

OUTCROP
Newsletter of the Rocky Mountain Association of Geologists
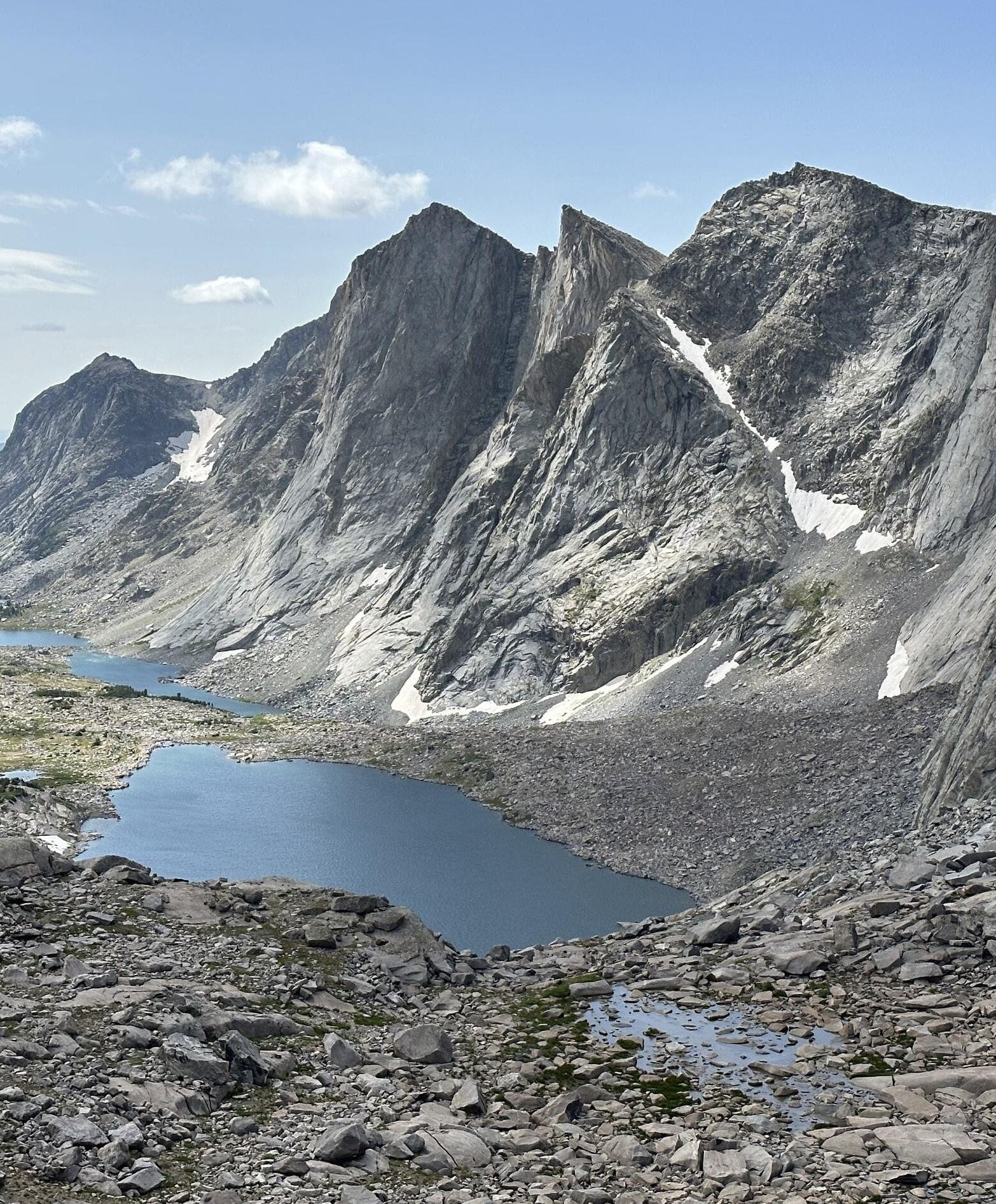
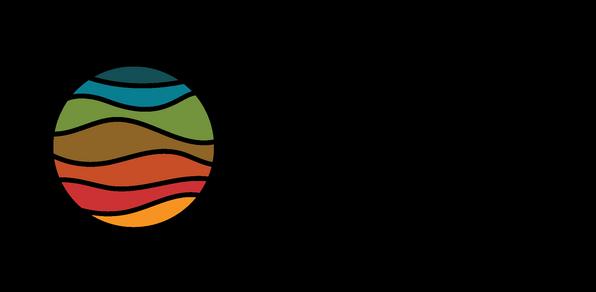
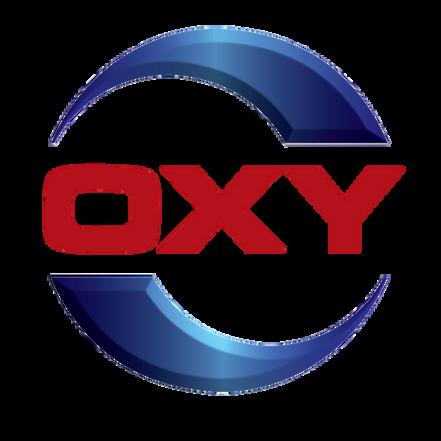
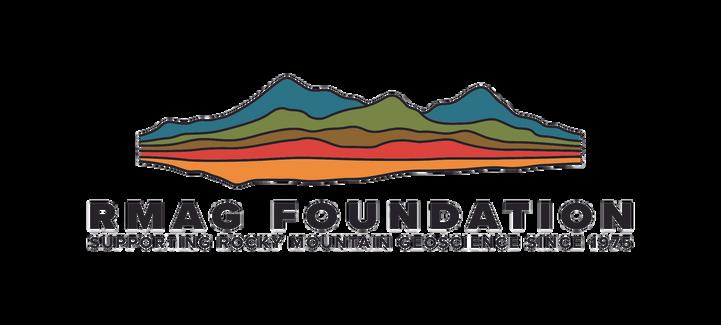
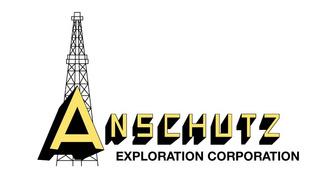
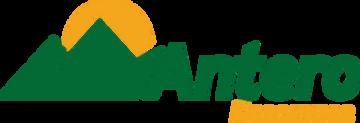
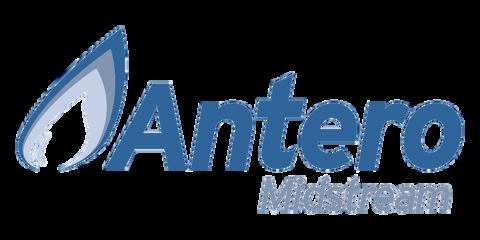
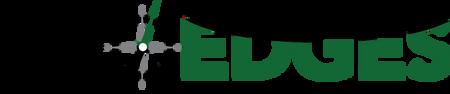
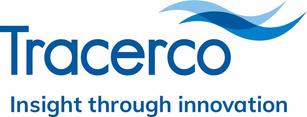
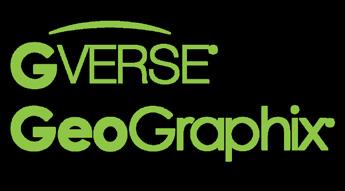
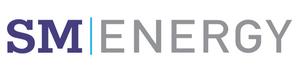
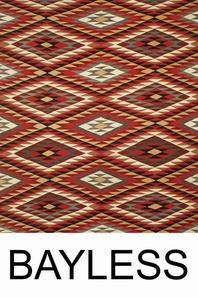
OUTCROP
Newsletter
of the Rocky Mountain Association of Geologists
730 17th Street, B1, Denver, CO 80202 • 720-672-9898
The Rocky Mountain Association of Geologists (RMAG) is a nonprofit organization whose purposes are to promote interest in geology and allied sciences and their practical application, to foster scientific research and to encourage fellowship and cooperation among its members. The Outcrop is a monthly publication of the RMAG.
2025 OFFICERS AND BOARD OF DIRECTORS RMAG STAFF
PRESIDENT
Matthew Bauer matthew.w.bauer.pg@gmail.com
PRESIDENT-ELECT Sandra Labrum slabrum@slb.com
1st VICE PRESIDENT
Nate La Fontaine nlafontaine@sm-energy.com
1st VICE PRESIDENT-ELECT Rachael Czechowskyj sea2stars@gmail.com
2nd VICE PRESIDENT Ali Sloan ali@sloanmail.com
2nd VICE PRESIDENT-ELECT Lisa Wolff lwolff@bayless-cos.com
SECRETARY Drew Scherer latirongeo@gmail.com
TREASURER
Astrid Makowitz astridmakowitz@gmail.com
TREASURER-ELECT
Walter Nelson wnelson@integratedenergyresources.com
COUNSELOR
John Benton jbenton@haitechinc.com
ADVERTISING INFORMATION
Rates and sizes can be found on page 50. Advertising rates apply to either black and white or color ads. Submit color ads in RGB color to be compatible with web format. Borders are recommended for advertisements that comprise less than one half page. Digital files must be PC compatible submitted in png, jpg, tif, pdf or eps formats at a minimum of 300 dpi. If you have any questions, please call the RMAG office at 720-672-9898.
Ad copy, signed contract and payment must be received before advertising insertion. Contact the RMAG office for details.
DEADLINES: Ad submissions are the 1st of every month for the following month’s publication.
The Outcrop is a monthly publication of the Rocky Mountain Association of Geologists
DESIGN/LAYOUT: Nate Silva | n8silva.com
EXECUTIVE DIRECTOR
Bridget Crowther bcrowther@rmag.org
LEAD EDITOR
Danielle Robinson danielle.robinson@dvn.com
CONTRIBUTING EDITORS
Elijah Adeniyi elijahadeniyi@montana.edu
Marlee Cloos marlee.cloos@bpx.com
Nate LaFontaine nlafontaine@sm-energy.com
RMAG CODE OF CONDUCT
RMAG promotes, provides, and expects professional behavior in every engagement that members and non-members have with the organization and each other. This includes respectful and inclusive interactions free of harassment, intimidation, and discrimination during both online and in-person events, as well as any content delivered by invited speakers and instructors. Oral, written or electronic communications that contain offensive comments or demeaning images related to race, color, religion, sex, national origin, age, disability, or appearance are not appropriate in any venue or media. RMAG reminds members of the diversity and mission statements found on our website. Please direct any questions to staff@rmag.org
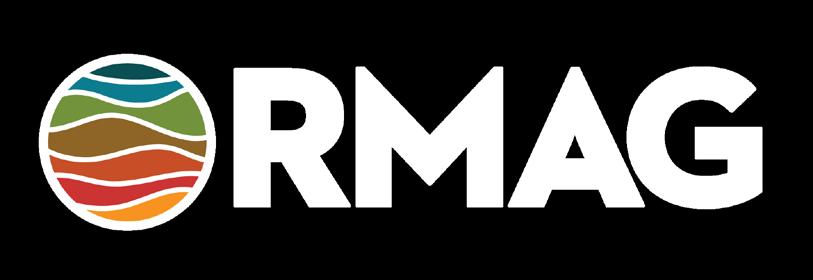
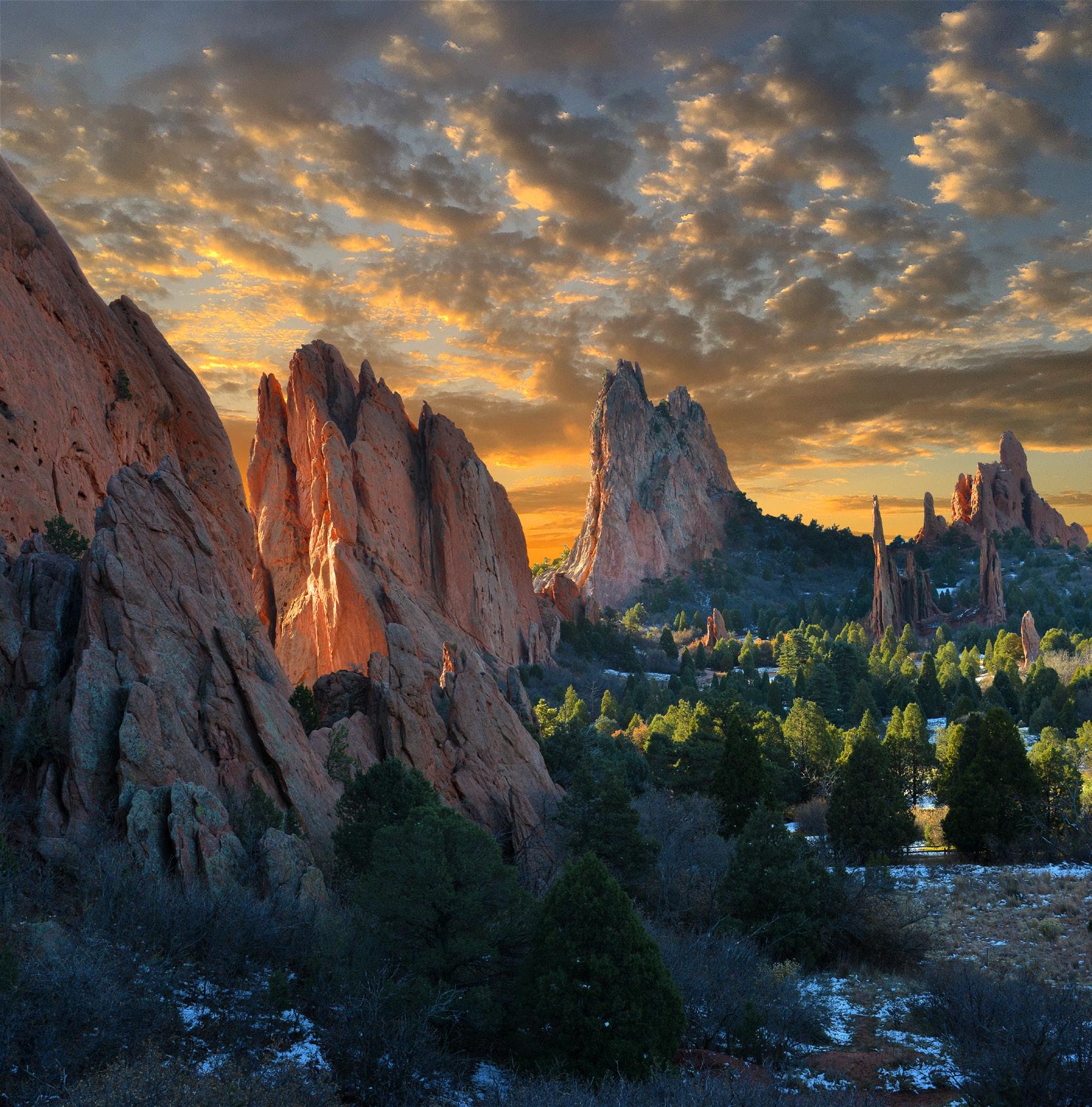
HAPPY HOUR HAPPY HOUR HAPPY HOUR
OUTCROP
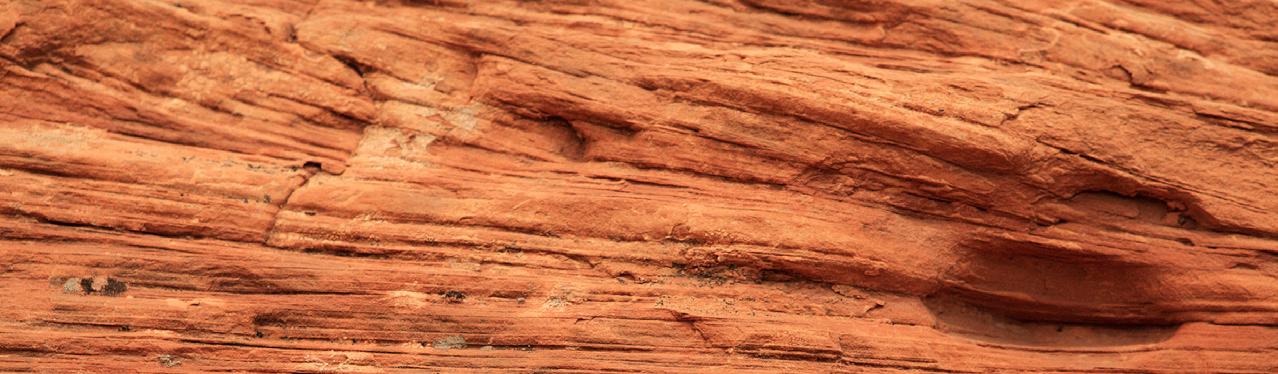
FEATURES
6 2025 RMAG Summit Sponsorship Packet
14 Lead Story: Outstanding in the Field, Since 1894 – A Brief History of Brunton, with an Update Regarding its New Ownership and a Paragraph about the Importance of Analog Measurements
30 Mineral Of The Quarter: Serpentine
NEWS
2 RMAG Summit Sponsors 4 RMAG Networking Events
11 RMAG Coffee Hour 25 RMAG Happy Hour
27 North American Helium & Hydrogen Conference
DEPARTMENTS
10 RMAG February 2025 Board Of Directors Meeting
12 President’s Letter 24 Hybrid Lunch Talk: Jonathan McKenna
Hybrid Lunch Talk: Mike Kuligowski
In The Pipeline
Welcome New RMAG Members!
Outcrop Advertising Rates 51 Advertiser Index
COVER PHOTO
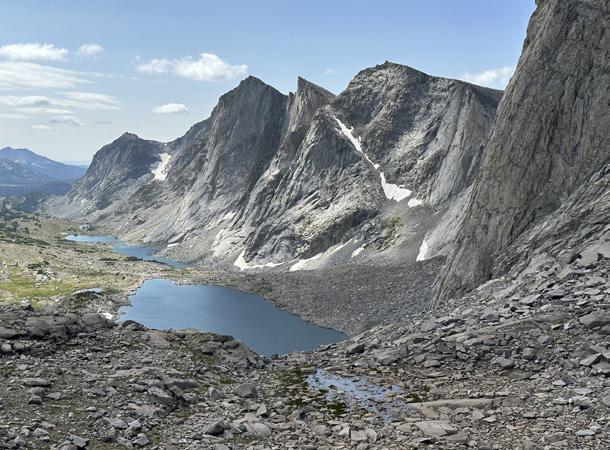
Raid Peak Basin in the Winds by Lauren and Dave Heerschap.
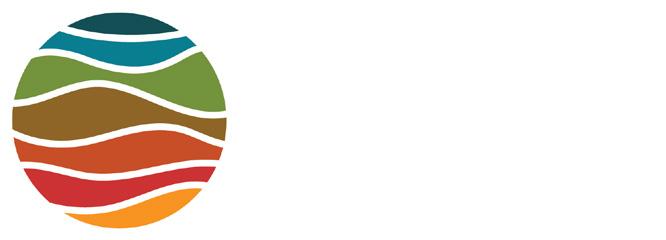
MEMBERS
EVENT ATTENDEES
WEBSITE VISITORS
OUTCROP READERS
EMAIL SUBSCRIBERS
NETWORKING EVENTS
CONTINUING EDUCATION EVENTS
FIELD TRIPS
COMMUNITY CONTACTS IN 2024YOUR SUMMIT SPONSORSHIP DOLLARS SUPPORTED: 1,200 1,200 8,000 8,000 5,000 4,000 23 13 10
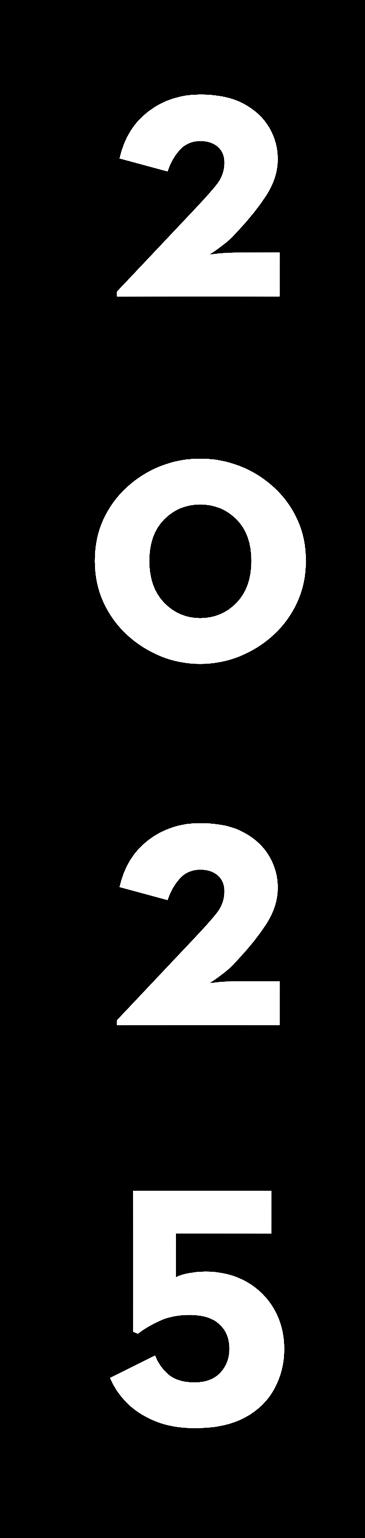
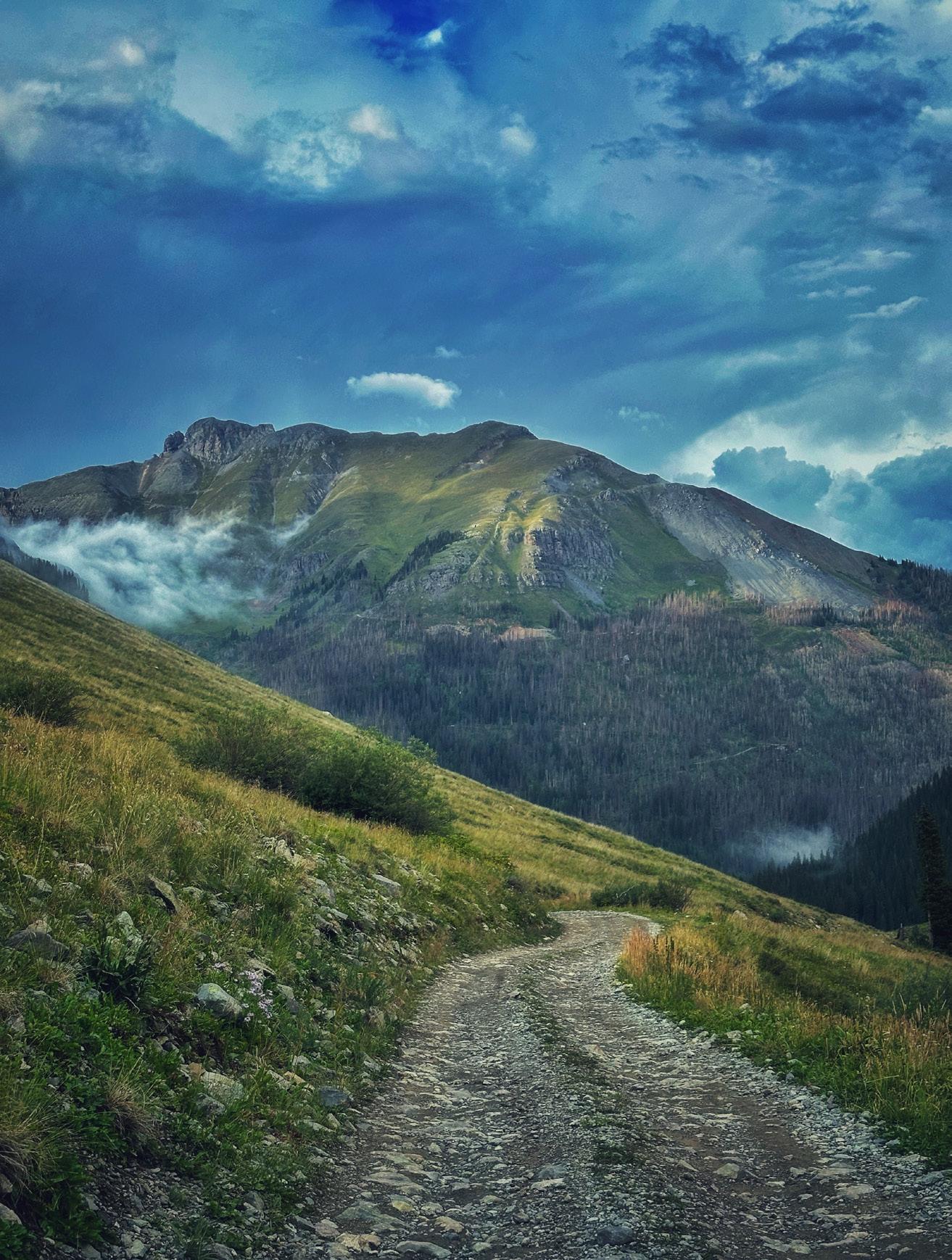
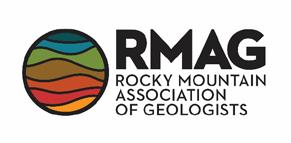
October 30, 2024
Geoscience Community:
We sincerely appreciate the support every Summit Sponsor and Event Sponsor provided over the past year. Your contributions are vital to the success of the Rocky Mountain Association of Geologists (RMAG).
In 2024, the RMAG was proud to host a dynamic lineup of events, including the CCS Workshop, which provided an in-depth look at advancements in carbon capture and storage. Members explored the beauty and geological wonders of the Colorado Rockies with ten diverse field trips and shared our passion for geoscience with students across the region through classroom visits and community festivals. Additionally, we fostered connections among members through monthly lunches, coffees, happy hours, and our annual Golf Tournament.
Looking ahead to 2025, we are excited about new opportunities for RMAG. Your sponsorship will help RMAG realize a robust calendar of continuing education opportunities, an exciting season of field trips, high-impact short courses, and a dynamic lineup of luncheon speakers. In April 2025, we look forward to the North American Helium & Hydrogen Conference, building on the success of our 2023 North American Helium Conference. Your sponsorship empowers RMAG members to impact the next generation at outreach events throughout the community and provides invaluable networking opportunities for the geoscience community. Furthermore, your financial support plays a crucial role in our publication efforts, which include the monthly Outcrop newsletter and the quarterly Mountain Geologist journal.
In recognition of your financial commitment to supporting geoscience in the region we recognize our sponsors through in-person signage, advertising on our website, publications, and social media promotions leading up to each event. With a LinkedIn group of almost 3,000 members, we ensure our sponsors are visible to the geoscience community for both virtual and in-person events.
Thank you to our current Summit Sponsors; we look forward to your continued support in 2025. For those considering sponsoring, we encourage you to explore the many benefits included at each sponsorship level and consider how you can promote geoscience in the Rockies. If an annual sponsorship doesn’t suit your company’s needs or if you wish to sponsor a specific event, please inquire about our single-event sponsorship opportunities. Feel free to reach out to our staff with any questions about sponsorship at bcrowther@rmag.org or by phone at 720- 672-9898 ext. 102.
On behalf of the RMAG staff, volunteers, and myself thank you all for your continued support, and we look forward to connecting with you in 2025.
Sincerely,
Matthew Bauer
Bridget Crowther 2025 RMAG President RMAG Executive Director
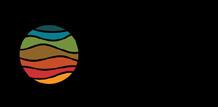
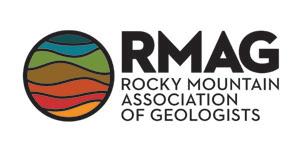
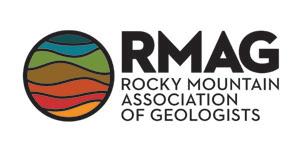
RMAG 2025 SUMMIT SPONSORSHIP
All sponsor benefits event tickets follow RMAG event registration deadlines. All benefits end 12 months after registration.
RMAG 2024 ANNUAL SUMMIT SPONSOR OPPORTUNITIES
Platinum Sponsor
Gold Sponsor
Silver Sponsor
Summit Sponsorship benefit term is for 12 months! Specify type of payment on signed form, and send logo and advertisements to staff@rmag.org
Company:
Company Representative:
Address:
City: State: Zip Code:
Phone: Email:
Payment Method: Credit Card ACH Check
Credit Card Information:
Select Card: AMEX Mastercard VISA Discover
Name as it appears on Credit Card:
Credit Card #:
Expiration Date:
Signature:
Security Code:
ACH: contact the RMAG office at staff@rmag.org for directions.
Mail Checks payable to RMAG:
Rocky Mountain Association of Geologists (RMAG)
730 17th Street, B1 Denver, CO 80202
RMAG events are subject to change. Cancellation or rescheduling of events does not give the sponsor the right to refund. Summit Sponsors will receive benefits at any new events added into the RMAG schedule.
Thank you for your generous support!
P: (720)672- 9898 staff@rmag.org www.rmag.org
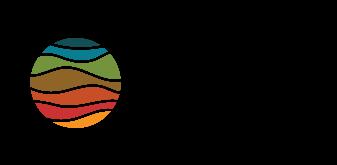
730 17th Street, B1 Denver, CO 80202
RMAG FEBRUARY 2025 BOARD OF DIRECTORS MEETING
By Drew Scherer, Secretary flatirongeo@gmail.com
As we roll into another exciting month, here’s a quick look at what’s been happening behind the scenes at RMAG!
The board met on February 19th to discuss our ongoing activities, and we’re thrilled to report that January was a fantastic month! Our events saw strong turnouts, especially the Luncheons and the Rock Busters’ Ball, and membership is on the rise, thanks in part to registrants from the Hydrogen-Helium Conference. Financially we are in great shape, with strong net operating revenue and market gains keeping us on solid footing.
Looking ahead, our committees are hard at work making sure there’s something for everyone this year. The Continuing Education team has lined up luncheon speakers through May (and February’s
event had an excellent showing!). The Membership Committee is cooking up some family-friendly recruitment events for spring and summer, and they have seen strong engagement at both Coffee and Happy Hour gatherings. If you haven’t been to one just yet, what are you waiting for? We hope to see you at some of our events soon!
On the publications side, we’re gathering articles for The Outcrop’s summer issues, and the next Mountain Geologist should be hitting the website around late February or early March. Meanwhile our GO Committee has been making waves at outreach events across the Metro area, if you’re looking for a chance to volunteer and share your passion for geoscience, now’s the time to get involved!
And of course, field season is right around the corner! The On-The-Rocks Committee has an incredible lineup of trips kicking off in May with a visit to the Glenwood Springs Caves and Carbonates. They’re also working to expand accessibility and trip diversity, including potential field courses like Wilderness First Aid training, stay tuned for more details!
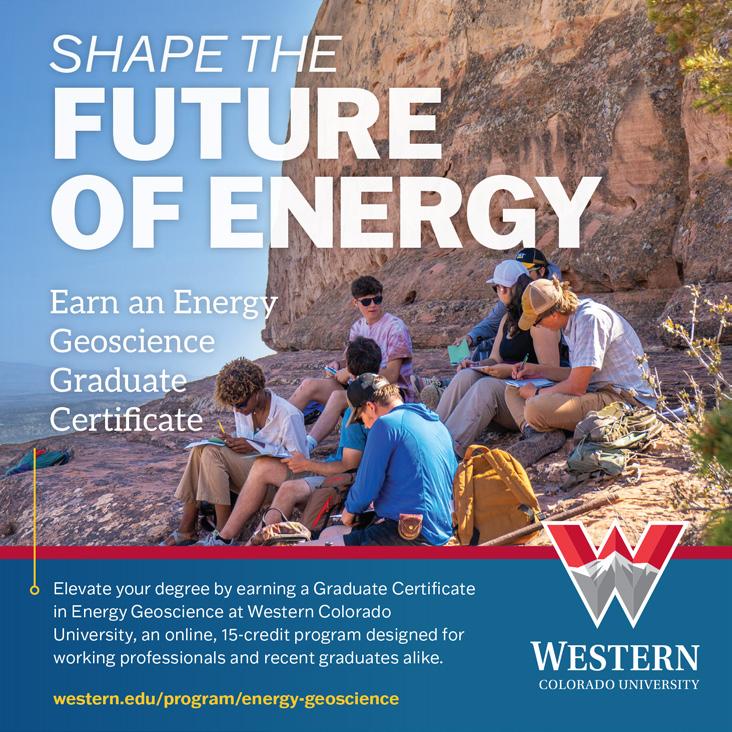
We hope you enjoy this month’s articles, and we can’t wait to check in with you again next issue. Until then, stay curious, stay engaged, and we’ll see you at an upcoming event! Happy reading!

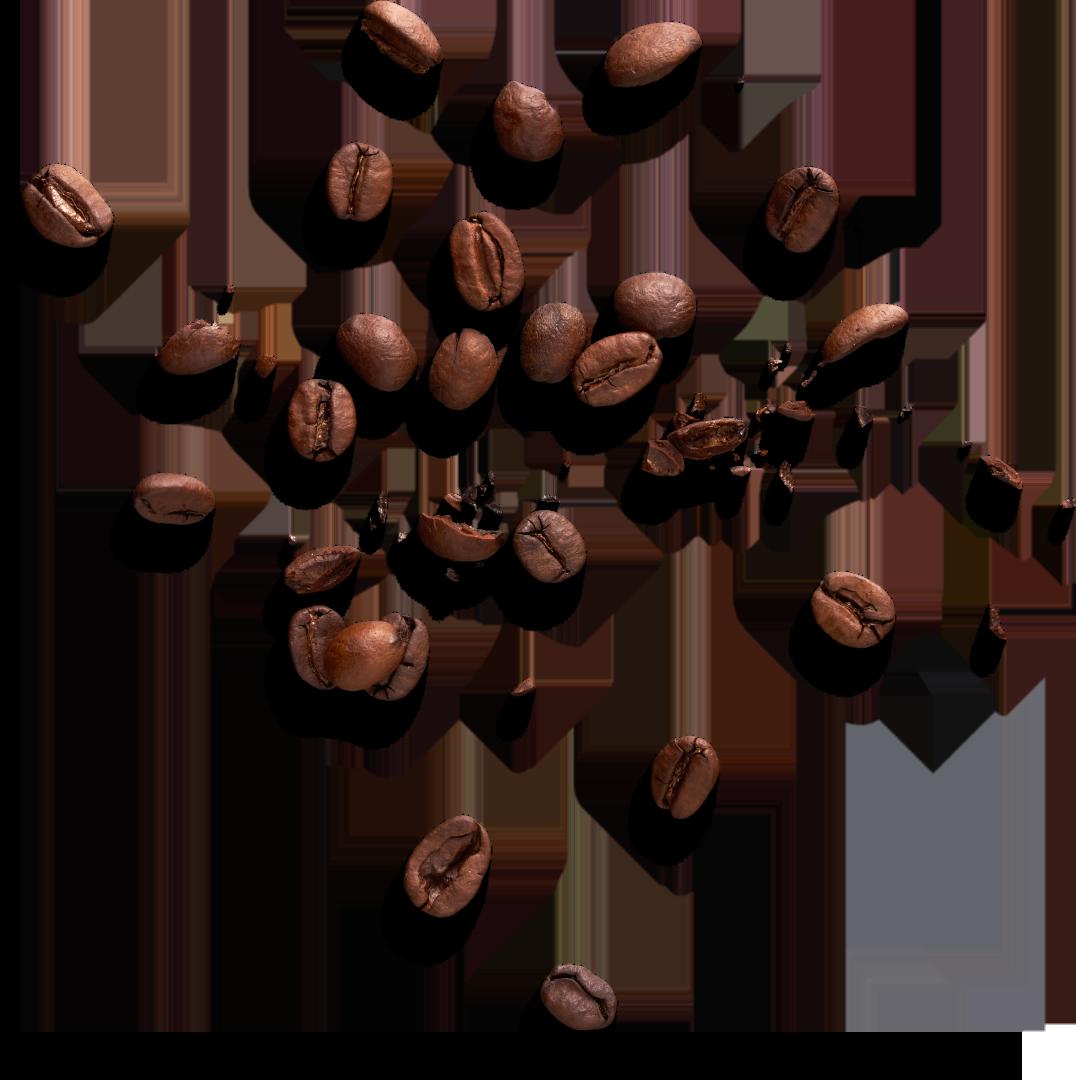

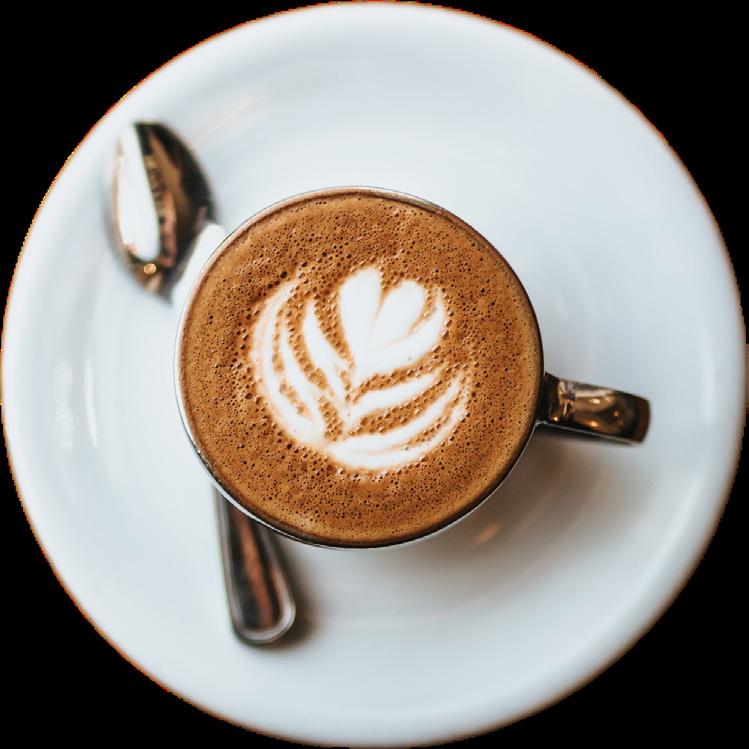
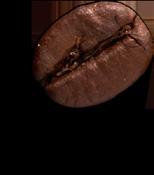
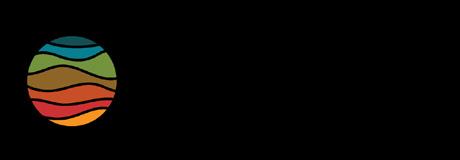
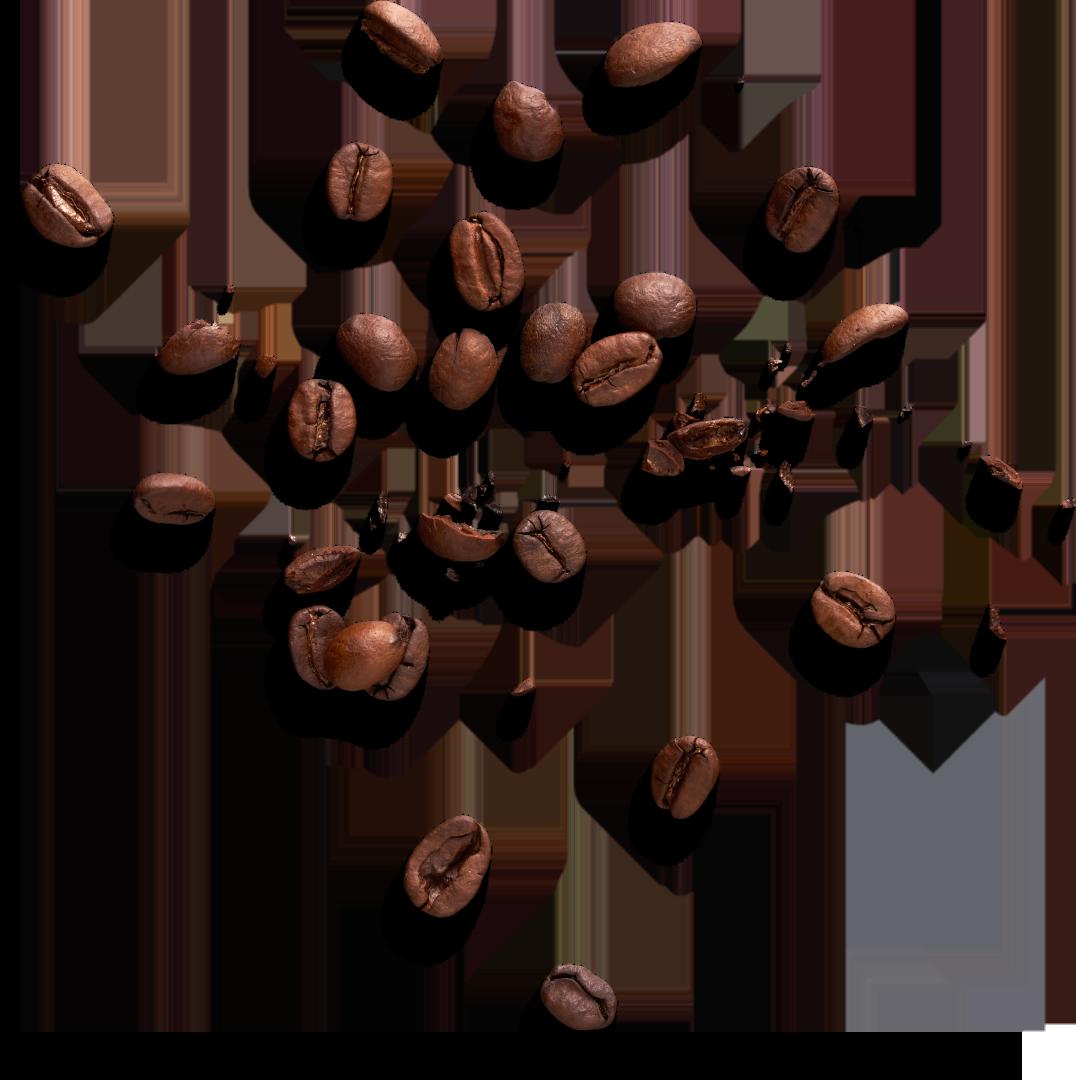



PRESIDENT’S LETTER
By Matthew Bauer
Dear Fellow Members,
Many of the historical color choices we’ve used in scientific visualization can either obscure or highlight trends, especially when applied to uneven color gradients. Crameri et al., 2020 offers a comprehensive discussion on the misuse of color in scientific communication and how the scientific community can improve. It’s definitely worth a read if you have a moment.
S&P Global’s Petra® software is widely used by oil and gas geologists. Have you ever wished you
could easily add additional color maps to Petra? With just a little Python code, we can export matplotlib color ramps as ZPL files, allowing them to be easily loaded into Petra. If one of the 170 color maps included with matplotlib doesn’t fit your project, check out PyPalettes, which offers over 2,500 additional palettes. The PyPalettes site also helps teams select appropriate colormaps, especially if you or a colleague have protanopia, deuteranopia, or tritanopia.
Stay Curious & Be Good to People,
Matthew W. Bauer, PG
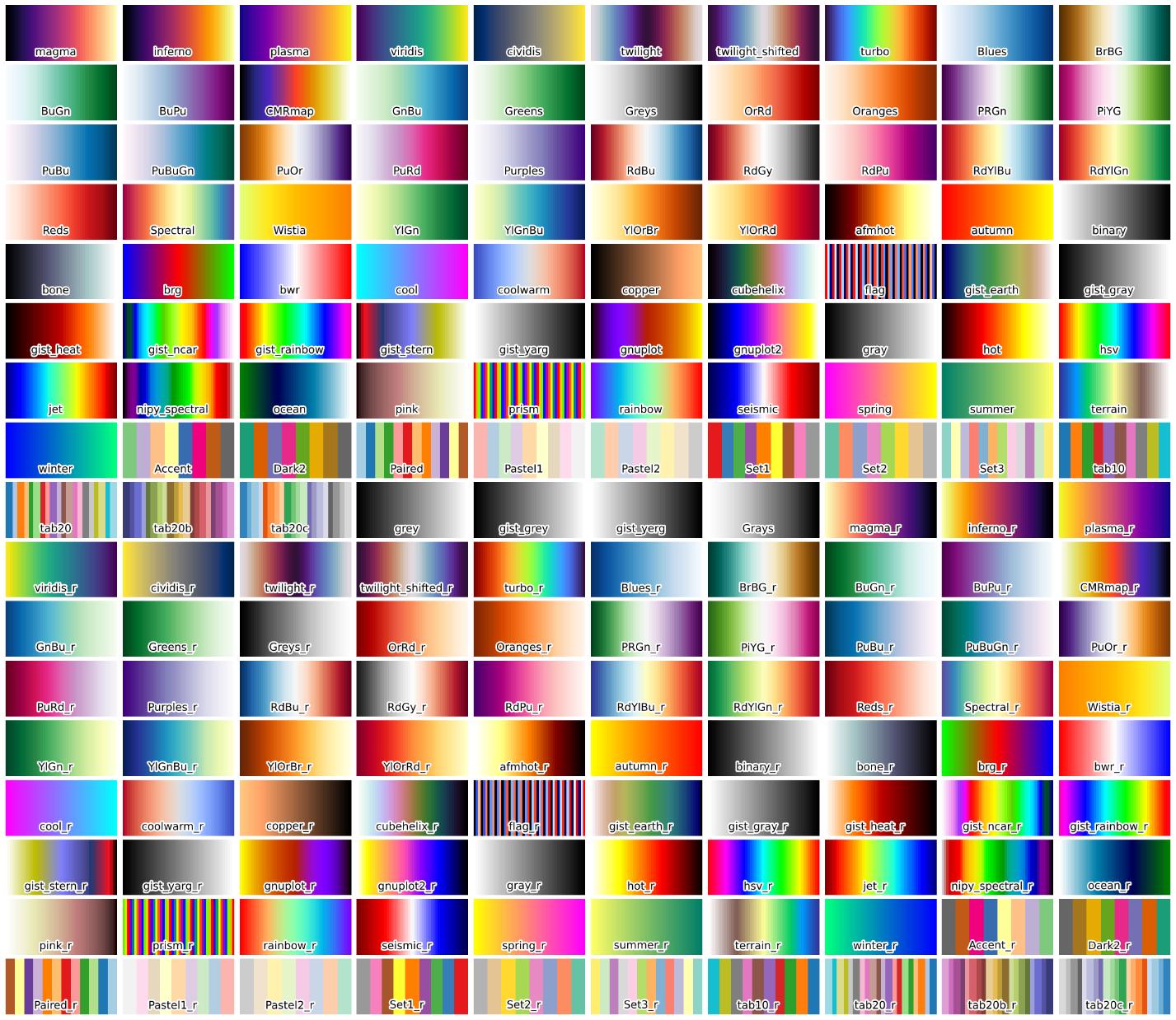
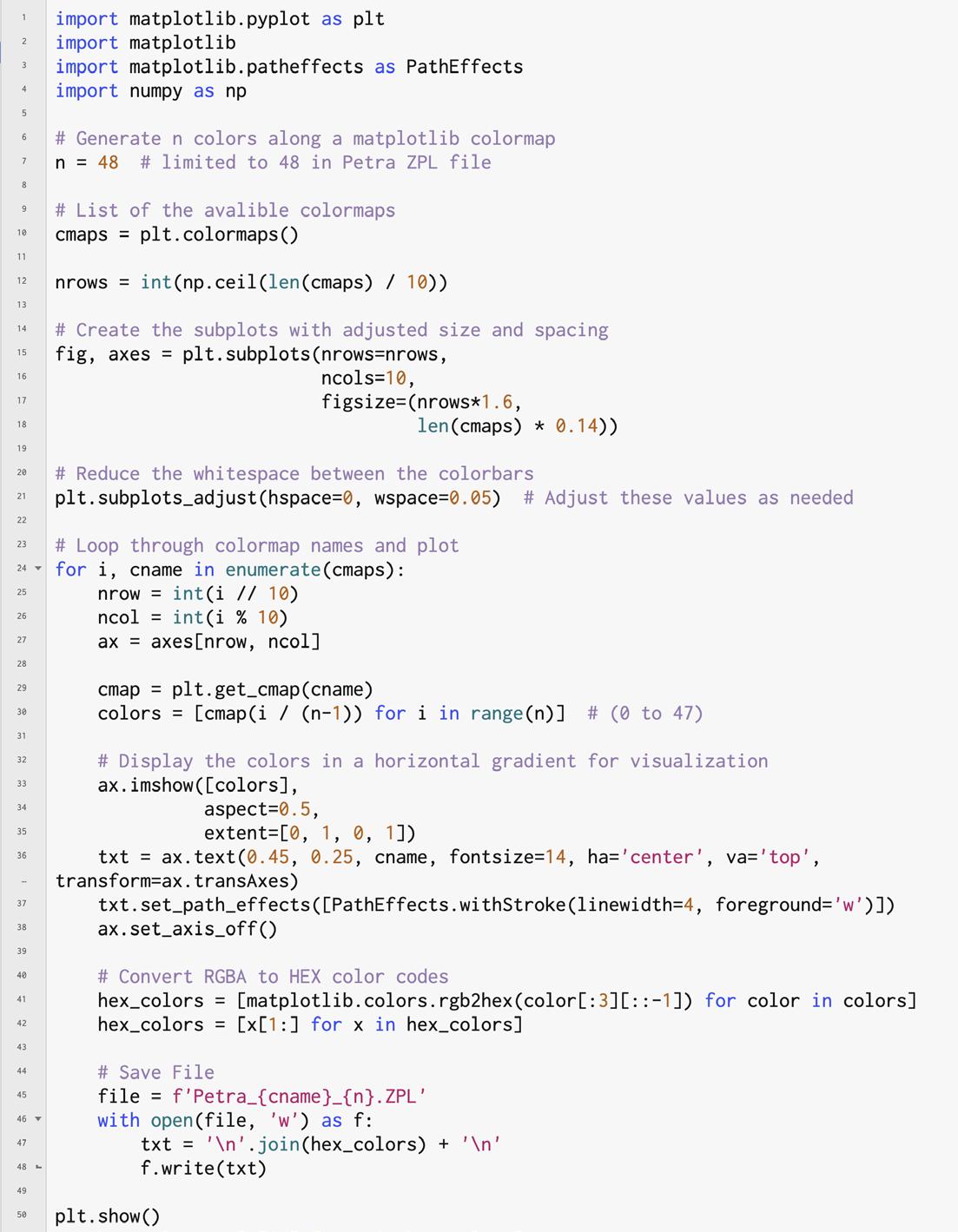
CODE FILE
The code shown here has been stored online for easy access. We have provided both a python file, and a plain-text document. These files can be accessed here.
OUTSTANDING IN THE FIELD SINCE 1894
A Brief History of Brunton, with an Update Regarding its New Ownership and a Paragraph about the Importance of Analog Measurements
BY LAUREN HEERSCHAP Brunton International LLC | lauren.heerschap@brunton.com
WHEN YOU FIND YOURSELF
at an outcrop measuring strike and dip with your Brunton compass, how often do you think about the background of that trusty tool? It turns out that this humble instrument has a long, complicated story that mirrors the history of geology in the Western U.S. and reflects global events over the past 130 years. Brunton is arguably the oldest brand in geology and one of the discipline’s most recognizable names. It has the distinction of being the world’s oldest compass brand and holds some of the longest-standing military contracts with the U.S. government of any company. In the realms of field geology, military, and outdoor recreation, the Brunton compass remains the steadfast tool around the world for navigation and field measurements not dependent on batteries, GPS signals, digital devices, or terrain conditions. The simple function of a magnetic needle pointing north leads
to the enduring legacy of the Brunton compass, one that has no end in sight.
But let’s start at the beginning. In the late 1800’s, America’s westward expansion had grown into a fullscale industrial boom fed by the rich natural resources of the West. Exploration for minerals and metals was in full swing; it was the golden age of most western mining efforts, when what are now ghost towns were bustling with all manner of industry and population diversity. Exploration efforts were reliant on bulky surveying equipment; it often took a mule or two to carry the transits, tripods, clinometers, alidades, and plane tables of the time.
It was in this setting that David William Brunton took the stage. D.W. Brunton was a Canadian-born mining engineer working in Aspen, Colorado, and he recognized that most exploratory geology didn’t require such high-precision or burdensome equipment. Exploration efforts were also slowed down by the time-consuming setup process of all that equipment
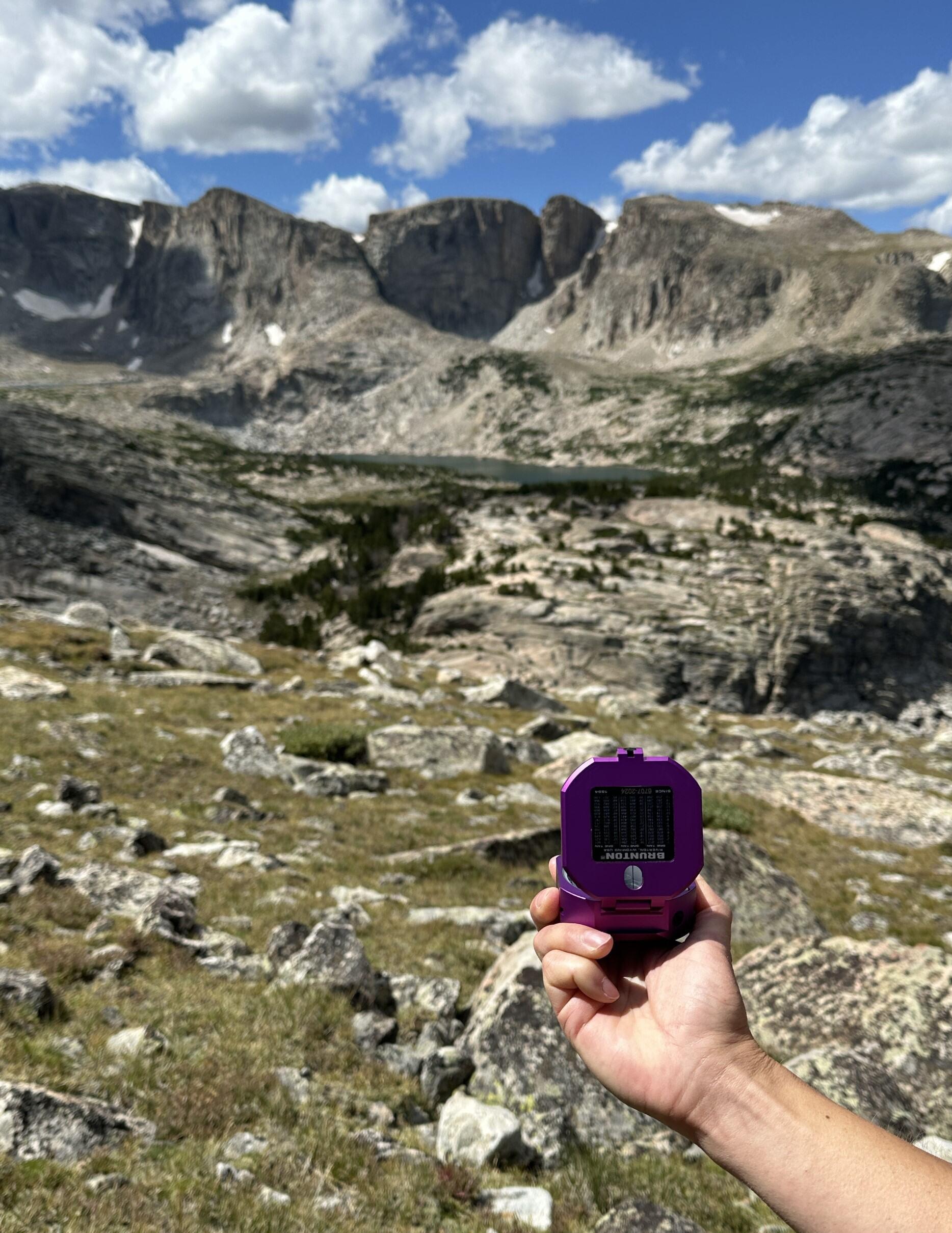
2025 Paleo Purple Standard Transit.
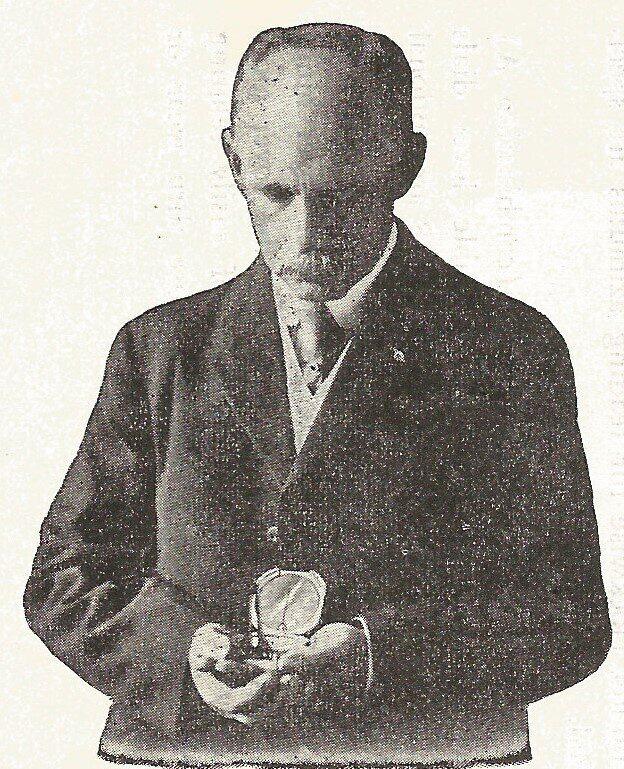
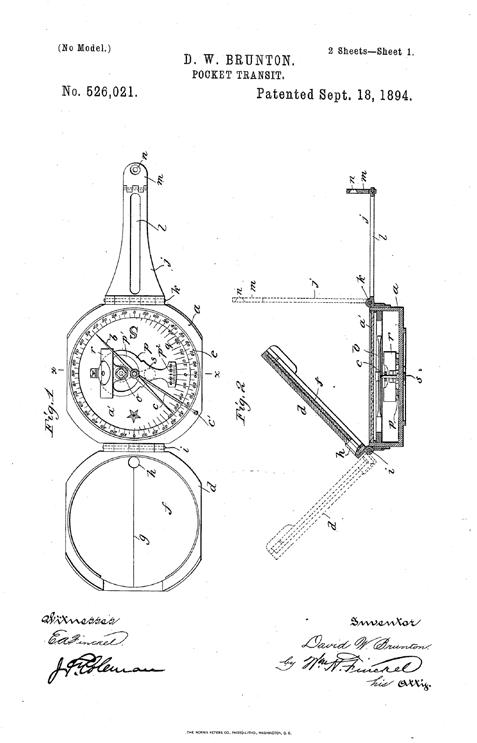
at each measurement-worthy locale. So, on September 18, 1894, D.W. Brunton was awarded his first patent for a pocket transit, cleverly named as a compact, portable version of the transit that could theoretically fit in one’s vest pocket. His first design featured a direct sighting magnetic compass, a mirror, a sighting arm, and a clinometer with a level, all housed in a rugged aluminum body. The pocket transit could measure precise horizontal bearings and vertical angles; essentially what sets it apart from a simple compass. Before this invention, geological measurements such as strike and dip required two separate instruments: a compass and a clinometer. Now those capabilities were combined into one compact instrument. The design, shape, components, and function of D.W. Brunton’s first model are still foundational to the classic Brunton pocket transit today.
D.W. Brunton licensed what became a growing list of patents to the Ainsworth Gold Scale Company in
Denver, CO, where Brunton pocket transits were manufactured from 1894 until 1972. D.W. Brunton incrementally added improvements and experimented with design elements through a series of at least six additional patents. These innovations improved upon the leveling, sighting, and tripod-mounting capabilities of the original design. During World War I, D.W. Brunton adapted the pocket transit to military use, experimenting with illumination and additional adaptations. By the time of his passing in 1927, about 30,000 pocket transits had sold worldwide, and the U.S. military had begun ordering batches of what is now called the M2 transit for navigation and artillery aiming. The design had quickly become the preferred tool for geologists and any other professional who needed precision packed in a portable device.
By the 1960’s, the manufacturer Ainsworth & Sons was in decline, with Brunton transits in jeopardy of disappearing with it. Thankfully in 1972 a group of
ABOVE: D.W. Brunton.
RIGHT: 1894 Original Patent Drawing for pocket transit.
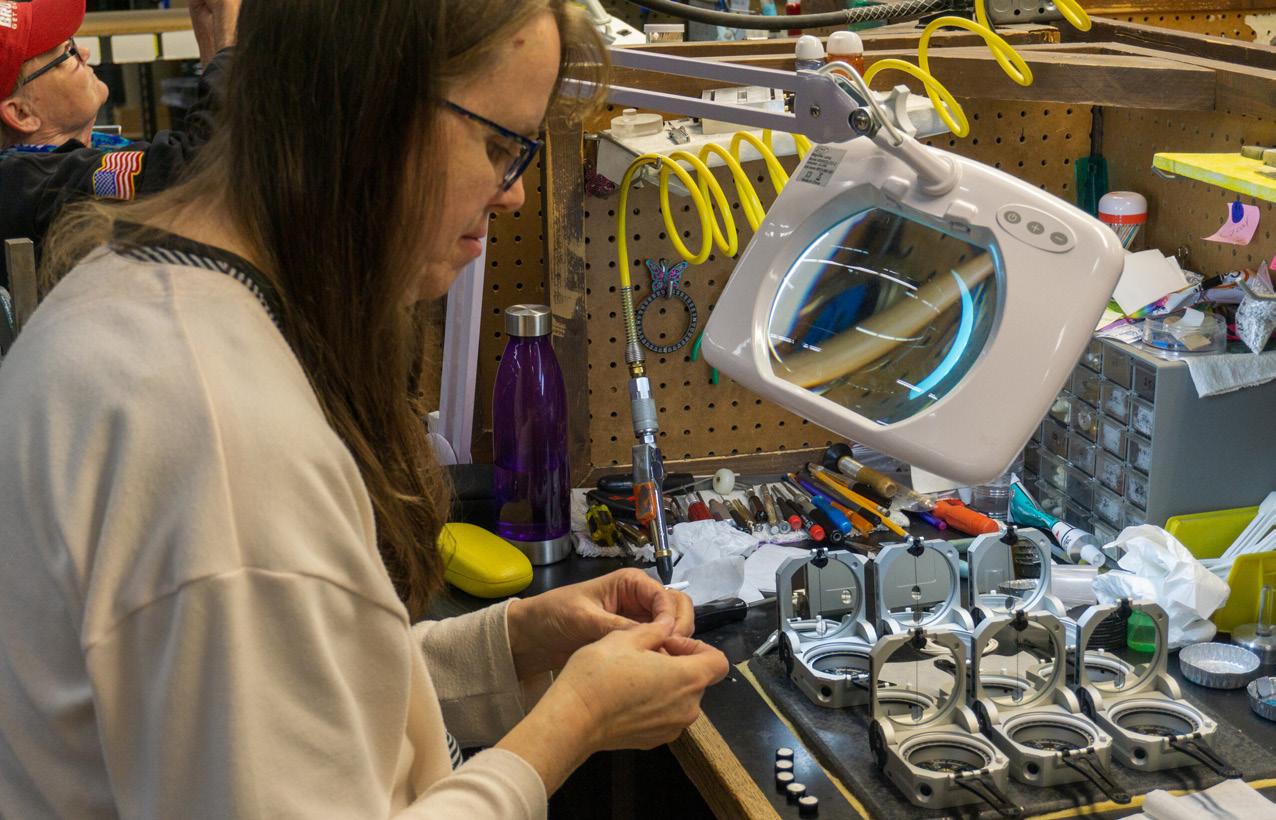
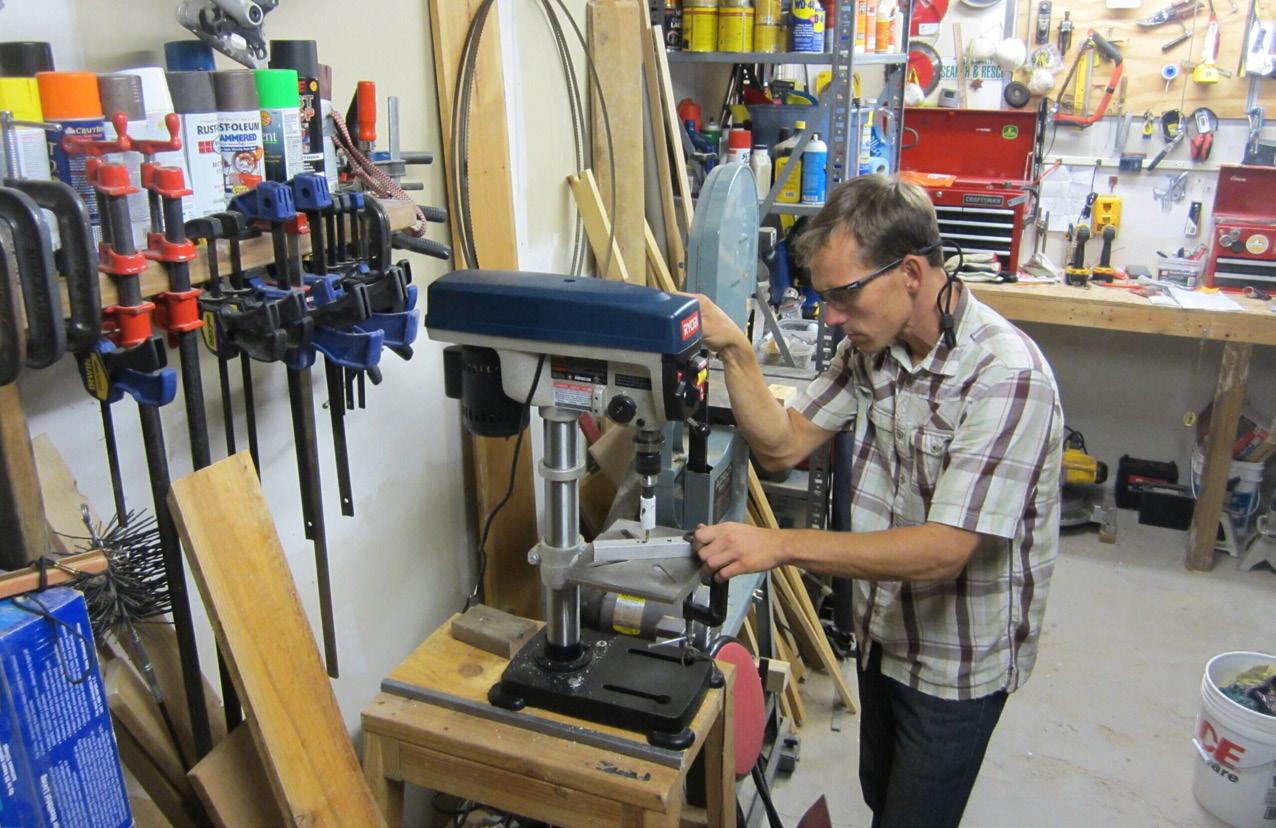
ABOVE: Geo Transit Production. BELOW: Dave's Garage Shop in Winter of 2014.
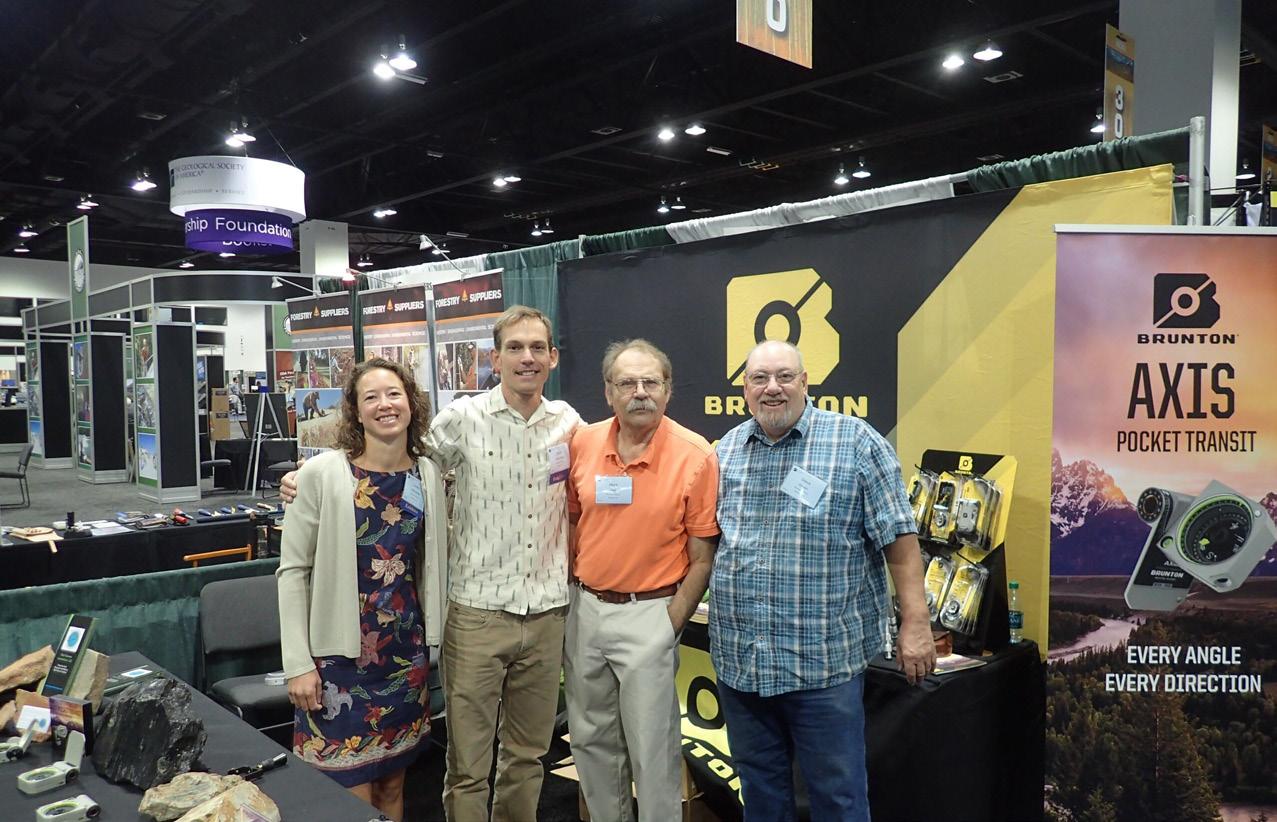
ABOVE: Axis Transit Introduction at 2016 Denver GSA. BELOW: Brunton Building.
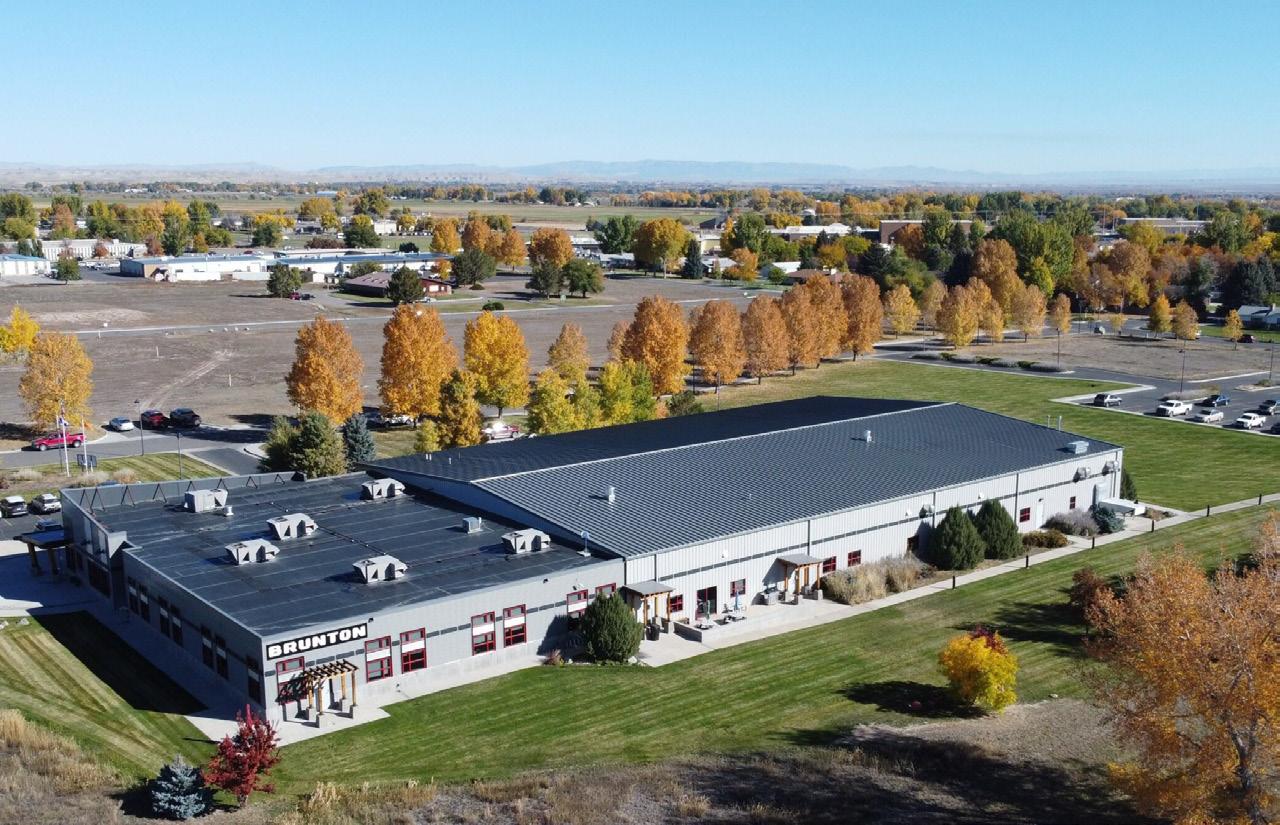
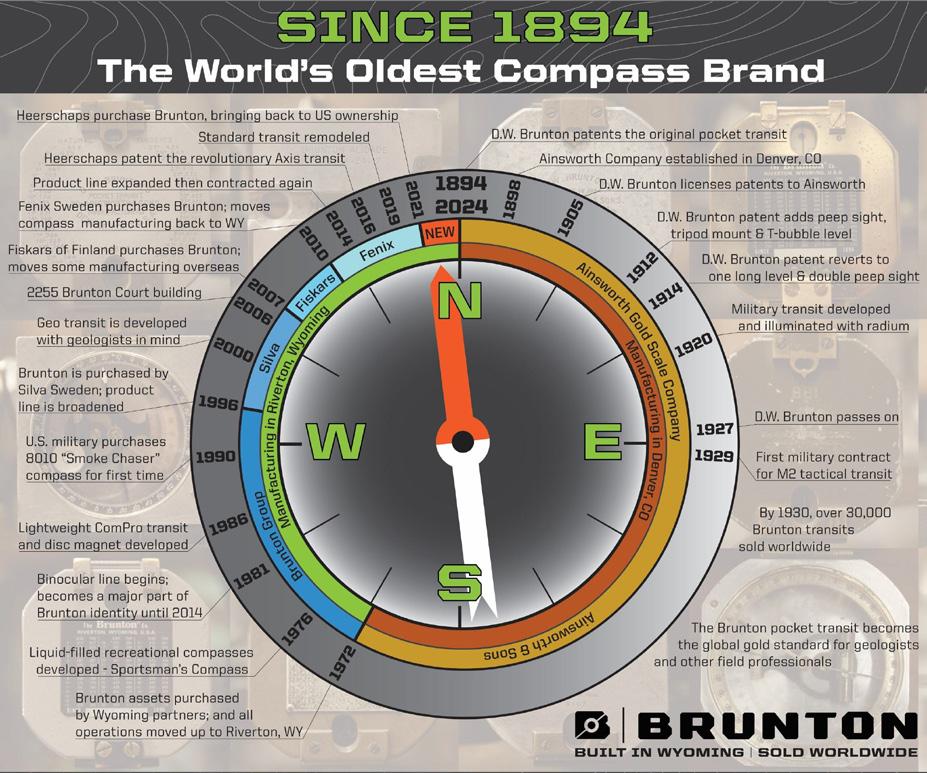
Wyoming businessmen backed by oil and gas funding purchased the Brunton brand, inventory, and assets, and moved it all (including machine shop machinery still in use today) up to Riverton, Wyoming. Riverton was, at the time, a major hub for the oil and gas industry, and the move was bolstered by economic development agencies eager to bring more manufacturing to the town.
Out in the middle of Wyoming, the Brunton company thrived and expanded. It took on new product lines, adding liquid-filled “recreational” compasses in 1976, binoculars and hunting optics in the early 1980’s, and additional improvements to the pocket transit line such as magnetic declination adjustments and international needles. The lightweight ComPro Transit, with its plastic composite body, was developed
in the mid 1980’s. Through targeted marketing efforts, the Brunton brand took on a Wyoming identity, gradually becoming known mainly as a hunting optics and sportsman’s brand in the region, all while steadily and quietly supplying the world’s geologists with their pocket transits and the U.S. military with the M2.
Then in 1996, after 102 years as an American brand and company, Brunton was sold to Silva, a Swedish manufacturer of nautical compasses and gear. This brought on additional product development and blending of various models of liquid-filled compasses between the two brands. In addition to compasses, Silva attached the Brunton brand to headlamps, early GPS, camp stoves, and other high-end camping accessories. The Geo Transit was developed around the turn of the millennium and was the first significant
History of the Brunton Compass.
change in the pocket transit design since D.W. Brunton’s original inventions. With its large hinge dials, the Geo added the capability to measure dip azimuth and dip angle simultaneously as well as certain lineation configurations more easily than the conventional models.
In 2007, Fiskars of Finland purchased Brunton from Silva. Monoculars were added to the optics line, and Fiskars brought an assortment of knives and other hunting accoutrement into the general brand line. Fiskars moved a lot of the recreational compass manufacturing overseas to China, but pocket transit manufacturing remained steadfastly quiet in Riverton, thanks to another community-wide effort to keep the company in town –this time by funding a new Brunton building.
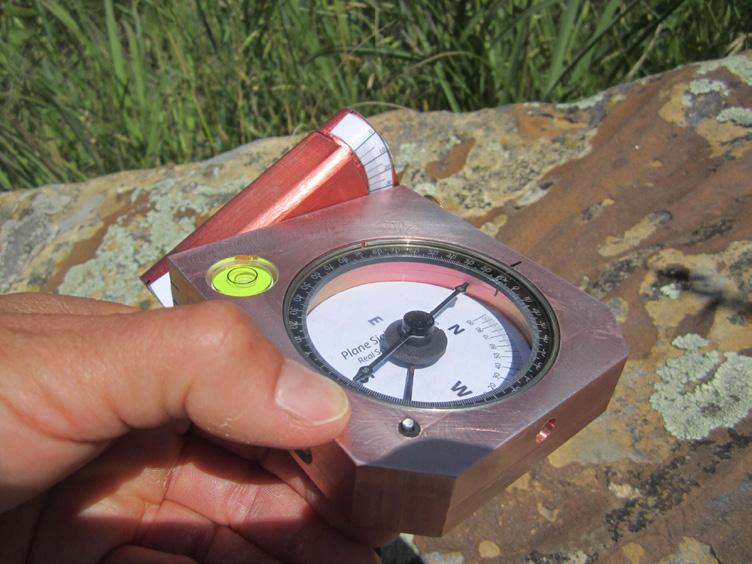
In 2010, yet another Scandinavian company took the reins with the Fenix Sweden takeover. Fenix moved all compass manufacturing back to Riverton but pushed all management to its North American headquarters near Boulder, Colorado. This caused a split in the product line, with the optics managers staying back in their beloved Wyoming and forming binocular companies of their own, including Lander’s Maven Optics and Riverton’s Lucid Optics. Compasses and transits were being manufactured in the new building, but the front office space started collecting dust soon after the paint had dried. With the move of management and the cutting of the optics line, a lot of the local Wyoming community assumed that Brunton had gone under. Fenix rapidly expanded into some additional imported camping accessories such as portable power and heat sources, possibly to try to fill the void left behind by binoculars, but then consolidated the Brunton line back to mainly compasses around 2016.
It was in this context that my husband, Dave, and I introduced a revolutionary new model of pocket transit and its associated measurement methods. I am a
structural geologist who was at that time working as a lecturer at Fort Lewis College in Durango, Colorado. Dave was teaching high school science, mainly physics and engineering, at Animas High School – a project-based charter school in Durango. In 2013 while teaching my sixth summer of field methods, an idea popped into my head as I tried to get students to visualize the planes and lines foundational to geological measurements. Almost in a single field session, an idea developed in my mind into a tool that could simultaneously measure strike and dip while illustrating the fundamentals of the measurement itself. It could do the same for trend and plunge of a lineation, and with a lid that moves 360 degrees around two axes, it would solve the problems of tricky overhanging and also low-dip measurements.
A busy teaching semester went by for both of us, with the idea not leaving me alone until we resurfaced during the winter holiday break. Dave purchased a mill and taught himself how to machine metal, and he used his self-taught 3D modelling skills to make three functional prototypes over the course of that winter. At one point, we ran out of aluminum stock, so Dave made a forge and melted down old climbing
2014 Plane Sight Lineation.
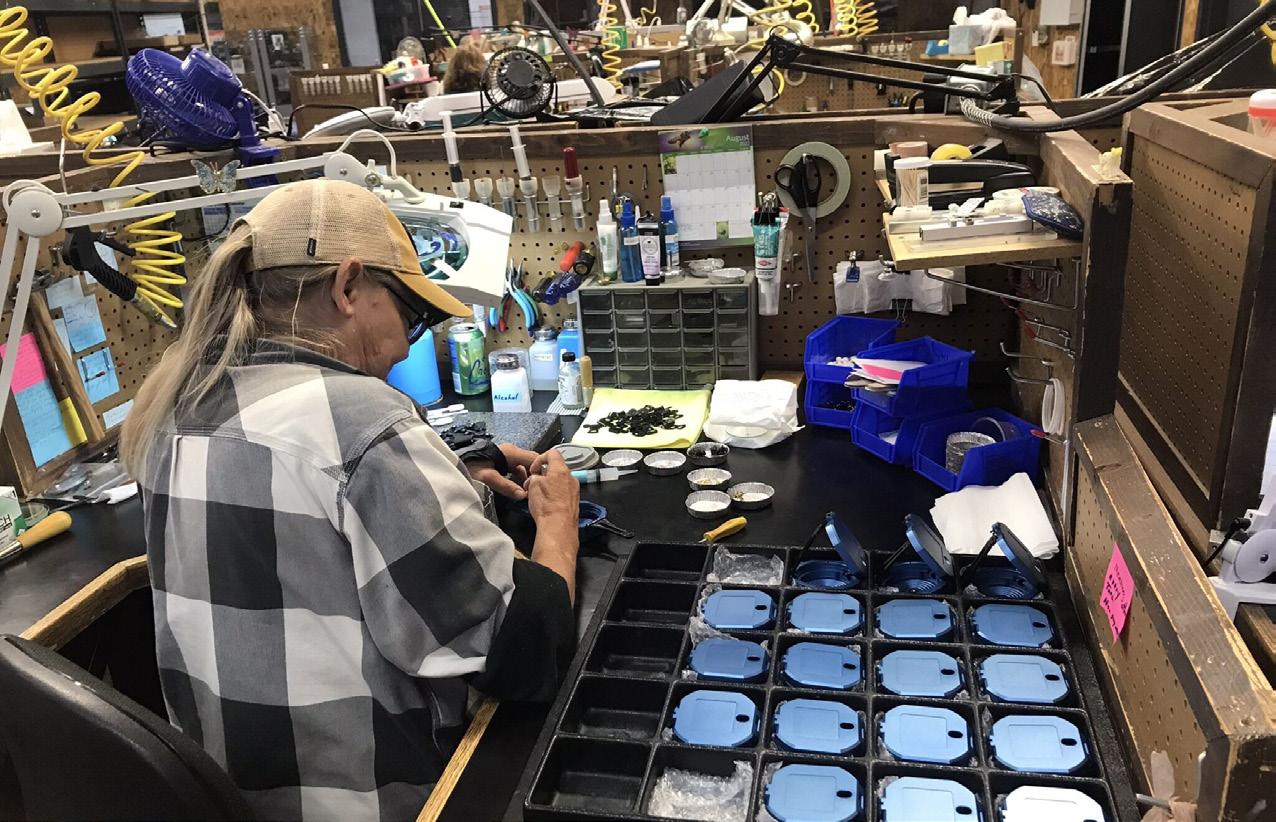
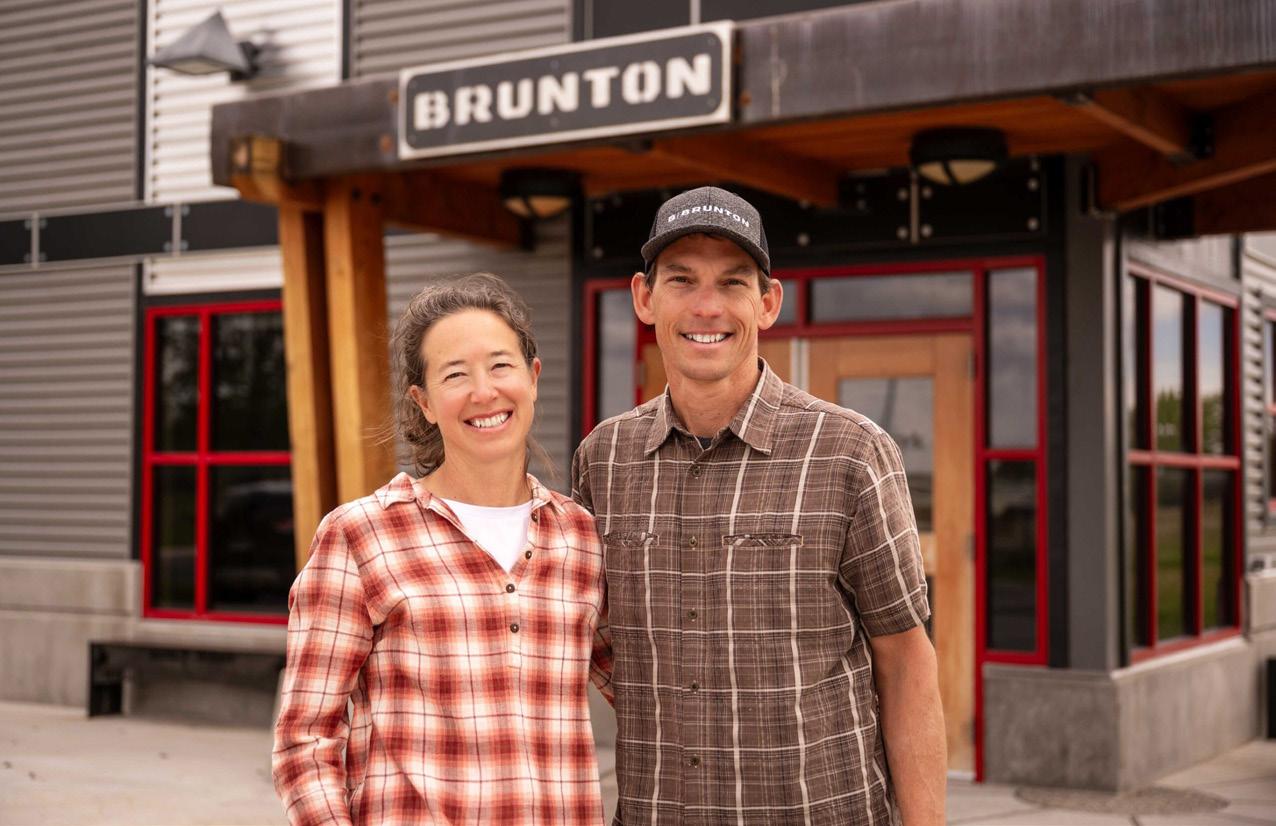
ABOVE: Blue Standard Transit Production. BELOW: Lauren and Dave Heerschap.
9_Orange Axis Transit
carabiners that we had plenty of as avid climbers. He also anodized the prototypes so the aluminum wouldn’t blacken users’ hands – so in addition to the forge, we had electrified acid baths hanging out in the garage. It was an interesting winter at the Heerschap household, to say the least!
The prototypes did exactly what I had envisioned, so we started protecting the idea through various patent applications that spring. During the summer of 2014, I took the prototypes of what we were then calling the Plane Sight Compass, to various field camps I was instructing, and the model was a hit with students and faculty alike. It was especially popular with students who were having difficulty grasping measurement methods with conventional Bruntons, and the variety of users and settings helped me discover even more benefits of the model such as easily and intuitively measuring fold geometry, conjugate fractures, and sighting strike and dip from a distance.
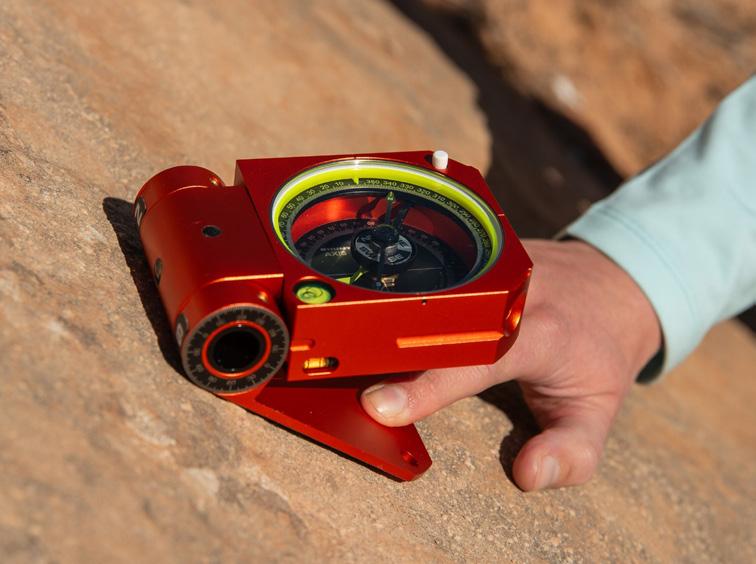
Brunton engineer for almost 40 years at that point, responsible for many of the incremental improvements to the pocket transit since D.W. Brunton himself. The collaboration with Brunton prompted us to uproot from Colorado and move to Lander, WY – a short drive from Brunton’s headquarters in Riverton.
We knew we had a good idea that we had to run with, but we also knew we couldn’t manufacture the Plane Sight Compass in our garage forever. We decided to reach out to Brunton to see if the company would be interested in the idea. In the Fall of 2014, we journeyed to Boulder and spent a full day pitching the idea to Brunton management. It would have been a shorter meeting, but none of the staff at the time knew how the conventional pocket transits took geological measurements, so we had to first show them those methods before we could expound upon the benefits of our invention.
What resulted was the licensing of the patent to Brunton to manufacture an official version of the invention. It was mutually decided to shorten the name to the Axis Transit, and we worked alongside Brunton’s engineer at the time, Hank Iden, to create a Brunton version of the prototype. Iden had been the
The Axis Transit officially launched in 2016, and the first public introduction of it was at GSA in Denver that fall. The Brunton booth was surrounded by threedeep crowds almost the entire meeting, with all ages and levels of geologists extremely psyched on the first significant innovation to the pocket transit since 1894. I remember daily visits from many of my geology heroes, including Eldridge Moores who even bowed to me over my invention. He also brought his 50+ yearold Brunton to the booth for some repairs… We continued to assist Brunton with the launch of the Axis and with broader support of all geological products at various events during 2016 and 2017. As Hank Iden’s health declined, Dave was officially hired as Brunton’s Production Engineer in 2018, right around the time that our daughter was born. I continued to volunteer with Brunton on various professional product support projects, and the whole family went
Orange Axis Transit.
to Sweden in 2019 for a Fenix sales meeting. We got to know the Brunton and Fenix management and leadership, and by late 2020, I was eager to get more deeply involved with Brunton. I started pushing the CEO to hire me as a much-needed Sales Manager for the geological line; little did I know that more was brewing behind the scenes.
In January 2021 in the midst of work-from-home Covid rules, I overheard a meeting between Dave and the Brunton CEO where the question was asked, “What would you and Lauren think of buying Brunton?” That started a year-long effort to negotiate a favorable deal across the Atlantic and to figure out financials that would enable two former educators to purchase a major brand. I did get hired on as the Geology Sales Manager, but we both had to keep the purchase deal a secret from almost all of the staff, including our direct supervisors. We felt the pressure building, too, realizing that the future of the entire Brunton brand depended upon the deal working out. Fenix had some other offers, and with other buyers there was a very real risk that Bruntons would soon be made in China or that the brand would get uprooted from its legacy products and turned into something else entirely.
In November 2021, the deal finally did work out, and we brought Brunton ownership and leadership back home to Wyoming after 25 years in Scandinavian hands. We regrew the management team in Riverton, and all of the existing production and warehouse staff stayed on with us, some of whom had been with Brunton for over 30 years. We quickly expanded from 18 employees to almost 30 people and helped bring back the family feel to the company. During the first three years, we have invested in several long-standing
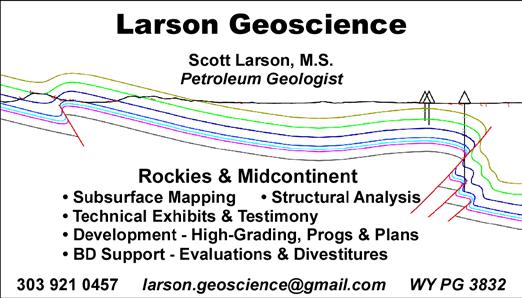
projects such as illuminating the M2 and ComPro (named the Miner’s D-Light after a nearby ghost town), creating our own version of the military lensatic compass, and adding high-visibility colors so fewer geologists will leave their pocket transit hiding on an outcrop. And there are plenty of innovations still in the funnel.
We are enjoying the challenges that come with business ownership and are confident in the enduring nature of the magnetic compass. We know that digital devices are capable of taking and mapping geological measurements, but they vary widely in accuracy and should be calibrated with an analog compass daily. Pedagogically, geology students are receiving a great disservice if they are not taught traditional measurement methods. The spatial awareness that comes with using an analog compass, the visualization of the planes and lines involved in the measurement, and the inherent slowing down and field checking involved in hand mapping a symbol on the spot are all lost if only digital measurements and mapping are taught. We’re confident that geoscience education will stay on course and continue to equip students with the foundational skills that field work provides – we’ll continue to provide the most iconic tool.
So, if you ever find yourself in Riverton, WY, stop by the Brunton headquarters. It’s a beautiful building next to the campus of Central Wyoming College. We enjoy giving tours, especially pre-arranged ones. You’ll get to see all sorts of compasses and pocket transits being carefully hand-made by a dedicated team. And the next time you’re at an outcrop measuring strike and dip, you’ll have a deeper appreciation for the humble yet historied geological compass in your hands.
HYBRID LUNCH TALK
Speaker: Jonathan McKenna
Date: March 5, 2025 | 12:00 pm - 1:00 pm
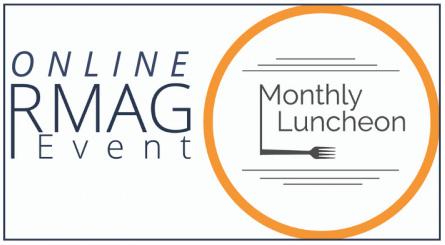
Optimizing Return on Investment and Estimated Ultimate Recovery through Advanced MicroSeismic Monitoring Techniques
Presenter: Jonathan McKenna, MicroSeismic
OBJECTIVE
Case studies are presented to evaluate Return On Investment (ROI) of fracture monitoring of hydraulic stimulation using geophysical methods. Time-dependent stress shadow effects cause rotation of principal stress and
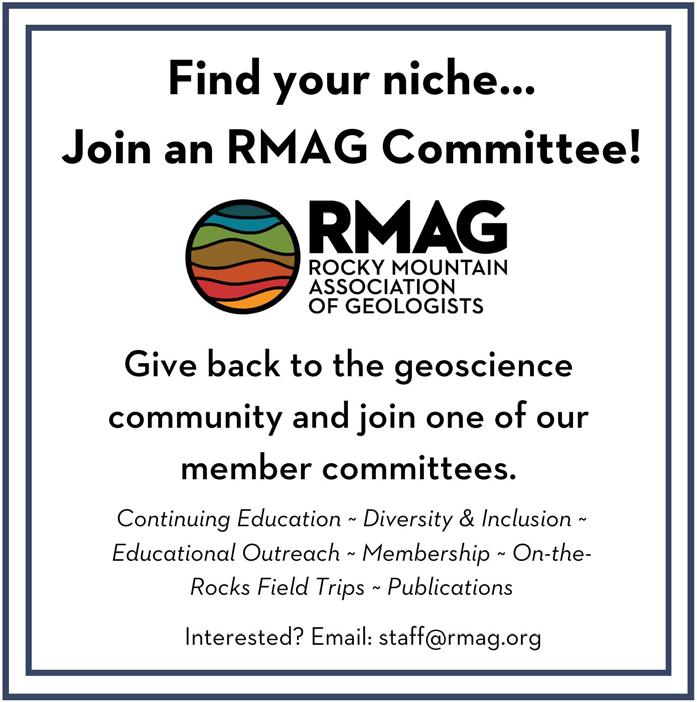
reduction of stress anisotropy. Over time, stress returns to the virgin reservoir stress. Focal mechanisms are analyzed to evaluate the dynamically changing stress field and to identify fracture sets and faults reactivated in real time. Operators can take advantage of these time-dependent effects by properly repressurizing parent wells prior to treating child wells, avoiding wellbore-casing deformation by identifying and skipping stages that intersect fault planes, and modifying well spacing to maximize the number of wells per drilling spacing unit (DSU).
METHODS
Case histories presented include refracturing parent wells followed by offset child wells, wells treated in vicinity of active faulting, and situations where number of wells drilled per DSU are improved. ROI is calculated with consideration of monitoring costs and reported as improved revenue per well compared to local production type curves over the life of the well and calculated based on protected asset value per well when geohazards were detected and avoided.
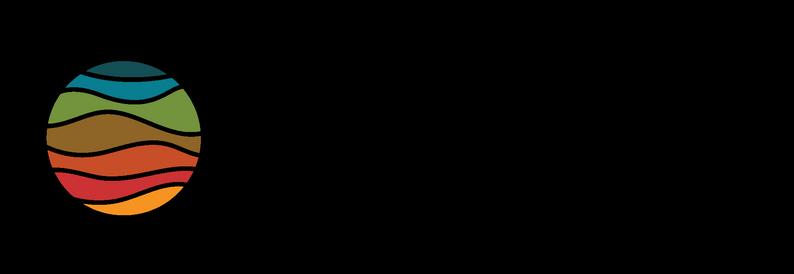
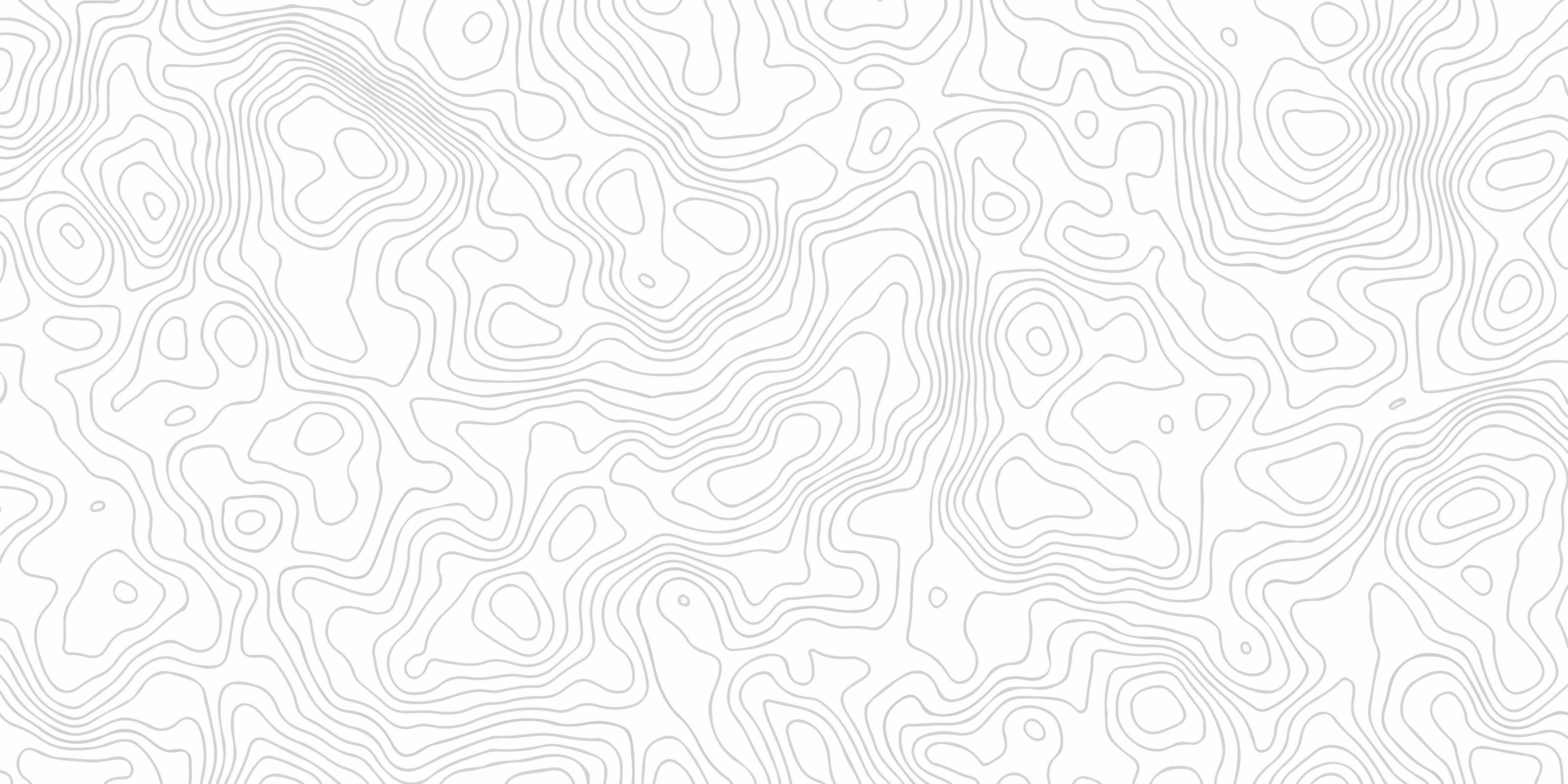
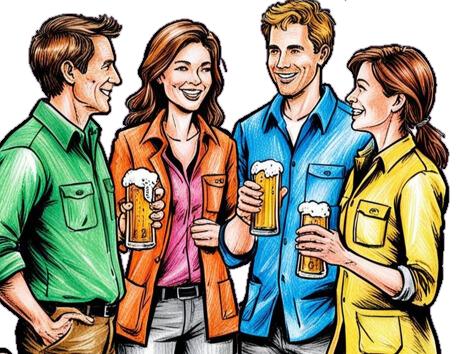
RESULTS AND CONCLUSIONS
A significant ROI can be obtained by proper evaluation of microseismic focal mechanisms. For successful refracturing parent wells and creating containment of immediate child well treatments resulting in increased production on parent well by 35% and eliminates production loss on child wells by 25% allowing for 100% ROI after producing for 1 month. Furthermore, skipping stages identified as being susceptible to wellbore casing deformation saves as much as $2M/well and monitoring costs are recouped by saving one well. An increase of one well per DSU increases production by nearly 30% which yields a 6-month ROI.
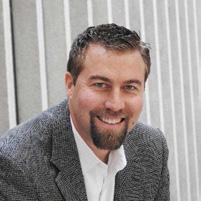
SIGNIFICANCE
Operators can take advantage of time-dependent stress shadow effects that can be quantified using advanced analytical techniques of microseismic focal mechanisms. Furthermore, geohazard avoidance is possible through real-time microseismic monitoring. In addition, maximizing number of wells per DSU increases production and allows operators to drill and complete their acreage correctly the first time through without the need for refracturing old parent wells and drilling child wells. These efficiencies lead to significant production gains and savings which are much more significant than microseismic monitoring costs.
JON McKENNA is a geological engineer at MicroSeismic, Inc. His work relies on microseismic measurements during hydraulic stimulation to quantify dynamic stress changes in the reservoir and develops accurate fracture models to simulate proppant placement and forecast production.
He holds a B.S. and a M.S. from the University of Georgia in Geology and Geophysics and a Ph.D. from the Colorado School of Mines in Geological Engineering. He has over 20 years of engineering geology experience, has published over 50 journal articles or abstracts, and is the
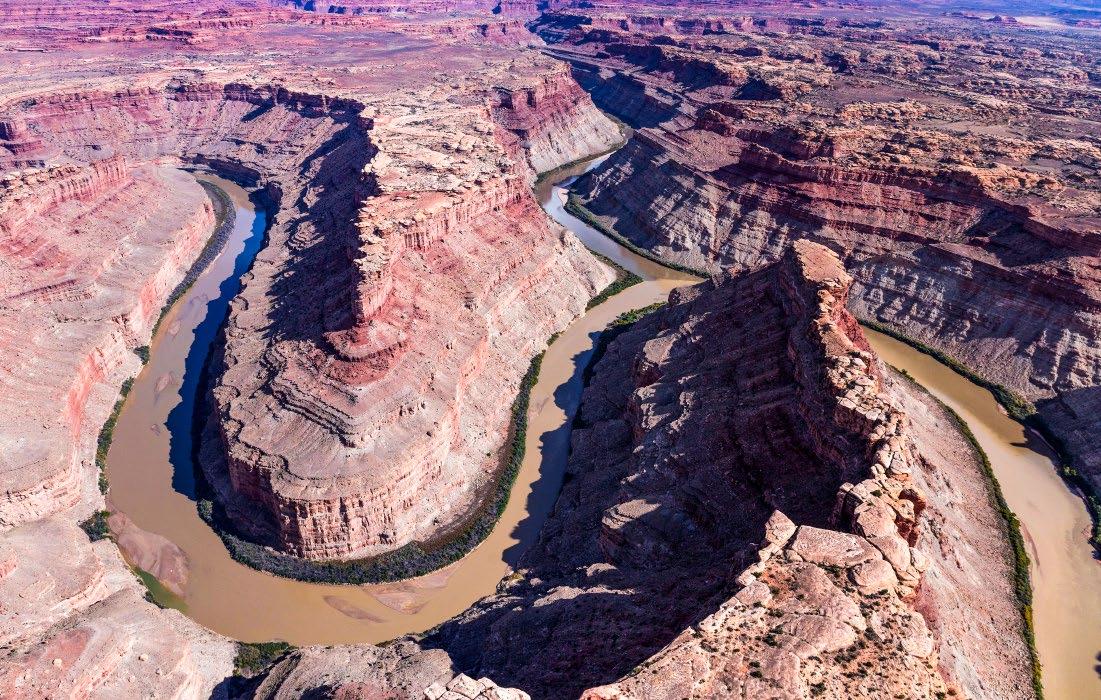
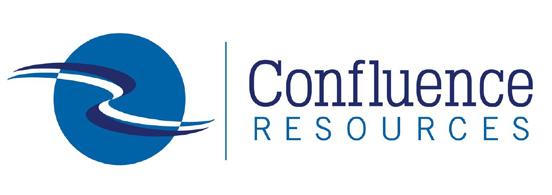
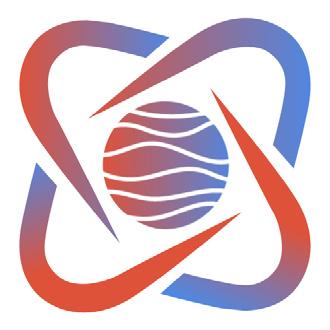
HELIUM & HYDROGEN
Denver, Colorado ~ April 9-10, 2025
REGISTRATION
PROSPECT AND EXPO FAIR
SPONSORSHIP
OPPORTUNITIES
RMAG MEMBER - $700 NON-MEMBER - $800
INCLUDES BOOTH, REGISTRATIONS & ADVERTISEMENT EXPO BOOTH - $2000 PROSPECT BOOTH - $1000
OPTIONS INCLUDE: ADVERTISING - DIGITIGAL AND ON-SITE
SIGNAGE - COMPLIMENTARY BOOTH SPACE & REGISTRATION - HAPPY HOUR DRINK TICKETS - AND MORE CHOOSE FROM 5 LEVELS: DIAMOND ($10,000), TITANIUM ($5,000), PLATINUM ($2,500), GOLD ($1000), SILVER ($500)
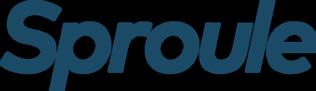
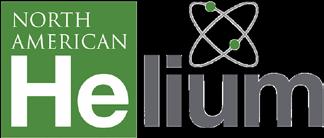
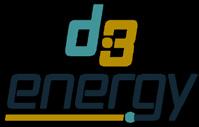
THANKS TO OUR SPONSORS REGISTER PROGRAM EXHIBIT SPONSOR
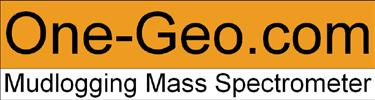
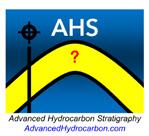
HYBRID
Speaker: Mike Kuligowski
Date: April 2, 2025 | 12:00 pm - 1:00 pm
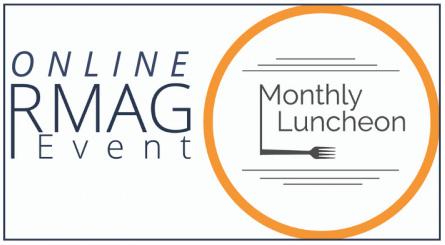
Unlocking the Full Potential of Geology Data in Drilling and Completions A Workflow Approach
Presenter: Mike Kuligowski, Core Geologic
Understanding well performance and operational challenges requires a multi-disciplinary approach that integrates geology, drilling, and completions data. Too often, geosteering and subsurface operational geological data are underutilized in post-well analysis, leaving key questions unanswered. Is a performance issue caused by the rock or by drilling and completions decisions?
Traditionally, geoscience and engineering data have existed in separate silos, with
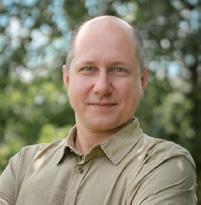
analysis performed independently. This disconnect often leads to incomplete assessments of well performance and subsurface challenges. By placing geology at the core of the workflow and utilizing a geocentric database, all engineering data can be tied back to the rock itself. This integration provides a more accurate and holistic view of well behavior, allowing for better-informed decision-making and a deeper understanding of drilling and completions challenges.
MIKE KULIGOWSKI is a Senior Technical Advisor at Core Geologic, specializing in multi-disciplinary data analytics for drilling and completions optimization. He holds a B.S. and M.S. from Missouri School of Science and Technology and an MBA from Saint Louis University.
Mike began his career in hard rock mining from 2005 to 2007 before transitioning to coal from 2008 to 2014. In 2014, he joined Pioneer Natural Resources, where he worked until 2020, gaining extensive experience in geoscience applications within oil and gas development. From 2020 to 2023, he served at Driftwood Energy as a Project Geologist. In late 2023, he joined Core Geologic, where he continues to drive innovation by leveraging geology-centered data solutions to improve well performance.
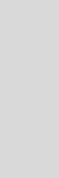
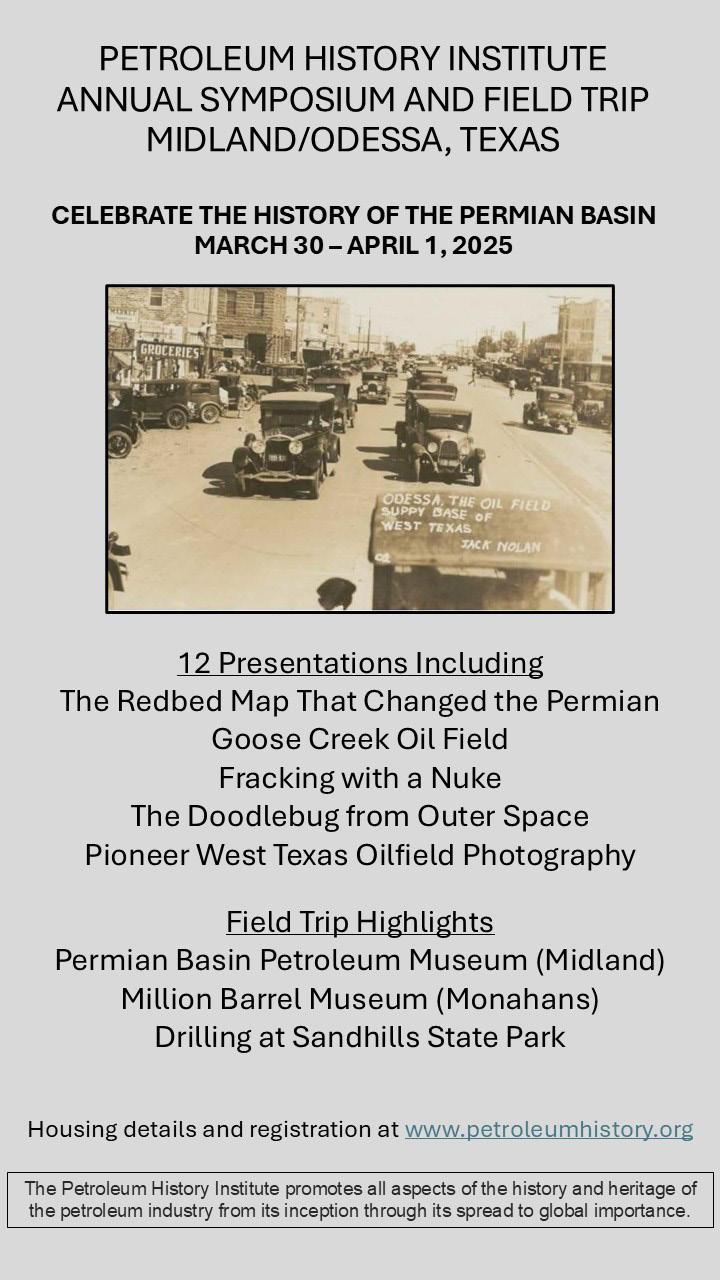
By Ronald L. Parker
Geologist,
Specialists,
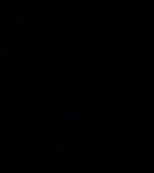
SERPENTINE
The Good, the Bad, and the Miraculous
Snapshot of a decorative ophicalcite Verde Antique wall slab in the porch of the Equitable Building at 17th and Stout in downtown Denver, Colorado. According to Murphy (2004), this material is from the South of France, where it is known as Verde Alps. The shot includes variable green colors from the serpentine, a highly-sheared internal fabric with abundant mineralized fractures hosting carbonate (calcite, dolomite, magnesite), quartz, and invisible reflective metal oxides (magnetite and chromite). This view is about 50 cm wide.
Photo by Ronald L. Parker
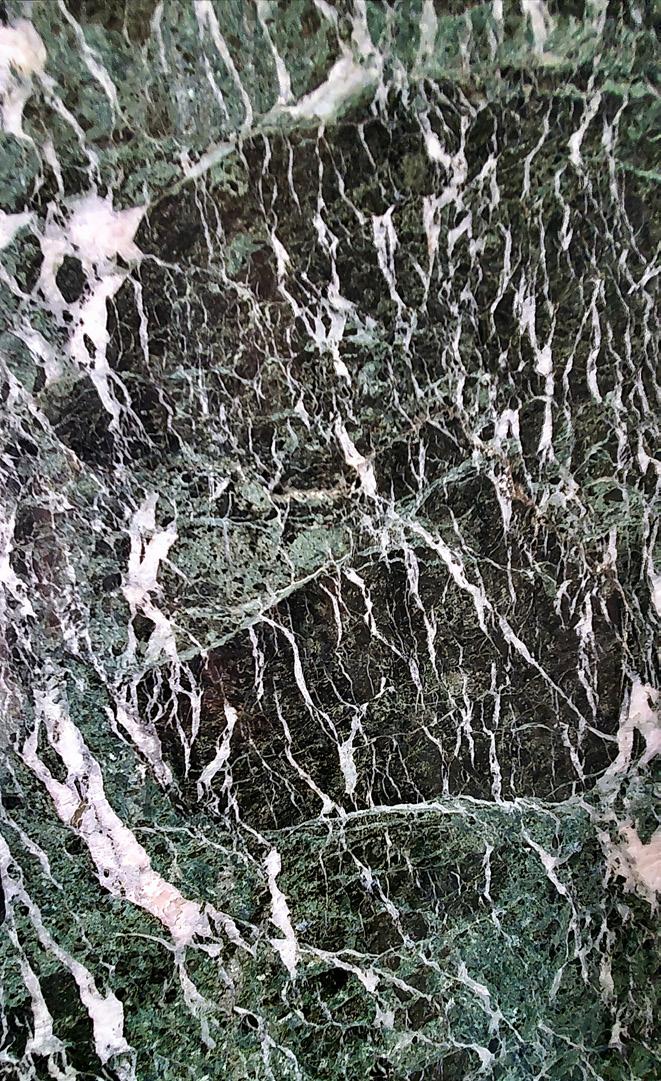
MINERAL OF THE QUARTER: SERPENTINE
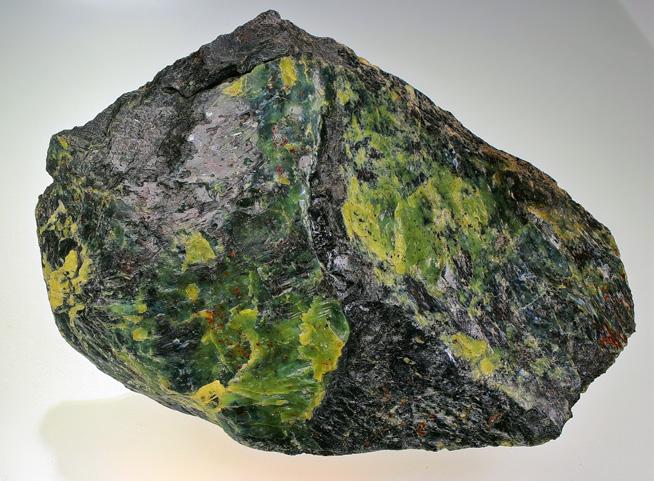
Serpentine is a fascinating mineral family that contains the ingredients of both life and death in its crystal lattices. Here, I hope to visit both territories. In my research into serpentine, I discovered that I am ill-suited to comprehend, much less adequately convey, the Jupiter-sized magnitude of scientific knowledge about serpentine. This is my attempt at skimming the surface.
Serpentine, Mg3Si2O5(OH)4, is a 1:1 trioctahedral phyllosilicate most often known by its three “pseudo”polymorphs: lizardite, antigorite, and chrysotile. Serpentine is frequently found with its 2:1 trioctahedral cousin – talc, as well as other associates: chlorite, epidote, magnesite, dolomite, calcite, magnetite, and chromite. Serpentine is formed by the hydrothermal alteration of mafic and ultramafic minerals (olivines and pyroxenes) from mantle-derived peridotites, dunites, harzburgites, and lherzolites. This alteration occurs at slow-spreading mid-ocean ridges, oceanic transform faults, and during subduction. Exposure of serpentinites is facilitated by ophiolite obduction and by disarticulation of oceanic lithosphere during collisional orogenic events. Serpentinization at mid-crustal depths during subduction creates rock weakness, intensive fracturing, and substantially lower density, facilitating ophiolite skinning and emplacement onto continental
Lizardite chunk from an unknown locality in California. 17 cm in the long dimension.
by Ronald L. Parker
crust. Serpentines in ophiolites are ubiquitous in modern and ancient accretionary margins around the globe. Humans have utilized serpentine and the associated minerals in serpentinites for 10s of thousands of years for art, decoration, household goods, and building materials. Serpentines form strange landscapes, where exposed, characterized by poor soil development and low ecosystem productivity. Modern extractive industries have utilized serpentine for architectural purposes, but primarily for asbestiform chrysotile, which infiltrated a majority of manufacturing processes around the world until extreme toxicity was comprehensively established in the 1970s. Despite this dark side, modern appreciation of serpentine has expanded to recognize its role as a source of molecular hydrogen (H2) and abiotic methane (CH4) to the hydrosphere, atmosphere, and biosphere. Serpentinization reactions provided the energy and the building materials necessary for the early evolution of life on Earth. Serpentine discovered on Mars suggests this potential on other terrestrial planets. Serpentine holds promise for its capacity to mineralize CO2 out of the atmosphere. As the mechanism for generating renewable molecular hydrogen, serpentinization is being evaluated for its potential to lead humanity into a new age of carbon-free energy. Serpentine: It’s good, it’s bad,
Photo
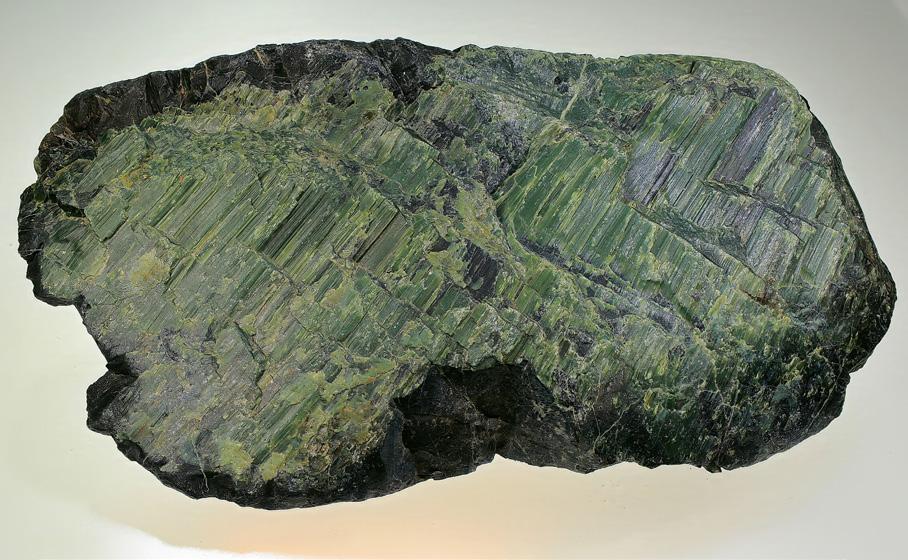
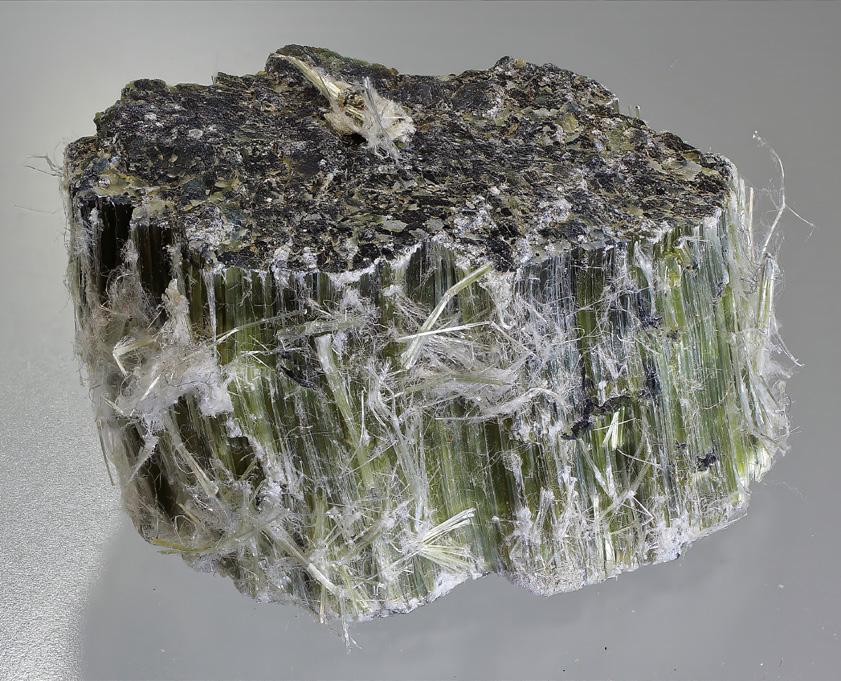
Friable chunk of cross-fiber asbestiform chrysotile from Thetford Mines, PQ, Canada. 2 cm tall.
Photo by Ronald L. Parker
Crenulated antigorite from California, unknown locality. 20 cm in the long dimension. Photo by Ronald L. Parker
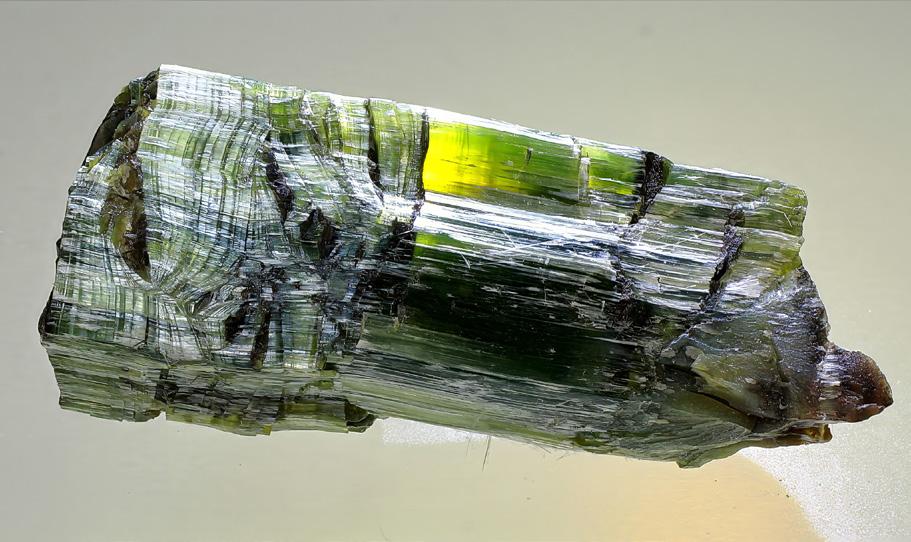
A small fragment of chrysotile displays an upper (left) section of microscale layered cross-fibers with small, dark breccia fragments on top of a more extensive, translucent crossfiber section. From Thetford Mines, PQ, Canada. 4.5 cm in long dimension. Photo by Ronald L. Parker
and it’s miraculous!
The name serpentine is an apparent reference to snakes, purportedly referencing the dappled and variegated greens familiar to them. Faust and Fahey (1962) dedicate an entire page to tracing the usage of the name serpentine. Suffice it to say that the name ‘serpentine’ has been used by humanity since antiquity.
The serpentine group includes about twenty 1:1 phyllosilicate minerals with the general formula M3T2O5(OH)4 where cations in the octahedral sheet (M) can be Mg, Fe2+, Fe3+, Al, Ni, Mn2+, Zn, Co, Cr and Ti or vacancies and the cations in the tetrahedral sheet (T) are Si, Al or Fe3+ (Faust and Fahey, 1962; Rakovan, 2011). More commonly, we consider serpentine to refer to the three polymorphs of Mg3Si2O5(OH)4, lizardite, antigorite, and chrysotile, and we will focus on these three here. These 3 “polymorphs” differ in the degree of layer curvature, with other slight differences in actual composition and other mineral properties (which renders them not true polymorphs, per VanBaalen et al., 2009). Lizardite, named for the Lizard Point Peninsula in Cornwall, England, is a
massive, microcrystalline, or platy variety with the least-layer curvature (Bonewitz, 2008). Lizardite is the most common type of serpentine. Antigorite is a hard, compact variety that displays systematic bands of alternation in the direction the tetrahedra point in the T layer relative to the a-axis. This gives the mineral a wavy or undulating character (Rakovan, 2011). Chrysotile is a fibrous variety, where the 1:1 sheets curl into cylinders with the tetrahedral sheets on the inside. The fibers are elongated parallel to the a-crystallographic axis (Rakovan, 2011). Chrysotile is readily identified by its splintery fracture and a silky, sometimes chatoyant, luster. This fibrous habit is often asbestiform, and chrysotile is the most common of the asbestos minerals, the others all being amphiboles (Johnsen, 2002). The average serpentine has a specific gravity of ~2.51 to 2.63, a value that is notably less than that of average continental crust (2.65) and far less than serpentine parent peridotites (3.30). Serpentine hardness ranges between 3 and 5 (Klein, 2002).
Serpentines commonly occur with associate minerals, including chlorite, brucite, Fe-Ni-Cr
Jon Kim, Geologist with the Vermont Geological Survey, points to meter-scale elongate “slip-fiber” chrysotile from the Belvidere quarry near Lowell, Vermont. Slip fibers elongate parallel to fractures. Historically, slip fiber chrysotile was destined for woven asbestos products like blankets, drapes, and clothing. Photo used with permission from Jonathan Kim.
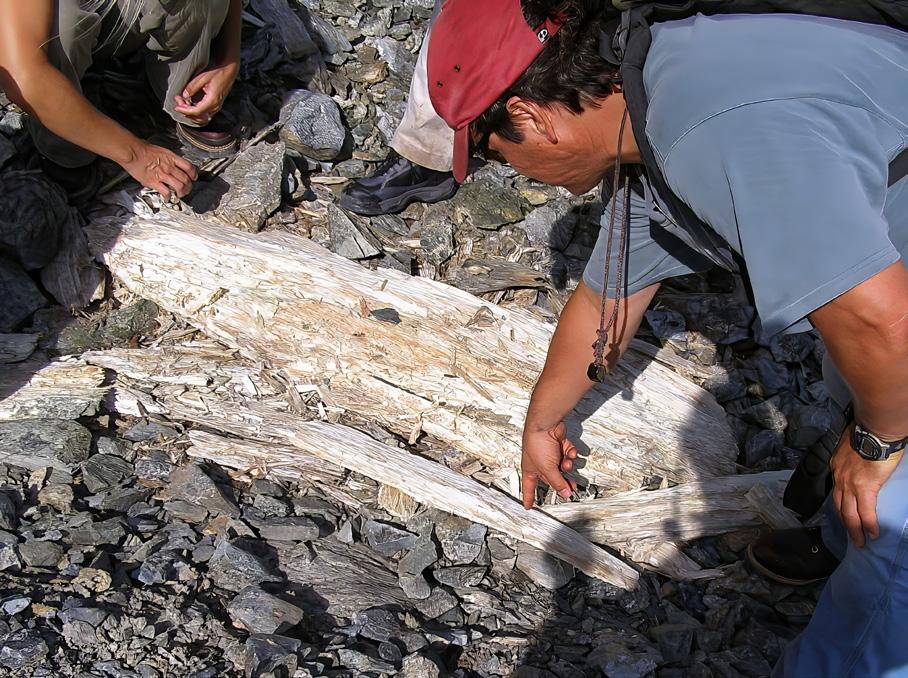
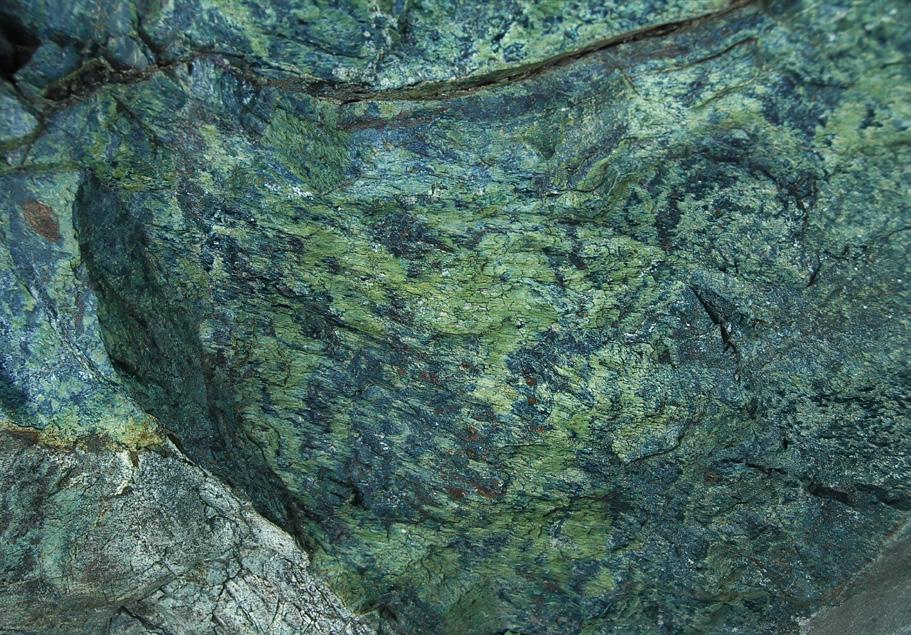
A sample of serpentinite from the late Neoarchean (2.5 to 2.7 Ga) Deer Lake Peridotite is part of the Ishpeming Greenstone Belt in the Upper Peninsula of Michigan. This outcrop looks to be the antigorite variety of serpentine. Note the mottled green coloration that lends serpentine its name. CC BY: James St. John
oxides (magnetite, chromite), magnesite, talc, dolomite, and calcite, along with precursor minerals olivines and pyroxenes. When ultramafic rocks undergo hydrothermal metasomatism, the result is a rock with a mixture of these minerals, yet primarily serpentine. This rock is called serpentinite.
Serpentinites form by the metasomatic alteration of olivines and pyroxenes in mantle peridotites at moderate temperatures (150° to 500°C). Oceanic crust hydration occurs at slow-spreading mid-ocean ridges, oceanic transforms, and in the outer rise region of subduction zones (Vogt and Gerya, 2014). Slow-spreading ridges are loci of serpentinization because they are too cool to generate significant basalt (Sleep et al., 2004), and tectonic erosion exposes mantle material to seawater (Schwarzenbach, 2016). At the outer rise region of a subducting slab of lithosphere, deep faulting at the bend promotes hydration of the dry upper mantle and lower crust to 15 km depth (Rupke et al., 2004).
Even partial serpentinization changes the physical and chemical behavior of the altered material. Plumper et al. (2012) stated, “Mantle peridotite serpentinization is arguably the most important metamorphic hydration reaction.” They elaborate that serpentinization has “1st order effects” on mantle weakening; it alters seismic velocities and changes material delivery to subduction zones. Serpentine hydration involves a substantial volume increase (25%-50%), leading to significant changes in topography and focused microseismic activity from continuous expansion cracking (Schwarzenbach, 2016). Volume increase also substantially lowers bulk density (from 3.3 g/cc to ~2.5 g/cc). A weaker serpentinized crust is more easily deformed and faulted. The hydration reaction during serpentinization is highly exothermic, releasing up to 40 kilojoules/mole of water (Sleep et al., 2004). This, in
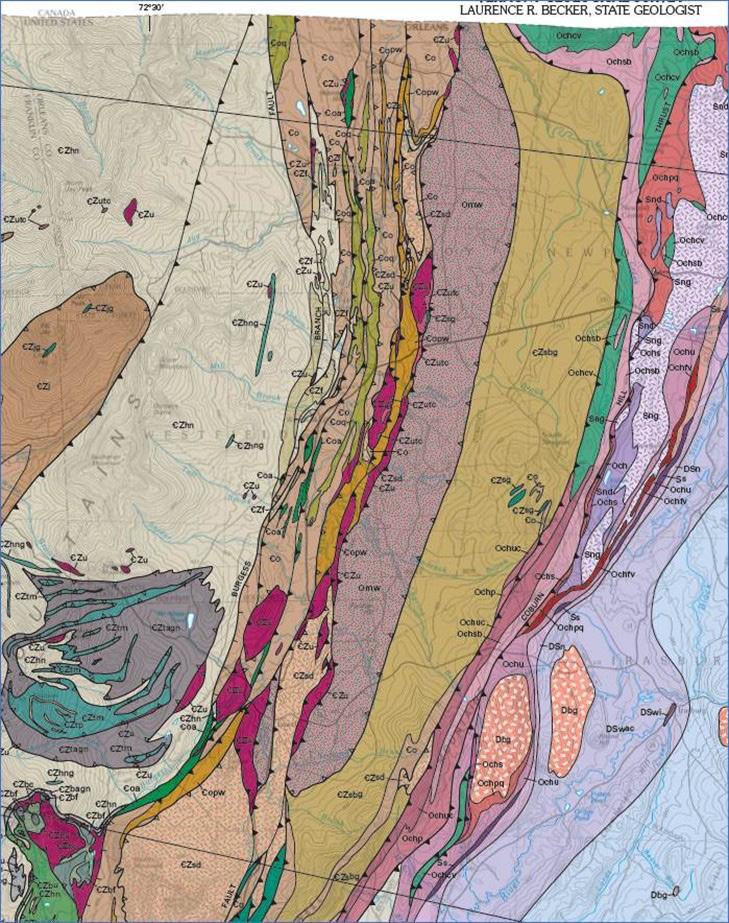
Excerpt from the Bedrock Geologic Map of Vermont (Ratcliffe et al., 2011), displaying the swarm of cranberry-colored ultramafic pods decorating multi-generational thrust slices along the spine of the Green Mountains in north-central Vermont. These ultramafic pods are likely ophiolitic fragments emplaced during the Taconic Orogeny (Van Ballen et al., 2009).
turn, raises rock temperatures and the temperatures of circulating fluids, contributing to the formation of non-volcanic hydrothermal systems. A robust example of this is the Lost City Hydrothermal field (LCHF), discovered in the year 2000, approximately 15 km off the axis of the Mid-Atlantic Ridge north of the Atlantis Fracture Zone. (More about the LCHF later). These factors related to serpentinization change the oceanic crust’s behavior – and its fate.
Serpentinites exposed on land arrived by
convergence, either as ophiolites or disarticulated thrust fault slivers transported toward the hinterland. Ophiolite obduction is problematic, yet oceanic fragments are commonly observed at all modern and ancient accretionary plate boundaries. Highly deformed serpentines are associated with all ophiolitic fragments, hinting at a linkage. Serpentinization of mantle in oceanic lithosphere is suggested as a mechanism triggering “skinning” of ophiolite material from a downgoing subduction slab. Serpentinization creates highly fractured, lower-density, weaker rocks (Schwarzenbach, 2016). These rocks are much more susceptible to decoupling and detachment - slab “skinning” - where an ophiolite is shaved off the top of the subducting slab and underplated beneath an accretionary prism or offscraped onto continental crust. Seismic, gravity, and magnetic investigations have mapped stacked layers of oceanic crust in modern accretionary complexes, which can be tied to obducted ophiolites. (Vogt and Gerya, 2014).
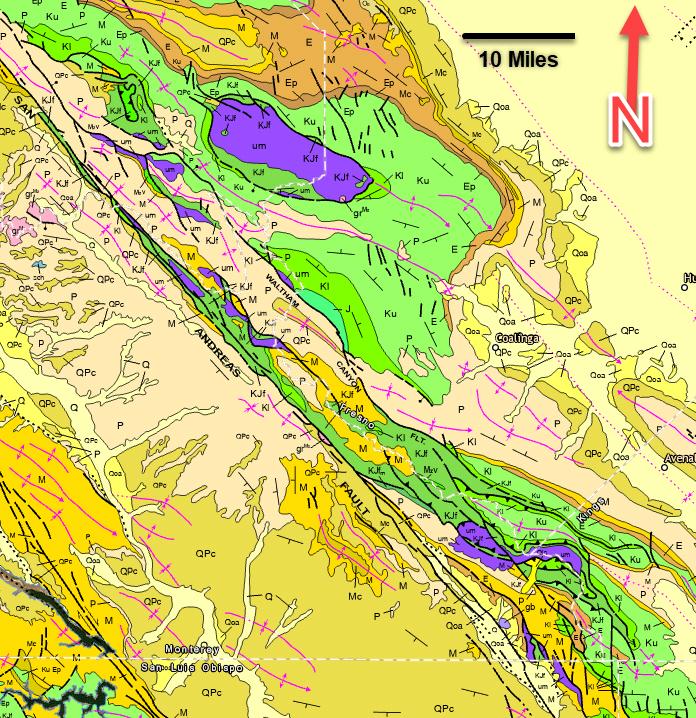
Excerpt of the Geology Map of California from the DataBasin website
The map shows the distribution of ultramafic pods (purple) emplaced as diapirs during the Miocene passage of the Mendocino triple junction (Van Baalen, 2004). Later, these pods were modified by the dextral San Andreas strike-slip fault. The largest pod, the footprint-shaped New Idria serpentine, is shown in two Google Earth zooms. The W tip of the New Idria ultramafic pod is ~10 miles from the NW-SE trace of the San Andreas.
A good example of the occurrence of serpentinites from a collisional orogen is found in Vermont. I’ll use this example because I used to live in Vermont, and I like it. I have included a map that shows a snapshot of the north-central part of the “new” Geologic Map of Vermont (Ratcliffe et al., 2011). In this zoom-in, there is a swarm of cranberry-colored thrust-fault bounded serpentinite pods and slivers that are distributed along a NNE-SSW line. These are a part of the Appalachian serpentine belt, which runs from a hornblende metagabbro in Putnam County, Georgia, to the Bay of Islands ophiolite in Newfoundland (continuing up the side of the Scandanavian Caledonides from the time they were one mountain chain). These ultramafic pods are likely Cambro-Ordovician ophiolitic fragments, emplaced along thrust faults during the Taconic Orogeny (Gale, 1986; Van Baalen et al., 2009) and deformed further during the subsequent Acadian
orogeny in the Late Devonian. Ultramafic pods like this trace the entire Appalachian chain and have been recognized and exploited by humans for a long time (Dann, 1988). The serpentinites mined at Belvidere Mountain near Lowell and Eden, Vermont, were especially well-known for their asbestiform chrysotile, and this locality was the top –and for a while the only - producer of this material in the U.S. (Mindat, 2025). (More on asbestos later). Another distinction of Belvidere Mountain, and other serpentine occurrences along the Appalachians and elsewhere, is an exceptional endowment of mineral treasures highly-prized by mineral collectors and research scientists. Serpentine is not particularly desired as a collector’s mineral,
The footprint of the serpentine barrens at New Idria is clearly etched upon the landscape in the Google Earth view. The New Idria barrens is approximately 12 miles by 3.5 miles with an area of ~44 miles2
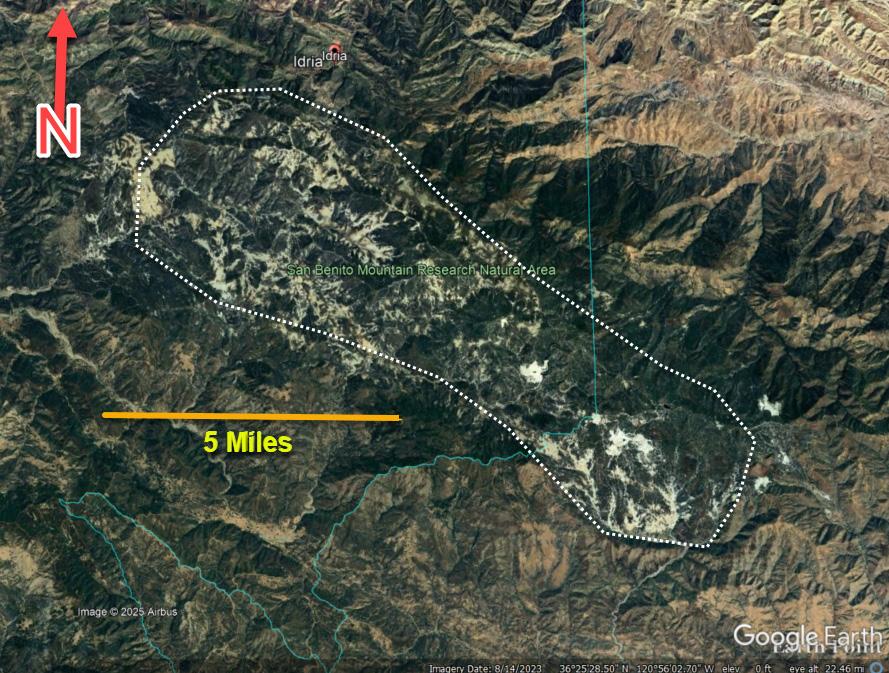
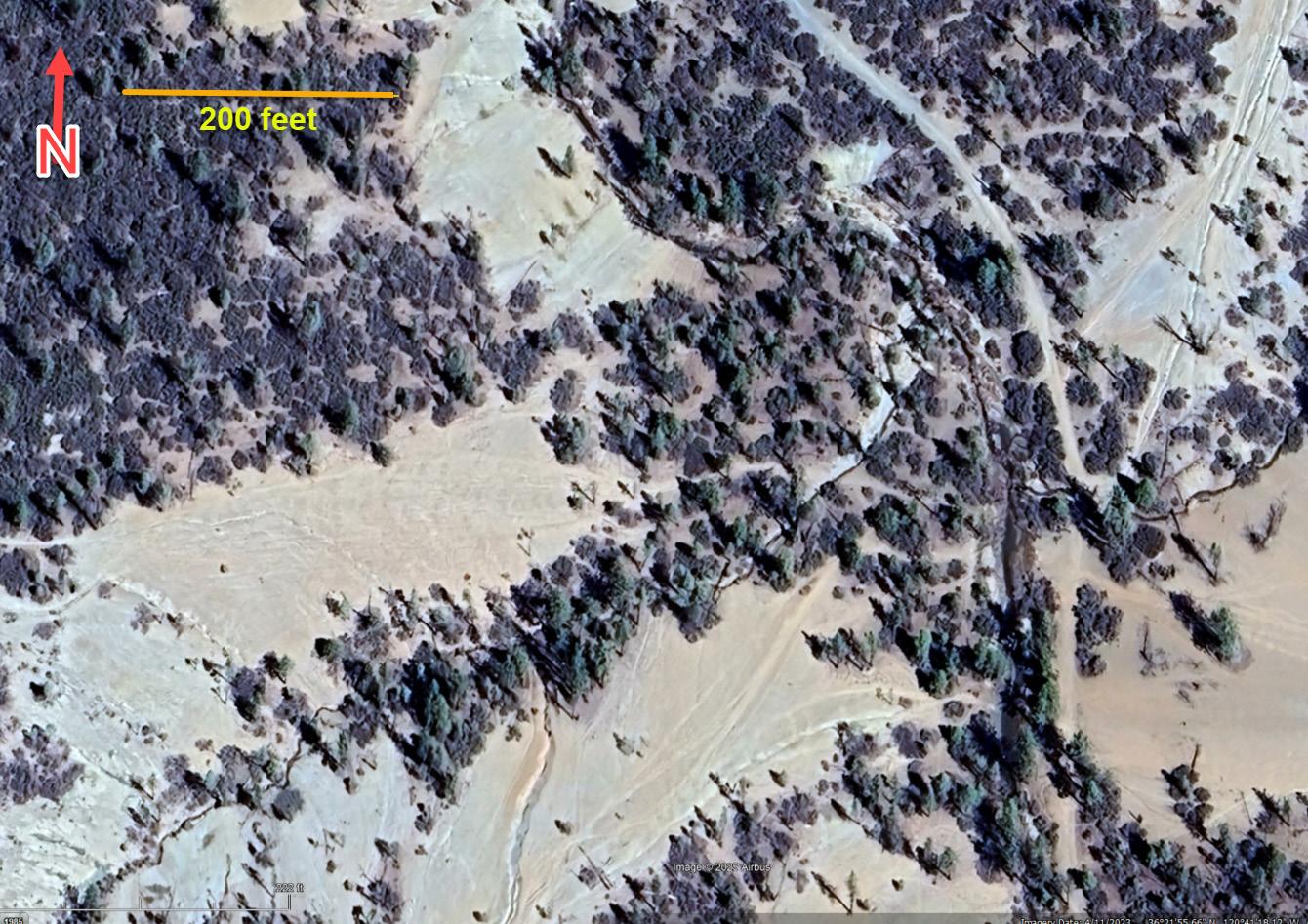
Google Earth zoomin of the New Idria serpentine barrens displaying sparse vegetation cover and eroding soils.
but it keeps good company. Belvidere Mountain is especially well known for world-class grossularite, vesuvianite, and epidote (Carlsen et al., 2015).
Another example of serpentinites in an active tectonic setting is in the New Idria area of California. New Idria hosts an elongate shoeprint-shaped ultramafic pod that is clearly evident from space because of the impact of serpentine on vegetation. The New Idria body is enormous, measuring 12 miles by 3.5 miles. It is only 10 miles from the NNW-SSE trace of the San Andreas fault. Like Belvidere Mountain, the New Idria serpentinite exposure is a complicated mess. It comprises a strange ultramafic diapir that incorporated a mixture of distinct crustal blocks as it rose from mantle depths during the subduction of the Farallon Plate (Tsujimori et al., 2007). Other serpentinite pods decorate the eastern margin of the San Andreas. An interesting feature of the New Idria serpentinites is the occurrence of unusual mineralization, including Hg (which was mined here) and
unusual Ti—bearing species (Van Baalen, 2004). Included in the 162 mineral inventory on Mindat (2025b) are super rare benitoite, BaTi(Si3O9), neptunite, KNa2Li(Fe2+, Mg, Mn)2Ti2(Si4O12)2 and joaquinite, Ba2NaCe2Fe(Ti,Nb)2Si8O26(OH,F)*H2O New Idria is the type locality for 20 minerals on this list, many of which - including benitoite – are known from this locality only. Van Baalen (2004) develops a model to explain the 2 phases of metamorphic alteration at New Idria, with the 2nd resulting from the migration of the Mendocino Triple Junction past this region ~12 ma.
Minerals aren’t the only reason people like serpentine. Serpentine is attractive to the eye, and the human family has utilized it for 10s of thousands of years. One of the earliest known human sculptures is carved from serpentine. The Venus of Galgenberg, discovered near Stratzing, Austria, is carved from serpentine occurring nearby and is dated to ~30,000 BCE (Bonewitz, 2008). Humans have been enthralled with blue and green minerals
Dunite outcrop from the Clear Creek Valley, New Idria, California. The unusual vegetation cover is a common feature of serpentine barrens. Photo used with permission from Mark Van Baalen, Harvard University.
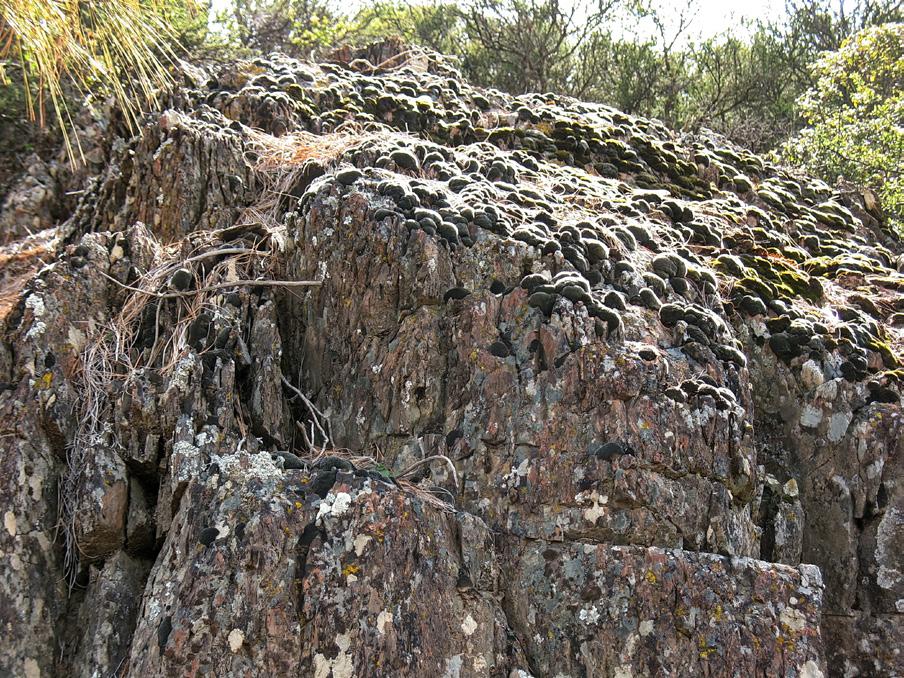
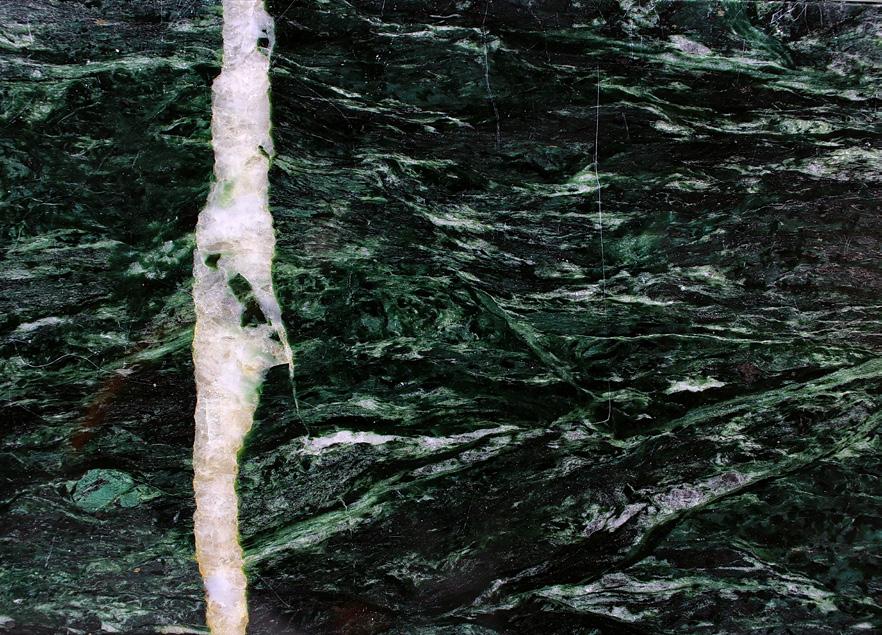
Polished paperweight slab of Verde Antique serpentine “marble” from Rochester, Vermont. The polished face looks highly sheared and is transected by a fracture filled with breccia fragments and dolomite (or magnesite). Asbestiform chrysotile is absent. The slab is 15 cm across. A zoomed picture shows an oblique view of the lower right part of the slab, highlighting oxides (magnetite and chromite) in the serpentine. Photo by Ronald L. Parker.
Oblique view of the serpentine paperweight in the previous picture in reflected light. The light colored blebs are reflections off the metallic luster of magnetite and chromite within the serpentine mass. These features are strongly magnetic. The base of the picture is ~5 cm across.
by Ronald L. Parker.
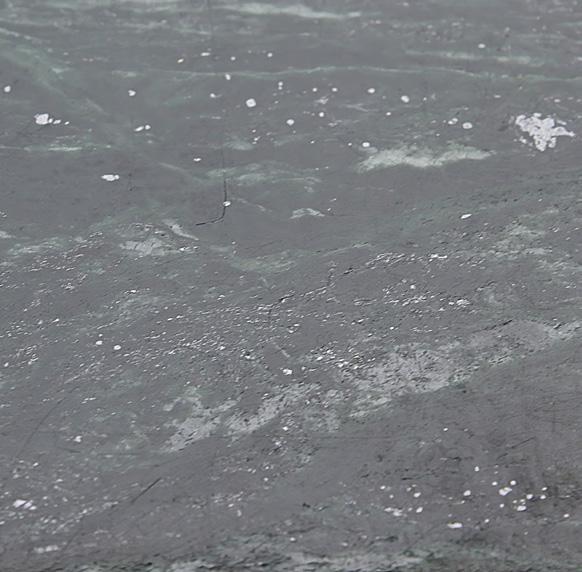
Photo
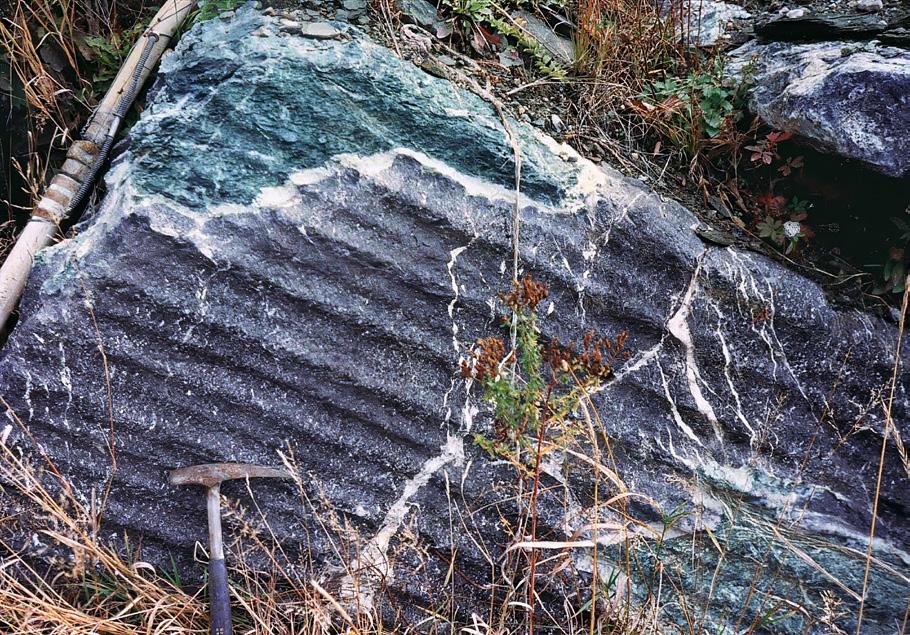
Drone photo of the Vermont Verde Antique Quarry, Rochester, Vermont. Mineralogical analyses of Vermont Verde Antique demonstrate that it is comprised of lizardite and is free of chrysotile asbestos. Photo used with permission from Vermont Verde Antique, LLC.
Block exposing gray magnetite bounded by green serpentine in a roadside block at the Vermont Verde Antique Quarry, Rochester, Vermont. Chromite is likely a significant component of the oxide layer. Photo taken on a Vermont Geological Society field trip in 1992 by Ronald L. Parker.
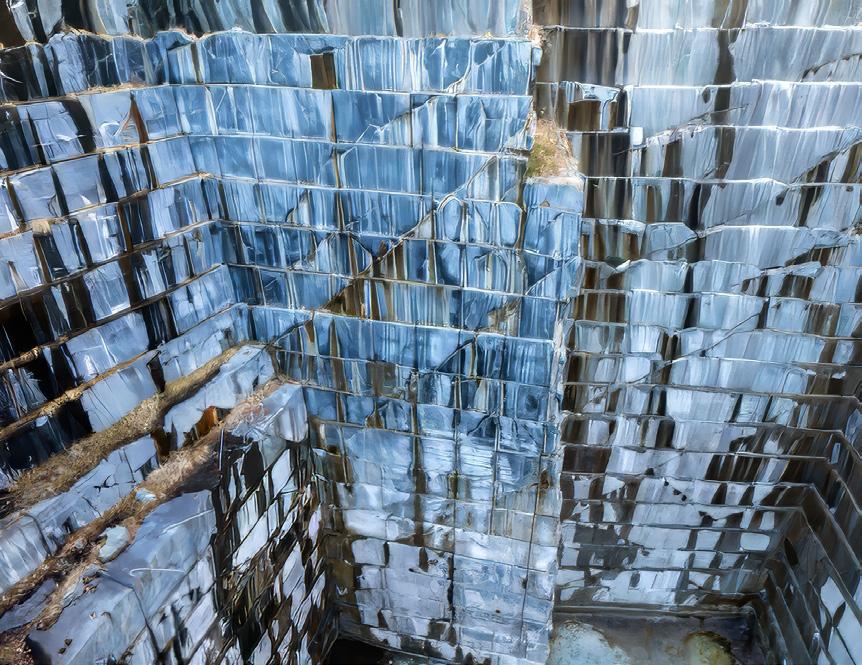
for a long time, as evidenced by the first appearance of green stone beads and pendants at the dawn of the Neolithic (~13,500 to 11,500 B.C.E.). Green stone beads, including serpentine, required extensive trade networks and occurred when the human subsistence strategy shifted to agriculture (Bar-Yosef Mayer and Porat, 2008). Human history is also rife with evidence of people utilizing serpentine’s cousin, talc. Talc [Mg3Si4O10(OH)2] has almost the same chemical formula as serpentine [Mg3Si2O5(OH)4], and for good reason. It forms with serpentine by metasomatic alteration of ultramafic precursor minerals. Talc differs from serpentine in that it is a 2:1 trioctahedral phyllosilicate – it has two tetrahedral silica sheets sandwiching the octahedral layer, not just 1. Archaeologists recognize that serpentine’s cousin rock, soapstone or steatite (aka serpentine talc), was a prized commodity to ancient cultures because it was so soft that it could easily be carved into bowls, pipes, oil lamps, and many other valuable items. Ancient cultures spent valuable energy harvesting material from soapstone quarries before the full implementation of pottery techniques. Today, soapstone’s high heat retention and chemical inertness guarantees its continued use as pancake griddles, wood-burning stoves, and chemistry lab counter tops and sinks (Dann, 1988; Parker, 2018).
Since ancient times, People have used serpentine as decorative building material, quarrying this stone in slabs and columns for use inside and outside. Variable mineral composition and deformation history impart distinct color and textural variations to serpentines quarried for architectural purposes. Carmignano et al. (2023) catalog more than 60 different names of serpentine used as architectural stone since antiquity. Some examples include: Ophite, Serpentina Fiorita, ophicalcite, Verd Antico Sanguigno Picchiettato, and Verde Antique. I have a small paperweight made of Vermont Verde Antique that I have had since 1992 (photos). This “serpentine marble” is very dark green, is highly-sheared, and captures a bright white magnesite (MgCO3) filled fracture with breccia fragments. In reflected light, this small slab has visible metallic oxide grains, which are strongly magnetic. (Try to detect metallic oxides when looking at a
serpentine building stone slab.)!
Serpentine is not always sympathetic to human agendas. Soils that develop on exposed serpentine bedrock are typically poor media for plant growth, leading them to be termed “barrens” (Dixon, 1989; Dann, 1988). In the Alps, farmers refer to serpentine barrens (bosses) as “monts morts” – dead mountains (Faust and Fahey, 1962). Serpentine barrens resist the most dedicated human endeavors to subdue them – they won’t grow crops no matter the amount of fertilizer or irrigation (Dann, 1988). Arnold et al. (2016) put it this way: “Serpentine barrens are enormously hostile to plant life” (p. 8320). As a result of a poisonous environment, the plants that DO colonize serpentine soils are highly adapted and specialized, some being endemic only to serpentine barrens. Genetics study of plants adapted to serpentine barrens may help to design resilient crops (Arnold et al., 2016).
In his excellent book, “Traces on the Appalachians: A Natural History of Serpentine in Eastern North America”, Dr. Kevin Dann describes his initiation to serpentine barrens on a 1977 college field trip to New Idria, California.
“After piloting our two vans along some precipitous logging roads, we ended up at what looked like the seventh ring of Dante’s Inferno. Desolate gray-green hills broken only by an occasional sickly looking digger pine stretched in every direction. All of us – geologists and botanists alike – were outraged that we’d left the paradisical Big Sur for this hell” (Dann, 1988, p. xi).
Low ecosystem productivity in serpentine landscapes reflects adverse nutrient supply conditions, poor moisture delivery, and heavy metal toxicity. Elemental components of serpentine soils are highly skewed - often typified by very low Ca2+ to Mg2+ ratios and limiting abundances of the fertility elements K, N, and P. Root penetration in these spare soils is weak; little humus is added from biomass, and poor water retention facilitates erosion. The hostility of serpentine barrens to plant growth is accentuated by heavy metals (Cr, Fe, Ni, Co), often at phytotoxic concentrations. These characteristics render serpentine barrens unfit for other uses, with no pasture where grass doesn’t grow and no timber industry where the trees are
stunted and misshapen. Serpentine bedrock repels human invasion, except where mineral resources are concerned (Dann, 1988; Arnold et al., 2016).
Serpentine is perhaps best known among the general public for the (now) skunk at the picnic: asbestos. Asbestos is a generic name for a select group of minerals with asbestiform habit, defined as an aspect ratio of length to width greater than 100 to 1. Two types of asbestiform silicates are recognized: the fibrous form of serpentine (chrysotile) and five amphibole varieties. Chrysotile, known as white asbestos, was ~95% of all asbestos production. Only 2 other asbestiform minerals were mined commercially: grunerite (brown asbestos or amosite) and riebeckite (blue asbestos or crocidolite). Anthophyllite, actinolite, and tremolite were never commercially exploited (Chang, 2002).
The ancient Greeks and Romans considered asbestos a miracle material: cloth woven from it could not be consumed by fire. Known as amiantos, asbestos was thought to ward off evil influences. In the 1st century, Pliny the Elder was the first to recognize that respiratory diseases bedeviled asbestos weavers. Because life expectancy was shorter then, asbestos-related deaths were probably unrecognized due to a long latency period for asbestos diseases (Chaline, 2012). During the century before ~1970, asbestos was regarded as a miracle substance for the industrialized world. Chrysotile properties include a high melting point, flame resistance, high tensile strength, the ability to be woven, electrical resistivity, inertness to most chemical reactions, low solubility, etc. At one time, chrysotile was one of the most essential substances on the planet, with more than 3,000 applications. These uses included brake and clutch linings, high pressure gaskets, fire-retardant insulation, roofing, siding, flooring, drywall and textiles, heat resistant coatings for ducts, appliances, boilers and hot water systems, cemented composite board, adhesives, chlorine gas (Cl2) and sodium hydroxide (NaOH) manufacture for chemical feedstocks and water disinfection uses (Chang, 2002).
Today, asbestos is a recognized human health hazard, directly responsible for asbestosis, lung, ovarian, and laryngeal cancer, and mesothelioma
(cancer of the pleural lining of the lung). Because of the recognition that breathing asbestos fibers constitutes a serious human health hazard, regulatory programs have been put in place around the globe to govern the use of asbestos or to ban it completely (Pena-Castro et al., 2023). Regulatory responses are not uniform globally. On March 28, 2024, the United States Environmental Protection Agency delivered a Final Rule (40 CFR Part 751) summarily banning chrysotile asbestos’s manufacture, import, processing, distribution, use, or disposal (USEPA, 2024).
Like many others, I have a personal relationship with asbestos. As Chang (2002) stated near the turn of the century, the properties of chrysotile “…are a special combination and make it difficult to find an asbestos-free substance (p. 16). In 1986, the United States passed the Asbestos Hazard Emergency Response Act (AHERA). AHERA governed the mitigation of asbestos hazards in schools across the country. In the summer of 1986, I worked as an asbestos remediation worker, removing asbestos pipe insulation and cement coatings from boilers and other HVAC system components in schools around Burlington, Vermont. It was hard, hot work in a full-face respirator and full Tyvek suit seamed with duct tape. We worked in rooms fully shrouded in duct-taped clear plastic sheeting with negative airflow pulled through high-efficiency particulate air (HEPA) filters. Entering and exiting the plastic-covered rooms was accomplished through a 2-stage shower system. My experience doing this work was in the summer, so I was always very hot, sweaty, uncomfortable, and very much in a close, eye-contact relationship with chrysotile. I’m sure almost everyone has an asbestos story. (I have more!).
Despite this dark side, serpentine is also a magical substance. The magic lies within the thermodynamics that drive the serpentinization reaction to the lowest redox conditions possible when hydrating mantle peridotite. This chemical transformation may be summarized as follows:
olivine ± orthopyroxene + H20 serpentine ± brucite ± talc ± magnetite + H2
This simplified chemical reaction (as all
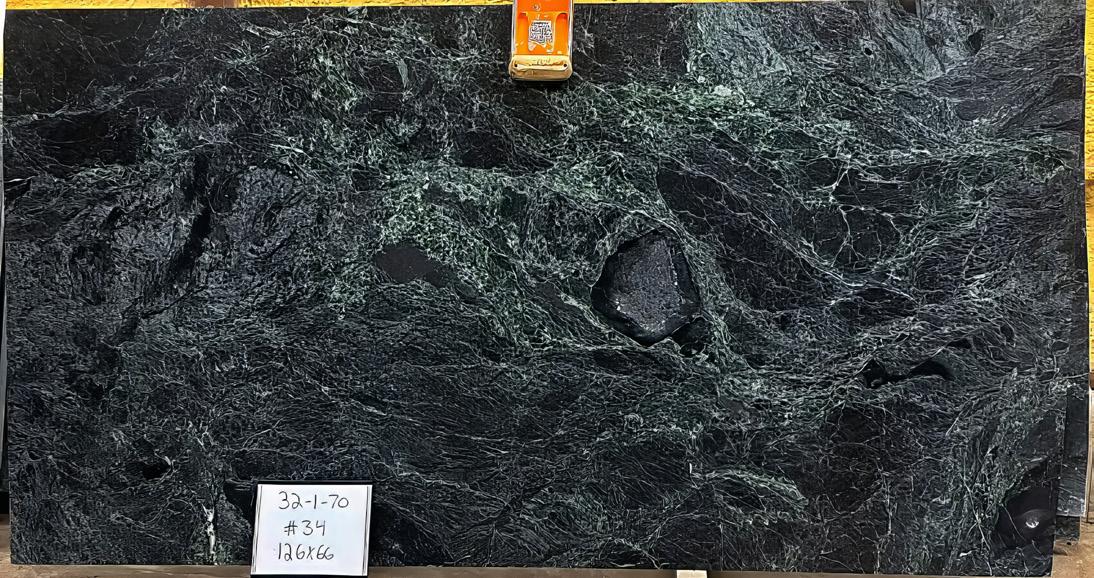
Large slab of polished Verde Antique measuring 126” x 66” from the Vermont Verde Antique Quarry in Rochester, Vermont. There appears to be a metallic oxide-rich pod surrounded by a reaction rim just to the right of center. Verde Antique does not contain asbestos. Photo used with permission from Vermont Verde Antique, LLC.
Two rows of Vermont Verde Antique columns in the Plaque Gallery of the Baseball Hall of Fame, Cooperstown, New York. Straight ahead, the Rotunda is a glass-topped Verde Antique dome. Photo used with permission from Vermont Verde Antique, LLC.
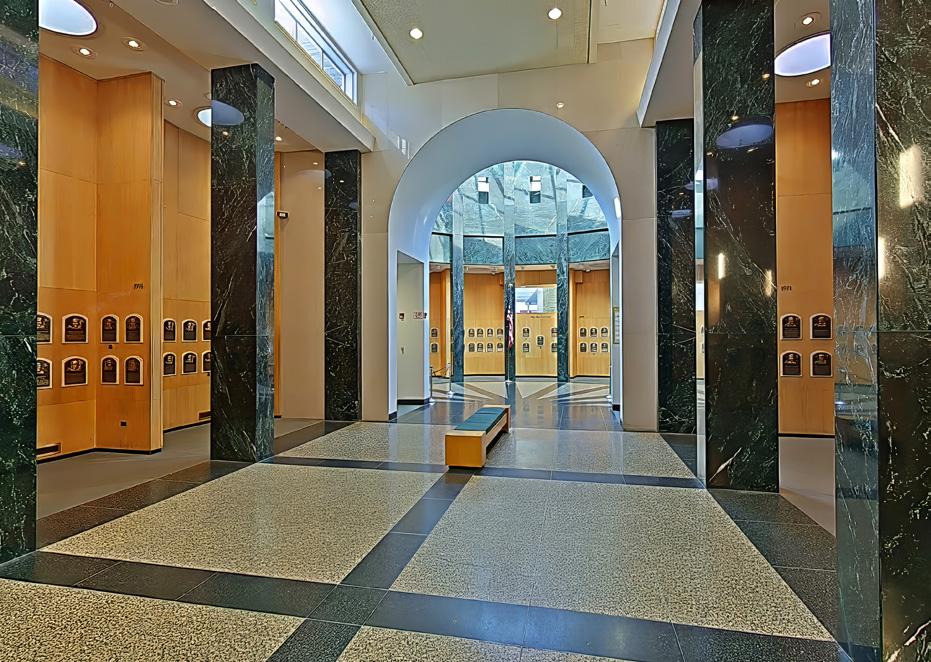
chemical reactions) depends upon conditions such as pressure, temperature, concentration gradients, etc. The most significant part of this chemical reaction is that it yields molecular dihydrogen – H2. During the serpentinization reaction, Fe2+, removed from olivine and Opx, is oxidized by H2O to form the Fe3+ in magnetite (Fe3O4). (Sleep et al., 2004). In the highly reducing conditions of this reaction, the remaining H2 is liberated as a free molecule.
Hydrogen gas from serpentine has been shown to reduce CO2, creating abiotic methane (Etiope and Sherwood Lollar, 2013). The reaction is:
4H2 + CO2 CH4 + 2H2O
Abiotic methane is the building block for the abiotic construction of more complex organic molecules. This process is observed on Earth today. Abiotic CH4, derived by low temperature (<150°) serpentinization of ultramafic rocks, was discovered at the submarine Lost City Hydrothermal field (LCHF) in 2000. Kelley et al. (2005) cite evidence that ultramafic-hosted hydrothermal systems similar to the LCHF have been operating for most of Earth’s history. Abiotic methane is also noted from ophiolites in the Philippines, Oman, New Zealand, Turkey, Italy, Greece, and Japan (Etiope and Sherwood Lollar, 2013). Serpentinization was operational on early Earth and is considered the principal energy source for pre-photosynthetic life here (Muntener, 2010). Without the thermodynamic magic of serpentinization, it is arguable that there would be no life on Earth.
When looking for evidence of life on Mars or other terrestrial planetary bodies, evidence of serpentine is a strong indicator that life is possible. McCollom et al. (2022) put it this way. “Serpentinization of olivine-rich ultramafic rocks is increasingly recognized to have been widespread in the solar system throughout its history. This process has gained particular attention among planetary scientists because it generates molecular hydrogen (H2) and methane (CH4), compounds that can supply metabolic energy to biological communities and contribute to greenhouse warming of planetary atmospheres.” Serpentine has been identified as a rare mineral on Mars by the Compact
Reconnaissance Imaging Spectrometer for Mars (CRISM) (Ehlmann et al., 2010). More recently, additional serpentinites have been identified on Mars, and their distribution permits a better understanding of past climate and the history of water on the planet (Emran, 2025). Serpentine minerals have also been identified on the dwarf planet Ceres and are proposed to occur in the rocky interiors of the icy moons Europa and Enceladus (McCollom et al., 2022).
Serpentine can interact with the atmosphere in another way, namely by removing CO2. Natural carbonation by weathering and low-temperature alteration of exposed mantle peridotites is a significant sink for CO2, creating stable carbonate minerals like calcite or magnesite (Keleman and Matter, 2008).
Molecular hydrogen from serpentinization is an essential energy source for many microorganism communities. It could also be a vital energy source for humanity (Hand, 2023). Hydrogen is a desirable fuel because its combustion products are water and heat. The future hydrogen economy will use H2 produced in many ways. One source of hydrogen germane to the discussion here is Natural, Native, or Geologic Hydrogen (aka white or golden hydrogen), which is derived from Precambrian crystalline shields, serpentinized ultramafic rocks, or uranium-rich rocks (Gaucher, 2020). H2 seeps, often with attendant abiotic CH4, are known from most of the ophiolite complexes of the world. One of these, Mount Chimaera in Turkey, hosts an eternal flame that has been burning for at least 2000 years. The gas at Mount Chimaera is 87% abiotic methane and 10% hydrogen (Zgonnik, 2020). The source of the gas seep is low-temperature serpentinization below the mountain (Gaucher, 2020). The world’s only documented hydrogen-producing well is in Bourakebougo, Mali, yielding approximately 1,500 m3 of 98% H2/day without slowing down. The International Energy Agency (IEA) estimated that in 2023, 40 companies were active in the search for natural hydrogen (IEA, 2024). One upside of serpentine-derived hydrogen is that, in some situations, it appears to be continually manufactured – a renewable resource. This explains why the Turkish eternal flame and
D eta ile d a nd ac c ura te g eo lo gy at yo u r fin g ertip s in Pe tra , Ge o Grap h ix, A rc GIS , A cc u Map , ge o S C OU T an d o th er d ig ital ma p pin g a p plica tion s
We ste rn USA
Geol ogi cal Edge Set
Ene rgy Al te rnati ve s & Cri ti cal Mine ral s - USA
We ste rn Cana da Geol ogi cal Edge Set
Ene rgy Al te rnati ve s & Cri ti cal Mine ral s - Ca nada
Ea ste rn USA & Ea st ern Ca nada
Geol ogi cal Edge Set
Cent ral USA
Geol ogi cal Edge Set
Me xi co
Geol ogi cal Edge Set
Map la ye rs p rov ided in s ha pe file fo rma t fo r ea sy impor t into a ll ma ppin g app lic at ions .
De liv er ab le s inc lude : f orma tion limits , o utc ro ps, su bc rop e dge s , O &G fie ld s, st ruc tu ra l eleme nt s, re ef s, sh ore lin es , c ha nne ls, pro duc tion f airw a ys , s ha le ga s tr en ds, st ru ct ure con tou rs , iso pa chs , g en era l cult ure , r ene w able & no n-r en ew ab le ene rg y pr ojec ts , min era l dep os it s, mine s, Pet ra T he mat ic Ma p pro je ct s, G eoG ra phix G e oA tla s pro je c ts , Ar cG IS M XD a nd La y er f ile s, re giona l c ro ss -s ec tion s, an d full te ch nica l s upp ort .
For mo re inf orma tio n: Joe l H a rding at + 1 4 03 87 0 8 12 2 joe lha rding@ g eoe dg es .c om ww w.ge oe dge s.c om
the Mali hydrogen well don’t experience depletion (USGS, 2023; Jackson et al., 2024).
The USGS has published research mapping the potential for natural hydrogen occurrence (USGS, 2023; Gelman et al., 2025). Natural hydrogen is already recognized from the midcontinent, the Four Corners (AZ, CO, NM, and UT), and coastal California. In their hydrogen prospectivity map, Gelman et al. (2025) break out five serpentine-related components with hydrogen potential. Among these, the East Coast Magnetic Anomaly (ECMA) is significant, an offshore positive magnetic anomaly at the rifted Atlantic margin separating oceanic from continental crust. This feature runs from Georgia to Nova Scotia. Hydrogen genesis and long-distance transport from the ECMA have been implicated as causal factors in the creation of Carolina Bays (Zgonnik et al., 2015; Gelman et al., 2025). Another important serpentine-hydrogen component is failed continental rifts filled with mafic and ultramafic rock. These include the Reelfoot rift, the Ouachita aulacogen, and the Midcontinent Rift (MCR) (Gelman et al., 2025). As the upcoming (April 8-10, 2025) RMAG North American Helium & Hydrogen Conference attests, exploring for natural hydrogen is hot right now. The future will tell whether developing these resources will pan out or not. If so, another feather in the cap of serpentine!
Serpentine and serpentinites! Beautiful minerals and rocks that humanity has used for thousands of years (Good!). Deadly respiratory health hazard that has adversely impacted the lives of many (Bad!) A genuinely remarkable mineral family that may have been the prerequisite material initiating life in the solar system (Miraculous!).
ACKNOWLEDGEMENTS
Several people helped me with this project, and I would like to thank them. I received photos and advice from former Colgate classmate Jonathan Kim from the Vermont Geological Survey, from former office mate Greg Walsh at the USGS, from serpentine (and New Idria) expert Mark Van Baalen of Harvard University, and from Peter Fabbioli of Vermont Verde Antique, LLC. The idea to
write about serpentine started after I read Kevin Dann’s excellent natural history of Appalachian serpentines. Thanks, Kevin, for the concept and the conversations!
ONLINE REFERENCES
• https://en.wikipedia.org/wiki/Serpentinite
• https://en.wikipedia.org/wiki/Serpentine_subgroup
• https://www.mindat.org/min-11135.html
• https://www.mindat.org/loc-4559.html
• https://www.mindat.org/loc-6418.html
• https://geology.com/minerals/serpentine.shtml
• https://vtverde.com/
• https://www.congress.gov/bill/99th-congress/house-bill/5073
• https://www.atsdr.cdc.gov/asbestos/about/index.html
• https://www.usgs.gov/news/featured-story/ potential-geologic-hydrogen-next-generation-energy
REFERENCES
Arnold, Brian J., Brett Lahner, Jeffrey M. DaCosta, Caroline M. Weisman, Jesse D. Hollister, David E. Salt, Kirsten Bomblies and Levi Yant, 2016, Borrowed Alleles and Convergence in Serpentine Adaptation, Proceedings of the National Academy of Sciences, 113 (29): 83208325. https://doi.org/10.1073/pnas.1600405113
Bar-Yosef Mayer, Daniella E. & Naomi Porat, 2008, Green Stone Beads at the Dawn of Agriculture, Proceedings of the National Academy of Sciences, 105:8548–8551. Available on-line https://www.pnas.org/ content/pnas/105/25/8548.full.pdf
Bonewitz, Ronald Louis, 2008, Rock and Gem: The Definitive Guide to Rocks, Minerals, Gems and Fossils, New York, New York: Dorling-Kindersley Limited, 360 pp.
Carlsen, Kenneth S., Marjorie H. Gale, Woodrow B. Thompson and Matthew Kierstead, 2015, Belvidere Mountain Asbestos Quarries, Lowell/Eden, Vermont, Rocks & Minerals, 90(6): 510-551. https://doi. org/10.1080/00357529.2015.1080535
Carmignano, Ottavio Raul Domenico Riberti, and Paulo Roberto G. Brandao, 2023, Employment of Serpentine Rock in Architecture, Material Science & Engineering International Journal, 7(2): 79-81. https://doi.org/10.15406/mseij.2023.07.00208
Chaline, Eric, 2012, Asbestos, in Fifty Minerals that Changed the Course of History, Buffalo, New York, Firefly Books, Inc., pp. 30-33.
Chang, Luke L.Y., 2002, Asbestos, in Industrial Mineralogy: Materials, Processes and Uses, Prentice Hall: Upper Saddle River, New Jersey, pp. 5-18.
Coveney, Raymond M., Edwin D. Goebel, Edward J. Zeller, Gisela A. M. Dreschhoff and Ernest E. Angino, 1987, Serpentinization and the Origin of Hydrogen Gas in Kansas, AAPG Bulletin, 71: 39-48.
Dann, Kevin T., 1988, Traces on the Appalachians: A Natural History of Serpentine in Eastern North America, Rutgers University Press, 159 pp. DataBasin, 2025, Geology Map of California, DataBasin website. https://databasin.org/maps/new/#datasets=d558e622040d494eb71c37c74f7b6994 Accessed 2/23/24.
Dixon, J. B., 1989, Chapter 10: Kaolin and Serpentine Group Minerals, in Minerals in Soil Environments, 2nd ed, edited by J. B. Dixon and S. B. Weed, Soil Science Society of America Book Series #1, pp. 467-525.
Ehlmann, B. L., J. F. Mustard and S. L. Murchie, 2010, Geologic Setting of Serpentine on Mars, Geophysical Research Letters, 37: L06201, 5 p., https://doi:10.1029/2010GL042596
Emran, A., J. D. Tarnas and K. M. Stack, 2025, Global Distribution of Serpentine on Mars, Geophysical Research Letters, 52, https://doi. org/10.1029/2024GL110630
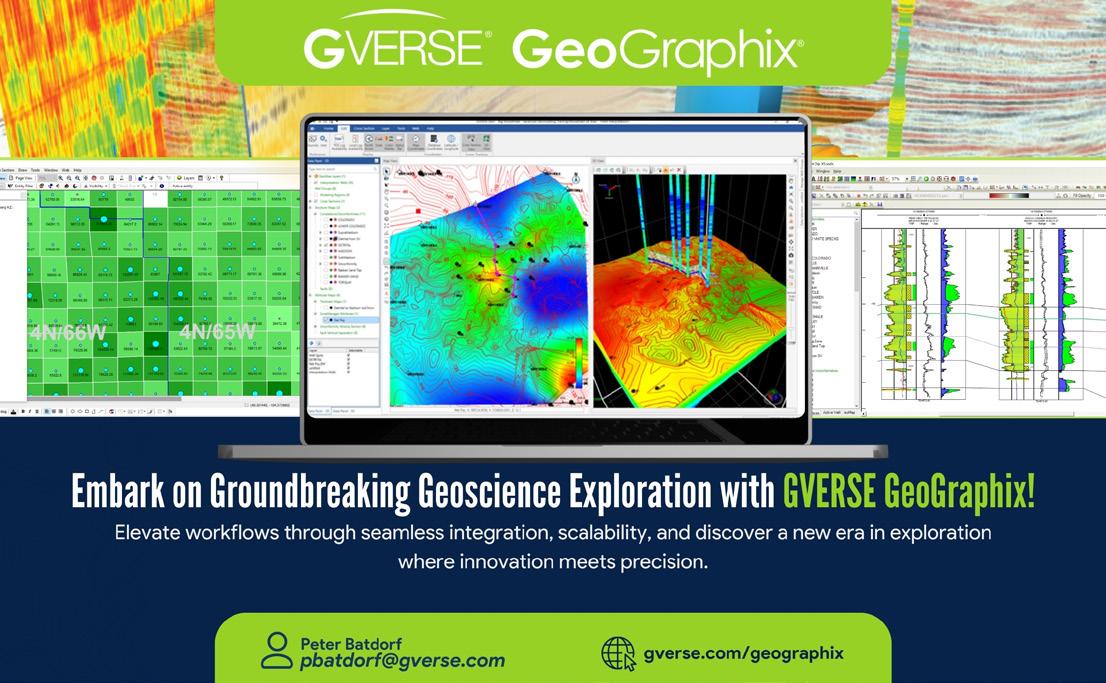
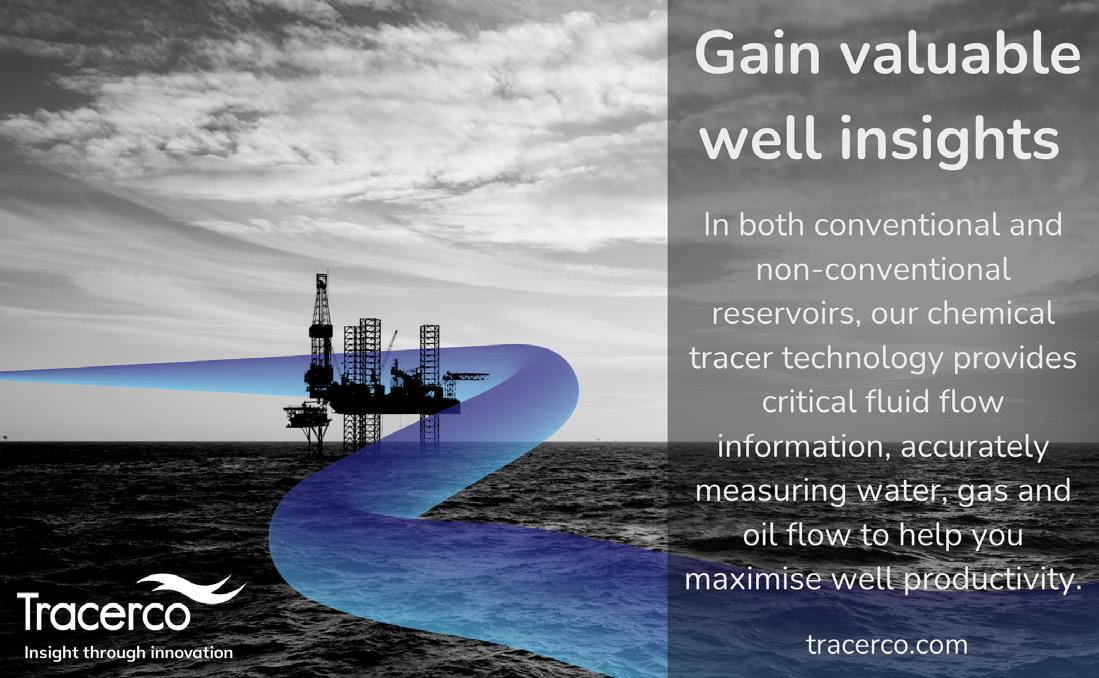
Etiope, Guiseppe and Barbara Sherwood Lollar, 2013, Abiotic Methane on Earth, Reviews in Geophysics, 51: 276-299.
Gale, Marjorie H., 1986, Geologic Map of the Belvidere Mountain Area, Eden and Lowell, Vermont, USGS Miscellaneous Investigations Series I-1560 https://doi.org/10.3133/i1560
Gelman, Sarah E., Jane S. Hearon and Geoffrey S. Ellis, 2025, Prospectivity Mapping for Geological Hydrogen, USGS Professional Paper 1900, 43 pp. https://doi.org/10.3133/pp1900
Gaucher, Eric C., 2020, New Perspectives in the Industrial Exploration for Native Hydrogen, Elements, 16(1): 8-9. https://doi.org/10.2138/ gselements.16.1.8
Hand, Eric, 2023, Hidden Hydrogen: Does Earth Hold Vast Stores of a Renewable, Carbon-Free Fuel? Science, 379(6633): 630-636. doi: 10.1126/science.adh1460
International Energy Agency, 2024, Global Hydrogen Review 2024, https://iea.blob.core.windows.net/assets/89c1e382-dc59-46caaa47-9f7d41531ab5/GlobalHydrogenReview2024.pdf
Jackson, Owain, Steve R. Lawrence, Ian P. Hutchinson, Andrew E. Stocks, Andrew C. Barnicoat and Mike Powney, 2024, Natural Hydrogen: Sources, Systems and Exploration Plays, Geoenergy, 2: 1-9, https://doi.org/10.1144/ geoenergy2024-002?ref=pdf&rel=cite-as&jav=VoR
Johnsen, Ole, 2002, Minerals of the World: Princeton University Press, Princeton, N.J. 439 pp.
Keleman, Peter B., and Jug Matter, 2008, In Situ Carbonation of Peridotite for CO2 Storage, Proceedings of the National Academy of Sciences, 105: 17,295-17,300. https://doi:10.1073/pnas.0805794105
Kelley, Deborah S., Jeffrey A. Karson, Gretchen L. Fruh-Green, Dana R. Yoerger, Timothy M. Shank, David A. Butterfield, John M. Hayes, Matthew O. Schrenk, Eric J. Olson, Giora Proskurowski, Mike Jakuba, Al Bradley, Ben Larson, Kristin Ludwig, Deborah Glickson, Kate Buckman, Alexander S. Bradley, William J. Brazelton, Kevin Roe, Mitch J. Elend, Adelie Delacour, Stefano M. Bernasconi, Marvin D. Lilley, John A. Baross, Roger E. Summons and Sean P. Sylva, 2005, A Serpentinite-Hosted Ecosystem: The Lost City Hydrothermal Field, Science, 307: 1428-1434. https://doi. org/10.1126/science.1102556
Klein, Cornelis, 2002, The 22nd Edition of the Manual of Mineral Science: New York, John Wiley & Sons, Inc., 641 pp. McCollom, Thomas M., Frieder Klein and Mitchell Ramba, 2022, Hydrogen Generation from Serpentinization of Iron-rich Olivine on Mars, Icy Moons and other Planetary Bodies, Icarus, 372:114754, https://doi.org/10.1016/j.icarus.2021.114754
Mindat, 2025a, Belvidere Mountain Quarries, Lowell & Eden, Orleans & Lamoille COs., Vermont, USA, https://www.mindat.org/loc-4559.html Accessed 2-20-25
Mindat, 2025b, New Idria Mining District, San Benito County, California, USA, https://www.mindat.org/loc-6418.html Accessed 2/27/25
Muntener, Othmar, 2010, Serpentine and Serpentinization: A Link Between Planet Formation and Life, Geology, 38(10): 959-960 https:// doi.org/10.1130/focus102010.1
Murphy, Jack A., 2004, Geology Tour of Denver’s Buildings and Monuments, Denver, Colorado: Historic Denver, Inc., 96 pp. Parker, Ronald L., 2018, Talc: Usefully Soft, Outcrop, the Monthly Newsletter of the Rocky Mountain Association of Geologists, 67(2): 18-25. https://issuu.com/rmagdenver/docs/ february_2018_outcrop/18
_____________, 2021, Wollastonite: The Most Versatile Pyroxenoid, Outcrop, the Monthly Newsletter of the Rocky Mountain Association of Geologists, 70(4): 34-41. https://issuu.com/rmagdenver/docs/
oc_apr2021_v02/34
Pena-Castro, Margareth, Michelle Montero-Acosta, and Manuel Saba, 2023, A Critical Review of Asbestos Concentrations in Water and Air According to Exposure Sources, Heliyon e15730. https://doi. org/10.1016/j.heliyon.2023.e15730
Plumper, Oliver, Anja Royne, Anna Magraso and Bjorn Jamtveit, The Interface-Scale Mechanism of Reaction Induced Fracturing During Serpentinization, Geology, 40(12): 1103-1106. https://doi:10.1130/ G33390.1
Rakovan, John, 2011, Word to the Wise: Serpentine, California’s State Rock, Rocks & Minerals, 86(1):63-68. https://doi.org/10.1080/00357 529.2011.537187
Ratcliffe, Nicholas M., Rolfe S. Stanley, Marjorie H. Gale, Peter J. Thompson, and Gregory J. Walsh, 2011, Bedrock Geologic Map of Vermont, United States Geological Survey Investigations Map 3184, https://pubs.usgs.gov/sim/3184/
Rupke, Lars H., Jason Phipps Morgan, Matthias Hart and James A. D. Connelly, 2004, Serpentine and the Subduction Zone Water Cycle, Earth and Planetary Science Letters, 223(1-2):17-34. https://doi. org/10.1016/j.epsl.2004.04.018
Schwarzenbach, Esther M., 2016, Serpentinization and the Formation of Fluid Pathways, Geology, 44(2): 175-176 doi:10.1130/ focus022016.1
Sleep, N. H., A. Meibom, Th. Fridriksson, R. G. Coleman and D. K. Bird, 2004, H2-Rich Fluids from Serpentinization: Geochemical and Biotic Implications, Proceedings of the National Academy of Sciences, 101(35):12818-12823.
Tsujimori, Tatsuki, Juhn G. Liou and Robert G. Coleman, 2007, Finding High-Grade Tectonic Blocks from the New Idria Serpentinite Body, Diablo Range, California: Petrologic Constraints on the Tectonic Evolution of an Active Serpentine Diapir, in, Geological Society of America Special Paper 419, p. 67-80, doi:10.1130/2007.2419(03).
USEPA, 2024, Asbestos Part 1: Chrysotile Asbestos: Final Rule, Federal Register, 89(61): 21970-22010 https://www.govinfo.gov/content/ pkg/FR-2024-03-28/pdf/2024-05972.pdf
USGS, 2023, The Potential for Geologic Hydrogen for Next GenerationEnergy, https://www.usgs.gov/news/featured-story/potential-geologic-hydrogen-next-generation-energy website, Accessed 2/27/25
Van Baalen, M. R., 2004, Migration of the Mendocino Triple Junction and the Origin of Titanium-Rich Mineral Suites at New Idria, California, International Geology Review, 40: 671-692.
Van Baalen, M. R., Brook T. Mossman, Mickey E. Gunter and Carl A. Francis, 2009, Environmental Geology of Belvidere Mt. Vermont, in D. S Westerman and A. S. Lathrop, eds., Guidebook for Fieldtrips in the Northeast Kingdom of Vermont and Adjacent Regions, New England Intercollegiate Geologic Conference, 101st Annual Meeting, pp. B1-1 to 23.
Vogt, Katharina and Taras Gerya, 2014, Deep Plate Serpentinization Triggers Skinning of Subduction Slabs, Geology, 42(8): 723-726. https://doi:10.1130/G35565.1
Zgonnik, Viacheslav, 2020, The Occurrence and Geoscience of Natural Hydrogen: A Comprehensive Review, Earth Science Reviews, 203: 103140 https://doi.org/10.1016/j.earscirev.2020.103140
Zgonnik, Viacheslav, Valerie Beaumont, Eric Deville, Nikolay Larin, Daniel Pillot and Kathleen M. Farrell, 2015, Evidence for Natural Molecular Hydrogen Seepage Associated with Carolina Bays (Surficial, Ovoid Depressions on the Atlantic Coastal Plain, Province of the USA), Progress in Earth and Planetary Science, p. 2-31 DOI 10.1186/ s40645-015-0062-5
IN THE PIPELINE
MARCH 5, 2025
RMAG Luncheon.
Speaker Jonathan McKenna. Talk Title: “Optimizing Return on Investment and Estimated Ultimate Recovery Through Advanced Micro-Seismic Monitoring Techniques.” In Person or Online. Denver Earth Resources Library, 730 17th Street, B1, Denver.
MARCH 7, 2025
DAPL Ski Day. Keystone, CO.
MARCH 20, 2025
WOGA Lean-In. Speaker: Kat Mann. “Making Big Asks.” Dominion Plaza, 600 17th Street, Ste. 1400n, Denver. 11:00 AM12:30 PM.
WELCOME NEW RMAG MEMBERS!
Claudia Duenas with Koloma, from Denver, Colorado
Michael Tummons with S&P Global from Aurora, Colorado
Emily Swanson with Civitas, from Wheat Ridge, Colorado
Kassandra Steele from Golden, Colorado
Allegra Hosford Scheirer with Geomodeling Solutions, from Mountain View California
Patrick Morton with the Department of Interior, from Fort Collins, Colorado
Christopher Wesley with Rogii from Highlands Ranch, Colorado
Jeff Kiernan from Denver, Colorado
Trent Reese with Ops Geos LLC., from Arvada, Colorado
Elizabeth Felts from Thorton, Colorado
John Singleton with Colorado State University, from Fort Collins, Colorado
Benjamin Baugh with Entrada Consulting Group, from Lakewood, Colorado
Robert Stewart with the University of Houston, from Houston, Texas
John Zetzman with D3 Energy Limited, from Highland Village, Texas
Meredith Greager with ECMC from Lakewood, Colorado
David Hertzke from Broomfield, Colorado
Ronald Bell with Drone Geoscience, LLC, from Lakewood, Colorado
Sydney Shortridge is a student at the University of Colorado – Boulder
Kehinde Bosikun from Cantonsville, Maryland
Spencer Musgrove with Samuel Gary Jr. & Associates, Inc. from Denver, Colorado
Bryce Grote with Timberline Oil & Gas Corp from Rapid City, South Dakota
Denny Migl with Lost Soldier Oil and Gas LLC, from Centennial, Colorado
Olivia Brow with Daniel B. Stephens & Assoociates, from Highlands Ranch, Colorado
Darren Kirkwood from Casper, Wyoming
OUTCROP ADVERTISING RATES
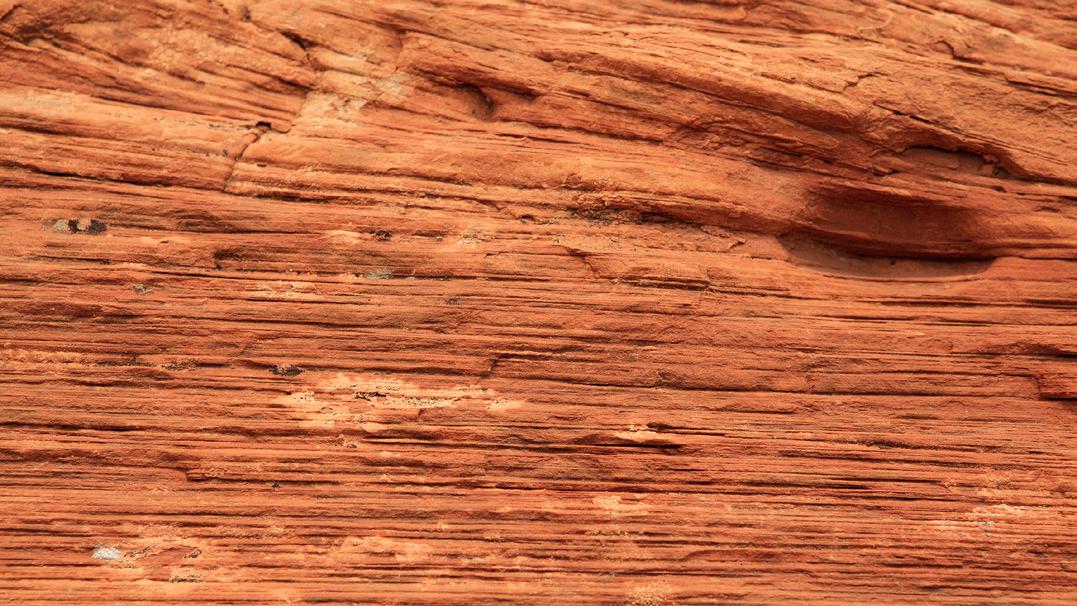
Publish with…
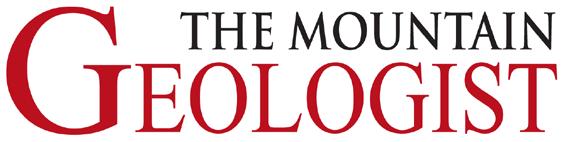
Expanded geologic focus:
• Entire greater Rocky Mountain area of North America
• West Texas and New Mexico to northern British Columbia
• Great Plains and Mid-Continent region
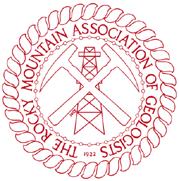
Why contribute?
• Reach a broad industry and academic audience
• Quarterly peer-reviewed journal
• Permanent archiving includes AAPG Datapages
• Quick turn-around time
• Every subdiscipline in the geosciences
Email: mgeditor@rmag.org
https://www.rmag.org/publications/the-mountain-geologist/
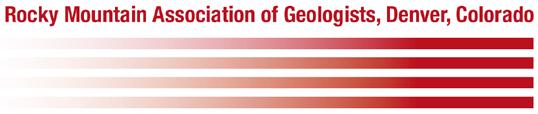
RMAG Happy Hour
RMAG Luncheon.
RMAG Coffee Hour WOGA Lean-In.
DAPL Ski Day.