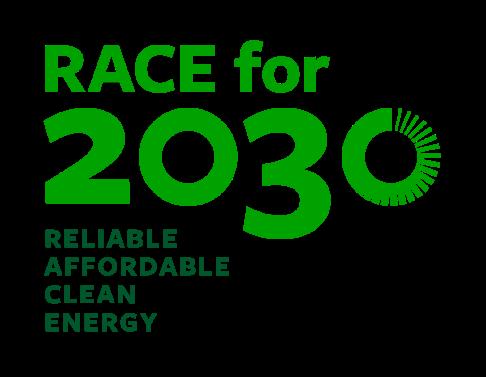
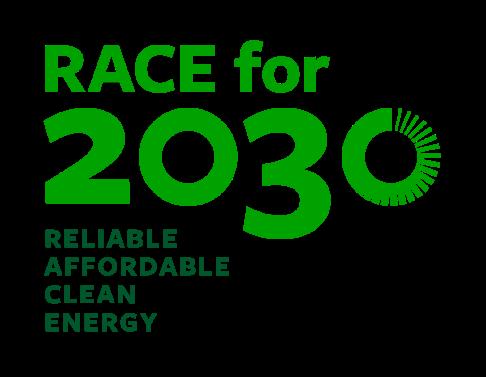
Using a Systems Thinking Approach and Consumer and Distributed Energy Resource Integration as a Case Study
JULY 2024
Research Theme BT1: Digitalising industry
ISBN: 978-1-922746-53-5
PhD Report
Developing Policy for Australia’s Clean Energy Transition Using a Systems Thinking Approach.
Project team
Dr Vikki L McLeod Centre for Clean Energy Technologies and Practices / School of Design Queensland University Technology
Citations
McLeod, V.L., Developing Policy for Australia’s Clean Energy Transition Using a Systems Thinking Approach. Consumer and Distributed Energy Resource Integration Case Study. Prepared for RACE for 2030. June 2024
Project partners
Acknowledgements
Acknowledgement and thanks to RACE for 2030 for a grant to write up the findings of the PhD thesis entitled “The role of demand flexibility trading in Australia’s clean energy transition: A systems thinking approach” published by QUT eprints.
Acknowledgement of Country
The authors of this report would like to respectfully acknowledge the Traditional Owners of the ancestral lands throughout Australia and their connection to land, sea and community. We recognise their continuing connection to the land, waters and culture and pay our respects to them, their cultures and to their Elders past, present, and emerging.
RACE for 2030 CRC is a 10-year co-operative research centre with AUD350 million of resources to fund research towards a reliable, affordable, and clean energy future. https://www.racefor2030.com.au
Disclaimer
The authors have used all due care and skill to ensure the material is accurate as at the date of this report. The authors do not accept any responsibility for any loss that may arise by anyone relying upon its contents.
As part of Australia's clean energy transition and the decarbonisation of its economy, the electricity market and grid will need to be transformed. This transformation will involve the replacement of coalfired power stations with higher levels of variable renewable energy, smart grid solutions, new business models, new markets and software trading platforms that will enable trade in new commodities such as 'demand flexibility'.
This report examines a current policy issue critical to Australia’s Clean Energy Transition of integrating end consumer-owned Distributed Energy Resources (DERs) onto the electricity grid. A "systems thinking" approach is used to understand the emerging opportunities and challenges related to integrating Distributed Energy Resources (such as end consumer owned solar, hot water systems or batteries). It then uses systems thinking techniques to designing policy interventions to unlock market potential.
Governments internationally face the complex challenge of developing policies to decarbonise their nations’ economies to benefit from the energy transition at the pace and scale required. In the case of Australia, the energy transition is characterised by the enthusiastic uptake of rooftop solar PV by end consumers. Therefore, the electricity system and electricity market need to transform to accommodate large-scale generation and millions of end consumer-owned energy generators.
It will require a decentralised, intelligent, networked system capable of managing and trading energy and power system services at the local level. It will take formulating appropriate transition policies to overcome existing political and institutional barriers.
Australia’s energy-related policy has historically been characterised by clashing political ideologies and interests. Australia’s energy market reforms involve multiple state government jurisdictions transitioning at different rates, involving different energy resources and policy responses. The electricity system is complex, and the market, regulations, policy, and politics overlap and intersect with the technical aspects. These factors contribute to energy policy uncertainties and other barriers.
For coal-fired power stations to exit the electricity system, it is necessary to replace the energy and the power system services they currently trade into the energy market. Consumer-owned DERs have potential as a low-cost source of energy and power system services but have to be capable of responding reliably to a third-party signal.
Referred to as DER integration, it is the process of controlling and coordinating DERs from the current un-coordinated and uncontrolled state. DER integration is a remarkably complex problem involving end consumer behaviour, transactions involving multiple parties, energy market reforms, policy development and politics, technology economics and innovation.
This report outlines how complexity theory and a system thinking approach can be used to navigate DER integration policy development.
Foreword Professor Marcus Foth
As the world moves towards a sustainable and clean energy future, the challenges and opportunities in transitioning from traditional fossil fuel-based power systems to renewable energy systems are becoming increasingly apparent. It is clear what we need: Reliable Affordable Clean Energy – RACE. Yet, getting there is easier said than done.
I commend Dr Vikki McLeod for producing this timely report for the RACE for 2030 CRC as it provides a comprehensive analysis of the intricacies involved in transitioning to a renewable energy future within Australia’s National Electricity Market (NEM). Her work highlights the necessity of a holistic systems thinking approach to lead this endeavour and for developing effective policy and regulation.
Developing policy for Australia’s clean energy transition is not an easy feat due to the inherent complexities of the energy system and the diversity of agents and stakeholders. Conventional approaches to policy development often fail to capture the dynamic interrelationships between various components of the energy system. Systems thinking, on the other hand, allows for a holistic view, enabling policymakers to understand and manage the interdependencies and feedback loops that characterise complex systems like the NEM.
Systems thinking is particularly useful in identifying and addressing the unintended consequences and emergent behaviours that can arise from policy interventions. By considering the entire system, including technical, economic, social, and environmental dimensions, systems thinking provides a robust framework for designing policies that can navigate the complexities of the energy transition effectively.
In the context of the energy sector, strategic design and design thinking methods are invaluable. Being a professor in the QUT School of Design, I admit that I may be biased here. However, strategic design methodologies have proven to facilitate lateral connections across a diverse array of stakeholders, including those responsible for the physical infrastructure of generation and distribution, as well as the commercial, legal, regulatory, environmental, and consumer aspects. Rather than keeping each area in its own silo, strategic designers act as intermediaries, ensuring that the perspectives and needs of all stakeholders are considered, and that the solutions developed are mutually beneficial for industry, communities, and the planet.
Dr McLeod’s report corroborates the importance of strategic design in creating an integrated approach to energy policy. By employing design thinking, stakeholders can collaboratively explore innovative solutions that balance the competing demands of reliability, affordability, and sustainability. This approach not only enhances the effectiveness of policy interventions but also fosters greater acceptance and engagement from all parts of the energy ecosystem.
I commend Dr Vikki McLeod for her contributions to this field. Beyond this report, I recommend her PhD thesis, completed at Queensland University of Technology (QUT), to those seeking a deeper understanding of the complexities and potential solutions in this area. It offers an in-depth exploration of demand flexibility trading and its role in Australia’s clean energy transition to.
I thank the RACE for 2030 CRC for its visionary leadership and commitment to driving innovation in Australia’s energy sector. This report is a testament to the CRC’s dedication to advancing research and development that support a secure, affordable, and clean energy future for Australia.
I extend my sincere gratitude to Dr Vikki McLeod for her thought leadership and the comprehensive analysis presented in this report. Her work provides a critical foundation for developing the policies and strategies urgently needed to expedite Australia’s clean energy transition. I trust that the insights and recommendations within this report will serve as a valuable resource for policymakers, industry stakeholders, and researchers alike.
Professor Marcus Foth
PhD FACS CP FQA MACM Dist. MDIA JP (Qual.) Qld
School of Design
Faculty of Creative Industries, Education and Social Justice
Queensland University of Technology
June 2024
m.foth@qut.edu.au | LinkedIn
We are amidst the climate crisis, and we have an opportunity to reinvent our energy system, which will not only address this crisis but also champion values of fairness, community connectedness, and affordability. Electrifying our homes and cars and powering them with locally produced renewable energy is the unsung hero of the energy transition and recognises that households can be critical energy infrastructure that brings a range of benefits to Australians.
Electrification will be the fastest, cheapest way to lower energy bills and eliminate polluting fossil fuels. Doing that electrification on our rooftops and in our communities is the fastest of all as it does not require transmission infrastructure and large-scale projects. Because Australia leads the world in rooftop solar, the economics are extraordinary. If we electrified every Australian household and vehicle, we could save $1.7 trillion by 2050 on energy costs, while also decarbonising our economy. Once a home is fully electric with solar and a battery, they can save over $1000 per year on fossil fuels and no longer be locked into the spiralling prices of gas and coal-powered energy. The bigger savings are in also electrifying our vehicles, where driving on sunshine is 2c/km and driving on fossils is 20c/km, worth $23000 dollars a year in additional savings to most Australians.
As we move to a totally renewable energy system, customer-owned solar panels, batteries, electric vehicles, and other machines will play a critical role. Customers will provide energy services to the electricity market at the local level. If the system is designed correctly, incorporating households and vehicles into the electricity market will increase reliability and resilience and reduce the total cost of energy for all of us. I believe in a policy of grid-neutrality, treating households and vehicles as national infrastructure, just as we treat snowy hydro and transmission projects.
Allowing households with solar panels, batteries and electric vehicles to trade electricity with their neighbours will level the playing field between energy consumers and the big energy companies. If we
position the Australian household as the linchpin with primacy, they could be playing an active part in the market to bring down the energy cost for everyone.
This is why I started Rewiring Australia and the lighthouse pilot project of Electrify 2515. We aim to be the first fully electric suburb and prove that the power grid can benefit from the extra electrification load. There are no simple solutions. We need a whole-system fix, and Vikki’s research clearly and succinctly outlines this. The results are timely and relevant.
With a policy focus on households and small businesses, Australia could turbocharge the energy transition and show the rest of the world the way out of the climate crisis. Our NEM was designed to protect generator assets and infrastructure assets. That was probably the right thing in the 1990’s, but it is no longer the right thing. Our AEC, AER, and AEMO, are not yet asking the right questions or modelling the right solutions - a classic example is that we consider the “lowest cost” system, only up to the point of generation and transmission, not the lowest cost of delivery to the Australian business or household. If we consider the latter, our regulatory and policy emphasis would be on bi-directional trading of electricity locally and incentivizing the maximum buildout of rooftop solar and community renewable energy. We need not tinkering at the edges, but wholesale reform of our markets and governance. If we want to get to the future sooner, and cheaper, we should begin a redesign of the Australian energy system with a blank sheet of paper.
Dr Saul Griffith Founder Rewiring Australia Founder Rewiring America
Dr Vikki McLeod initially trained as an electrical engineer and later moved into energy policy. She has worked across four government jurisdictions and at all levels of government including Australian parliament. She has developed and implemented energy and climate change policies and jurisdictional strategies.
Dr McLeod completed her PhD research project in 2023 through QUT’s Centre for ‘Clean Energy Technologies and Practices’ and ‘School of Design’. The project was entitled ‘The Role of Demand Flexibility Trading in Australia’s Clean Energy Transition: A Systems Thinking Approach’ and with an Australia’s clean energy transition and energy policy focus. Her academic research builds on her career achievements in designing and implementing energy and environmental markets to support the clean energy transition.
She was awarded a write-up scholarship from the RACE for 2030 CRC, and this report outlines some of the research findings from her PhD research. Dr McLeod is currently employed by Rewiring Australia as their Energy Market Reform Adviser. Vikki is also active in the community energy sector.
Dr Vikki McLeod
PhD, Masters Bus (Research), Grad Dip Bus Admin, B. Elec Eng
National Energy Market Reform Adviser, Rewiring Australia
June 2024
Systems thinking is an effective technique used to solve complex and transdisciplinary problems like the energy transition. A complex system cannot be analysed by simply breaking it apart into subsystems and subcomponents, it needs to be approached holistically rather than from a reductionist perspective.
Many policy-makers approach change management projects with linear thinking, even when the system is complex. Complex systems are distinguished from complicated or simple systems due to their interconnected and non-linear nature. As systems become complex, the system’s behaviour can become surprising. Any action on a complex system may not be easy to predict due to its non-linear nature, unpredictable side effects, unintended consequences, and interactions beyond the chosen system boundary.
Systems thinking is a technique used to solve complex and multidisciplinary problems like the energy transition. The practice method for complex systems is to understand the system before intervening or disturbing the system. It requires understanding how the system behaves by understanding the behaviours of individual participants and the challenges and problems they face; to then hypothesise and test policy interventions based on and depending on a holistic and inclusive understanding of the state of the system; and to observe the emergent behaviour of the system.
Complex system behaviour does not necessarily react predictably under any given condition, and historical behaviour does not necessarily indicate future behaviour.
Systems thinking practitioners have observed that as systems become complex, the system’s behaviour can become surprising. Complex systems display self-organisation, path dependency, emergence, coevolution, and adaptation attributes that cannot be predicted by understanding each component element separately. Identifying the intervention points may become counterintuitive or worsen the problems they seek to solve. A complex system such as the electricity system is typically adaptive or evolutionary and is influenced by complex social, political and technological dynamics. The challenge for developing, implementing, and managing policy under these conditions is understanding the system's complexity.
The practice method for complex systems is to understand the system before intervening or disturbing the system. It requires understanding how the system behaves by understanding the behaviours of individual participants and the challenges and problems they face; to then hypothesise and test policy interventions based on and depending on a holistic and inclusive understanding of the state of the system Systems thinking has two elements: analysis and synthesis. Analysis is a top-down approach to breaking down a complicated topic, reducing it and considering each component topic. Synthesis is a bottom-up approach combining two or more entities to form a more complex entity.
The first step in dealing with complex systems and managing a complex domain is to confirm the context as complex. Having established that it is a complex situation, observing the system's system dynamics holistically leads to identifiable patterns of system behaviour and the ability to discriminate in favour of desirable patterns that will support a more successful system dynamic relative to identified objectives. Electricity systems are undergoing an energy transition and a grid transformation challenge, where the difference between transition and transformation is the degree of management required. The challenge of transforming the electricity system is that it occurs within a complex adaptive system that is in transition. As such, electricity systems are considered complex systems and applying system thinking and complexity theory is appropriate.
Using systems thinking to develop energy policy is an emerging practice internationally, and policy design innovation is required. Systems thinking has been applied to energy transition policy in the USA, at the US Department of Energy, and by the UK Cabinet Office to design policy and manage, amongst other things, the increased risk of unintended consequences that may be detrimental to resilient operation. These risks include the potential for stranded infrastructure investments as system transformation progresses, the blockage of energy innovation and resultant value streams associated with new products and services, and the mismatch of policy directives concerning the operational realities related to the grid.
Successful policy innovation design requires an understanding of the system and knowledge of the technologies, markets, agents, institutions, and connections within the system. High levels of competence in policy evaluation and assessment are also required. Identifying the enablers of systems change, such as innovations in technology, practices and economics, or transformative level changes from change agents, including governments, may lead to counterintuitive policy intervention points. Alternatively, failure to recognise historical policies and regulations embedding system responses may cause policy interventions to worsen the problems they seek to solve. Global megatrends and behaviour change through shifts in social norms may also impact optimising policy intervention points.
Systems dynamic modelling aims to model and understand the system's dynamics to identify interventions to improve the system. It is a method of problem-solving in complex and trans-disciplinary environments. Systems thinking tools are used to guide and formulate system intervention hypotheses. Systems thinking has two elements: analysis and synthesis. Analysis is a top-down approach to breaking down a complicated topic, reducing it and considering each component topic. Synthesis is a bottom-up approach combining two or more entities to form a more complex entity. In practice, it requires understanding the system as follows:
Australian households and businesses are installing 3 gigawatts of rooftop solar annually and currently contribute over 11 per cent of total generation. As Australia moves towards net zero electricity grid and net zero economy, achieving the potential of end consumer owned Distributed Energy Resources (DERs) is a critical component of Australia’s Clean Energy Transition. Currently subject to policy debate is how this can be achieved at speed and scale. This case study applies systems thinking techniques to a complex problem of integrating higher Distributed Energy Resources (DERs) levels on the distribution network system.
A key factor in Australia’s clean energy transition has been the reducing costs and maturity of solar technology. Solar is now Australia's cheapest source of new electricity generation, even after accounting for grid integration costs. These DERs provide a significant challenge for network distribution.
Integrating solar and other DERs into the distribution network is critical to maximising local renewable energy generation and electricity system services, which coal and gas fired power stations currently supply. As these generators exit the system, additional sources of power system flexibility will be required at every level of the electrical system.
DERs are both the cause of system impacts and a potential solution. Uncontrolled and uncoordinated, DERs are a technical problem for the grid and the electricity system. However, controlled and coordinated, orchestrated DERs are potentially the solution to transitioning to a secure and reliable fully renewable electricity system.
While the challenge of integrating DERs is currently driving up the cost of transforming the electricity grid, with proper policy design and implementation, they can also be an economic solution.
The current business-as-usual situation and system dynamics are described as follows.
The high uptake of DERs by end consumers has led to the problem of reverse flow into the distribution network and left uncontrolled results in system impacts, such as the technical limits of the distribution network being exceeded. As a result of the increased stress, the business as usual (BAU) response under current regulations will be for the distribution network operator to augment the network. Accordingly, the distribution network will be identified for network upgrade. In turn, the costs of network upgrades are passed on to the end consumer, driving up their electricity bills. Experiencing increased electricity bills, more end consumers will invest in DERs (such as rooftop solar but increasingly battery energy storage systems (BESS), and electric vehicles (EVs) to control their electricity bills and take action on climate change. As more and more end consumers take up more
DERs, it results in compounding system impacts and increasing network stress, leading to increased network augmentation. As long as DERs remain uncontrolled, they cannot be utilised for demand flexibility and the BAU system dynamics automatically default to network augmentation. This cycle reinforces these behaviours and acts as a positive feedback loop or ‘vicious’ cycle. This dynamic is shown in Figure 1 as a Causal Loop Diagram (CLD), with the end consumers' behaviour and resulting course of action drawn as a feedback loop on the right.
The distribution network operators' (DNSP) behaviour and course of action are drawn as positive feedback or reinforcing (R) loops of investment behaviour on the left. In system dynamics, a positive (R) feedback loop is self-reinforcing - the more it works, the more it gains power. In the absence of reversing the reinforcing (R) cycle to a balancing (B) cycle, an intervention that delays or slows growth is preferable to letting the reinforcing loop (R) continue to gain power. Such a delay intervention will slow the reinforcing (R) loop and reduce the gain around the reinforcing (R) loop, giving the system time to function.
In the business-as-usual case, the interventions from the perspectives of the end consumer and DNSP can be observed, mapped, and examined for consequences (refer to Figure 1). Confronted by increasing electricity bills, the end consumer rationally intervenes by increasing their private investment in DERs 1 , such as oversized Solar PV, BESS and EVs.
From the DNSP perspective, in the situation of high uptake of Solar PV or EVs in a suburb with conducive socio-economic conditions, the result will be increased stress to the network and risk of blackout. The intervention options available to the DNSPs under current regulations are limited. The DNSP can only intervene as the regulatory environment allows, and their business model incentivises. The options available to the DNSP include investment in network augmentation or incentivising end
1 Note; where end consumers cannot purchase DERs such as Solar PV due to the upfront capital cost, or if they are renters, the end result is an inequity as these consumers cannot take action to control their electricity bills by investing in DERs and are consequently exposed to increased electricity bills.
consumers to participate in demand flexibility trading via network control tariffs. However, no financial nor regulatory incentive for DNSPs to choose demand flexibility over network augmentation exists.
By drawing a CLD, it may be possible to identify systemic responses as archetype s There are patterns or combinations of feedback loops, widely discussed in the literature, such as; the Vicious Cycle, Drifting Goals, Shifting the Burden, Limits to Success, Success to the Successful, Fixes that Backfire, Tragedy of the Commons, and Escalation (Kim & Lannon, 1997). By recognising the archetype, an archetypal system intervention can be selected and ascribed to resolve the system's problematic dynamic behaviour and guide the formulation of proposed system interventions and policy recommendations.
The CLD for the BAU reference case described above, reveals as a 'vicious cycle' archetype, and it can play out as an ‘addiction’ behaviour pattern.
In the case of the BAU reference case, the addiction is network augmentation driven by the DNSPs' regulated business model, which acts as a perverse incentive against demand flexibility trading.
With the successful trialling of Dynamic Operating Envelopes (DOE), DNSPs can increase the hosting capacity of the network and reduce network stress. From a system thinking perspective, the DOE intervention introduces a delay in the Causal Loop Diagram as the DOE increases the DER hosting capacity of any given network circuit by a significant margin.
Referring to Figure 2, the DOE is shown as a balancing loop as it alleviates network stress and is drawn with the symbol ‘II’ to indicate a slowing of the system dynamic and the reinforcing loop.
The BAU with the DOE option is revealed as a ‘Shifting the Burden’ archetype. This archetype hypothesises that a 'delay solution' may alleviate the problem symptoms. However, it reduces the imperative to implement a more fundamental solution. Therefore, the symptomatic solution systematically undermines the capability and development of a more fundamental solution.
The fundamental system dynamic problem is still present, with the cost of network augmentation being passed on to the end consumer. It results in increased electricity bills that act as a price signal for the uptake of more uncontrolled DERs, which results in additional network stress.
The current DOE and DER controls are classified as symptomatic solutions as they do not result in demand flexibility trading being used to alleviate the network stress.
Figure 2. Reference case redrawn to include End consumer and DNSP investment intervention options in blue (author)
The previous section concluded the BAU case with DOEs is revealed as a shifting the burden archetype.
In this situation, unable to control DERs as ‘demand flexibility’, the reliability and safety standards of the network become compromised. Where DERs remain uncontrolled and unvalued, network augmentation addiction will continue to make the reinforcing ‘vicious’ cycle worse. Addressing the ‘shifting the burden’ archetype, the choice is to apply either a symptomatic or fundamental solution.
As we have seen, symptomatic solutions do not address the fundamental problem; therefore, a fundamental reform of the system is still required.
While a fundamental solution to address the source of the 'Infrastructure addiction’ suggests a reform of the DNSP-regulated business model. This action alone could be considered as a ‘shifting the burden’ type solution.
Therefore, for the purposes of this case study, the policy challenge is defined as:
How can an investment environment be created for technical and business model innovation to result in the economic integration of DERs
Indicating the corresponding policy design hypothesis in this case study to be:
The creation of a market to trade in the services that DERs provide (DER services).
In order to develop the policy design hypothesis, we consider the following questions: DER services: What are they? Who has them and how do you get it off them?
DER Services: What are they?
'Distributed Energy Resources' (DERs) refers to any technology connected to the energy distribution system that can be controlled and operated flexibly. DER services can be produced by altering the end consumers' demand curve or generation levels, and they will present to the electricity grid as a load shimmy, shed, shift, or shape ((Alstone et al., 2016).
Common equipment and appliances well suited to producing DER services are household rooftop solar and appliances (HWS, Air Conditioning, pool pumps, Batteries and EVs), commercial building HVAC, and industrial processes enabled with technology (such as DREDs, ICT, comms, HEMS, BEMS, and optimisers). For example, charging or discharging a battery (BESS) and electric vehicle (EV) from rooftop solar at times of high solar generation on the network.
DER services can be commoditised and traded only if they can reliably respond to a command by a third party (such as the market or network operator) within the required timeframe and are delivered where and when needed.
It will then be ‘aggregated’ with other end consumers' DER services in the same area and traded as marketable amounts. The 'Aggregator' can then sell it into the wholesale market or a (yet to be established or designed) local energy market.
The 'need' for DER services depends on local factors such as distribution network or upstream transmission congestion or constraint conditions, local outages, time of day, local generation levels, and prevailing electricity prices.
Dimensions, specific technical, geographic and cost attributes describe DER services:
• Dimensions include up / down direction, rate of change, time between trigger and starting time, and duration.
• Technical attributes include controllability, predictability, response time, delivery time, and availability.
• Geographical attributes include proximity to the location where the DER services are needed (e.g., a substation catchment area) and where trade is considered to occur.
• Techno-economic attributes include the cost of technology and the cost of delivering to the geographical location relative to other technologies and options.
Rather than a single application for DER services, there is a mix. DER services that can be used for:
• Electricity system services for use by the Distribution Market Operator (DMO)
• Balancing services for use by the Distribution System Operator (DSO).
• Active network management for use by the network operator (DNSP) to alleviate congestion and constraints on the network.
Given the right policy design and energy market reforms, a trade in DER services can be created.
It will require the removal of disincentives from the DNSPs regulated business model to trade in DER services in favour of network augmentation.
Align incentives and introduce government support for the market to develop from a niche to a reliable and cost-effective product to alternative options such as diesel or gas peaking plants.
Key to the trade of DER services is the ability to control and coordinate the end consumer's owned appliances and equipment and ‘deliver’ to the purchaser. The end consumer will need to agree to participate in the trade and ideally not be able to detect or be inconvenienced by interruptions or have the option to override interruptions.
As the market matures, different ownership structures will likely develop. For example, network-owned batteries for active network management to avoid costly network augmentation, third-party-owned generation connected to the distribution network, such as a ‘Community Battery’, ‘Solar Bank’, or ‘Virtual Power Plant’ (VPP), and flexible generation sources.
As the purchaser, the DMO / DSO / DNSP will compare the relative economics and other factors of procuring DER services directly from the end consumer or market intermediaries.
Table 1 is a summary of the potential DER services trade against the four demand flexibility categories (Alstone et al., 2016):
• A ‘shimmy’ requires a fast response and balances electrical power system demand to supply. The market operator will use shimmy actions as electricity system services. Batteries and EVs are best placed to provide shimmy actions.
• A ‘shed’ action reduces peak demand and supports the system in emergency or contingency events. Any discretionary load can provide a shed action and be used by either the market operator or the network operator.
• A ‘shift’ action moves energy demand to times when there is a surplus of renewable energy generation. A typical ‘shift’ action is to increase behind-the-meter energy consumption when there is a surplus of solar generation. A shift action can be used to reduce network congestion and network constraints.
• A ‘Shape’ action helps accommodate higher levels of renewable energy generation. A shape action is typically energy efficiency or permanent shift of load. It permanently reduces or matches demand permanently to times of high solar generation.
Table 1 DER technology, value drivers and application characteristics mapped by author against Demand flexibility categories (Alstone et al., 2016)
Purchaser TMO / DMO TSO DSO DSO
Response Time Seconds 10 min 30 min Permanent
Flexibility
Source Storage. Fast response flexible demand or flexible generation. Storage. Demand response. Flexible demand or flexible generation Storage. Demand response. Flexible demand or flexible generation. Permanent reduction as energy efficiency and shift of load to times of high solar generation.
Service to the Power System Fast frequency support Voltage support. Active network management of network Voltage support. Active network management. Reduce demand on distribution network
Application
Value Drivers to the electricity System
Ancillary Services. Balancing services. Unplanned maintenance. Loss of generation. Defer network augmentation. Planned maintenance. Optimise RE generation. Defer network augmentation. Optimise RE generation. Defer Network augmentation.
Safety, reliability, security, affordability. Avoided reliability penalties
Value Drivers to the End consumer
Receive payment for DER Service
Safety, reliability, security, affordability. NPV of avoided generation and network expenditure
Receive payment for DER Service. Avoided peak demand charges.
Safety, reliability, security, affordability. NPV of avoided generation and network expenditure
Receive payment for DER Service. Avoided peak demand charges.
Safety, reliability, security, affordability. NPV of avoided generation and network expenditure
Receive payment for DER Service. Avoided peak demand charges
Societal Value Carbon abatement. Economic Efficiency. Productivity. Carbon abatement Economic efficiency. Productivity. Carbon abatement. Economic efficiency. Productivity. Carbon abatement. Economic efficiency. Productivity.
Who has them and how do you get it off them?
A critical measure in affordably managing the clean energy transition is sourcing and procuring low-cost demand flexibility from end consumers. Demand flexibility is produced when end consumer-owned DERs (appliances, equipment, and industrial processes) reliably respond to a market signal and are thereby commoditised as DER services.
In most cases, reliably producing DER services will require facilitation by a third party, such as an Aggregator, or Virtual Power Plant operator or other non-traditional business model (NTBM). The NTBM facilitates this by addressing barriers to trade, such as providing information, financing, and installing trade-enabling technology.
The enabling technology is needed to support trading and delivery of DER services to market where and when required. In addition to enabling technologies, several other contractual preconditions must be met before the end consumer can participate in trade.
Consumer protections are needed to protect consumers from deceptive, misleading conduct, information, or unfair contracts. Requirements to act in the end consumer's best interests are needed, or there will be risks to the courts of setting new consumer protection law precedences
As the end consumer purchased these appliances and equipment not to produce DER services but for the services they provide (heating, cooling, etc.), the market for DER services are leveraging the end consumer's private investment to benefit the electricity system as a whole.
When the DER services are on-sold, the end consumer will expect profit sharing, compensation for wear and tear and inconvenience (if any), and the ability to override the DER Service market request if their circumstances do not allow it.
The NTBM will recover the costs of producing DER services plus their required profit from the DER services market, where they are on-sold and used as power system services and active network management alternatives. DER services will then compete with other sources of power system flexibility and network service on quality defined by delivery, reliability, volume criteria and available substitutes.
As DERs interact with the current electricity grid in unprecedented numbers, any unintended consequences must be understood within the context of the specific system. It is, therefore, necessary to embrace the complexity of the energy transition challenge across technical, commercial, policy and consumer attributes, to understand the implications on the overall system and how the system can be acted upon through policy interventions.
The high-level vision is to create a market to trade in DER services, with a goal of achieving DER integration (i.e. minimising the negative impacts of DERs) while maximising the economic potential of providing DER services as electricity system services.
Currently, DER services are a niche product and “produced” and “consumed” behind the meter by the householder or business to optimise their electricity bill and maximise their behind-the-meter solar
generation. There are barriers to supply at scale and the production capacity of the market to supply DER services.
It is anticipated that by 2040, Australia will need approximately 12 GW of dispatchable demand flexibility (NERA, 2022). This indicates the scale of production that is needed and the potential value of the market for demand flexibility. For demand flexibility delivered through DER services to be produced and traded at large scale, DER services would need to be widely commoditised and traded in the Australian National Electricity Market (NEM).
The design of the NEM determines who has 'access' to value, and this access shapes the future electricity system. The energy market rules define what commodities are valued and traded, and who and how these commodities are to be traded. Therefore, for DER services to be traded into the electricity system, the energy market rules would need to change from their current form. Achieving a cost-effective and energy-efficient transition requires an electricity market design that values and incorporates the characteristics of renewable energy technologies which by nature are variable.
The challenge is not simply to align the electricity market design with the technical essential of supporting the electrical system as if ‘power system flexibility’ exists in a socio-technical vacuum. The real challenge is to embrace innovation flexibility whilst incorporating resilience and adaptability into a regulatory environment that is itself being shaped by international and national political pressure to increase climate ambition This is a market where government and end consumers are becoming the primary stakeholders.
The starting point in conceptualising a trade or an idealised market for DER services is considering the supply-side and demand-side dynamics. To understand the multiple elements that result in the supply and demand for DER services, the trading dynamics and how they interact to determine the price of DER services.
It is known that each of the individual supply and demand elements has barriers. However, it is only when all barriers to trade are addressed through policy interventions that the individual elements, acting like links in a chain, can result in a functioning market for DER services.
The ‘DER services’ supply side is defined as the dynamic between the essential market participants plus a third element, which is the condition that results in DER services being produced at scale. These three elements function as the supply chain for delivering DER services to the market.
The first element is the end consumer, householder, or business, who owns and operates the DER assets.
The second is the new market entrant with a Non-traditional Business Model (NTBM). The NTBM addresses the barriers to participation by end consumers and facilitates the trade of DER services into the market. The NTBM is likely to be a Virtual Power Plant operator, Aggregator, or retailer who acts as
a market intermediary. It could also be an Original Equipment Manufacturer (OEM), contractor, local council, or even a community group.
There are well-documented barriers to participation by the end consumer in the electricity market, such as lack of information, search costs, split incentives and upfront capital costs. The upfront capital expenditure (CAPEX) to purchase DERs is a barrier to many end consumers.
Even if consumers have the technology and are enabled to trade, navigating the electricity market is confusing and takes expert knowledge and effort. End consumers' level of trust in the energy market is at an all-time low, with a majority having doubts that their best interests are considered. There are also questions regarding how DERS services are valued, how this value is shared, and a unique possessiveness referred to as "my stuff". For example, the end consumer needs to be comfortable and accepting of the potential interruptions to service, understand and agree to specific contract terms and conditions, and be satisfied with the level of compensation offered.
Barriers to participation by NTBMs may also delay the development of end-consumer-focused business models. NTBMs, such as Aggregators and VPPs, will need to ensure the end consumers' DERs are enabled to respond to a third-party market signal. They will aggregate DER services to meet the minimum trading volumes, ‘deliver’ them, and then undertake financial acquittal following market settlement. A contractual arrangement between the end consumer and the NTBM is needed to participate in trading DER services.
The NTBM will judge the investment attractiveness of participating in DER services trading as a function of the risk and the ability to make a profit. Barriers include the inability to value stack and, therefore, achieve profitability, the lack of enabling technology and infrastructure, and the lack of policy clarity.
The NTBM will manage the costs to enable and address the barriers to the householders’ ability to produce DER services
The DER services value chain is a function of revenue to the DER services producers and the investment attractiveness of trade and economies of scale resulting from production capacity. Both the end consumer and the NTBM will have threshold criteria around trading conditions and compensation.
As with any other trading business, the supply of DER services is a function of production costs. In the case of DER services trading, it is shaped by techno-economic and socio-technical factors, end consumer behaviour and decision-making.
Without an attractive investment environment supporting NTBM participation, the NTBM will not emerge, and without the NTBM, the end consumer is unlikely to participate in trade.
A partnership between the end consumer and the NTBM is the most likely favourable supplier context for demand flexibility trading at scale.
The 'consumers' of DER Services are on the demand side, including the market operator, system operator, and network operator.
This case study examines the distribution network operator (DNSP) purchasing DER services as nonnetwork services instead of the more costly option of network augmentation. DER service delivery, where and when required, is central to any DER service trade. In the case of DER services traded as nonnetwork services, it must be in the DNSPs network area.
The DNSP faces barriers that impact its demand for DER services, such as network reliability standards, the cost of DER integration infrastructure and who pays for it, and regulatory and business model uncertainty.
The DNSP may risk penalties if reliability standards are not maintained, and DNSPs will seek emergency override protections.
While the regulatory incentive toward network augmentation remains, the DNSP will choose this more expensive option over the cheaper option of purchasing DER services for non-network services. Even if network regulators were inclined to review the perverse regulatory incentive toward network augmentation, a regulatory reset occurs once in five years.
Another complicating factor is the ownership of DSNPs, which presents a vested interest in the status quo. Without resolving the DNSPs' utility business model and economic regulation, the transition to high levels of renewable energy will be less than optimal and result in higher energy bills for the end consumer.
The 'Demand Management Incentive Scheme' (DMIS) is an imperfect incentive for DNSP to trade in DER services and therefore classified as a 'substitute'.
The current network regulations and market rules limit their operation to the DNSPs network applications, and the community does not benefit from them. Under the DNSP's control and operation, Community Batteries' CAPEX is expensed and used in preference to end consumer-sourced DER services from behind-the-meter batteries and EVs.
A Causal Loop Diagram (CLD) is now drawn, showing the supply dynamics on the left, demand dynamics on the right, and barriers in red (refer to Figure 3).
In the Australian National Electricity Market (NEM), the policy environment is a complex intersection of engineering, electricity markets, economic regulation, technology development, planning, endconsumer behaviour, and decision-making. It is challenging to design policy in such a complex environment, particularly when policymakers have a perspective of one dimension only.
As previously shown, causal loop diagrams (CLDs) are a systems thinking tool that helps policymakers navigate complex environments by allowing them to appreciate the system. CLDs also guide policy design and improve the general feasibility of policy interventions.
For this case study, the policy design aim is to create a market that results in the trade of DER services and an investment environment for technology and business model innovation. The policy interventions emerge from the systems thinking process and are designed to address barriers to the trade in DER services, shift behaviour patterns, align incentives and support innovation.
The causal loop diagram (CLD) is now used to guide the development of policies to address the barriers to the supply of DER services. The barriers are shown in red on the left side of Figure 4.
Policy intervention options are now designed to:
1. Address the barriers to the End Consumer producing DER services and their participation in the trade of DER services.
2. Improve the attractiveness of the DER services trading environment and participation in businesses with non-traditional business models, such as aggregators and virtual power plant operators, and support business model innovation.
3. Improve the production capacity of DER services at scale and support technology innovation.
The policy intervention options are now discussed in detail.
End consumers (including renters) already face challenges when affording and selecting appliances and comparing retail electricity offers. In an environment of declining trust in energy providers, asking end consumers to participate in and enable their appliances with technologies to become DER services trading ready and enter into trading contracts adds multiple layers of complexity to decision-making.
Any policy interventions that address the end consumers’ barriers to participation and simplify, protect and improve consumer interests and experience are warranted. These policy interventions could include information and rebates at the point of sale, financing, improved community-level governance, consumer protections, government guarantees and facilitation, government-defined cost reflective two-way tariffs and value sharing, programs to prepare the end consumer for DER services trading, alignment and improvement of appliance energy efficiency and enabling technology technical standards and grid interaction.
Any policy intervention that improves the certainty of the DER services market would support the trade in DER services and improve the investment attractiveness of DER service trading to NTBMs. For example, the market could be designed so that the NTBM could 'value stack' by accessing multiple DER service applications and markets simultaneously and generating an income stream from each. For example, NTBMs could 'value stack' by selling DER services into separate markets for electricity, frequency response, network support, and carbon abatement.
With improved market conditions, the NTBM is more likely to innovate and become entrepreneurial in responding and adapting to uncertain and complex environments. Any policy interventions that de-risk participation would support the NTBM and, in turn, help the NTBM to address the barriers to participation by the end consumer.
Currently, DER service trading in Australia is a niche market.
Without experience in DER service trading, governments are unlikely to oblige market participants to trade in DER services trading as alternatives to current practices. Any policy interventions to:
• Support the DER service production capacity from a low-level, low-risk environment to building a trade.
• Support the marketplace capability to deliver large volumes and with high reliability.
• Improve the economies of scale.
are appropriate. Referred to here as a ‘Demand Flexibility Roadmap’, it would include clear statements of direction, targets, innovation policies and funding.
The causal loop diagram (CLD) is now used to guide the development of policies to improve the demand for DER services. The demand side with barriers shown in red is on the right side of Figure 4.
The fundamental assumption of market economics is that the market determines the price of DER services as a function of supply and demand dynamics. That is a function of production costs, the demand for, and the cost of DER services substitutes, such as the network augmentation option. For trade to be successful, the supply and demand dynamics need to work optimally to achieve DER services trading at scale.
Policy interventions are needed to improve the demand side dynamics and to:
1. Incentivise the ‘Consumer’ to purchase demand flexibility.
2. Improve the reliability of DER services delivered.
3. Improve the overall demand and supply dynamic.
DER services have several potential applications and consumers with differing circumstances and perspectives on what price they are prepared to purchase.
Referring to Figure 4, the consumer is listed as the Distribution Network Service Provider (DNSP), Distribution System Operator (DSO), Distribution Market Operator (DMO), Distribution Network Operator (DNO).
The DSO and DMO are identified here as there is an international trend to introduce these new actors with specific roles to support DER service trading. However, while there are discussions regarding their introduction in Australia, this has yet to happen. Therefore, this case study considers DNSPs as the 'consumers' purchasing DER services for non-network services to manage network constraints and congestions and as active network managers.
The DNSP is a regulated monopoly, and its business model is defined by the National Electricity Law and economic regulation.
Some discuss splitting the DNSP into the DSO and DNO, at which time the roles, responsibilities and business model could be revisited. Until this happens, the business model is revisited only once every five years as a regulatory reset.
In the absence of a 'Regulatory Roadmap ' or another form of 'Regulatory Innovation ' from the government or the market bodies, there is no incentive for DNSPs to change current business practices.
Regulatory innovation could include using the ‘regulatory sandbox’ provisions or other regulatory innovations to encourage DSNPs to experiment with DER services for active network management ahead of the 5-year reset period.
In the absence of clear direction from governments and market bodies, uncertainty regarding the future operation of the energy system will continue.
Under the current regulations, DNSPs must meet certain reliability of supply standards or risk financial penalties if they breach these standards. The DNSPs' experience in the trade of DER services considers third party-supplied DER services for active network management to increase their risk of breaching system security and reliability standards.
Australian DNSPs lack experience using DER services trading for active network management. The absence of reliability standards and a lack of confidence in the DER services product will continue to be barriers.
A future electricity system will require new system security and planning frameworks. Policy options for consideration include amending the standards or ensuring that the trade in DER services does not compromise technical and reliability standards.
Technical standards and governing legislation must be updated and enforced. Findings from 'Project Symphony' identified the poor uptake of standards by manufacturers and technology providers, resulting in limited interoperability between DER devices and technology platforms (ARENA, 2022b). It is particularly relevant with the uptake of EVs and bidirectional charging. For DER Services to be viable in network constraint management, the technical standards must consider safety, interoperability, cybersecurity, energy efficiency, energy management, and optimisation issues.
Any policy interventions to support DNSPs in gaining confidence in third party-supplied DER services are appropriate. Policy interventions include government involvement, resourcing of the Australian Standards committees, and ensuring consistency across legislation and regulations for appliances, equipment, solar, batteries, and electric vehicles.
As discussed, network augmentation is the primary substitute for trading in DER services. Due to the current market rules, DNSPs have a perverse incentive to choose network augmentation over trading in DER Services.
As the BAU CLD outlines, the current DNSPs' utility business model and economic regulation results in the system dynamic archetype of “infrastructure addiction” or "gold plating", as it is referred to in the electricity industry. While this perverse incentive remains, the transition to high levels of renewable energy will be less than optimal, resulting in higher energy bills for the end consumer.
Removing the perverse incentive from the DNSPs’ business model would require economic regulatory reform, such as:
● Changing the current Capex-Opex to a Totex revenue calculation.
● Alignment of penalties and incentives to trade in DER services.
● Redesigning Demand Management Incentive Scheme (DMIS).
● Introducing performance - based regulation.
An example for Australia is the UK’s use or regulatory design and the economic regulation of their Distribution Network Operators DNO, the Australian equivalent of DNSPs.
With the introduction of the RIIO-ED and RIIO-2, the UK has used regulatory design to introduce penalties and incentives as drivers for the DNO to trade DER services. RIIO-ED is short for ‘Revenue = Incentives + Innovation + Outputs’ electricity distribution (ED) price control determination. The RIIOED determines the business and revenue model of the DNO. There is £100 million for priority areas of energy storage, flexibility resource development and trading, plus £450m available through Ofgem’s RIIO-ED2 price control as funding for network and energy security innovation.
It has resulted in DNOs tendering directly with end consumers (or their aggregators, traders, and agents), starting from a low-risk and low-volume base. This approach has seen the trade of DER services rise to 3,700 MW, a 76 per cent increase since 2020 (ENA-UK, 2022). By the end of 2022, Ofgem had funded 60 innovation projects related to flexibility, with project benefits estimated in the multi- billions (OfGEM, 2023).
Even with supportive regulatory design and aligning incentives, a commitment to creating an environment for and direct support for technology and business model innovation is needed. This includes supporting the development of trading platforms as a mid-term objective. Additional incentives would enable DNSPs to trade in DER services, creating investment certainty for NTBMs and end consumers. Other facilitation actions include publishing data on network constraints and congestion status to the market as a ‘hot spot map’.
Government oversight and regulatory determination on tariff setting, value sharing, and consumer protections are also needed. Commercial and contract standards must be considered, including consumer protections where third-party control of behind-the-meter appliances is involved.
Finally, policy interventions are needed to improve the overall demand and supply dynamic between the cost of producing DER services and the network augmentation option. A contract for the difference to cover the price gap (if any) would provide the DER services industry access to a guaranteed market, thereby improving investment certainty and achieving economies of scale. For example, the Australian Government's Capacity Investment Scheme could be extended to include distribution network-level investments.
The causal loop diagram (CLD) is now updated to show the supply-side policy interventions in green and how they address the barriers in red (refer to Figure 5).
The previous section outlined several policy intervention options but not the implementation pathway. Some policy options apply to the end consumer and are suitable for the Australian or State and Territory governments to implement. Other options are considered as NEM market reforms. The NEM market bodies, including AEMO, AER, and AEMC, play a crucial role in implementing these under the oversight of the Energy and Climate Change Ministerial Council (ECMC).
The ECMC comprises the Australian, State, and Territory government Ministers of Energy and Climate Change and votes and acts in consensus. Once identified, the policy interventions will inform who has responsibility for initiating change. Individual Energy Ministers will act in accordance with their jurisdictional politics and with pressure from their constituents. The governance surrounding the NEM
is complex and intricately intertwined. It involves the NEM market bodies (AEMO, AER, and AEMC), which provide advice to and receive direction from the ECMC.
The NEM energy market reforms are occurring within a complex adaptive system that is in transition, involving multiple state government jurisdictions with different resources and policies , and transitioning at different rates The institutional and governance arrangements of the NEM are subject to a regulatory process, which may not always be responsive to political pressures or disruption. Australia’s energy market reform pathway is incremental, with market bodies ‘layering’ rule changes to the existing centralised dispatch arrangements rather than designing an optimised decentralised system (Judson et al., 2020).
The energy transition is an intersection of multiple regimes, from the political, engineering, and institutional power of incumbents and our institutions.
Changing a complex and institutionally invested system is challenging, as resource flows, business models, and regulations serve and reinforce the current system’s purpose. In an environment dominated by market incumbents, with potentially inherent conflicting interests and system-leveraged institutional bias towards the status quo. These incumbency issues (may or may not) extend to the advice the Energy Ministers receive.
The NEM governance arrangements become even more challenging when dealing with a transitioning electricity system and sourcing services from end consumer-owned assets. The system will only change with an unbiased and comprehensive approach that the incumbent actors do not unduly influence. Governments must not only invest in independent advisory bodies and institutions but also engage with the systemic regulatory change that the energy transition requires.
Policy and regulatory reforms can be either orderly or disruptive and subject to governments' ability to propose solutions that are politically and socially acceptable, technically, and economically viable. Key questions then emerge about how to manage the pressures of the energy transition and societal expectations around affordability.
Questions remain about the NEM's design and governance and the consequences of the complex NEM governance arrangements. Specifically its inability to adapt and keep pace with the energy transition and hence it’s suitability for high levels and different scales of renewable energy generation.
In a policy environment like this, a reconfiguration pathway to transition to a decentralised energy system will likely only emerge with concerted and coordinated government policy intervention. This highlights the need for a systemic approach, a critical assessment and review of the NEM governance arrangements, and the identification of comprehensive solutions.
Without a systemic approach, the energy system will remain resistant to a fundamental change of mission or purpose. The urgency and importance of an integrated and coordinated policy with an appreciation of the technical aspects of the electricity grid cannot be overstated. To do otherwise would result in increased risk of unintended consequences; stranded infrastructure investments; stifled innovation and blocked value creation and value streams associated with new products and services (Taft, 2019).
Section 3.1 and 3.2, identified the end consumers, NTBM, and DNSP as pivotal actors in the energy system transition. The need to redefine and split the DNSPs' utility business model was also recognised as crucial in the realignment process towards an optimised decentralised energy system. This case study's system of interest is the integration of DERs onto the distribution network and the trade of demand flexibility as a source of network and power system services
From a systems perspective, creating ‘Local Renewable Energy Zone’ (LREZ) would be a significant policy tool for optimising the energy transition pathway. Declaring a LREZ would allow for innovation and be the pathway to regulatory and NEM reform. The objective would be to design policies and undertake regulatory reform in an integrated manner and ultimately a whole-system transition
Such a zone would be a bounded, place-based, and coordinated approach to large-scale innovation. It would be an opportunity to roll out successful ARENA trials and support technical, business model, and regulatory innovation.
Ideally, a LREZ would be declared in each of the 18 distribution network areas and, at minimum, in each state and territory to allow for experimentation and with consideration of state differences in policy, geography and demographics.
The LREZ would need equal focus, planning or resourcing on both the supply and demand sides of the trade of DER services while supporting regulatory, technology, and business model innovation.
To emphasise the importance of this and referring to Figure 5, the DER services demand side is labelled 'Local Renewable Energy Zone' (LREZ) and the production the supply side is labelled 'Zero Emissions Community' (ZEC)
The choice of the Zero Emission Community (ZEC) label reflects the 200-plus communities across Australia that are self-organising to promote the uptake of DERs.
The ZEC refers to the suite of policies directed at the community that lives within the LREZ. It is designed to support the community to transition and work to achieve very high to saturation levels of rooftop solar storage flexible demand conditions
The choice of the Local Renewable Energy Zone (LREZ) label contrasts with the existing government policy of large-scale 'Renewable Energy Zones' (REZ). It calls for the same level of investment, focus, and planning that is given to the transmission-level REZs to be given to the LREZ
A LREZ would be physically located within the distribution network and the downstream catchment of a zone substation. Therefore, the objective of the LREZ is to implement the identified policies in an integrated manner to:
• Create an opportunity for energy market reform and market realignment.
• Create a pathway to achieving community-scale electrification at pace and scale and demonstrate the potential of the demand side.
• Test methods to promote and gain community social licence for saturation levels of DERs and preparedness of the end consumer for DER Services trading.
• Support retailers, trades, and skilled labour to provide the end consumer with information at the point of sale.
• Coordinate state based rebates and incentives to achieve high levels of DER uptake.
• Support and activate LREZs to promote trust in action and manage expectations and consumer protections.
• Research into how end consumers participate in, understanding their experience of, and the barriers to demand flexibility trading would be a valuable input into current NEM reforms and policy design debates.
A key finding of the systems thinking approach is the need to create an investment environment for the trade in DER services by incentivising or otherwise changing the DNSP regulated business model. That is split the functions of the DNSP into Distribution System Operator (DSO) and Distribution Network Operator (DNO).
The five-year DNSP regulatory reset would be the time to introduce reforms, but the opportunity cost of delay also needs to be considered. Options include shortening the time between resets or introducing fixed and flexible components of the regulated asset base to allow for greater regulatory agility and innovation. Another option is to declare the LREZ a regulatory sandbox and allow for any sovereign risks to be managed under such a provision.
As a minimum the LREZ would be an opportunity to:
• Roll out smart grid technology to support saturation levels of DERS, including the rollout of AMI and Dynamic Operating Envelopes (DOEs).
• Define and test the models of Distribution Network Operator (DNO), Distribution System Operator (DSO) and Distribution Market Operator (DMO) roles and responsibilities in action.
• Split the DSNP business model into DSO and DNO, re-regulate and align incentives to trade in DER services.
• Test the ability of the DMO to coordinate the distribution-level activity and bid into wholesale markets 2
• Use a redesigned Demand Management Innovation Scheme (DMIS) to incentivise the trade in DER services.
• Allow for participation by new market entrants with Non-traditional business models (NTBM).
• Test successful ARENA-funded trials in interaction and other regulatory, NTBM, and technical innovations.
• Explore how multiple markets may be accessed and how value streams can be stacked to improve the economics of DER integration.
• Incentivise the trade of DER services by extending the Australian Governments Capacity Investment Scheme or introducing a contract for difference.
• Test performance-based or other forms of agile economic regulation.
Such an approach underscores the need for a unified jurisdictional approach, with the Australian, State, and Local governments collaborating on the implementation of a comprehensive and national roadmap for DER integration and trading DER services. Such a roadmap requires explicit statements of intent, targets, industry, innovation, and procurement programs aligned with the energy transition objectives.
Developing an energy transition policy through such an integrated approach will necessarily involve jurisdictional-level policy responsibility. Given the energy security, decarbonisation, and local and private economic benefits of high levels of electrification, the benefits will also be split over national, state and local jurisdictional levels
2 Via the Wholesale Demand Response Mechanism, energy, frequency, reliability and carbon markets.
The results of this research project and the application of a systems thinking approach to energy policy development is a novel application for navigating Australia’s transition to clean energy.
An energy system in transition is complex, involving many levels, regimes and disciplines, including technical, regulatory, market economics, policy, and politics. However, our ability to deal with complexity is limited and we seek to simplify complex problems so we can apply simple solutions.
Applying a systems thinking approach and complex system dynamics has been demonstrated as a useful tool to navigate policy development in complex environments.
It allows the limitations and constraints of the system to be made explicit, grouped, and prioritised. It also allows for the easy conceptualisation, design and mapping of policy interventions.
The systems thinking process has proven to not just be a means to an end but a powerful tool in its own right.
Not only does it facilitate problem conceptualisation, but it also facilitates open discussion and collaboration between colleagues to design a comprehensive solution development.
In practice the diagrams have resulted in shifts of perspective and realisations of the nature of embedded problems and the consequences of the current reform pathway. It empowers policymakers, equipping them with a whole-system perspective to confidently address complex energy issues.
As the clean energy transition gains momentum, the need for tools that foster collaboration, extend stakeholder involvement, and promote co-design opportunities becomes increasingly urgent.
Systems thinking tools, with their potential for positive, productive, and far-reaching impacts, could be the key to shaping the future of energy policy and energy market reforms. It is underappreciated and under-studied area of research that holds significant potential for shaping the future of energy policy.
Active Network Management (ANM) . Methods other than network augmentation to manage constrained, congested or under-utilised electricity network to manage the electrical network within its technical limits Includes export limitation, timed export methods, demand flexibility control systems, digital platforms etc.
Advanced Metering Infrastructure (AMI) An integrated system of smart meters, communications networks, and data management systems that provide a two-way digital link between the DNSP, retailer, aggregator and customers.
Balancing Services corrects the supply/demand balance in response to minor deviations in demand or generation supply. If there is a minor imbalance between supply and demand, the system sends control signals out to restore the supply/demand balance so frequency is maintained within the normal operating band of 49.85 hertz (Hz) to 50.15 Hz
Behind the meter . Generation equipment or energy technology assets that provide or use energy onsite without passing through the meter and located on the end consumers' side of the meter.
Control technology Allows for the active control of the customers’ electricity demand profile - such as diverting solar output to battery and in response to a market price signal. DERs are integrated with a controller unit which enables remote control and can optimise the operation of the system in response to remote communication signals. For DERs to be integrated into the DER platform they need to be able to provide defined power products, be visible on the platform, and be remotely scheduled and dispatched.
Demand Flexibility. A sub-category of 'power system flexibility' which is an essential element of the operation of the electricity system to balance the electricity supply to the demand. Demand flexibility is produced through the control of 'Distributed Energy Resources'. Demand flexibility will present to the electricity grid as a ‘shimmy’, ‘shed’, ‘shift’ or ‘shaping’ of end consumers’ electricity demand curve relative to a specific time frame or response time of seconds, minutes, hours, days or years.
DER Services . DER Services are produced through the control of DER assets. DER services are consumed by the end consumer as electricity consumption, the market operator as balancing/security/reliability services and the DNSP for active network management
Demand response enabled devices (DREDs) . Allows control of DERs appliances including by a thirdparty. Commonly fitted to Electric Hot Water Systems and Air-conditioners and combined with dedicated communications for rapid demand response deployment.
Demand Management Incentive Scheme (DMIS) payments regulated by AER to the DNSP as an incentive to use demand management in preference to network augmentation and with the objective of managing the increasing costs of network charges.
Distributed Energy Resources (DERs) A DER is any distribution network connected resource capable of being controlled to supply DER services. DERs include standby generation, solar PV, interruptible demand and processes.
Distribution Network The electricity network typically consists of high voltage transmission network and the medium and low voltage distribution network.
Distribution Network Service Provider The DNSP is the owner and operator of the distribution network.
Distribution System Operator (DSO) is a new role with responsibility of balancing electricity supply and demand at the distribution network level. To optimise their network for maximum DER operation, identification of network constraints and the need for services to alleviate those constraints.
Distribution Market Operator (D MO) manages distribution level markets and services as well as the integration with the energy, FCAS and other system services.
Dynamic Operating Envelopes technical innovation solution that varies import and export limits from an End consumer premises, dynamically over time and location based on the available capacity of the local network or power system as a whole.
Hosting Capacity The amount of Solar PV that can be added to distribution system before control changes or system upgrades are required. Although not a hard threshold, adding new Solar PV systems may ultimately trigger upgrades or changes to the electrical distribution system.
FCAS Contingency services . FCAS Contingency Services (or system stabilisation services) are sourced from equipment that can respond to the frequency without a central command or instruction. There are six (6) distinct requirements that is either Raise and Lower and for different response times of fast (6 second), slow (60 second) and Delayed (5 minute) time frame.
FCAS Regulation Services (or load matching) . A small adjustment of up or down (raise or lower) is sent from AEMO to enable generators (and loads) to alter their generation output, thereby restoring the frequency to 50Hz.
Innovation . The invention, development, and diffusion of new goods, services, or production processes. Innovation is the study of how society expands its production possibilities frontier.
Interoperability Interoperability is the ability for different information technology systems, devices and software applications to enable two-way communication, use, and exchange of data accurately, effectively, and consistently.
Non - network services / non - network alternatives. Use of demand flexibility as active network management and as an alternative to network augmentation
Non - Traditional Business Model (NTBM) . Describe new electricity market participants with a business model conducive to demand flexibility trading such as an Aggregator, Virtual Power Plant Operator, Community Battery Owner or operator, Flexi-trader. The NTBM is customer facing and address the traditional barriers to participation in the market by end consumers the owners of DER Services.
Performance-based regulation is an alternative form of regulation compared to traditional rate-based economic regulation. It is used internationally for electricity network transformation outcomes.
Renewable Energy Zones (REZ) The Renewable Energy Zones are areas with high renewable energy resource potential. By declaring a REZ, governments and regulators can engage strategically with local communities and energy generation investors to deliver new generation and storage potential to the system as coal generation plants retire. Supported by new or strengthened transmission network, REZ will be important to maintaining reliability and enable an orderly and coordinated transition to renewable energy.
Smart Meters . Smart meters collect data at regular intervals (typically 5 minutes) for the purpose of billing and financial settlement provided they meet with wights and measures standards. Smart meters are enabled with communication technology to allow for remote communication, connection and disconnection.
T otal expenditure' (TOTEX) is a network price determination framework. Under the TOTEX framework, the distinction between capital (CAPEX) and operating expenditure (OPEX) is removed and regulated DNOs were given a single expenditure allowance. It involves changes to how expenditure allowances are set, measured and reported.
Two-sided market. Is one of the proposed ‘Post 2025 energy market’ reforms and represents a highlevel vision and considerations of how DERs can be integrated at the energy market, technical and consumer levels.
Vehicle to Grid (V2G) Electric vehicles have batteries that can vary in size from 30kWh to well over 100kWh. Emerging as a technology, Vehicle to Grid (V2G) electrical design allows the vehicle battery to discharge and be usable as an energy generator when it is connected to the grid. Typical household batteries are 10kWh, so the capacity of an electric car battery is substantial.
Virtual Power Plants (VPPs) . The aggregation and direct load control of multiple end consumers’ loads to derive local grid and market benefits. A number of VPP trials have been carried out, particularly in South Australia, aimed at controlling a fleet of batteries and at demonstrating the ability of a fleet to operate as effectively as larger single batteries.
V2L (Vehicle to load) , V2H (Vehicle to Home), V2B (vehicle to building) and V2X (vehicle to everything) . Also, part of the electric vehicle lexicon. Definitions should distinguish between grid synchronised and off-grid applications (e.g. the battery drives standalone equipment like power tools) but the terms are often used ambiguously.
Virtual Power Plants (VPPs) The aggregation and direct load control of multiple end consumers’ loads to derive local grid and market benefits. A number of VPP trials have been carried out, particularly in South Australia, aimed at controlling a fleet of batteries and at demonstrating the ability of a fleet to operate as effectively as larger single batteries.
Alstone, P., Potter, J., Piette, M. A., Schwartz, P., Berger, M. A., Dunn, L. N., Smith, S. J., Sohn, M., Aghajanzadeh, A., Stensson, S., Szinai, J., & Walter, T. (2016). Final Report on Phase 2 Results 2015 California Demand Response Potential Study Charting California’s Demand Response Future (LBNL-2001114). Lawrence Berkeley National Laboratory. https://etapublications.lbl.gov/publications/demand-response-potential-california
ENA-UK. (2022, 14 July 2022). Britain breaks flexibility records for four years running – almost 4GW tendered in 12 months https://www.energynetworks.org/newsroom/britain-breaks-flexibility-records-for-four-years-running-almost-4gwtendered-in-12-months
Kim, D. H., & Lannon, C. (1997). Applying systems archetypes. Pegasus Communications. https://thesystemsthinker.com/applying-systems-archetypes/
NERA. (2022). Valuing Load Flexibility in the NEM. Prepared for the Australian Renewable Energy Agency. Australian Renewable Energy Agency. https://arena.gov.au/knowledge-bank/valuing-load-flexibility-in-the-nem/
OfGEM. (2023). Call for Input on the Future of Distributed Flexibility2023. Office of Gas and Electricity Markets. https://www.ofgem.gov.uk/publications/call-input-future-distributedflexibility?dm_i=1QCB&trk=public_post_comment-text