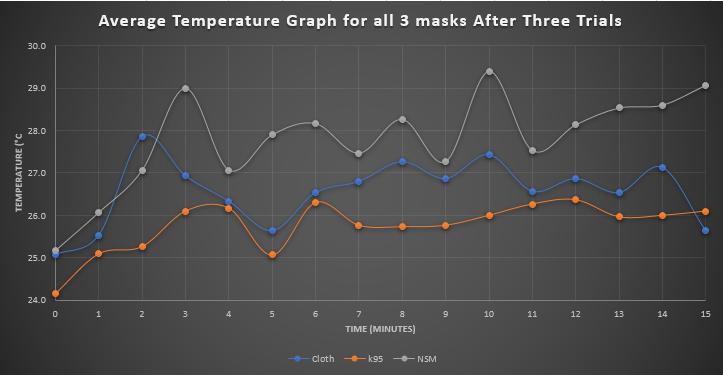
8 minute read
Indira S. Ribeiro
Mentor: Kazeem Olanrewaju Chemical Engineering Department
Introduction: Cardiovascular system, amidst the list of body systems, is critical to the survival of organs of itself and other systems[1, 2]. The physiological operation of other systems, including the nervous system, owes its functionality to the cardiovascular system[3, 4]. The major gateway of nutritional and enervating non-gaseous species accessing the systemic and portal circulation of the cardiovascular system is through the digestive system. Moreover, nutritional species transported via the vascular stream are products of enzymatic and mechanical monomerization of life-supporting macromolecular compound (polysaccharides, protein, and lipid) within the digestive system into simple molecules like glucose, amino acids, and fatty acids[5-7]. Nutritionally rich deoxygenated blood get oxygenated through pulmonary circulation and ejected by the heart via vascular channel to different cells within the systemic and portal circulatory circuit for metabolism. A careful qualitative description of these processes will unequivocally create opportunity to numerically assess the mechanisms associated with fluid-structure interaction of species transported within the systemic and portal circulatory system. Much work has been done to elucidate the qualitative description of these mechanisms, but studies on numerical quantification of these mechanisms are still relatively sparse[8-11]. Therefore, significant effort will be spent analyzing these mechanisms computationally while quantifying in tandem the corresponding physiological and pathophysiological implications of organs under this cardiovascular system coverage. Material and Methods: Highlighting Species Transport and Transformation in the Pulmonary Circulation Loop: A schematic flow process of species in the systemic and portal circulation loop will be developed to have a clear perspective of species transport and transformation from one stage to another. Species transport begins at the respiratory, digestive, and integumentary entrance, traverse and transformed through several organs, tissues, and cells applicatory to the aforementioned systems, and afterwards disperse to all other systems of the body via the systemic and portal circulation flow ducts for metabolisms and various physiological functionalities. Defining Transport and Transformation Mechanism at each Stage of flow process: Transport mechanism at each stage of the process was defined, and transformational mechanism was equally elucidated as the flow progresses within the systemic and portal circulation loop. Specific mechanism was allotted to each stage in the flow process. Numerical Experimentation: This study will adopt two computational modelling platforms to numerical assess fluid-structure interaction of species with systems and organ within the systemic and portal circulatory unit of the cardiovascular system. The two computational platforms, which include Simpleware (Synopsys) and Comsol Multiphysics softwares, will be utilized sequentially for complex geometry development, meshing of complex human physiological structures, and simulation of the physics (modelling equations) of interest[12, 13]. Results and Discussion: The chart depicting the flow processes involve in the systemic and portal circulation within the cardiovascular system was developed. The flow chart further unfold species transport and transformation within the loop. The essence of the flow chart is to vividly delineate the different stages where transport and transformation of species occur within the system and portal circulation. Various organs within the loop are arranged in sequential order in the red blocks, while transport and transformation of species at different stages in the systemic circulation are highlighted in blue blocks. The rationale behind the flow chart is the creation of a platform that will help in the detailing of the various physiological mechanisms underlining the different specie transport and transformation processes involve in the systemic and portal circulation. The flow chart portray a visual qualitative description of the species transport and transformation mechanism within the systemic and portal circulation. Conclusion/Summary: The creation of the schematic flow chart advanced the research task with a major step forward by setting a realistic platform for a qualitative representation of the physiological mechanisms required to clearly understand the transport and transformation of species inside the systemic and portal circulation loop. A vivid description of where species transport and transformation apply within systemic and portal blood circulation loop are made evident. Modelling and quantification of species transport and transformation processes describe by detail physiological mechanisms will be subsequently examined through computational simulation. Understanding acquired from modeling will be utilized to gain insight into a range of pathophysiological conditions associated with systemic and portal circulation and the relevant diagnostic procedures and therapeutic measures needed to ameliorate the disease in question. The next task is to adopt these
qualitative description and develop/adopt various numerical model to quantitatively describe and characterize the physiological processes. Quantification of species transport and transformation within the systemic and portal circulation will be conducted through numerical experimentation by the use of computer modeling software. Development of a modeling simulation platform to study range of pathophysiological conditions associated with systemic and portal circulation loop.Major challenges faced during this research work are software acquisition bureaucracy and bottleneck with remote software accessibility cause by the recent cyber attack.
References
[1] (July 6). Study Guide to the Systems of the Body. Available: https://www.acls.net/study-guide-body-systems.htm. [2] N. Kitadai and S. Maruyama, "Origins of building blocks of life: A review," Geoscience Frontiers, vol. 9, (4), pp. 11171153, 2018. [3] P. K. JANGHU. (July 3,). Systemic, Pulmonary and Portal Circulation , Anatomy QA. Available: https://anatomyqa.com/systemic-pulmonary-portal-circulation/. [4] D. N. Ku, "Blood Flow in Arteries," Annual Rev Fluid Mech, vol. 29, pp. 399-434, 1997. [5] B. O. Schneeman, "Gastrointestinal physiology and functions," Br. J. Nutr., vol. 88, (S2), pp. S159-S163, 2002. [6] B. Greenwood-Van Meerveld, A. C. Johnson and D. Grundy, "Gastrointestinal physiology and function," in Gastrointestinal PharmacologyAnonymous Springer, 2017, pp. 1-16. [7] H. Singh, A. Ye and D. Horne, "Structuring food emulsions in the gastrointestinal tract to modify lipid digestion," Prog. Lipid Res., vol. 48, (2), pp. 92-100, 2009. [8] B. Rubik, "The biofield hypothesis: Its biophysical basis and role in medicine," The Journal of Alternative & Complementary Medicine, vol. 8, (6), pp. 703-717, 2002. [9] J. G. Weers, "A physicochemical evaluation of perfluorochemicals for oxygen transport applications," J. Fluorine Chem., vol. 64, (1-2), pp. 73-93, 1993. [10] D. Ramsay and S. Woods, "Clarifying the Roles of Homeostasis and Allostasis in Physiological Regulation," Psychol. Rev., vol. 121, pp. 225-47, 2014. . DOI: 10.1037/a0035942. [11] Anna Kucaba-Pietal, "Blood as complex fluid, flow of suspensions," in Blood Flow Modelling and Diagnostics, Tomasz A. Kowalewski., Tomasz Lekszycki., Andrej Nowicki, Ed. Warsaw, Poland: Institute of Fundamental Technology Research, 2005, pp. 9-30. [12] COMSOL Inc., "Simulate real-world designs, devices, and processes with multiphysics software." . [13] Synopsys, "Simpleware ScanIP," 2020.
Awardee and Student:
Dr. Kazeem Olanrewaju is an Assistant Professor with research interests in Fluid-Structure in Human Systems, Biorenewable and Supercritical Fluid Reactions, Energy and Environmental Systems Sustainability Indira Ribeiro is a senior, majoring in Chemical
Utomwen Irabor
Mentor: Nabila Shamim Chemical Engineering Department
Introduction: From the end of 2019 till date, Coronavirus has invaded our personal and professional lives. To prevent Coronavirus infection and its spread, individuals must put on facemasks, maintain social distances, and continue adequate hygiene practices. This project’s objective is to study the thermal changes and properties of face masks using Attenuated Total Reflection (ATR). This research also aims to identify masks that performs best after an extensive period of usage to reduce heat on the face that causes rash, discomfort, and fatigue. Materials and Methods: Masks used in this experiment include a Badger Smith Cloth Mask, a KN95 Protective facemask, and a non-surgical disposable mask (NSM). The equipment used in this project are a Dino-lite premier camera, a FLIR One Thermal Camera, and an infrared spectrometer to find the Attenuated Total Reflection (ATR). A healthy young man volunteered to wear the masks and conduct the study on himself. The volunteer wore each mask for 15 minutes, and the thermal images were continuously captured using the FLIR One camera five feet apart. The breathing temperature in the masks was recorded as a function of time. The Heat Flow Rate equation was used to find the heat transfer through each mask’s membranes. The Dino-lite camera was used to capture magnified photographer of each mask’s membrane. Results and Discussion: To calculate the rate of heat transfer between each mask layer or membrane, the equation of heat flow through a multilayer wall was used. The equation states that,
5 =
6%
−65 ∆83 93.+ ∆84 34.+ ∆83-.
6%
−65 :3 +:4 +:-
Where the resistance is :3 =∆83/(3-.) and so on. 6% −65 is the difference in temperature from the first membrane to the last membrane of the mask. q is the rate of heat flow in joules per second, x is the thickness of the mask in meters, k is the thermal conductivity in <=>?@A/A@'(°*, and A is the surface area in (! . Only conduction in heat transfer was considered in this experiment. As shown in table 1, the rate of heat flow in the non-surgical mask is the highest. This is because the non-surgical mask (NSM) does a better job at releasing the heat generated from the wearer into the atmosphere faster. Figure 1 also supports this claim. Figure 1 shows the temperature profile as a function of time for three different masks. The non-surgical mask has better thermal management largely because these types of masks have fewer layers. The non-surgical disposable mask only has three layers. It has two layers of polypropylene in a middle layer of micro-filtration melt-blown cloth.
Table 1: Showing the Mask’s Rate of Heat Flow in Watts or J/s
Conclusion: Face masks with better thermal management will be helpful for prolonged use and enhance comfort. The KN95 is the best option for protection against the Coronavirus but, the Non-surgical mask has a better thermal management. In the future, the plan is to improve the efficiency on health/virus protection of the non-surgical masks using nanofibers. Improve the efficiency on health/virus protection of the non-surgical masks using nanofibers and study the effects of stimuli-responsive polymer to advance thermal management of the non-surgical mask.
Mask’s layers were captured by Dino-Lite Premier Camera
A Thermal Image of the Cloth Mask Been Worn 1st Layer of Polypropylene in NSM Mid Layer of micro-filtration melt-blown cloth Final Layer of Polypropylene in NSM
References
Geankoplis, Christie John. “Introduction to Heat Transfer.” Transport Processes and Separation Process Principles, edited by A. Allen Hersel, 5th ed., Pearson Education, 2018, pp. 413–452.