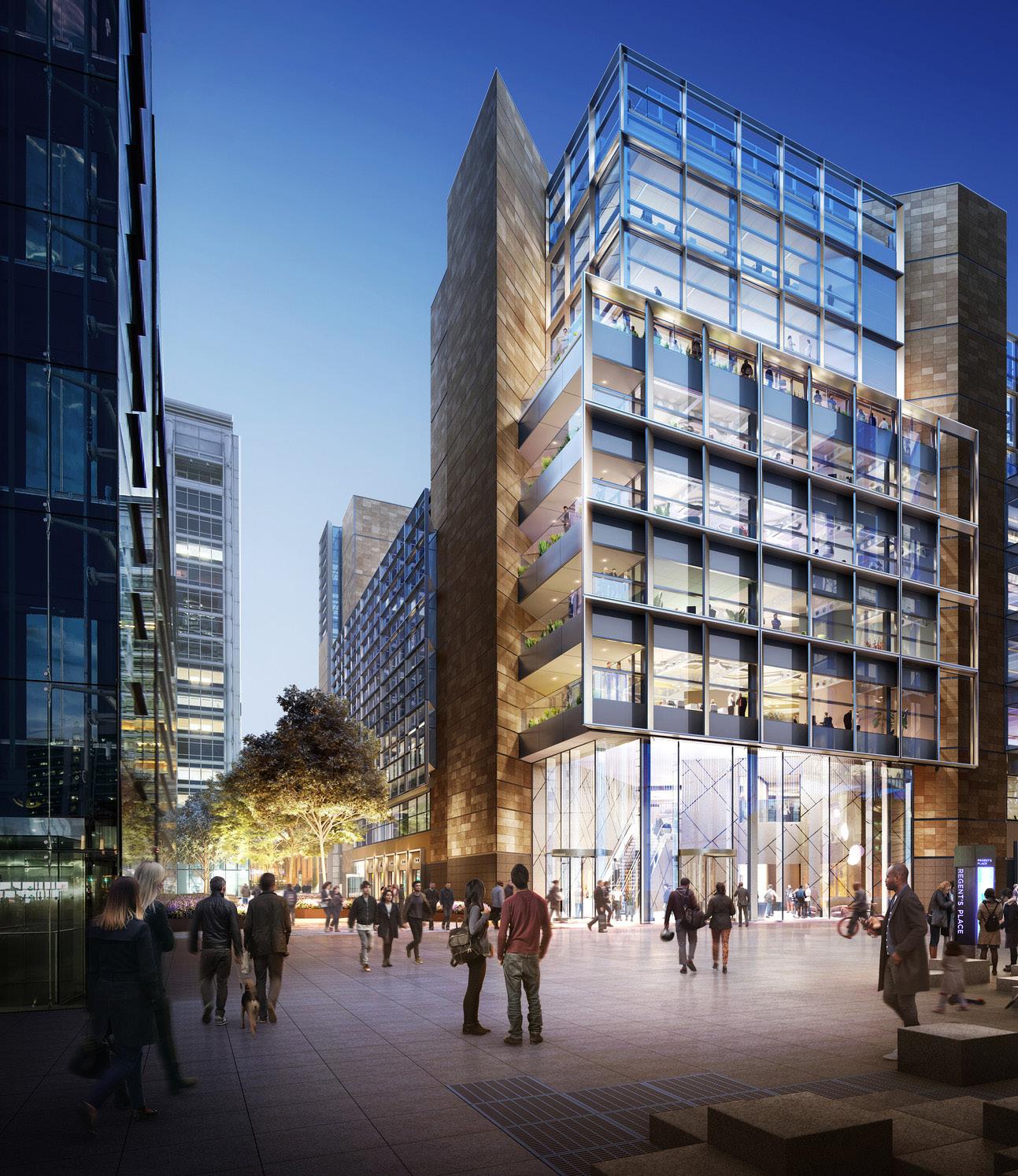
14 minute read
THE CONSEQUENCES OF CARBON - WHAT IS RESPONSIBLE INNOVATION? Graham Dodd - (Arup Fellow), Giovanni Zemella - (Associate) and Laura Solarino - (Façade Engineer), Arup
(fig 8. 1 Triton Square) © DBOX for Arup
The Consequences of Carbon
Advertisement
- what is responsible innovation?
“What should our designs try to achieve? We must take a critical look at the brief, make it more comprehensive. We must look beyond the narrow object and ask ourselves: What will be the ecological consequences? Ove Arup in 1972
Nearly 50 years later, as an industry, are we yet to fully understand what the ecological consequences of our actions are? It can be challenging to relate the UN Sustainable Development Goals to a façade panel and understand how, in this assembly of metal, glass and gaskets, we can make the significant contributions that are needed now. Still more challenging to plan how we are going to decarbonise the making of the built environment over the next few years while transitioning to a circular or doughnut economy. (fig 1. Opportunities in the circular lifecycle beyond carbon targets)
The Consequences of Carbon
- what is responsible innovation?
(fig 1. Opportunities in the circular lifecycle beyond carbon targets © Arup
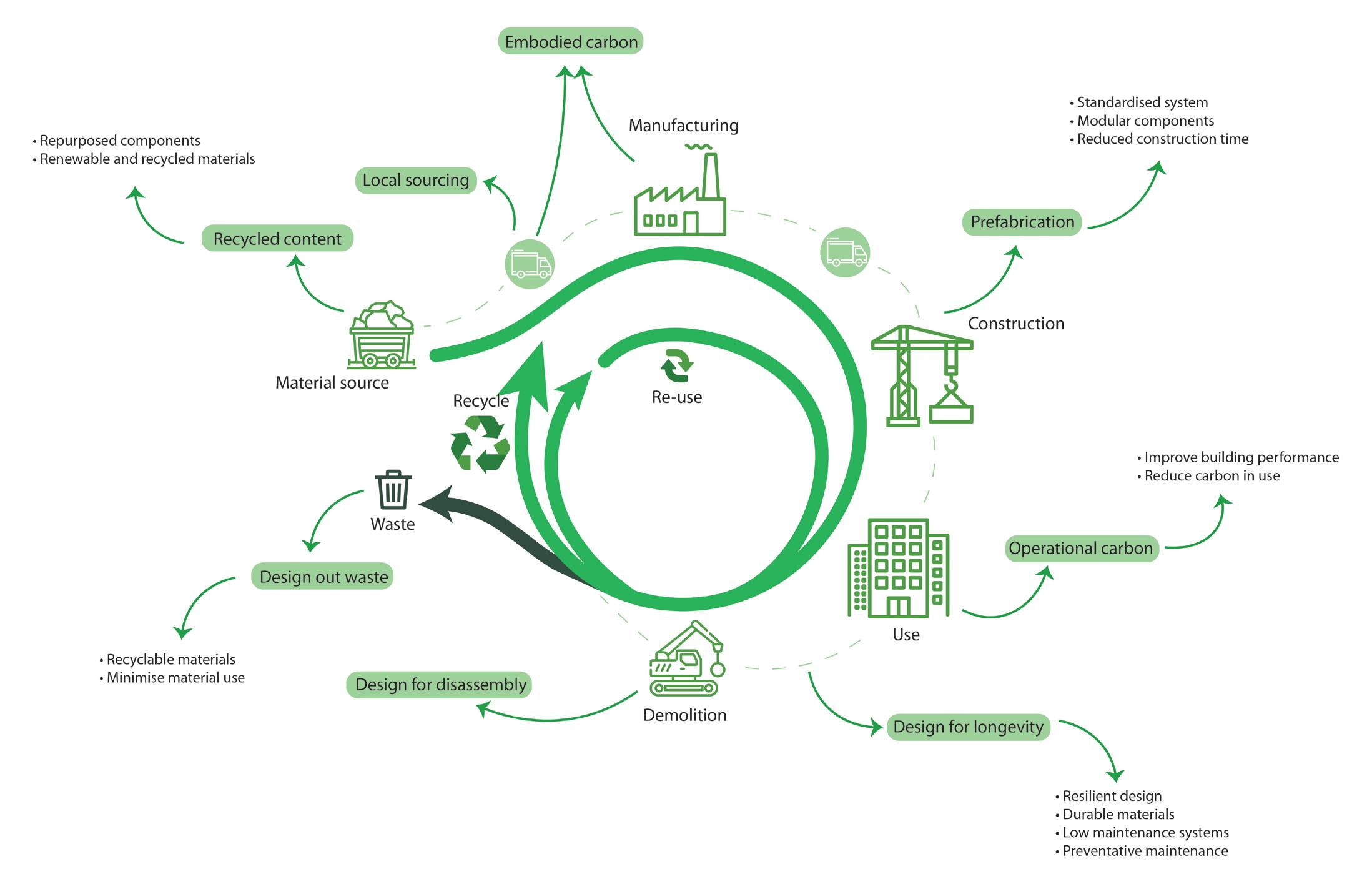
Certainly, bringing existing building stock up to 21st-century performance standards would provide tangible impacts in operational carbon emissions and living conditions and there is not time or resource available to replace existing stock.
The facade can have a significant influence in driving down carbon emissions in the built environment: it plays a vital role in the building energy strategy; it promotes the building’s purpose and is subject to changes in fashion; material choice and selection can be broad from an embodied carbon perspective; there is opportunity to create a façade that has low embodied impacts and delivers operational benefits by being fit for its unique environment.
Glazed facades are proprietary systems rather than assemblies of standard components, so upgrading demands a full understanding of how the system works and for someone to take responsibility for how the modified system performs, including the integrity and durability of retained parts. Innovation is needed in the business model around this kind of work, by designers, clients and contractors together. Improvements in technology to measure,
100%
Environmental impacts and costs 75%
50%
25% Possibility to in uence impacts and costs
Cumulated impacts and costs
Planning
(fig 2 impact of decisions over time) © Arup Construction Time Use and maintenance
inspect and verify existing work will hopefully reduce the ‘unknowns’ and we will jointly devise schemes that avoid ‘risk’ resulting in wasted material and lost opportunity. luxury, incurring design and development costs and some tooling. However bespoke might be the most materially efficient solution, deploying just enough material and no more. In fact there is an argument that every component should be optimised to its duty, a possibility that gets
15000 Building’s materials and construction
(Fig 3. Whole life carbon) © Arup
10000
5000 Facades Share ~20%
Operational carbon Maintenance and repairs
Facades Share ~35% End of life and disposal
Year 0 Year 10 Year 20 Year 30 Year 40 Year 50 Year 60 Design life
Operational carbon Maintenance and repairs carbon Embodied carbon Embodied carbon target kgCO2e/m2 GIA (Fig 4. Beyond embodied carbon) © Arup
Option 1 Single skin + opaque panels Option 2 Single skin + ns Option 3 Optimised ns per orientation Option 4 Double skin Option 5 Double skin + features
closer with each advance in designing with digital fabrication, and leads to very lightweight solutions with the minimum embodied impact. In the context of the climate emergency, emissions savings in the immediate decade are of much greater value in averting the crisis than potential savings at a future time when it is all too late. Regardless of whether the project is a new-build or a refurbishment, there are significant ways we as façade designers and engineers can influence the project for the best outcomes.
So, what drives responsible façade innovation?
Well-designed facades can have a significant environmental impact in terms of carbon reduction, water management and biodiversity support. However, only a design that can provide a positive economic contribution for investors can have the opportunity to deliver in terms of environmental advantages. Add to this the positive well-being impacts such as visual and thermal comfort, as well as contributing to social value impacts. It is our role and responsibility to help find a good balance and make projects win-win cases. The opening statement advises that “we must take a critical look at the brief”. To be able to interrogate a brief effectively and set a meaningful course for the façade, it is essential to be present at the outset and in a position to set ambitious targets before pen has been put to paper. (fig 2 ‘impact of decisions over time)
In the case of residential developments, there are conflicts to address like avoidance of overheating vs daylight levels, noise levels vs natural ventilation, and strategies for low energy consumption and low embodied carbon. By threading a design path between these potentially contradictory criteria, we can begin to influence in the early stages of the project, to deliver as many opportunities as possible for the well-being of occupants, the reduction in energy consumption and the value of the development. Orientation and massing, for example, will focus on the well-being of residents by maximising views and daylight levels to each apartment but without increasing the risk of overheating inside, thereby reducing energy consumption and therefore carbon emissions.
As designers, we have a great responsibility when defining façade systems and material selection to challenge ourselves to limit carbon from the outset. When looking at the carbon emissions of a building, the embodied carbon, related to material and construction, has the first big impact. After 25 years or so there is a second substantial impact to allow for the replacement of sealed insulating glass units, and finally at end of life for disposal. Meanwhile, operational carbon stays mostly consistent for the whole building’s life, reduced only by decarbonisation of the electricity supply. It is in our hands, as designers, suppliers and manufacturers, to impel a transformative change and focus, now, on the reduction of high embodied carbon materials as our best way of substantially impacting carbon emissions overall. (Fig 3. Whole life carbon)
Passive vs active systems
Targets for façades in office buildings typically vary between 20% and 15% of the total carbon target for the building. Carbon has now become a key factor in the system selection but it is not just embodied carbon we need to look at. (Fig 4. Beyond embodied carbon)
Curtain walling is often the primary façade system of choice. In a single skin or passive facade, for example, we typically have 2 options to limit solar gain targets - add opacity using solid panels with different materials and features or introduce fins. The first option gives us a limited window to wall (WWR) ratio of 30-45%. Fins reduce the solar gain and achieve a higher WWR of, say, 40/50%. However, both result in additional embodied carbon. For double skin or active facades, where solar gain is limited with interstitial blinds, we can achieve an increased glazed percentage of 70-80%
Solar control coating Solar control coating Solar control coating Low-E Coating Low-E Coating
SINGLE SKIN WITH SOLAR COATING SINGLE SKIN WITH FIXED SHADING & SOLAR COATING
PASSIVE
g-value Solar Gain (W/m2)
0.28 45
(fig 5. System comparison) © Arup WWR
100% 90% 80% 70% 60% 50% 40% 30% 20% 10% SINGLE SKIN WITH ACTIVE SHADING & SOLAR COATING CLOSED CAVITY FACADE (CCF) EXTERNAL VENTILATED FACADE (EVF)
ACTIVE
g-value Solar Gain (W/m2)
0.10 45
WWR
100% 90% 80% 70% 60% 50% 40% 30% 20% 10%
(fig 6. Material comparison with impact of standard vs sustainable aluminium framing) © Arup
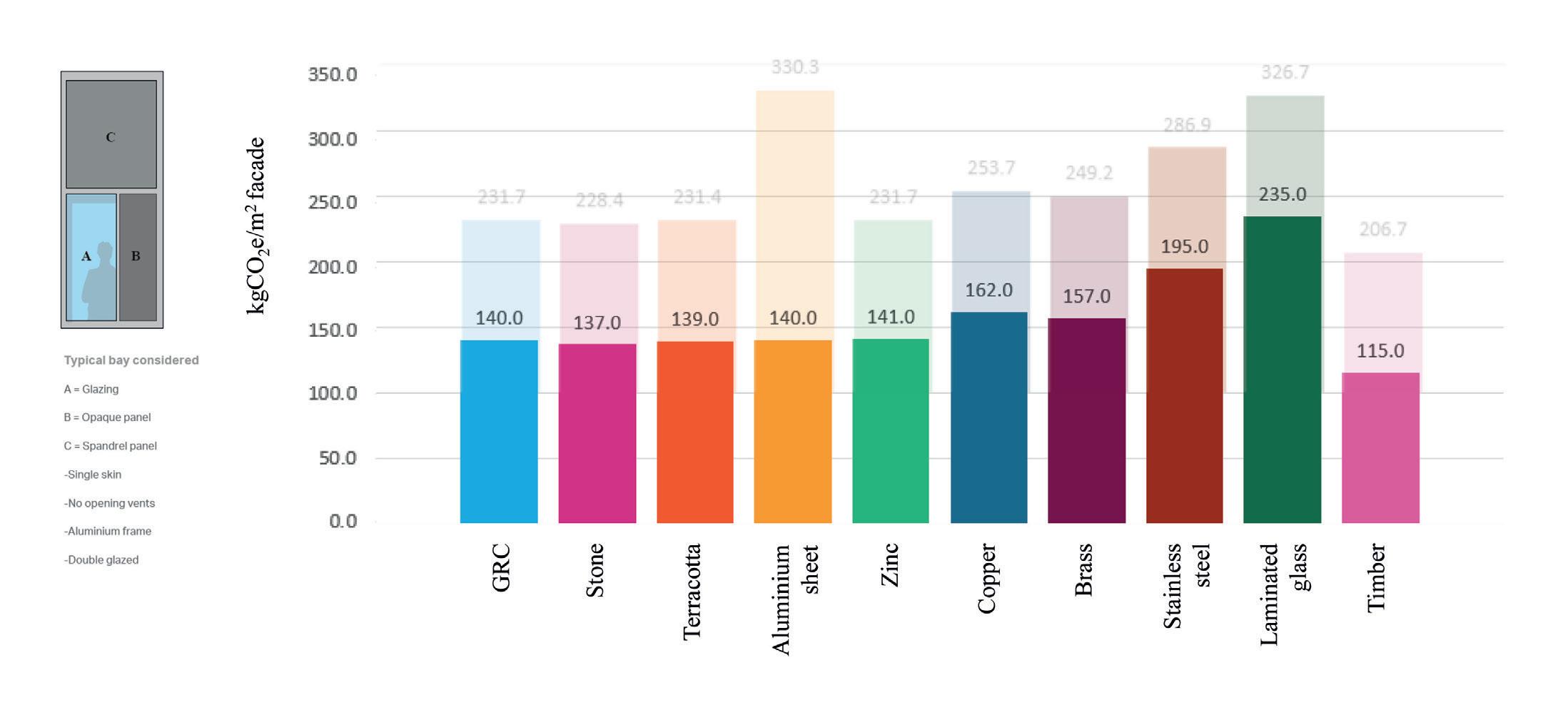
WWR. So, thinking in terms of daylighting for wellbeing and reduced energy consumption, two systems with a solar gain target of 45 or even higher will result in WWR ranges between 20 and 50% for single skin and WWR ranges between 60 and even 85% for double skin. (fig 5. System comparison)
If we were to make a comparative analysis of a typical unitised curtain wall, we need to look beyond the materials alone. How is the facade constructed i.e. supports and brackets or the weight of the material itself? What about the life span of the material and how often it will need replacing? Even in the earliest stages, we can make a reasonable estimation without the project being fully defined. And what about the end of life? Is the material reusable or recyclable and to what degree?
The curtain wall framing can have a large impact on the overall embodied carbon. Reduction strategies would start with trying to reduce the amount of framing and then the specification of the material itself: looking for a certified higher percentage of recycled content or the selection of manufacturing companies that use sustainable energy sources. (fig 6. Material comparison with impact of standard vs sustainable aluminium framing)
The possibilities of reuse
To drive down carbon we need to shift our focus from ‘cradle to gate’ mindset and move beyond even operational carbon targets to ‘whole life’ targets for recycling, reuse and disposal. For this to work effectively, we need good data. It is possible to deliver circular projects if we understand the materials that
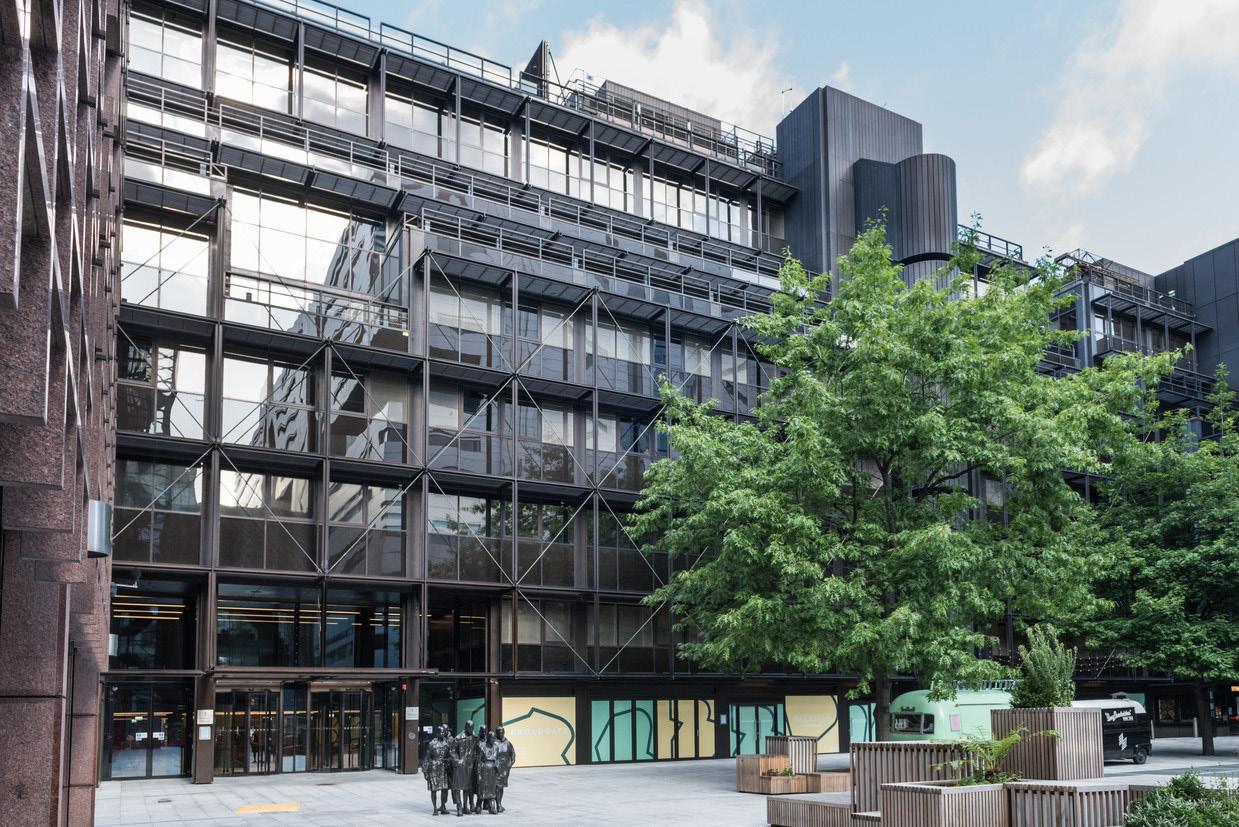
(fig7. triton) © Arup

(fig 9. 1 Finsbury Avenue) © Paul Carstairs, Arup
form the façade, their value and their potential to provide extended performance. For No1 Triton Square, we were able to assess which façade elements could be dismantled and reinstalled, which could remain in place and which could be removed, thanks to the availability of detailed as-built drawings and being part of the design concept team that created the refurbishment strategy. (fig7. triton)
That project was unusual in that the scheme was to extend the building upwards, allowing the façade to be re-used higher up the building but in many cases the choice is between refurbishing an existing façade or scrapping it. (fig 8. Triton Square)
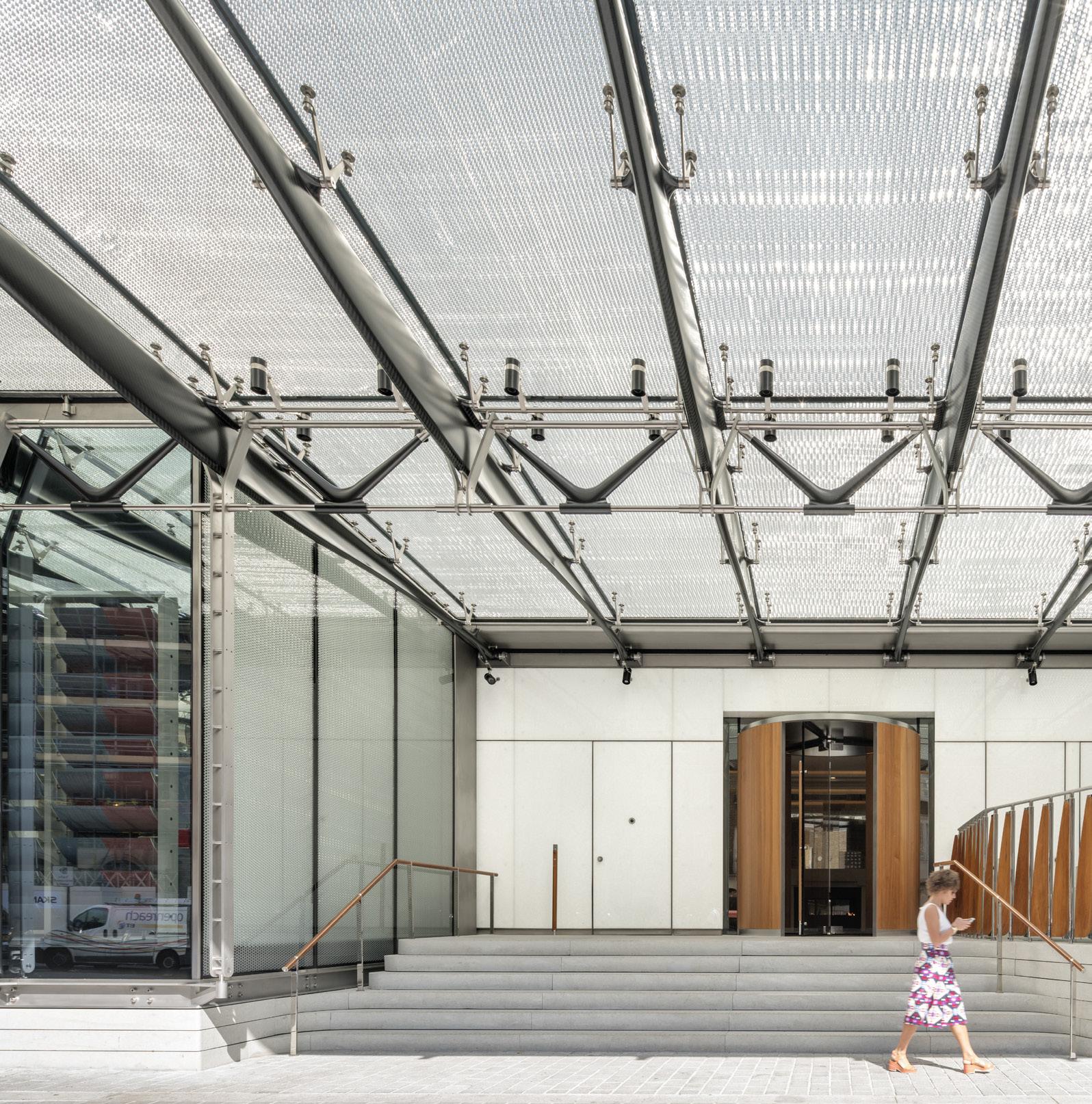
Aluminium has always had a good scrap value, so gets salvaged during demolition, but glass from an old façade has usually been a liability. However, things are beginning to change, with schemes emerging to take back glass from buildings when broken into the form of ‘clean cullet’. Glassmakers have always used cullet in the batch to aid melting and modern float lines could use about 50% cullet if it was available, reducing fuel consumption, carbon emissions and the increasingly scarce glassmaking sand but so little glass is recovered from buildings that the highest recycled content is only about 25% at present. The ultimate step to minimise the embodied impacts of glass would be to recover it without breaking then clean and re-use it without melting. Re-use has proved economic on 1 Triton Square and the Lloyd’s building as detailed in Rethinking the Lifecycle of Architectural Glass, and further innovation is required in the development of a ‘reverse supply chain’ to recover these durable materials and preserve their maximum value.
Ideally, we would not have to replace entire insulating glass units after 25-30yrs when condensation occurs in the cavity. In practice, many good quality insulating glass units last considerably longer than the 25yr anticipated service life, as does laminated glass, if installed in well designed and maintained systems. For 1 Finsbury Avenue, built in 1985, Arup assessed the existing façade, basing decisions on each element’s performance and heritage significance. The glass units were kept, which resulted in significant cost savings and reduced lifecycle carbon emissions. (fig 9. 1 Finsbury Avenue )
However, sealed units do have a finite life as moisture is drawn past seals into the very dry
(fig 10. Berkeley Hotel) ©Joas Souza
cavity. In some projects we have devised alternatives, allowing the units to equalise to atmospheric pressure while keeping the cavity dry enough to avoid condensation during cold conditions.
Hydraulic systems, fuel systems, large gearboxes and oil-filled transformers all need to allow for flows of air to compensate volume changes and all need to avoid condensation from moist air drawn in, so a range of industrial desiccating breathers is available. These breather units contain silica gel granules, which are better than molecular sieves at releasing moisture into outgoing warm air, and inward and outward relief valves or an oil trap to keep the desiccant separated from external air until a small pressure difference exists to be relieved. Some systems even include moisture sensors, valves and a heater to re-generate the silica gel when it approaches saturation. In most cases, the lowcost silica gel is replaced periodically. The air inside the system does not achieve the very low dew-point typical of a new hermetically sealed insulating glass unit but in most cases will be low enough to prevent transient condensation when the glass gets cold.
We applied this system to the honeycomb sandwich panels of the Berkeley Hotel entrance in London and spherically curved insulating glass units on the Las Vegas High Roller observation wheel in Nevada, USA. (fig 10. Berkeley Hotel)
Retrofit systems have also been used to dehumidify and thereby preserve double glazed structural glass walls suffering from premature condensation.
Various curtain wall systems, especially those with large cavities, are also being studied for application of the same technique. The primary advantage is that because the desiccant can be replaced or regenerated remotely from the insulating glass, the life can be greatly extended. It may also be feasible to re-configure glazing design without the permanent bonding of panes, which would have advantages for
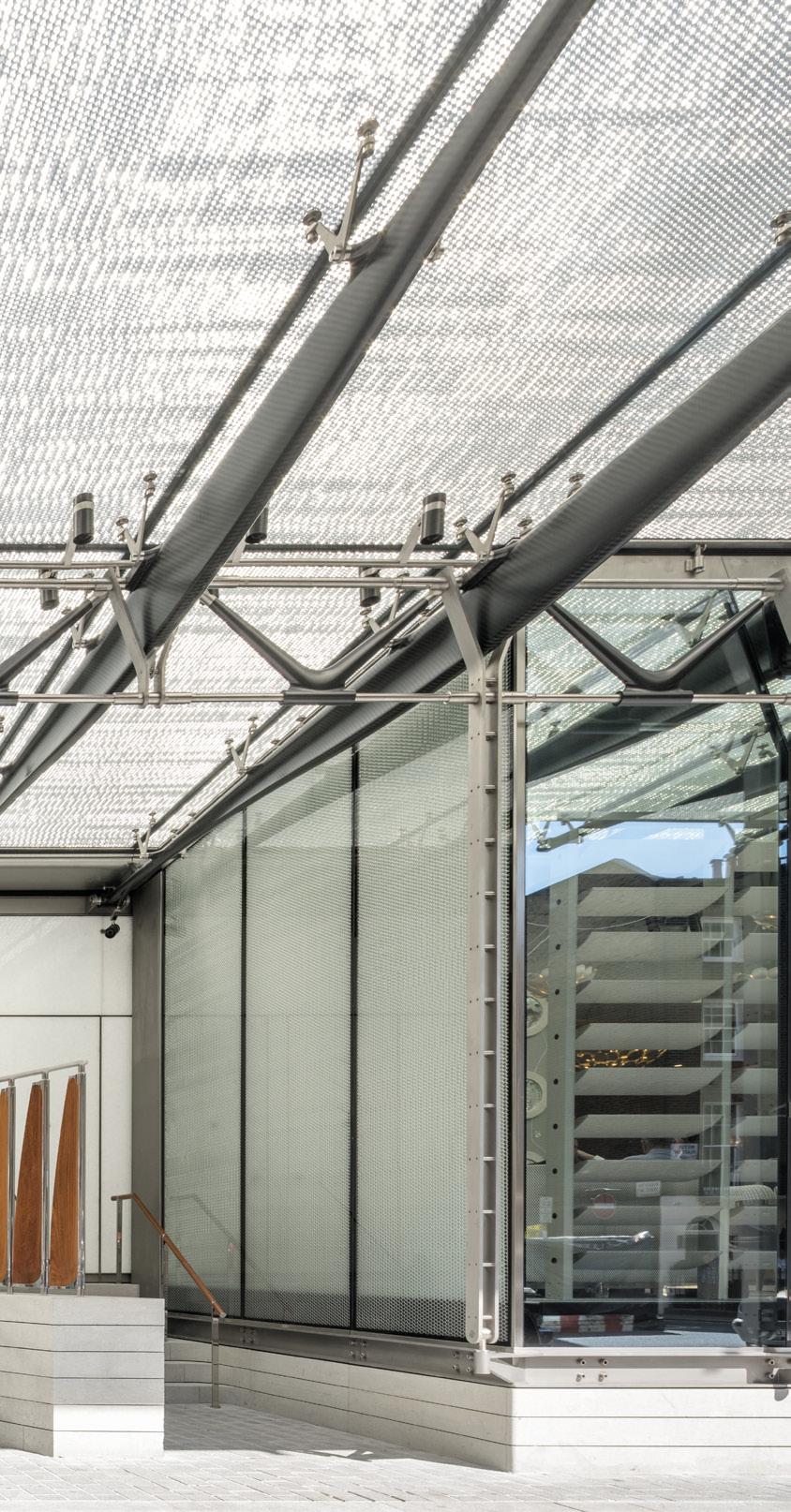
upgrading in service and the re-use or recycling of materials at end of life. (fig 11. Dessicant system – façade can be replaced from either side)
Digital transformation
Increasingly, digital innovation drives the agenda through the development of tools. At the early stages, we can quantify the impacts of solar exposure in terms of risk of overheating and daylight and the availability of views with digital modelling that helps to make real-time decisions involving all the design and client teams. Early parametric studies can inform the envelope geometry to provide self-shading facades without introducing solar shading devices which would increase cost and the embodied carbon of the façade. (fig12. Arup Solar model of preliminary solar gains assessment)
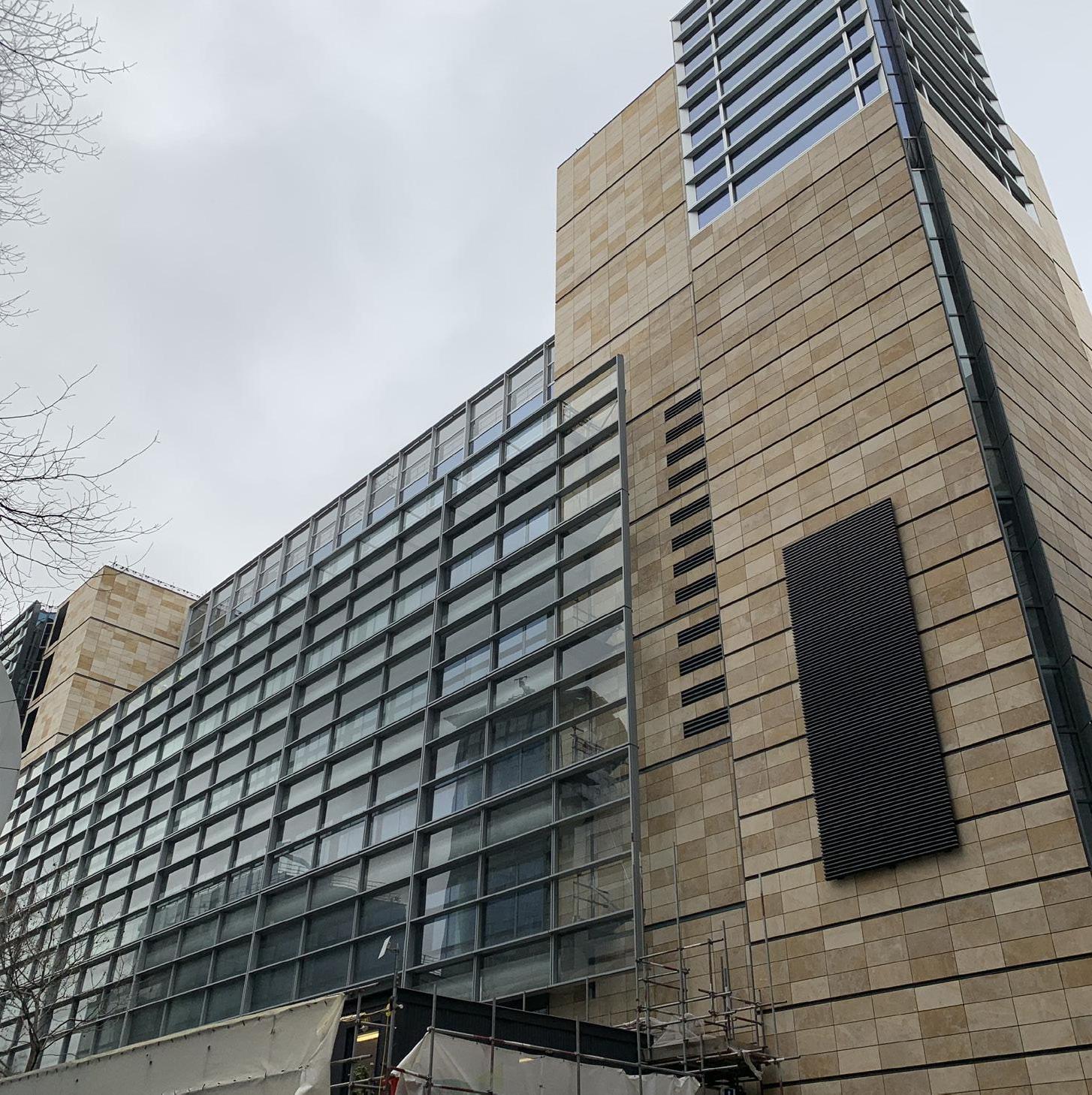
In later stages, they help to document the design throughout the project lifecycle, communicating the complexities to clients and the supply chain in an intuitive way. We record every detail about the facade for future reference, information that
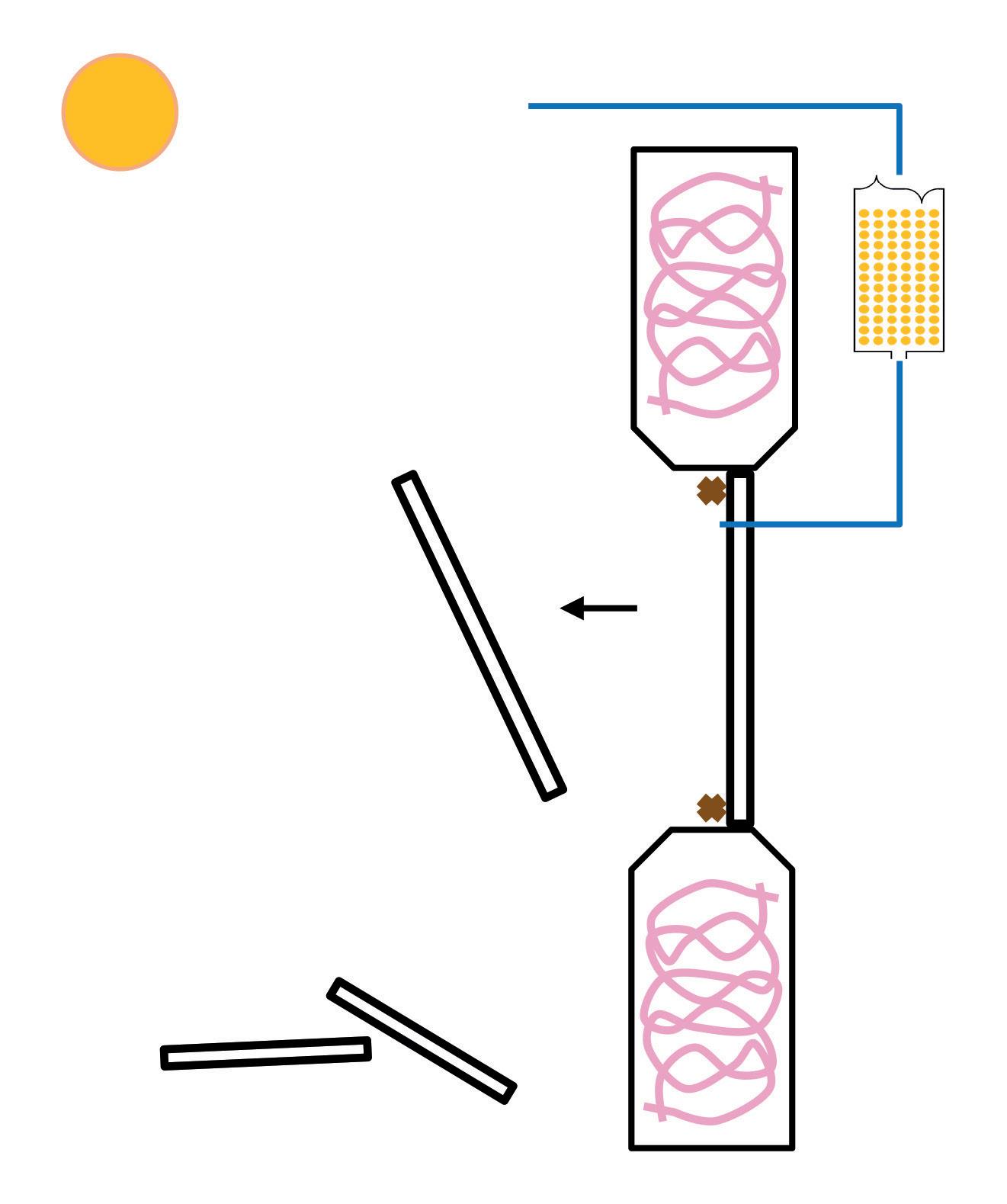
(fig 11. Dessicant system – façade can be replaced from either side) © Arup
Triton Square © Arup
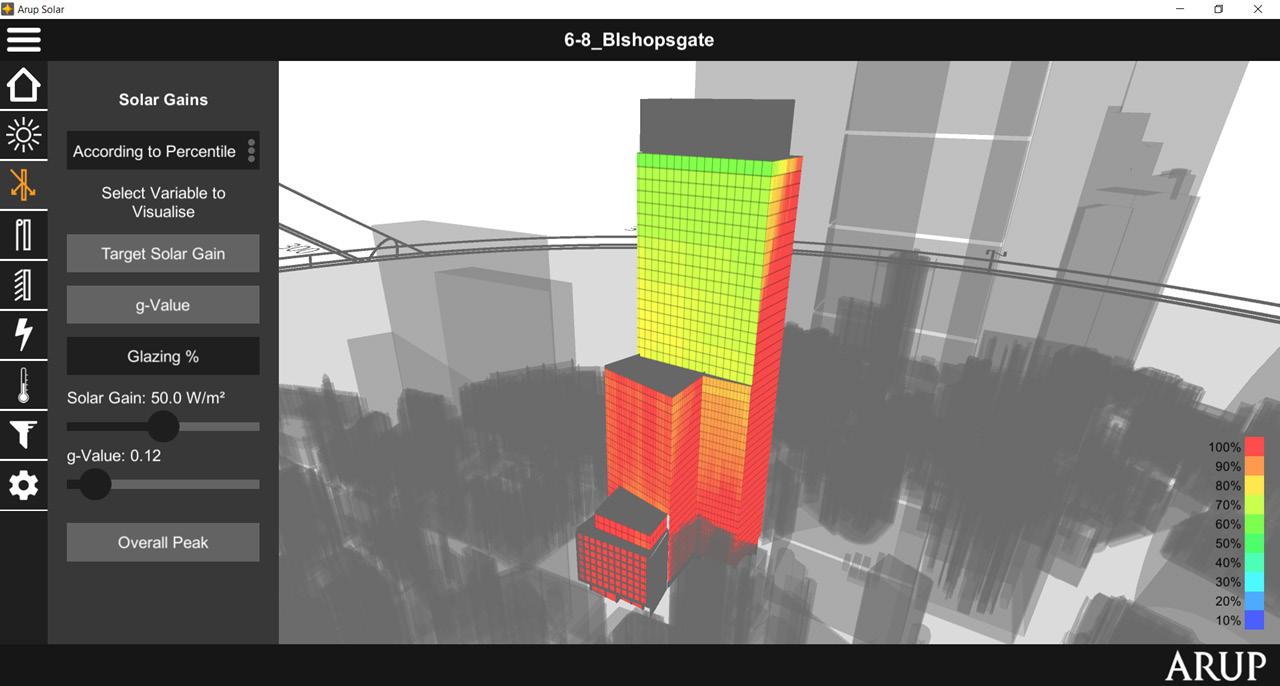
fig12. Arup Solar model of preliminary solar gains assessment © Arup
can be used to model the facade, define on-site inspection regimes, and maintain the health of the building throughout its lifecycle and the safety of its occupants.
This reflects our opinion that we all must be responsible for how we record and store data, in line with Dame Judith Hackitt’s post-Grenfell recommendations and the concept of the golden thread of information. The opportunity of a data chain can be exploited if we know and understand the supply chain and the chain of value. If by design the components of a façade are accessible to dismantling, the availability of information about them and their materials increases their value and potentially avoids the emissions inherent in making new. Not only is the golden thread crucial to safe construction and operation of buildings but to their modification and dismantling, and to a circular economy in facades, interiors, services and structure.
References 1. Simondetti, Alvise. Designing with digital fabrication. London : Arup, 2020. 2. DeBrincat, Graeme and Babic, Eva. Rethinking the life-cycle of architectural glass. Glasgow : Arup, 2018. Viability study & value report.
Graham Dodd
Graham is a Chartered Mechanical Engineer, Arup Fellow and Vice Chair of the Society of Façade Engineering. He has over thirty years of experience in structural glass design and previously worked in appliance manufacturing, glass processing, contracting and façade engineering. He is a keen advocate of using materials and processes in ways that lessen their impact on our environment.
Giovanni Zemella
Giovanni is an Associate at Arup with a background in building physics. Building envelope optimisation is a key focus and he works with his clients at the earliest stages of projects to identify opportunities for low carbon design, circular economy adoption and wellbeing. He leads the UK building envelope physics team in Arup, advising on façade performance and innovation.
Laura Solarino
Laura is a Facade engineer at Arup. She is passionate about sustainable design, applying low carbon strategies, lifecycle assessments and innovative building technologies to reach sustainability goals. She completed the MSc Architecture, Urbanism and Building Sciences program at TU Delft and has worked as an architectural assistant, focusing on existing building renovations, lighting studies and designs for historic buildings.
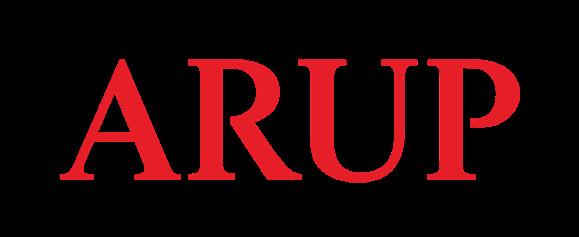
Shaping sustainable futures
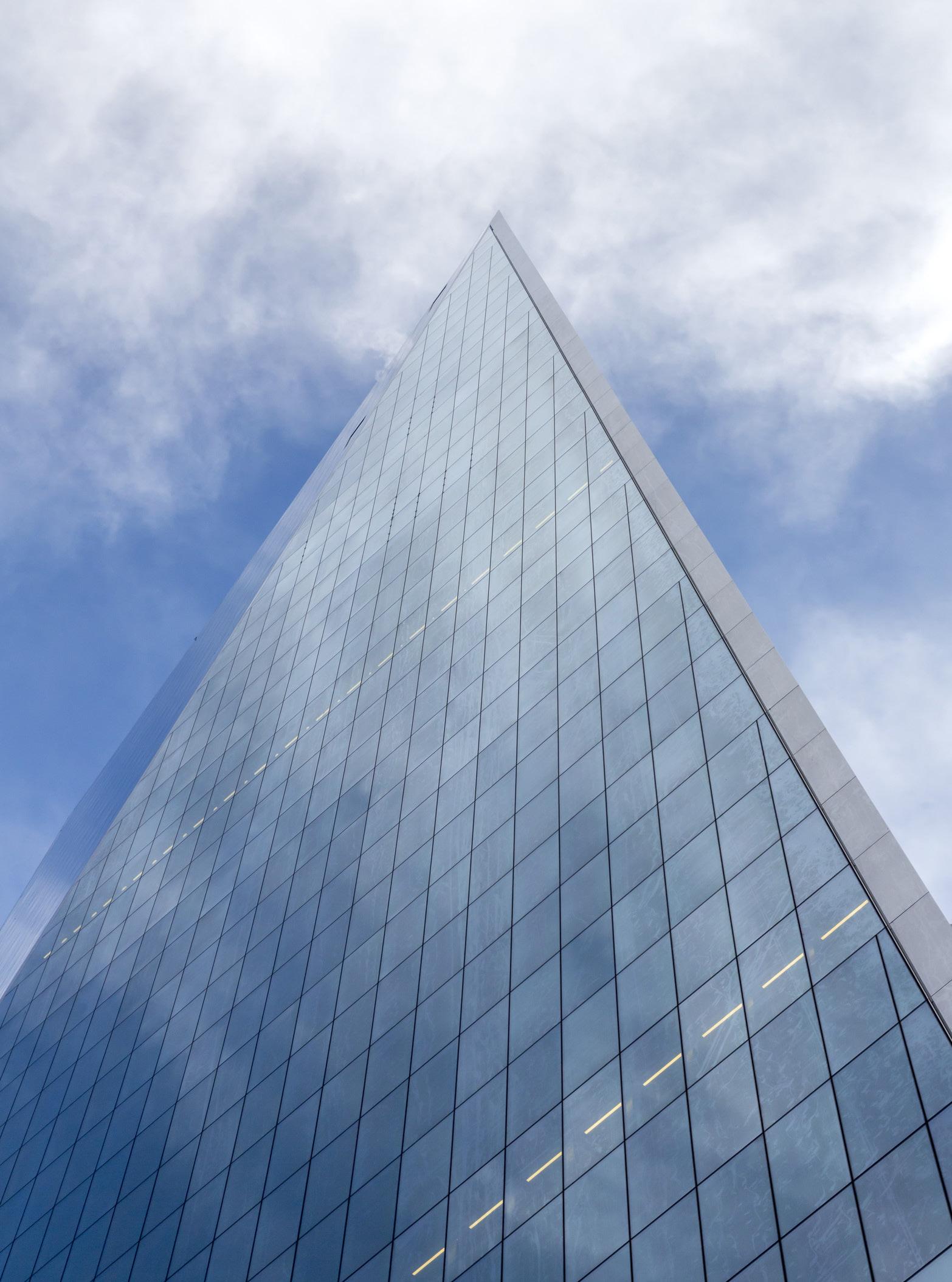
© Paul Carstairs