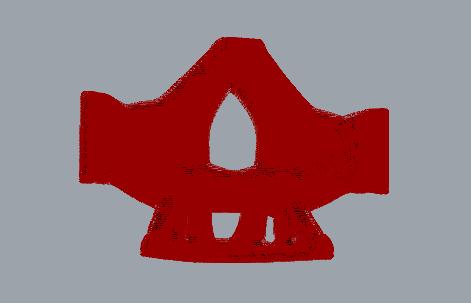
7 minute read
Prevailer Mba
Geometric Stiffness Control Techniques for Metal-based Implants Prevailer Mba
Mentor: Jaejong Park Mechanical Engineering
Introduction: Bone defects can develop from various situations such as trauma, infections, tumor, surgery, etc. In some cases where the defect is large, making bone not being able to regenerate itself, the defect site needs to be replaced for functional requirements and aesthetic reasons. Bone grafting is one of such methods available for such purposes. This method includes repurposing another bone in the body (or using a substitute) by modifying it to fit into the affected area. Metal-based bone implants are another option. Titanium and its alloys are widely used in reconstruction surgeries. Since the design and fabrication are done using virtually Ex vivo, metal-based bone implants have geometric freedom compared to bone grafts. However, one of the main shortcomings of metal-based bone implants is their inherent strong mechanical properties. A substantial mismatch in mechanical properties between the metal implant and the bone may lead to a failure in the long run. The property gap between the metal implant and neighboring bone needs to be closed to avoid stress shielding. This study aims to focus on various techniques for stiffness control by changing the geometry of the overall structure confined in a design domain. We are studying how to make the structures ‘porous’ to control the stiffness of the structures. While making structures porous intuitively reduces the stiffness of the structure, the study explicitly pursues algorithms where the stiffness can be systematically controlled. Various internal structuring techniques, such as lattice structures with different geometric configurations, architectural materials, and auxetics, are being investigated. Methods and Materials: In our review of published manuscripts, different methods were identified for stiffness control. This summer, we decided to focus our efforts on understanding two of these methods. They include creating pores on implants and creating lattice structures. To be able to effectively control the creation of the pores, software was needed that supported the creation of designs from an algorithm. This ensured creativity and flexibility in design. We utilized the combination of Rhino and Grasshopper to learn the basics of creating new designs from an algorithm. For the lattice structures, Crystallon was considered an excellent choice for the work. It was to be used as a plug-in to Rhino for the creation of the lattice structures. Results and Discussion: As mentioned in the last progress report, the ability to edit imported implant designs is crucial to this work. However, we have been having some issues in this regard. We have not been able to edit imported designs such as .stl files of previously optimized bone-implant geometries in Rhino. It is crucial that we are able to do this as not all implant models will be created in Rhino. It is essential that imported models can be edited. Some solutions that we found include converting the files to a surface and working with them from there. Another option is to use the local code import plug-in to import the .obj files directly into a grasshopper. We started testing out these possible solutions; however, we haven’t been able to completely solve the problem. In the case of the file conversion, the conversion was successful; however, the file was converted into an indefinite list of surfaces, which makes it difficult to work with. The image in figure 2 above shows this obstacle. The Rhino program has to be able to recognize the model as a single surface for effective use. This issue is currently being mitigated. The local code import was successfully implemented to a grasshopper. The plug-in is still being explored, and as such, it has not yet been used on implant models.
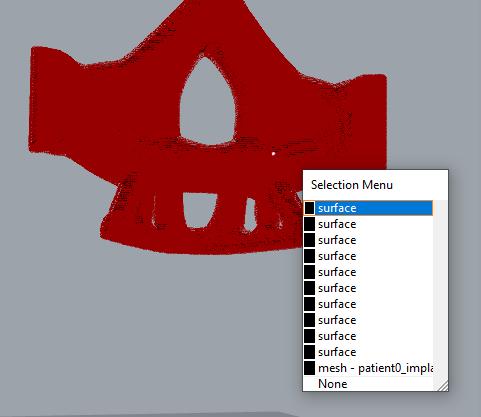
Fig 1: Bone Implant .obj file from earlier work [1]. Fig 2: Bone Implant .obj file showing surface list.
Conclusion
The issue encountered with Rhino is currently being mitigated, and more practice is being put in to understand the local code import and how to implement it properly. Upon successful completion, this will provide a simpler way of creating controlled porosity in any target object for stiffness control. Partial findings of this research have been included in a recently submitted manuscript [2].
References
1. Sutradhar, A., et al., Experimental validation of 3D printed patient-specific implants using digital image correlation and finite element analysis. Computers in Biology and Medicine, 2014. 52: p. 817. 2. Park, J., et al., A two-scale multiresolution topologically optimized multi-material design of 3D printed craniofacial bone implants. Micromachines, Biology and Biomedicine B, 2020, in review.
Awardee and Student:
Dr. Jaejong Park is an Assistant Professor of Mechanical Engineering with research interests in structural optimization, mechanical design, and advanced manufacturing. Prevailer Mba is a Senior majoring in Mechanical Engineering.
Numerical Experimentation of Bio-physicochemical Interaction of Airborne Species in the Pulmonary Circulation
Diamy Bekale Camara
Mentor: Dr. Kazeem Olanrewaju Chemical Engineering
Introduction: Cardiovascular system comprises the complex fluid (blood), the heart, and all the blood vessels embedded within the vascular tree, which include the arteries, arterioles, capillaries, venules, and veins[1], [3]. As earlier depicted, the cardiovascular system essentially covers the operation of the circulatory system and other auxiliary activities such as the myocytes' innervation to enhance heart rate effectiveness and cardiac output [4]. The cardiovascular/Circulatory system mainly addresses the systemic, portal, and pulmonary circulations of blood to organs of the body and the lungs, respectively [5]. Vital species needed for proper physiological functioning and homeostatic stability of the body systems are received at various sites, transported, and delivered via the bloodstream to the site of utilization (mostly cells) and disposal[6]. These physiological species, which are products of macromolecular (liquid and solid) monomerization and gaseous molecule, access the human physiological system via the respiratory, digestive, and integumentary tracts [7], [8]. Life-supporting gaseous molecules (O2 and CO2) and other airborne species (antigen) or gases access the physiological system through the respiratory tracts and are transported downstream to the alveoli, where exchange via diffusion into the bloodstream occurs. The oxygenated blood is subsequently conveyed through the pulmonary vein and received into the cardiac unit (heart) via the left atrium [3], [5]. Preceding description succinctly delineates pulmonary circulation. This project will systematically explore various mechanisms associated with physiological and pathophysiological implications of bio-physicochemical interaction of airborne species in the cardiopulmonary circulation system. Materials and Methods: Highlighting Species Transport and Transformation in the Pulmonary Circulation Loop. A schematic flow process of species in the pulmonary circulation loop will be developed to have a clear perspective of species transport and transformation from one Stage to another. Species transport began at the respiratory system entrance, traverses and transformed through the various organs, tissues, and cells germane to the cardiopulmonary systems, and afterward disperse to all other body systems via the systemic and portal circulation flow vessel for metabolisms and other physiological functions. Defining Transport and Transformation Mechanism at each Stage of the flow process. The transport mechanism at each stage of the process was defined, and the transformational mechanism was equally elucidated as the flow progresses within the pulmonary circulation loop. The specific mechanism was allotted to each Stage in the flow process. Results and Discussion: A flow chart portraying step-by-step processes involve in the pulmonary circulation loop within the cardiovascular and respiratory systems was developed. The flow chart further stretches out species transport and transformation within the loop. The flow chart's essence is to lucidly depict the different stages where transport and change of species occur within the pulmonary circulation. Organs within the loop are arranged in sequential order in the red borderline rectangular block flow diagram. Simultaneously, the transport and transformation of species at different stages in the pulmonary circulation are highlighted in a blue marginal rectangular block. The red arrow connects organs in the flow chart. In contrast, the black hand connecting the red arrow and the red borderline rectangular block respectively depict the corresponding species transport occurring from organ to organ and transformation of species within the organ. The flow chart's rationale is developing a platform that will explicitly express the various physiological mechanisms accentuating the different species transport and transformation processes involved in the pulmonary circulation. The next step is to expound qualitatively on these mechanisms and 77
develop/adapt the various numerical model to describe and characterize these physiological processes quantitatively. Conclusion(s) or Summary: The schematic flow chart development advanced the research task a significant step forward and set a plausible platform for a qualitative depiction of the physiological mechanisms needed to clearly understand species transport and transformation within the pulmonary circulation loop. A clear view of where species transport and change apply within the pulmonary blood circulation circuit is evident. Numerical quantification of species transport and transformation processes characterized by detailed physiological mechanisms will be subsequently explored through modeling. The insight gained will help understand the range of pathophysiological conditions associated with cardiopulmonary circulation and the appropriate diagnostic procedures and therapeutic measures needed to remedy the disease of interest.