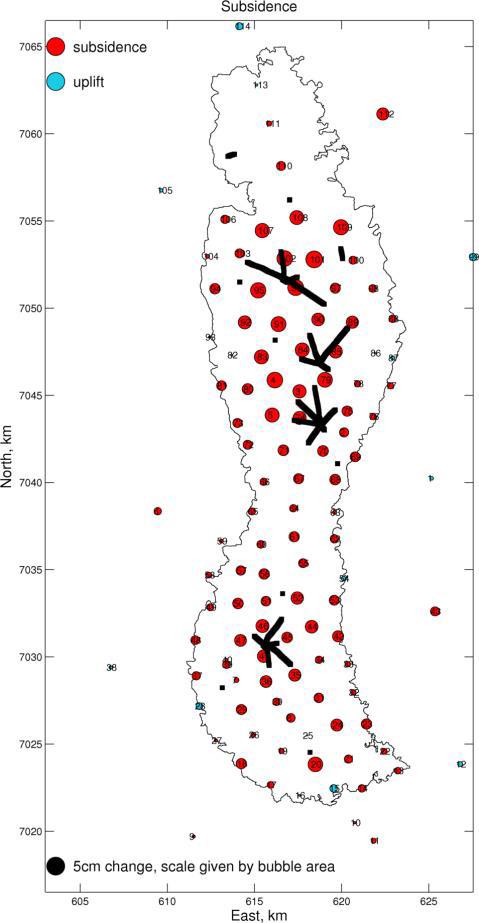
12 minute read
4D Gravity & Seafloor Subsidence Surveys for Cost-Effective Monitoring of Offshore Gas Reservoirs
Martha Lien, Hugo Ruiz, Martin Vatshelle, OCTIO AS
Introduction
Optimizing hydrocarbon recovery in offshore hydrocarbon fields involves decisions involving costly investments, like drilling infill wells or installing compression facilities. A good understanding of the dynamical behaviour of the reservoir over the lifetime of the field is of key importance to support and reduce the risk related to such investments.
Time-lapse seismic is generally accepted as a suitable monitoring technology. However, it involves considerable operational costs and it remains difficult to process 4D seismic information in a timely
manner for reservoir management decisions. Time-lapse gravity and subsidence surveys can provide complimentary information to 4D seismic in a timely manner at a cost of typically 10% of that of seismic surveys. This approach has been used on the NCS for two decades, and field cases demonstrate that 4D gravity and subsidence monitoring can sometimes provide information beyond the reach of seismic, and timely enough to guide important reservoir management decisions.
Time-lapse gravity changes at the seafloor are sensitive to fluid redistribution in the reservoir. As an example, vertical movements of water-gas contacts smaller than a meter can be detected under some circumstances (Ruiz et al., 2015). That is possible because of the high accuracy of the time-lapse gravity measurements, which is at the level of a few μGal (Agersborg et al., 2017). Seafloor subsidence monitoring uses precise water pressure measurements at the seafloor as a starting point. After processing which involves for example correcting for tides and oceanographic effects, the method reaches accuracies as low as 2 mm. Subsidence is not only a required correction for the interpretation of gravity results, but also a valuable monitoring tool by itself, as it is sensitive to important reservoir and overburden properties. The lateral development of a subsidence bowl is related to pressure depletion and lateral compartmentalization, and in some cases, it is a key factor for the safety of the installations.
The marine 4d gravimetry and subsidence method
Gravity and seafloor subsidence data are acquired simultaneously in combined surveys. A sensor frame containing three relative gravimeters and three pressure sensors is used for the measurements.
Gravity and water pressure are measured with the sensor frame at a number of lateral positions across the field. The sensor frame is placed on a semi-permanent concrete platform at the seafloor. The number of stations ranges from 20 to 120, depending on the size of the field, with station spacing being typically similar to the reservoir depth below the seabed. The top surface of the platforms is circular with a diameter of approximately 1 m, and their role is to guarantee time-lapse repeatability in the measurement location. The concrete platforms are left on the seafloor during the field lifetime and can be retrieved at the end of production. During a survey, a vessel is positioned sequentially above the concrete platforms, and a remotely operated vehicle (ROV) deploys the sensor frame to perform the 20-minute measurements on top of each of them. The duration of a survey ranges from one to five weeks.
Surveys are organized in loops starting and ending at one or two centrally-placed platforms, called base stations. Repeat measurements at the base stations are used as a reference to model instrumental drifts, by constraining the measurements at the different visits to have the same value after the correction.
Stations are located both above and surrounding the hydrocarbon field. Zero-level stations are placed in peripheral locations were production is expected to induce neither a change in gravity nor vertical seafloor deformation. That allows using those stations as a calibration reference in time-lapse computations. The survey aperture, including the offset of zero-level stations from the field outline is a function of reservoir depth.
In a subset of stations, tide gauges are deployed during the whole survey, as a means for correcting raw pressure measurements for tides and other oceanographic effects. By applying these corrections, pressure measurements obtained with the frame at the concrete platforms are converted in measurements of platform depths. Depth differences between surveys provide a measurement of subsidence with a precision of a few millimeters. Gravity changes are computed after correcting measurements for instrumental drift, tides, water density and the effect of seafloor subsidence. Gravity data is easy to integrate in history
matching workflows. This is because forward-modelling time-lapse gravity signals is an unambiguous and linear operation, involving a simple summation of Newton’s law on all reservoir cells. Contrary to seismic attributes, gravity is sensitive to density only, a scalar property without any dependence on other rock or fluid parameters.
Incorporating information from seafloor subsidence in the reservoir model can be a more involved process. The transfer function between reservoir compaction and the observed seafloor subsidence depends on properties of rocks outside the reservoir. Tools for modelling subsidence range from simple analytical models to advanced numerical simulations (van Opstal, 1974; Pettersen and Kristiansen, 2009). However, for several field applications, simple analytical modelling tools have been shown to be sufficient to provide an accurate picture of reservoir compaction or to identify non-producing compartments. See, for example Rocca et al. (2013), van Thienen-Visser et al. (2015) and the examples presented below.
Case studies
We use five case studies from the NCS to illustrate how subsidence and 4D gravity data are incorporated into reservoir management.
The first example refers to Midgard, a gas field within the sgard complex with seafloor depths between 240 and 310 m and the reservoir 2800 m below sea level. The reservoir segments in the south-east of the field feature challenging fault patterns that made seismic interpretations highly uncertain (Statoil ASA, 2015). By including information on fluid flow and reservoir compaction from 4D gravity and subsidence, it was concluded that one segment experienced less drainage than the rest of the field. The reservoir model was then updated to incorporate new sealing faults, and a decision was taken to drill a new production well in the segment. The well became the highest producing one in the sgard complex area.
Similar challenges were present at the Ormen Lange field, with initial gas reserves of 4x1011 Sm3. The seafloor depth ranges between 300 and 1,100 m, while the reservoir lies 2,600 to 2,900 m below sea level. In 2014, the discussion on near-future developments involved decisions on infill wells, installation of compression facilities, and enhanced gas recovery (van den Beukel et al., 2014). Additional information was required on compartmentalization and aquifer strengths. Feasibility studies concluded that gravity and subsidence would provide the required input earlier than time-lapse
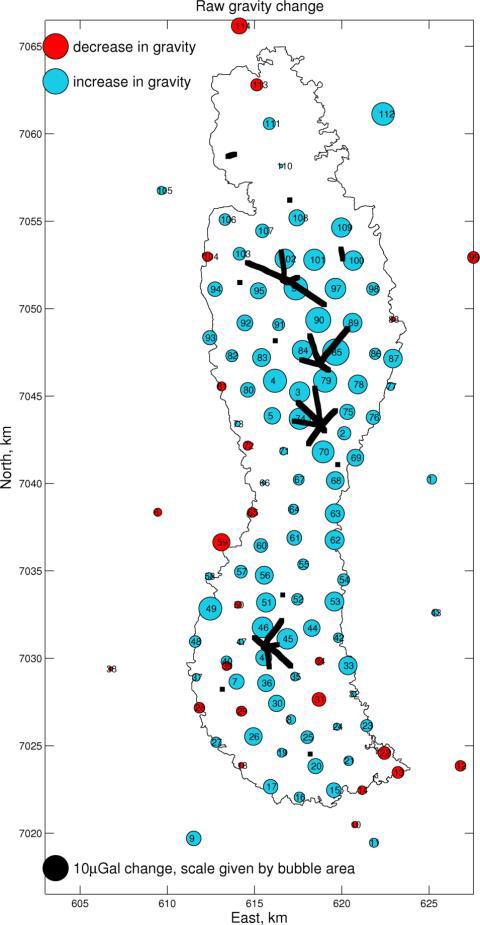
Fig 1: Changes measured in the 2012-2014 time-lapse at the Ormen Lange field: seafloor subsidence (left), and gravity changes (right) (from Vatshelle et al., 2017). The original gas-water contact is depicted as a black line, and wells as shorter, thick black lines.
Fig 2: Gravity changes corrected for seafloor subsidence in the 2012-2014 time-lapse at the Ormen Lange field (from Vatshelle et al., 2017). The original gas-water contact is depicted as a black line, and wells as shorter, thick black lines.
seismic. Thus, since 2012, full 4D gravimetric field surveys for acquiring gravity and subsidence data are carried out biannually over the field, the latest in the summer of 2018.
Figures 1 and 2 show the results of the 20122014 time-lapse. Note that signals compatible with zero are observed at the zero-level stations. For subsidence, two homogeneous and roughly independent subsidence bowls with different rates are observed. This data represents the main source of information on reservoir compaction. On the map of subsidence-corrected gravity, positive values indicate areas where the dominating effect on gravity
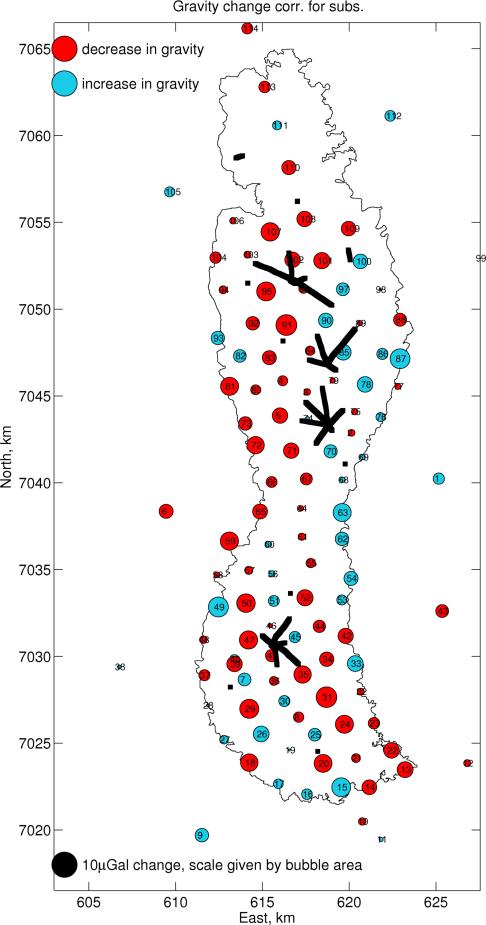
arises from water influx from the aquifer, while negative values correspond to areas where the mass reduction caused by gas production dominates.
Troll is a large field with an initial 13x1011 Sm3 of recoverable gas. Seafloor depths range between 300 and 340 m, with the reservoir lying between 1300 and 1500 m below the seafloor. Gas is produced from the thicker Troll East compartment, while both gas and oil are produced from Troll West.
At Troll East, a 1 cm/year subsidence is observed together with a clear positive signal in 4D gravity. The latter is interpreted as water influx from the aquifer and allows quantifying the aquifer strength. At Troll West, on the other hand, the descending gas-oil contact produces a smaller though significant gravity decrease signal, which provides insight in the drive mechanism and mass balance of the field. Gravity and subsidence data were used for improved material-balance calculations at Troll and to optimize the overall strategy of gas and oil production (Alnes et al., 2010).
Mikkel is a gas-condensate field with an initial estimate of reserves of 23x109 Sm3. The seafloor depth at Mikkel is 220 m, with the reservoir lying 2500 m below. It is a much smaller reservoir than that of Troll, at a much larger depth. Differences of about 4 μGal between measured time-lapse gravity data and reservoir model predictions lead to the conclusion that the water influx from the aquifer was significantly lower than expected (Vevatne et al., 2012). The information had important implications for reservoir management: it had been anticipated that in case of water breakthrough in one of the wells, the two remaining ones would not be able to sustain the minimum required flow rate in the pipeline. Updating the anticipated time for breakthrough allowed for a better management of pipeline occupation rights.
At the Statfjord oilfield, subsidence measurements were used to calibrate the geomechanical model of the overburden. From this improved geomechanical model, the discrepancies between the measured and modelled seismic time-shifts in the overburden could then be used to identify undrained segments in the reservoir (R ste and Ke, 2017).
Improving the cost efficiency of
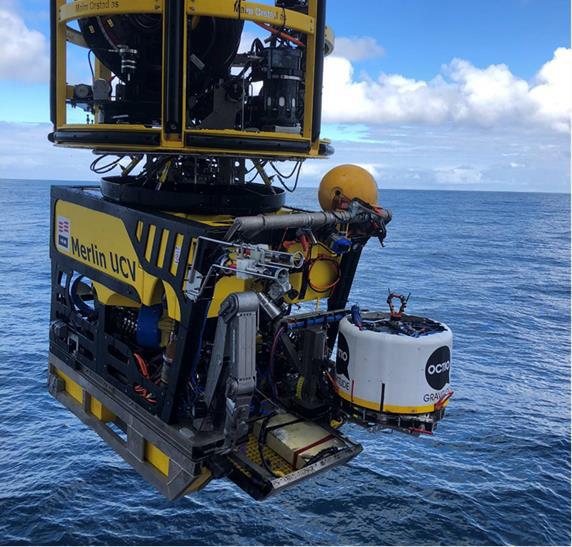
Fig 3: The new generation of gravimetry and subsidence monitoring instrumentation. The sensor frame is hold by a work-class ROV.
the surveys
A large fraction of the cost of gravimetry and subsidence surveys correspond to the vessel and the ROV. Cost-effectiveness can be introduced in two ways: by reducing the duration of the surveys and by making the instrumentation more compact, so smaller vessels and ROVs can be utilized.
An important driver of survey time is gravimeter drift. Multiple measurements need to be performed at the concrete platforms to construct an accurate drift model that can be used to correct the measurements.
A second driver is the duration of the measurements themselves, which is in turn driven by two factors. The first one is the high-frequency noise introduced by microseisms, that needs to be averaged out. The second is the initial instability of gravimeters that have been subject to tilt in the transition between concrete platforms (gravimeter recovery), that needs to be modelled and corrected for. The industry has recently introduced improvements in the gravimeters that provide higher frequency sampling, less recovery after tilt and less drift, together with a significant reduction of volume and weight of the instrumentation. A new generation of offshore gravimetry instrumentation has been introduced with new sensor technology, and much improved handling and automatization (see Figure 3). The effect on the reduction of requirements in ROV and vessel size has been realized in two surveys in 2018.
The data acquired on those surveys represent an excellent benchmark for planning for an optimal reduction of the duration of the surveys in the future.
PRESS RELEASE
ABS Consulting and Nozomi Networks Partner to Bolster Cybersecurity for Marine and Critical Infrastructure
Alliance combines each company’s extensive knowledge and expertise in operational technology (OT) and Industrial Internet of Things (IIoT) cybersecurity to solve critical challenges and combat advanced threats.
ABSG Consulting Inc. (ABS Consulting), a leading global risk management company, recently announced a strategic partnership with Nozomi Networks, the leader in OT and IoT security, to enhance ABS Consulting’s comprehensive operational technology (OT) network monitoring and managed services. This joint solution is aimed at organizations within industrial sectors such as oil and gas, power and energy, maritime, chemical and manufacturing. By combining their unparalleled knowledge and expertise in OT and Internet of Things (IoT) cybersecurity, ABS Consulting and Nozomi will help organizations meet their unique industrial cybersecurity challenges to address the ever-changing threat landscape.
ABS Consulting joins Nozomi Networks’ MSSP Elite Partners program, which enables managed security service providers (MSSPs) to build upon the existing capabilities of Nozomi Networks Vantage, Guardian and Central Management Console (CMC) products that Nozomi manages on behalf of customers. The program also gives customers confidence that MSSPs are trained, certified and available to incorporate Nozomi Networks’ solutions within their MSSP platform and/or SOC certifications. In 2021, the U.S. saw a number of high-profile cybersecurity attacks on critical infrastructure and industrial organizations. The incidents, which began in IT systems, caused the targeted organizations to proactively shut down operations to minimize damage and prevent threat actors from infiltrating OT systems. These actions not only highlighted the monumental differences in the methods used to manage OT and IT vulnerabilities, but they demonstrated the wide-spread effects on bottom lines, reputations and the public.
“These high-profile attacks on critical infrastructure demonstrate the divide between how organizations prepare for and manage the cybersecurity threats facing their OT and IT. The IT cybersecurity pace is much more advanced, while OT is an emerging target. This, combined with increased digitalization and connectivity, is creating an ever-expanding risk gap that requires specialized domain expertise to address,” said Ian Bramson, Global Head of Industrial Cybersecurity at ABS Consulting. “Through our collaboration with Nozomi Networks, our clients will now have access to OT-specific managed services, including 24/7 monitoring as well as asset and vulnerability management.”
“ABS Consulting is filling a critical role as a growing number of industrial CISOs look for cost-effective ways to strengthen their security postures across IT and OT while juggling resource limitations and skills gaps,” said Chet Namboodri, Nozomi Networks Senior Vice President of Business Development and Alliances. “With ABS Consulting on board as a member of our MSSP Elite Program, we can work more seamlessly together to deliver the Industrial IoT managed detection and threat remediation our joint customers are requiring.” Learn more about ABS Consulting’s Cybersecurity Managed Services, as well as security offerings for critical infrastructure, including the power and energy sector.
About Nozomi Networks
Nozomi Networks accelerates digital transformation by protecting the world’s critical infrastructure, industrial and government organizations from cyber threats. Our solution delivers exceptional network and asset visibility, threat detection and insights for OT and IoT environments. Customers rely on us to minimize risk and complexity while maximizing operational resilience.
About ABS Consulting
ABSG Consulting Inc. (ABS Consulting) is part of ABS Group of Companies, Inc. (www.absgroup.com) which is a wholly-owned subsidiary of ABS (www.eagle.org), one of the world’s leading marine and offshore classification societies. The subsidiary was recently named one of America’s Best Consulting Firms of 2021 by Forbes. With over 50 years of risk management and safety experience, ABS Consulting provides data-driven risk and reliability solutions and technical services that help clients confirm the integrity, quality and efficiency of critical assets and operations. Headquartered in Spring, Texas, ABS Consulting operates with more than 1,000 professionals in over 20 countries serving the marine and offshore, oil, gas and chemical, government, power and energy and industrial sectors.