptsisthelatest developmentthatmightrevolutionisenotonly complexscientificdisciplinesbuteveryday workandlife.
Partners: UniversityHospitalsStrasburg,Mainz,Freiburg; UniversitiesofPavia,Pamplona,Utrecht,Heidelberg; InstitutePasteur,UniversityofOslo
Financing: SNSFEUREKA32ER30-213721,Wellcome TrustInnovator’sAward
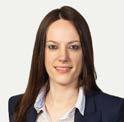
Prof.Dr.EnkelejdaMiho Groupleader,AIhealthlab +41612285847 enkelejda.miho@fhnw.ch
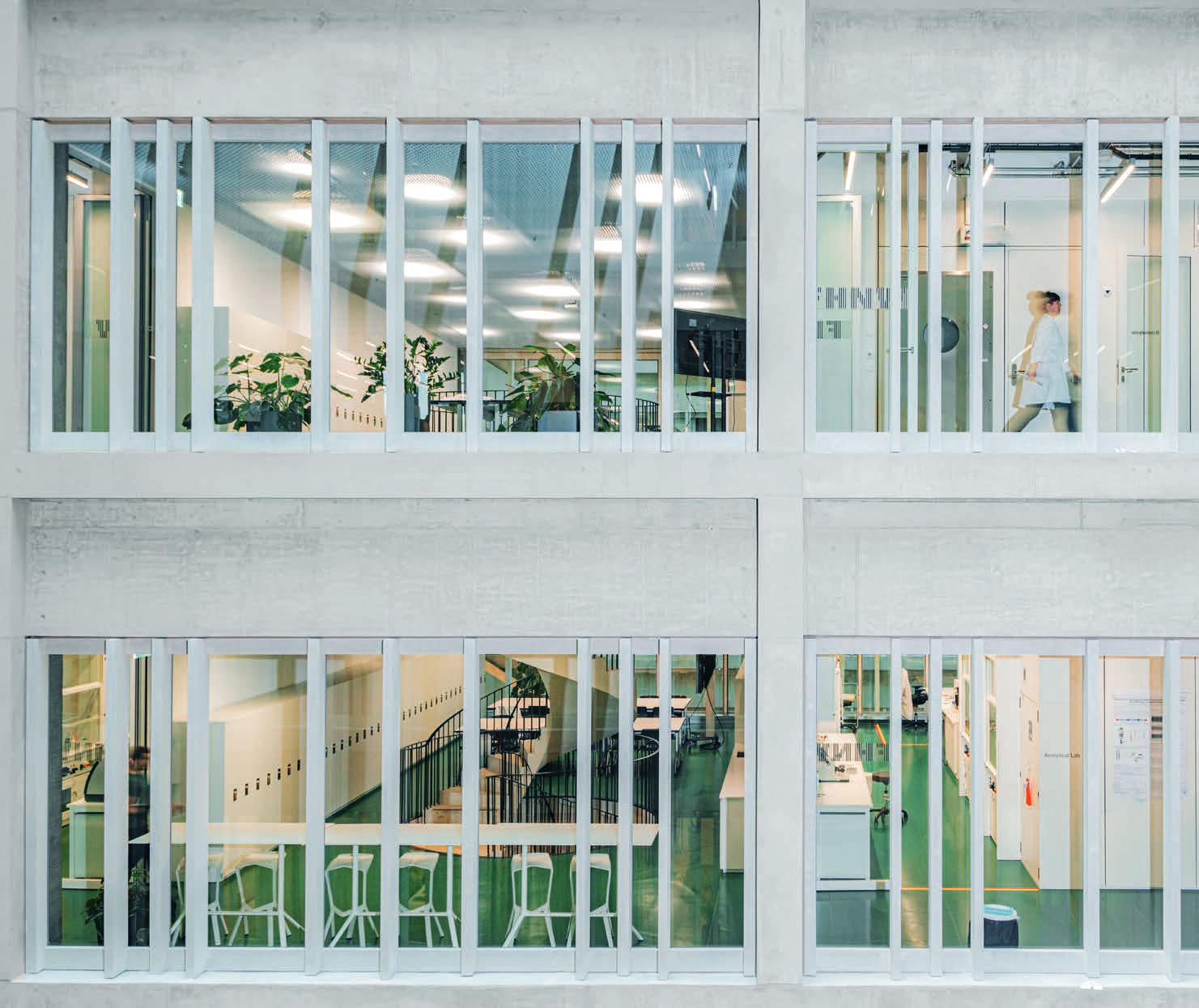
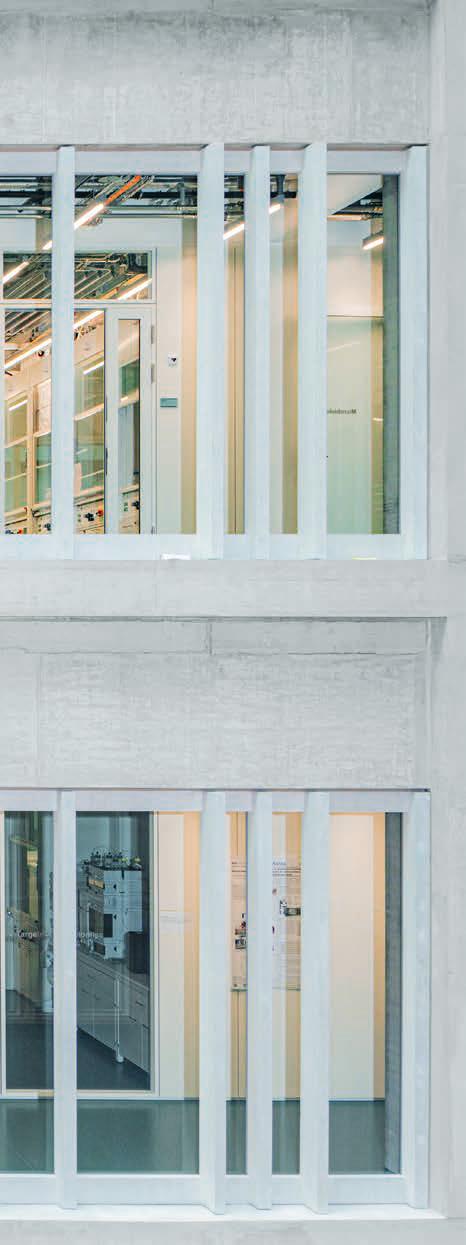
Ataglance
BasedinMuttenznearBasel,the FHNWSchoolofLifeSciencesispartof Europe’slargestlifesciencescluster. Itisherethatwetrainskilledspecialists andcomeupwithsolutionstothesocial andeconomicchallengesoftomorrow.
Ataglance: FHNWSchoolofLifeSciences
PEOPLE
300Staff Teaching,ResearchandSupport
800+Students
595BSc|232MSc 9International|70JointMaster's
100+ Participants inContinuingEducation
STUDYPROGRAMMES
11
DegreeProgrammes
8BSc|3MSc
30
ContinuingEducation MAS,CASandCourses
6Floors
atCampusMuttenz
5400m2
ofLaboratoryFacilities
577
ProjectsinCollaboration withNationaland International Industry
800+ LifeScience Companies
Process Technology Center
53 EuropeanProjects
32000+ LifeScience Jobs
AleadingUniversityof AppliedSciencesat theheartofEurope’slargest lifescienceregion
AtthenewCampusMuttenzintheheartofEurope’slargest lifesciencesregion,theFHNWSchoolofLifeSciencesdoes cutting-edgeresearchforabetterfuture.State-of-the-art infrastructureandequipment,includinganewProcessTechnologyCenter,enableourresearchersandindustrypartnersto worktogethertodevelopnewtechnologiesandproductsfrom concepttomarket.Thecampushasanideallocationcloseto publictransportandwithaviewoverBasel.
WhenyoucollaboratewiththeFHNWSchoolofLifeSciences, manyadvantagesawaityou.
Directcontacttoourapproachable expertsandtheirindustrialknowhow
Highlyqualifiedgraduateswithcloseto 100%placementratesinindustry
Efficientprojectexecution,inaccordancewith industrynormsandstandards
Moderncampusforcollegialexchangeand conferences,whereleadingmindsmeet
First-classlaboratoryandpilot-scale infrastructure
EstablishedrelationshipswiththeBaselArea LifeSciencescluster
Ourapplied researchexpertise
MedicalEngineeringand Informatics
Biofabrication
Additivemanufacturingofimplantsandorthopaedics
Diagnosticandtherapeuticdevicesandsensors
Computeraidedsurgeryandmedicaldecisionsupport
Medicalimageandsignalanalysis
AIandquantumcomputing
PharmaTechnologyand Biotechnology
Bioprocessing
Geneandcelltherapy
Drugformulation,deliveryandPK/PD
ChemistryandBioanalytics
Moleculardiagnostics
Proteins,enzymesandcellbiology
Nanotechnology,polymersandsurfaces
Chemistry,processes,andreactions
Analytics
Datascience
Ecopreneurship
Watertechnologies
Ecotoxicology
Circulareconomy
Imprint
Publisher
FHNWSchoolofLifeSciences
Projectmanagement,textandediting SoniaThomson,FHNWSchoolofLifeSciences
Proofreading
AndrewBrown,FHNWSchoolofLifeSciences
Design
AnDiCoLab,IDCEInstituteDigitalCommunicationEnvironments
Imagecredits
Leadphotographer:JoëlKarlin,FHNWSchoolofLifeScience
Coverpage,pages15 17,19,20,25 27,29,33,37,38, 43,45,47
p.3MathiasLeemann
p.4 5,9NicholasZonvi
p.7RitaHochstrat
p.10 11MarynaPeter
p.13FelixSchmidt
p.18Endress+Hauser
p.21Shutterstock,LoveEmployee
p.22 23OliverGermershaus
p.30 31AdobeStock,chandlervid85
p.35OrthopodoMalgaroli
p.39AdobeStock,Kenishirotie
p.40 41IBM
p.48PatiGrabowicz,Instagram:@patigrabowicz
Lithography
SturmAG
Printing
merkurmedienag
Copies
500English
Firstedition March2025
Statisticsonpage50 51asofMarch2025,sourcesFHNW
SchoolofLifeSciencesandLifeSciencesClusterBasel
TheFHNWincorporatestenschools:
–FHNWSchoolofAppliedPsychology
–FHNWSchoolofArchitecture,ConstructionandGeomatics
–FHNWBaselAcademyofArtandDesign
–FHNWSchoolofComputerScience
– FHNWSchoolofLifeSciences
–FHNWBaselAcademyofMusic
–FHNWSchoolofEducation
–FHNWSchoolofSocialWork
–FHNWSchoolofEngineeringandEnvironment
–FHNWSchoolofBusiness
FHNWUniversityofAppliedSciencesandArts
NorthwesternSwitzerland
SchoolofLifeSciences
Hofackerstrasse30
4132Muttenz
info.lifesciences@fhnw.ch
www.fhnw.ch/lifesciences