1
Assistant Professor of Swine Medicine and Reproduction, Farm Animals Clinic, School of Veterinary Medicine, Aristotle University of Thessaloniki, Greece Email: ptassis@vet.auth.gr
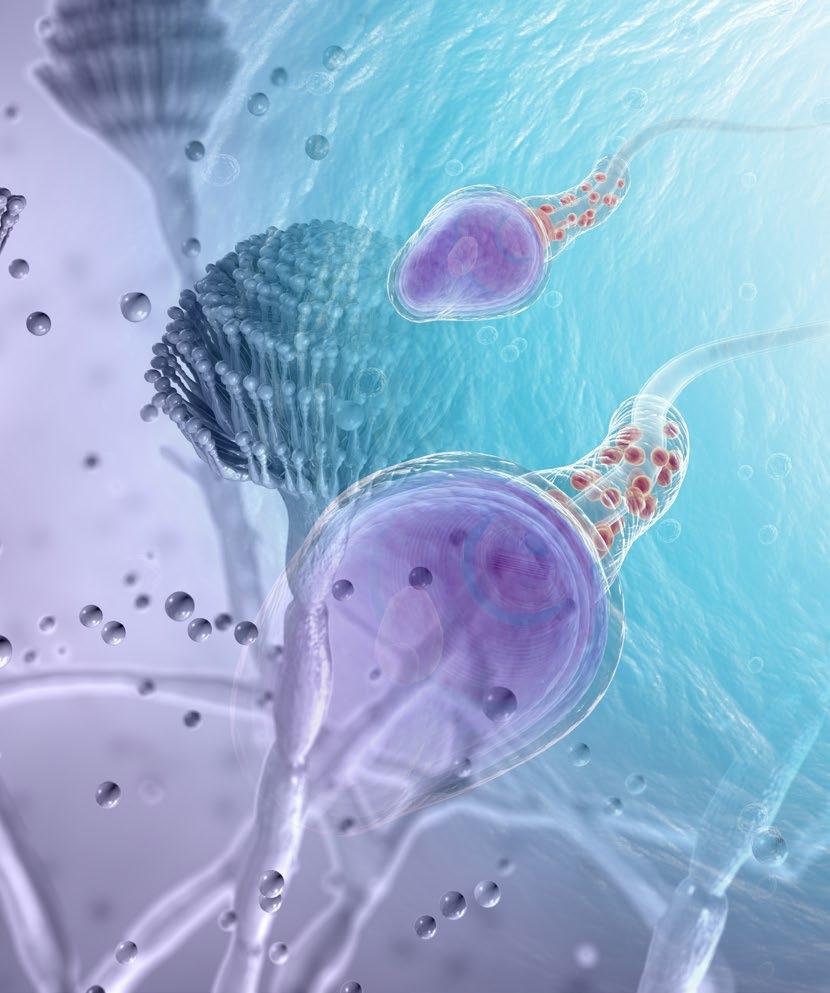
Panagiotis Tassis
THE EFFECTS OF MYCOTOXINS ON BOAR SEMEN
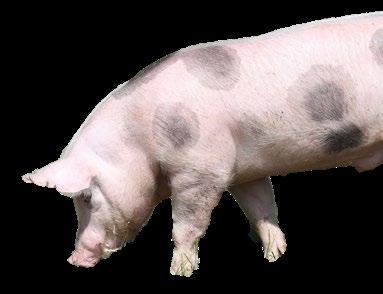
0.02 mg AFB1/kg feed 0.05 mg OTA/kg feed.
Aflatoxins (AFs, mainly AFB1), ergot alkaloids, fumonisins (FBs, mainly FB1), and trichothecenes (e.g., DON, T-2 toxin, nivalenol) ochratoxin A (OTA), and zearalenone (ZEN) are considered significant for their effects on boar health3,4
The significance of boar semen characteristics in the reproductive success and improvement of traits of economic importance (i.e., growth rate, feed conversion efficiency) in farrow-to-finish farms is undisputable8
0 1 mg ZEN/kg feed for piglets and gilts and 0.25 mg ZEN/kg feed for sows and fattening pigs, respectively.
Mycotoxins are secondary metabolites produced by certain fungi species (genera Aspergillus, Penicillium, Fusarium, Alternaria, and Claviceps) that can be found in grains worldwide.
Reproductive inefficiency is recognized as the costliest limiting constraint to efficient animal production9
2
0.9 mg DON/kg feed (complementary and complete feedstuffs).
80%
Among those, DON, ZEN and FB1 are the most frequently occurring mycotoxins produced by Fusarium species2
Data at a global scale show that up to 80% of feed and food crops are contaminated with mycotoxins, and that co-contamination of grains with more than one mycotoxin is common1,2
According to the European Commission5-7, the maximum recommended contamination levels for these mycotoxins in swine feed are:
0.25 mg T2 and HT2)/ kg compound feed.
5 mg FBs/kg feed.
They are produced before (fungi as plant pathogens) or after harvest of grains, as well as during storage (fungi growing saprophytically).
Nowadays, the demand for intensive reproductive performance with the use of hyperprolific sows can be fulfilled only with the utilization of high-quality boar semen that supports this challenging reproductive pace10
Results from previous studies with humans, rodents and domestic animals have provided evidence of deleterious effects of several contaminants on male gametes at various stages of spermatogenesis (i.e., in the testis) during passage through the epididymis, and in mature spermatozoa, after ejaculation and during capacitation11
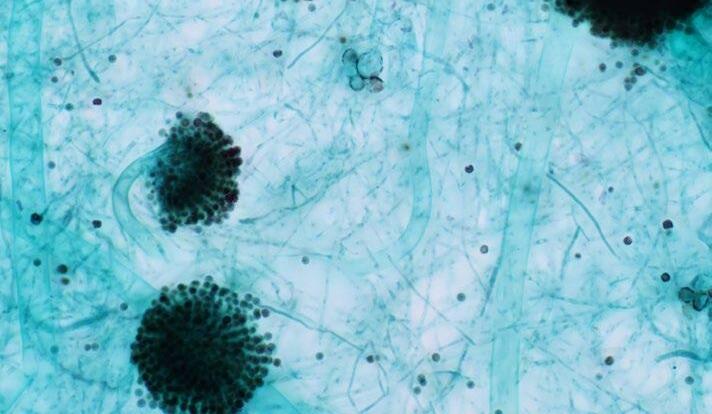
Approximately, 90% of swine reproduction has been performed with artificial insemination in the past two decades, 99% of which has been carried out with the use of liquid semen and ambient temperature extenders8,10
The present review will emphasize on the effects of the main mycotoxins on boar semen characteristics and boar fertility.
90%
3
The accumulation of sphinganine in the liver and kidneys has been associated with cell apoptosis and mitosis, leading to fibrosis and nodular hyperplasia, respectively18,19
FUMONISINS
DEOXYNIVALENOL
Diarrhea
Disruption of the intestinal barrier23 by affecting the tight junction function which means that, along with its ability to impair immune function, it can result in increased susceptibility of pigs to opportunistic pathogens24
DON predominantly inhibits protein elongation14. Clinical symptoms anticipated in cases of DON toxicosis include:
Major modes of action of significant mycotoxins in swine
4
Additional potential toxic effects of FB1 include:
Reduced feed intake
Vomiting and nausea
FB1 can inhibit sphingolipid biosynthesis through the inhibition of ceramide synthase16, resulting in impaired sphingolipid metabolism and a subsequent accumulation of sphinganine17
The above-mentioned significant mycotoxins for swine have specific modes of action and toxicokinetic properties that are under continuous investigation (reviewed by 12,13).
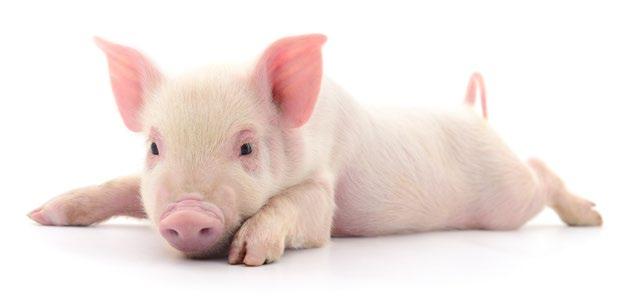
Cellular and Hyporexia,immunosuppressionhumoral20,21anddecreased weight gain22
Effects on intestinal function and immunosuppression have been also attributed to DON15
Hemorrhage/ necrosis
Emesis and diarrhea hemorrhagicNecrosis,ImmunosuppressionLethargyserous-necroticulcerative inflammation of the digestive tract
Leukopenia (leukocyte Hemorrhage,apoptosis) shock and death
TOXINT-2
T-2 toxin inhibits the initiation of the protein synthesis26, also affecting DNA and RNA synthesis thus hindering normal cell function27
Effects of AFB1 on cell function and integrity through the induction of oxidative stress have been also suggested37-39.
ZEARALENONE
Oral/dermal irritation
OTA has a quite complex mode of action that has not been fully explored yet. Possibly inhibition of protein synthesis and energy production, induction of oxidative stress, DNA adduct formation, as well as apoptosis/ necrosis and cell cycle arrest are involved in its toxic action30
As a highly unstable molecule, AFBO reacts with cellular macromolecules, including nucleic acids, proteins, and phospholipids, to induce various genetic, metabolic, signaling, and cell structure disruptions34-36
5
ZEN resembles 17β estradiol and, along with its metabolites (mainly αand β- zearalenol: α- and β- ZEL) are substrates for the estrogen receptors. It is capable of exerting estrogenic effects, impairing the reproductive system and fertility of pigs25
Renal toxicity is the predominant expression of OTA’s toxic potency, while it can also induce teratogenic, embryotoxic, genotoxic, neurotoxic, immunosuppressive and carcinogenic effects31,32
AFLATOXINS
ZEN toxicosis has been predominantly associated with reproductive disorders in sows and boars, along with effects on the intestine and immune system of pigs4
Aflatoxins exert various toxicological effects with different mechanisms, most of which are not yet fully elucidated.
It is considered to be more acutely toxic causing14,28,29:
The mutagenic effects of AFB1 are ascribed mainly to the intermediate metabolite AFB1-exo-8,9 epoxide (AFBO)33 AOCHRATOXIN
The effects of mycotoxins produced by Fusarium species (DON, ZEN, FBs, and T-2 toxin)
The effects of mycotoxins on boar semen characteristics and fertilizing ability
DON and ZEN are extremely significant as regards their impact on pigs and both have been shown to induce detrimental effects on the reproductive system of sows and boars4
Undoubtedly, mycotoxins pose a significant threat to the health and reproductive performance of swine.
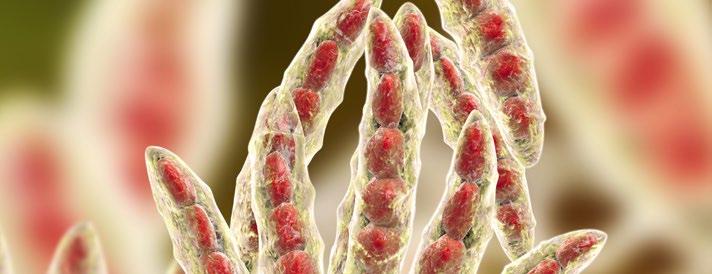
Although studies have reported effects of DON and T-2 toxin on sows, gilts, ovaries, follicular development, porcine oocytes and granulosa cells, there is lack of in vivo studies on their effects on boars41-44
6 AFLATOXINS
AFB1 has been linked with genotoxicity, immunotoxicity and acute intoxication by acting on genomic DNA, other functional macromolecules, and immunocompetent cells40
CELLSLEYDIGONTOXINT-2&ZENDON,OFEFFECTS
7
Moreover, ZEN and DON significantly reduced testosterone and progesterone secretion in Leydig cells (possible inhibition of the conversion of progesterone into testosterone), whereas T-2 toxin only reduced testosterone secretion
It should be emphasized that Leydig cells are very important for the maintenance of the male phenotype and spermatogenesis. Among their main functions are the synthesis and secretion of steroid hormones46, whilst they have increased susceptibility to mycotoxin contamination due to their high lipid content47
A recent in vitro study on the effects of DON, ZEN and T-2 toxin on porcine Leydig cells, demonstrated evidence of increased cell apoptosis, as well as disrupted mitochondria membrane potential and cell cycle distribution45
T-2 toxin was the most cytotoxic in Leydig cells followed by DON and ZEN, whereas all mycotoxins tested, exerted a cytotoxic effect on Leydig cells by increasing cell cycles at the G1 phase and preventing transition from the G1 to the S phase.
Results proved that exposure to ZEN, DON and T-2 toxins can result in reproductive toxicity involving the inhibition of steroidogenesis and cell proliferation, which contributes to the cellular apoptosis induced by mitochondrial injury in porcine Leydig cells.
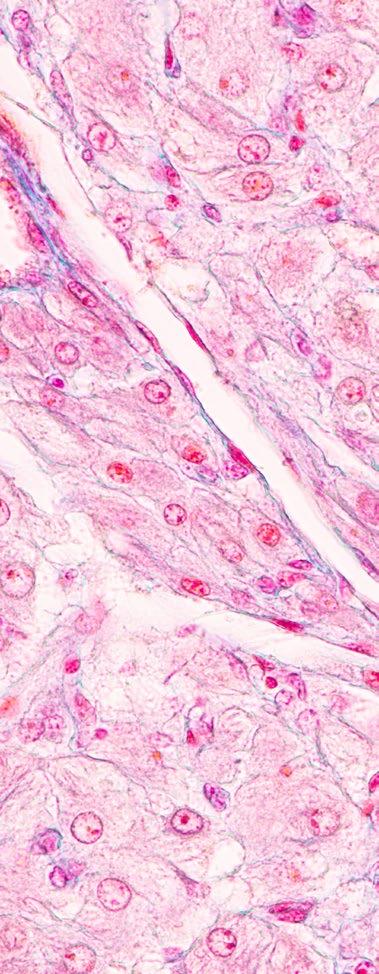
ZEN has been associated with alterations such as reduced:
In vitro studies have proven that ZEN and its major metabolites α- and β-ZEL can decrease sperm viability and progressive motility:
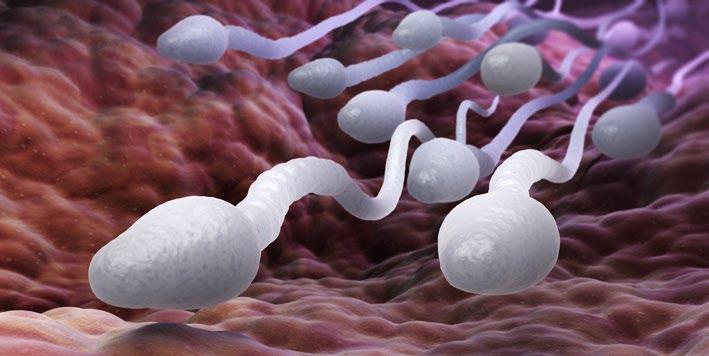
8
Serum testosterone levels and libido (feeding 40 mg ZEN/kg feed between 14 and 18 weeks of age)48
Previous studies have demonstrated the effects of ZEN on boar fertility and semen characteristics
CHARACTERISTICSSEMEN&FERTILITYBOARONZENOFEFFECTS
Spermatogenesis (9 mg ZEN/ kg feed from 32 days of age up to 145 or 312 days of age)50
ZEN, α-ZEL, β-ZEL at 1 Χ 10-8 and 1 M or 2 Χ 10-7 and 20 μM for 5, 16, 24 or 48 h53
ZEN and α-ZEL at 125, 187.5 or 250 μM54
Additionally, ZEN and α-ZEL can reduce the ability of boar spermatozoa to bind to the zona pellucida (125.6, 188.4, and 251.2 μM ZEN, as well as 124.8, 187.2, and 249.6 μM α-ZEL for 1, 2, 3 or 4 h)55 and affect sperm chromatin integrity (31.4, 62.8, and 94.2 μM ZEN, as well as a-ZEL at levels of 31.2, 62.4, and 93.6 μM for 4 h)56
Testis weights (30% reduced weight after ingestion of 0.5–0.6 mg ZEN/kg feed for 64 days)49
However, some feeding experiments with conventional or wild boars showed either lack of harmful effects or a transient decrease on boar semen motility parameters51,52
It seems that α-ZEL, which is the main ZEΝ metabolite in pigs, was more active in denaturing chromatin structure, while ZEΝ had greater toxic potency on semen viability. β-ZEL exclusively affected motility parameters.
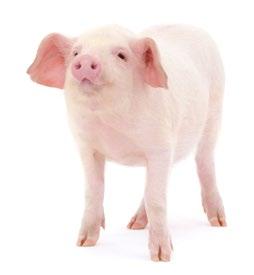
On the other hand, it has been demonstrated that, due to its structural resemblance with 17β-estradiol, ZEN can bind to estrogen receptors in various tissues, whereas α-ZEL binds more effectively to the receptors than the parent toxin57
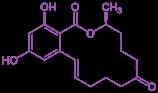
9 CHARACTERISTICSSEMEN&FERTILITYBOARONZENOFEFFECTS
According to Benzoni et al 53, ZEN, α- and β-ZEL influence a particular function of spermatic cells.
It seems that detrimental effects on boar fertility could be the outcome of the interaction between ZEN or α- and β-ZEL and estrogen receptors found in boar spermatozoa58.
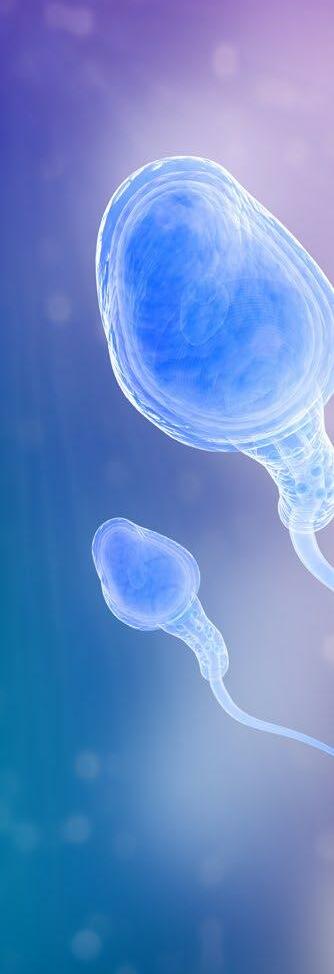
10 SEMENBOARONDON&ZENOFEFFECTS
Our group recently evaluated the effects of DON and ZEN alone or in combination, as well as the effects of their modified forms, namely deepoxy-DON (DOM-1) and hydrolyzed zearalenone (HZEN) on boar semen in vitro59,60
Results proved that concentrations of 50.6 μM DON and 62.8 μM ZEN induced significant negative effects on boar semen characteristics in vitro suggesting a negative effect of these mycotoxins on boar fertility.
Results of computer-assisted semen analysis (CASA) evaluations (time-points of semen observations were 0, 1, 2, 3, 4 hours after mycotoxin exposure) suggested that a greater number of immotile and non-progressive motile spermatozoa were present in all mycotoxin-exposed groups, (i.e., DON, ZEN, or DON + ZEN group) irrespective of incubation time.
Reduction of viable spermatozoa was observed after ZEN exposure, either alone or in combination with DON (DON-alone effect was present at the 4th hour of observations).
Evaluation of the effects of combined mycotoxins exposure on all parameters suggested less than additive effects on the vast majority of parameters, with an exception of a synergistic effect on viability and two CASA parameters (i.e., rapid moving spermatozoa and curvilinear velocity). Taken together, DON and ZEN either alone or in combination can be considered as harmful to boar semen characteristics59
When applied in combination, DON and ZEN exerted a greater effect on many tested parameters in vitro when compared with single DON or ZEN exposure.
Hypoosmotic swelling test (HOST) results demonstrated that both toxins, alone and in combination, can negatively affect spermatozoa membrane integrity. On the contrary, absence of effects on DNA integrity was reported in all types of exposure59
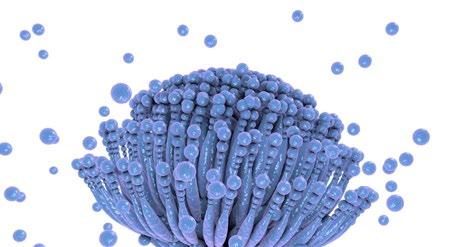
These results support the fact that conversion of DON to DOM-1 and ZEN to HZEN represents a detoxification process60
FB1 at contamination levels of 5, 10 or 15 mg/kg feed in pigs after 6 months of contaminated feed ingestion (start of feeding at 8-9 weeks of age) can be associated with reduced vigor and reproductive performance in boars.
11
Dietary FB1 above 5 mg/kg has been reported to delay attainment of sexual maturity in growing pigs9, reducing testicular and epididymal sperm reserves as well as the daily sperm production and semen quality in boars, with potential to impair fertility61,62
It was demonstrated that modified forms did not elicit impairment of motility, morphology, or viability of spermatozoa, and they did not induce negative effects on the integrity of spermatozoa membrane or nuclear chromatin.
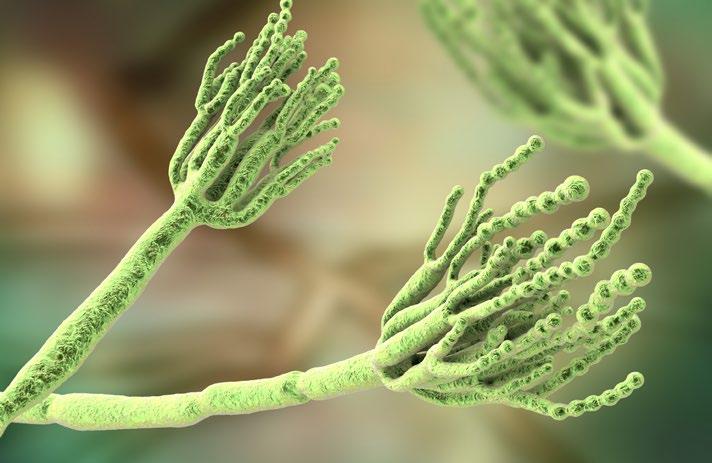
FBs have been also linked with effects on boar semen.
The results of the comparison between the effects induced on boar semen after in vitro exposure to DON and ZEN at the aforementioned levels (50.6 μM DON and 62.8 μM ZEN, respectively) and exposure to equimolar concentrations of their modified mycotoxins DOM-1 and HZEN provided further evidence.
SEMENBOARONFBsOFEFFECTS
Initial forward motility
FERTILITYBOARONOTAOFEFFECTS
Effects of mycotoxins produced by Aspergillus and Penicillium species (AFBs & OTA)
FERTILITYBOARONOTAOFEFFECTS
Moreover, boars with greater AFB1 levels in semen had fertility disorders, lower spermatozoa survival rate, as well as increased proportion of abnormal spermatozoa.
Sperm viability
FERTILITYBOARONAFLATOXINSOFEFFECTS
A later study hypothesized that OTA might spermatogenesisaffect since the length of porcine spermatogenetic cycle is 39 days and the results suggested inferior sperm quality approximately 40 days after starting toxin-feeding period.
In another in vivo study66, it was reported that OTA might have the potential to affect sperm production and boar semen quality by inducing reduction of initial motility and longevity of spermatozoa.
The effects of OTA have been assessed in a study with boars (250 kg weight), which received 0.08 μg/kg OTA orally for 6 weeks65. Results indicated a reduction of:
Motility after 24 hours of storage
12
OTA is capable of inhibiting protein synthesis (interfering with phenylalanyl-tRNA synthetase that may result in modification at the post-transcriptional level of specific mRNAs) and this specific mode of action could be also present during spermatogenesis, resulting in a disturbance that would induce abnormal stability of spermatozoa membrane65,66
The excretion of afatoxins in boar semen has been demonstrated along with their possible adverse effects on boar reproductive efficiency63
In a previous 12-month evaluation study, AFB1 concentrations in the seminal plasma of 21 boars of four breeds had been assessed. Results supported a seasonal increase of AFB1 concentrations in seminal plasma which was related to respective AFB1 levels in feed.
According to Zhang et al 68 decreased boar semen motility was observed at exposure level of 100 μM OTA for 24 hours, whereas reactive oxygen species (ROS) were significantly increased after 10 or 100 μM OTA exposure, and increased expression of apoptosis markers was observed in the OTA exposed sperm.
Based on the findings of this study OTA exposure affects sperm motility via the activated protein kinase (AMPK) and the phosphatase and tensin homolog deleted on chromosome ten (PTEN) signaling pathways.
Moreover, ROS generation in sperm may activate PTEN which, in turn, leads to diminished AKT (protein kinase B) and AMPK, and results in a decline in sperm motility69
Carryover effects on the developing embryo
Ewald and Heer67 suggested a decrease in boar sperm production and semen quality after OTA ingestion as a result of increased instability of the sperm membrane.
Oocyte activation
Zygote formation
Interactions during fertilization, include the delivery of paternal components from the spermatozoon into the oocyte. Such components are critical for70:
FERTILITYBOARONOTAOFEFFECTS
13
Further embryonic development
14
Findings, such as those from transcriptome profiles of cattle blastocysts derived from in vitro fertilization procedures with AFB1-treated spermatozoa72 are indicative of the need for further examination of the paternal carryover effect on the developing embryo.
Results from that study, demonstrated the presence of a large number of mRNA transcript genes in blastocysts developed after fertilization with treatedspermatozoa compared with untreated spermatozoa, thus a possible carryover effect from the spermatozoa to the developing embryo was suggested.
Paternal components that could be affected include DNA, epigenetic markers, RNA, and proteins71
Therefore, it could be deduced that environmental contaminants (which enter via feed into the body and are transported to the reproductive tract) that are able to modify paternal components, could subsequently affect the embryo at later developmental stages.
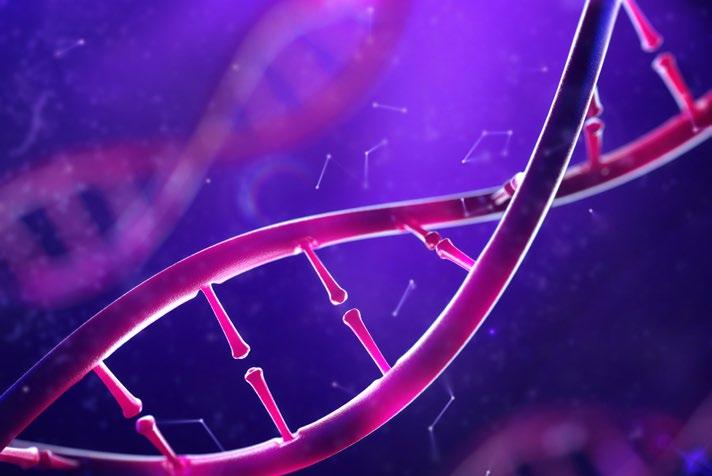
Therefore, a significant need for further in vitro and in vivo studies on the effects of mycotoxins, alone or in combination, is obvious, with emphasis on underlying cellular and molecular mechanisms that lead to impairment of boar semen characteristics
Furthermore, considering that occurrence and co-occurrence of mycotoxins is common in feed2, increased caution in minimizing the risk of mycotoxicosis on farm is critical for boar health and spermatogenesis.
15
CONCLUSIONS
by which mycotoxins impair spermatozoa seem to be multifactorial.
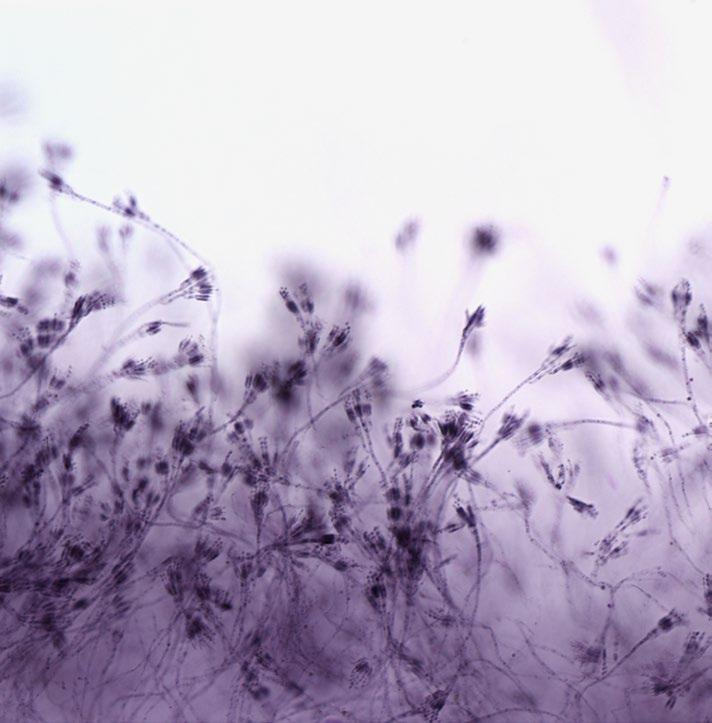
Although reprotoxicity of the afore-mentioned major mycotoxins is gradually revealed, it is obvious that there is a lack of evidence on the effects of several other mycotoxins, including emerging mycotoxins, on swine reproduction. Moreover, possible interactions among mycotoxins on spermatogenesis and boar fertility have not been adequately investigatedThemechanisms
A range of studies have proved in vitro and in vivo effects of various mycotoxins in boars and boar semen that could explain reduced fertility and impairment of reproductive performance in field conditions.
7. European Commission (EC).. Commission recommendation of 17 August 2006 on the presence of deoxynivalenol, zearalenone, ochratoxin A, T-2 and HT-2 and fumonisins in products intended for animal feeding. Off. J. Eur. Union. 2006, 229:0007–0009.
14. Ueno, Y. Toxicological features of T-2 toxin and related trichothecenes. Fund. Appl. Toxicol. 1984, 4, S124–132 2 PART 2.
16.2021Wang E, Norred WP, Bacon CW, Riley RT, Merrill AH Jr. Inhibition of sphingolipid biosynthesis by fumonisins. Implications for diseases associated with Fusarium moniliforme. J Biol Chem. 1991 Aug 5;266(22):14486-90.
15. Hooft JM, Bureau DP. Deoxynivalenol: Mechanisms of action and its effects on various terrestrial and aquatic species. Food Chem Toxicol.
24. Oswald, I.P.; Desautels, C.; Laffitte, J.; Fournout, S.; Peres, S.Y.; Odin, M.; Le Bars, P.; Le Bars, J.; Fairbrother, J.M. Mycotoxin Fumonisin B1
REFERENCES
13.Mar;137:111140.HolandaDM, Kim SW. Mycotoxin Occurrence, Toxicity, and Detoxifying Agents in Pig Production with an Emphasis on Deoxynivalenol. Toxins (Basel). 2021 Feb 23;13(2):171.
2. Gruber-Dorninger, C.; Jenkins, T.; Schatzmayr, G. Global Mycotoxin Occurrence in Feed: A Ten-Year Survey. Toxins 2019, 11, 375.
21. Marin, D.E.; Gouze, M.-E.; Taranu, I.; Oswald, I.P. Fumonisin B1 alters cell cycle progression and interleukin-2 synthesis in swine peripheral blood mononuclear cells. Mol. Nutr. Food Res. 2007, 51, 1406–1412.
23. Bouhet, S.; Hourcade, E.; Loiseau, N.; Fikry, A.; Martinez, S.; Roselli, M.; Galtier, P.; Mengheri, E.; Oswald, I.P. The my-cotoxin fumonisin B1 alters the proliferation and the barrier function of porcine intestinal epithelial cells. Toxicol. Sci. 2004, 77, 165–171.
1. Eskola, M.; Kos, G.; Elliott, C.T.; Hajšlová, J.; Mayar, S.; Krska, R.Worldwide contamination of food-crops with mycotoxins: Validity of the widely cited ‘FAO estimate’ of 25%. Crit. Rev. Food Sci. Nutr. 2019.
5. European Commission (EC). Directive 2002/32/EC of the European Parliament and of the Council of 7 May 2002 on undesirable substances in animal feed. Off. J. Eur. Union. 2002, 32:1–22.
10. Rodriguez, A.L.; Van Soom, A.; Arsenakis, I.; Maes, D. Boar management and semen handling factors affect the quality of boar extended semen. Porc. Health Manag. 2017, 3, 15.
3. Althouse, G.C. Applied Andrology in Swine. In Animal Andrology: Theories and Applications; Chenoweth, P.J., Lorton, S.P., Eds.; CABI: Oxfordshire, UK, 2014; pp. 404–417.
22. Bouhet, S.; Oswald, I.P. The intestine as a possible target for fumonisin toxicity. Mol. Nutr. Food Res. 2007, 51, 925–931.
12. Schelstraete W, Devreese M, Croubels S. Comparative toxicokinetics of Fusarium mycotoxins in pigs and humans. Food Chem Toxicol. 2020
8. Peltioniemi, O.A.; Kemp, B. Infertility in the Pig and the Control of Pig Herd Fertility. In Veterinary Reproduction and Obstetrics, 10th ed.; Noakes, E.D., Parkinson, T.J., England, G.C.W., Eds.; Elsevier: Amsterdam, The Netherlands, 2019; pp. 581–592.
9. Gbore F.A. Growth performance and puberty attainment in growing pigs fed dietary fumonisin B1. Journal of Animal Physiology and Animal Nutrition, 2009a, 93: 761-767.
4. Ensley, S.M.; Radke, S.L. Mycotoxins in Grains and Feeds. In Disease of Swine, 11th ed.; Zimmerman, J.J., Karriker, L.A., Ramirez, A., Schwartz, K.J., Stevenson, G.W., Zhang, J., Eds.; Wiley-Blackwell: Hoboken, NJ, USA, 2019; pp. 1055–1071.
16
17. Enongene, E.N.; Sharma, R.P.; Bhandari, N.; Miller, J.D.; Meredith, F.I.; Voss, K.A.; Riley, R.T. Persistence and reversibility of the elevation in free sphingoid bases induced by fumonisin inhibition of ceramide synthase. Toxicol. Sci. 2002, 67, 173–181.
20. Marin, D.E.; Taranu, I.; Pascale, F.; Lionide, A.; Burlacu, R.; Bailly, J.-D.; Oswald, I.P. Sex-related differences in the immune response of weanling piglets exposed to low doses of fumonisin extract. Br. J. Nutr. 2006, 95, 1185–1192.
19.413–417.Voss,K.A.; Plattner, R.D.; Riley, R.T.; Meredith, F.I.; Norred, W.P. In vivo effects of fumonisin B1-producing and fumonisin B1-nonproducing Fusarium moniliforme isolates are similar: Fumonisins B2 and B3 cause hepato- and nephrotoxicity in rats. Mycopathologia 1998, 141, 45–57.
18. Casteel, S.W.; Turk, J.R.; Cowart, R.P.; Rottinghaus, G.E. Chronic Toxicity of Fumonisin inWeanling Pigs. J. Veter Diagn. Investig. 1993, 5,
11. Komsky-Elbaz A, Kalo D, Roth Z. New evidence for deleterious effects of environmental contaminants on the male gamete. Anim Reprod Sci. 2021 Nov 6:106886.
6. European Commission (EC). Recommendations on the presence of T-2 and HT-2 toxin in cereals and cereal products. Off J Eur Union, 2013, 56, 12–15 L 91.
50. Young, L.G.; King, G.J. Low concentrations of zearalenone in diets of boars for a prolonged period of time. J. Anim. Sci. 1986, 63, 1197–1200.
38. Klaunig, J.E.; Kamendulis, L.M.; Hocevar, B.A. Oxidative stress and oxidative damage in carcinogenesis. Toxicol. Pathol. 2009, 38, 96–109.
34. Garner, R.C.;Wright, C.M. Binding of [14C]aflatoxin B1 to cellular macromolecules in the rat and hamster. Chem. Biol. Interact. 1975, 11, 35.123–131.Zhuang, Z.; Huang, Y.; Yang, Y.; Wang, S. Identification of AFB1-interacting proteins and interactions between RPSA and AFB1. J. Hazard. Mater. 2016a, 301, 297–303.
32. Yang S, Zhang H, De Saeger S, De Boevre M, Sun F, Zhang S, Cao X., Wang Z. In vitro and in vivo metabolism of ochratoxin A: a comparative study using ultra-performance liquid chromatography-quadrupole/time-of-flight hybrid mass spectrometry. Anal Bioanal Chem 2015, 33.407(13):3579-89.Benkerroum, N. Retrospective and prospective look at aflatoxin research and development from a practical standpoint. Int. J. Environ. Res. Public Health 2019, 16, 3633.
49. Christensen, C.M.; Mirocha, C.J.; Nelson, G.H.; Quast, J.F. Effect of young swine of consumption of rations containing corn invaded by Fusarium roseum. Appl. Microbiol. 1972, 23, 202.
43. Cortinovis C, Pizzo F, Spicer LJ, Caloni F. Fusarium mycotoxins: effects on reproductive function in domestic animals--a review. Theriogenology. 2013 Oct 1;80(6):557-64.
Increases Intestinal Colonization by Pathogenic Escherichia coli in Pigs. Appl. Environ. Microbiol. 2003, 69, 5870–5874.
30. Kőszegi T, Poór M. Ochratoxin A: Molecular Interactions, Mechanisms of Toxicity and Prevention at the Molecular Level. Toxins (Basel). 2016 Apr 15;8(4):111.
31. Klarić MS, Rašić D, Peraica M. Deleterious effects of mycotoxin combinations involving ochratoxin A. Toxins 2013, 5(11):1965-87.
37. Bedard, L.L.; Massey, T.E. Aflatoxin B1-induced DNA damage and its repair. Cancer Lett. 2006, 241, 174–183.
40. Benkerroum N. Chronic and Acute Toxicities of Aflatoxins: Mechanisms of Action. Int J Environ Res Public Health. 2020 Jan 8;17(2):423.
45. Sun, L.; Dai, J.; Xu, J.; Yang, J.; Zhang, D. Comparative Cytotoxic Effects and Possible Mechanisms of Deoxynivalenol, Zearalenone and T-2 Toxin Exposure to Porcine Leydig Cells In Vitro. Toxins 2022, 14, 113.
28. Ueno, Y., 1977. Mode of action of trichothecenes. Pure Appl. Chem. 49 (4–6), 1737–1745.
27. Sundstøl Eriksen, G., 2003. Metabolism and Toxicity of Trichothecenes. Doctoral Dissertation. Uppsala University, Uppsala, Sweden Department of Animal Nitrition and Management. Retrieved from. https://pub.epsilon.slu.se/287/.
36. Rushing, B.R.; Selim, M.I. Structure and oxidation of pyrrole adducts formed between aflatoxin B2a and biological amines. Chem. Res. Toxicol. 2017, 30, 1275–1285.
46. Tremblay, J.J. Molecular regulation of steroidogenesis in endocrine Leydig cells. Steroids 2015, 103, 3–10.
25. Zinedine, A., Soriano, J.M., Moltó, J.C., Mañes, J. Review on the toxicity, occurrence, metabolism, detoxification, regulations and intake of zearalenone: an oestrogenic mycotoxin. Food Chem. Toxicol. 2007, 45 (1), 1–18.
41. Caloni, F.; Ranzenigo, G.; Cremonesi, F.; Spicer, L.J. Effects of a trichothecene, T-2 toxin, on proliferation and steroid production by porcine granulosa cells. Toxicon 2009, 54, 337–344.
26. Smith, K.E., Cannon, M., Cundliffe, E., 1975. Inhibition at the initiation level of eukaryotic protein synthesis by T-2 toxin. FEBS Lett. 50 (1), 2–6.
39. Ayala, A.; Munoz, M.F.; Arguelles, S. Lipid peroxidation: Production, metabolism, and signaling mechanisms of malondialdehyde and 4-hy droxy-2-nonenal. Oxid. Med. Cell. Longev. 2014, 2014, 360438.
44. Zhang Y, Han J, Zhu CC, Tang F, Cui XS, Kim NH, Sun SC. Exposure to HT-2 toxin causes oxidative stress induced apoptosis/autophagy in porcine oocytes. Sci Rep. 2016b; 6:1-8.
29. Adhikari M, Negi B, Kaushik N, Adhikari A, Al-Khedhairy AA, Kaushik NK, Choi EH. T-2 mycotoxin: toxicological effects and decontamination strategies. Oncotarget. 2017, 8(20), 33933-33952.
47. Granum, C.; Anchersen, S.; Karlsson, C.; Berg, V.; Olsaker, I.; Verhaegen, S.; Ropstad, E. Steroidogenic differential effects in neonatal porcine Leydig cells exposed to persistent organic pollutants derived from cod liver oil. Reprod. Toxicol. 2015, 11, 130–139.
42. Kanora A, Maes D. The role of mycotoxins in pig reproduction: A review. Vet Med-Czech. 2009; 54: 565- 576.
17
48. Berger, T.; Esbenshade, K.L.; Diekman, M.A.; Hoagland, T.; Tuite, J. Influence of prepubertal consumption of zearalenone on sexual development of boars. J. Anim. Sci. 1981, 53, 1559–1564.
58. Rago V, Aquila S, Panza R, Carpino A. Cytochrome p450arom, androgen and estrogen receptors in pig sperm. Reprod Biol Endocrinol 2007;5:23, doi:10.1186/1477-7827-5-23.
18
62. Gbore F.A., Egbunike G.N.. Testicular and epididymal sperm reserves and sperm production of pubertal boars fed dietary fumonisin B1. Animal Reproduction Science, 2008, 105: 392-397.
72. Komsky-Elbaz, A., Kalo, D., Roth, Z. Effect of aflatoxin B1 on bovine spermatozoa’s proteome and embryo’s transcriptome. Reproduction, 2020, 160.
55. Tsakmakidis, I.A.; Lymberopoulos, A.G.; Vainas, E.; Boscos, C.M.; Kyriakis, S.C.; Alexopoulos, C. Study on the in vitro effect of zearalenone and alpha-zearalenol on boar sperm-zona pellucida interaction by hemizona assay application. J. Appl. Toxicol. 2007, 27, 498–505.
70. Dogan, S., Vargovic, P., Oliveira, R., Belser, L.E., Kaya, A., Moura, A., Sutovsky, P., Parrish, J., Topper, E., Memili, E., 2015. Sperm protamine-sta tus correlates to the fertility of breeding bulls. Biol. Reprod. 92, 92.
64. Kourousekos G. D., Theodosiadou E. Κ. (2018). Effects of aflatoxins on male reproductive system: A review. Journal of the Hellenic Veterinary Medical Society, 66(4), 201–210.
59. Tassis, P.D.; Reisinger, N.; Nagl, V.; Tzika, E.; Schatzmayr, D.; Mittas, N.; Basioura, A.; Michos, I.; Tsakmakidis, I.A. Comparative Effects of Deoxynivalenol, Zearalenone and Its Modified Forms De-Epoxy-Deoxynivalenol and Hydrolyzed Zearalenone on Boar Semen In Vitro. Toxins 2022, 14, 497. https://doi.org/10.3390/toxins14070497
63. Picha J, Cerovsky J, Pichova D (1986) Fluctuation in the concentration of sex steroids and aflatoxin B1 in the seminal plasma of boars and its relation to sperm production. Vet Med 1986, 31:347-357.
65. Biró K., Barna-Vetró I., Pécsi T., Szabó E., Winkler G., Fink-Gremmels J., Solti L. Evaluation of spermatological parameters in ochratoxin A— challenged boars. Theriogenology 2003, 60(2),199-207.
67. Ewald, C., Heer, A., 1989. The sperm quality of 4 boars during the assimilation of mycotoxin- containing feed (ochratoxin A) after changing the feed—a case study. Berl. Munch. Tierarztl. Wochenschr. 102, 261–266.
51. Sutkeviciene N., B. Bakutis, A. Banys, B. Karveliene, A. Rutkauskas, J. Sabeckiene, Zilinskas H. The effect of the estrogenic mycotoxin zearalenone on boar reproductive potencial and the dynamic of aspartate aminotransferase and alanine aminotransferase levels in the boar blood serum. Veterinarija Ir Zootechnika (Vet. Med. Zoot.), 2009;46(68): 73-77.
56. Tsakmakidis, I.A.; Lymberopoulos, A.G.; Khalifa, T.A.; Boscos, C.M.; Saratsi, A.; Alexopoulos, C. Evaluation of zearalenone and alpha zearalenol toxicity on boar sperm DNA integrity. J. Appl. Toxicol. 2008, 28, 681–688.
54. Tsakmakidis, I.A.; Lymberopoulos, A.G.; Alexopoulos, C.; Boscos, C.M.; Kyriakis, S.C. In vitro effect of zearalenone and alpha-zearalenol on boar sperm characteristics and acrosome reaction. Reprod. Domest. Anim. 2006, 41, 394–401.
57. Tiemann U, Dänicke S. In vivo and in vitro effects of the mycotoxins zearalenone and deoxynivalenol on different non-reproductive and reproductive organs in female pigs: a review. Food Addit Contam. 2007 Mar;24(3):306-14.
60. Tassis, P.D.; Tsakmakidis, I.A.; Nagl, V.; Reisinger, N.; Tzika, E.; Gruber-Dorninger, C.; Michos, I.; Mittas, N.; Basioura, A.; Schatzmayr, D. Individual and Combined In Vitro Effects of Deoxynivalenol and Zearalenone on Boar Semen. Toxins 2020, 12, 495.
68. Zhang TY, Wu RY, Zhao Y, Xu CS, Zhang WD, Ge W, Liu J, Sun ZY, Zou SH, Shen W. Ochratoxin A exposure decreased sperm motility via the AMPK and PTEN signaling pathways. Toxicol Appl Pharmacol. 2018 Feb 1;340:49-57.
61. Gbore F.A. Reproductive organ weights and semen quality of pubertal boars fed dietary fumonisin B1. Animal, 2009b, 3: 1133-1137.
69. Zhao, Y., Zhang, W.D., Liu, X.Q., Zhang, P.F., Hao, Y.N., Li, L., Chen, L., Shen, W., Tang, X.F., Min, L.J., Meng, Q.S., Wang, S.K., Yi, B., Zhang, H.F., 2016. Hydrogen sulfide and/or ammonia reduces spermatozoa motility through AMPK/AKT related pathways. Sci. Rep. 6 (37884).
71. Castillo, J., Jodar, M., Oliva, R., 2018. The contribution of human sperm proteins to the development and epigenome of the preimplantation embryo. Hum. Reprod. Update 24, 535–555.
53. Benzoni, E.; Minervini, F.; Giannoccaro, A.; Fornelli, F.; Vigo, D.; Visconti, A. Influence of in vitro exposure to mycotoxin zearalenone and its derivatives on swine sperm quality. Reprod. Toxicol. 2008, 25, 461–467.
66. Solti L., Pécsi T., Barna-Vetró I., Szász F., Biró K., Szabó E., Analysis of serum and seminal plasma after feeding ochratoxin A with breeding boars. Anim. Reprod. Sci. 1999, 56:2, 123-132.
52. Bielas W., W. Nizanski, J. Nicpon, J.E. Nicpon, A. Partyka, R. Mordak, M. Nowak, R. Ciaputa. Effect of zearalenone on circulating testosterone concentration, testicular and epididymal morphology and epididymal sperm characteristics in wild boars. Theriogenology 2017, 102 59-66.