02. DOMAIN
This chapter examines the challenges that Bangladesh faces due to climate change and natural disasters and explores ways to address them. It examines what strategies are already in place to manage disaster risks and how they can be improved. Particular attention is paid to how local communities can be involved in the process to make the proposed solutions more effective and sustainable in the long term. It also looks at examples of successful approaches from other countries that can be adapted to the specific context of Bangladesh to cope with increasing climate threats.
1 Get Bengal. “The Cyclone Which Became a Trigger for the Birth of a Nation.” https://www.getbengal.com/details/the-cyclone-which-became-a-triggerfor-the-birth-of-a-nation.
Fig.1. Aftermath of the Bhola Cyclone in East Pakistan, 19701
India Bangladesh
2.1 CLIMATE CHANGES
The planet is currently warming faster than at any time in human history. Rising temperatures are changing weather patterns and upsetting the normal balance of nature, creating new risks for life on Earth2. These changes have the greatest impact on the poorest countries such as Bangladesh, India, Myanmar and Thailand3. The populations of these countries will experience increasingly extreme temperature swings, changing rainfall patterns, rising sea levels and an increase in the frequency and intensity of extreme weather events4
Our study will focus on Bangladesh, which is located on the lower reaches of the Ganges, Brahmaputra and Meghna rivers, covers an area of about 1.5 million square kilometers and is home to more than 160 million people (Figure 2). Although the country accounts for only 0.56% of global carbon dioxide emissions, it remains one of the most vulnerable to the effects of climate change5
2 CH (2010). Climate Change and Child Health Case in Selected Cities of South-western Nigeria. The New York Academy of Medicine
3 Climate Security and Instability in the Bay of Bengal Region. Discussion Paper Series on Managing Global Disorder No. 13 April 2023. Sarang Shidore
4 Dutilly, C., & Hainzelin, E. (2019). Conclusion: Food system adaptation and mitigation: Managing trade-offs
5 Susmita Dasgupta et al., “Vulnerability of Bangladesh to Cyclones in a Changing Climate: Potential Damages and Adaptation Cost,” World Bank (Policy Research Paper no. 5280, April 2010)
6 The Report. “Cyclones of Bangladesh: What You Need to Know & How to Stay Safe.” https://thereport.live/bangladesh/cyclones-of--bangladesh-whatyou-need-to-know-how-to-stay-safe/30156
Bay of Bengal
BAY
Urir Char
Sandwip
Kutubia
Chakoria
Kutubia
Chakoria
Fig.2. Geographic Focus on Bangladesh6
Initiation (Step 1): The process begins over warm ocean waters, where the surface water temperature exceeds 27°C, and humidity levels are above 70%. These conditions cause the sea water to start evaporating, adding moisture to the atmosphere.
Cloud Formation (Step 2): As the warm, moist air rises, it meets colder air at higher altitudes, leading to the condensation of water vapor and the formation of clouds. This process releases latent heat, which fuels further evaporation and upward movement of air.
Cyclonic Development (Step 3): As the cycle of warm air rising and cool air descending continues, a circular wind pattern begins to form. The continuous evaporation of sea water and the rising of warm air lead to the development of organized storm systems, potentially culminating in a tropical cyclone.
2.2 NATURAL DISASTERS
Natural disasters such as cyclones and floods have long posed a serious threat to human life, infrastructure, and ecosystems in Bangladesh7. These events not only cause loss of life, but also significant economic losses and long-term problems for local communities. Our research aims to develop strategies to better manage the impacts of cyclones and floods. By studying the causes, dynamics, and impacts of these events, and analyzing existing mitigation methods, we aim to find solutions that can address current shortcomings, increase community resilience, and reduce negative environmental impacts.
2.2.1
TROPICAL CYCLONES
The coastal areas of Bangladesh are subject to some of the most powerful cyclones in the world, with 16 of the 35 deadliest cyclones in the world since 1584, each killing more than 5,000 people6. The most active region, where 10% of all tropical cyclones form, is the Bay of Bengal (Figure 3). Cyclones here are formed by the transfer of heat from the ocean and occur when sea surface temperatures rise above 27°C, which often occurs in early summer and the late monsoon season, when temperatures reach 27°C and above8 (Figure 4).
The Saffir-Simpson Hurricane Scale is used to classify these cyclones and assess potential damage worldwide, helping to design and implement protective measures9
6 Disaster Management Act, Epidemic Disease Act
7 Ali, Anwar.(1 999). Climate Change Impacts and Adaptation Assessment in Bangladesh. Climate
8 The World Bank Group. Climate Risk Country Profile: Bangladesh. 2021
9 WTVY News 4. 2021. “Hurricane Categories.” Last modified June 4, 2021. https://www.wtvy.com/2021/06/04/hurricane-categories
10 Lectures Bureau. “A Hurricane Is Indeed a Machine of Inconceivable Power.” Accessed September 15, 2024. https://www.lecturesbureau.gr/1/ hurricane-is-indeed-a-machine-of-inconceivable-power-1538/ 11 CEGIS. “Risk from Major Cyclones during 1960-2009.” 2010
Fig.3. Track of Cyclones in Bangladesh11
Fig.4. Formation of a Tropical Cyclone: A Step-byStep Process10
2.2.2 FLOODS
Bangladesh’s low elevation south of the Himalayas makes the country particularly vulnerable to flooding caused by melting glaciers and rising sea levels12. The country has a vast network of rivers, canals and estuaries that can quickly inundate inland areas (Figure 5-6). Flooding occurs in many different forms, each with its own characteristics and impacts.
Monsoon Floods: this type of flooding occurs during the monsoon season, when heavy rains cause rivers to overflow their banks and flood low-lying areas. Such flooding significantly limits the exploitation of some land in coastal areas. Water can remain in these areas for weeks, so people prefer not to build houses there, but to use the land for agriculture. However, this also has its limitations: during the rainy season, these lands are often flooded, making farming difficult. On the other hand, after the flood, these lands become more fertile, which contributes to good harvests in the future13
Flash Floods: These floods are the result of heavy rainfall or the failure of dams and embankments, causing water levels to rise rapidly12
Tidal and Coastal Flooding: These are caused by high tides, storms, and rising sea levels. They inundate coastal areas and cause damage to coastal infrastructure and ecosystems. Much of the coastal region of Bangladesh is protected by the world’s largest mangrove forest, which significantly reduces the severity of storm surges that come with cyclones. Mangroves works as a natural barrier, slowing the speed of winds and waves, which helps reduce the destructive impact of storms on coastal areas12
Each type of flood requires specific management and adaptation strategies, especially as climate change increases the frequency and intensity of these events.
12 As-Salek, JA. (1998). Coastal trapping and funnelling effects on storm surgeЦs in the Meghna estuary in relation to cyclones hitting Noakhali–Cox’s Bazar coast of Bangladesh
13 Baten, Abdul, Pedro Arcos González, and Rafael Castro Delgado. “Natural Disasters and Management Systems of Bangladesh from 1972 to 2017: Special Focus on Flood
Fig.5. Flood Situation Update - Bangladesh: Damages and Losses as of January 4, 202314
Fig.6. Flood Situation Update - Bangladesh: Damages and Losses as of January 4, 202314
2.3 DISASTER MANAGEMENT STRATEGY
Cyclones and devastating floods in Bangladesh can strike unexpectedly and vary in severity; typically, global media attention and international aid increase sharply after such disasters. For a few weeks, the situation becomes the center of global attention, and aid flows increase. However, after a few weeks, interest begins to wane, and with it, the amount of support decreases.
This temporary surge in attention and aid highlights an important issue: rebuilding the affected regions requires much more than short-term international support. Short-term interventions might indeed provide temporary relief but cannot answer the long-term concerns. Sustainable and comprehensive strategies are needed, including ongoing interaction between governments, international organizations, and local communities. Experience in other countries shows that, when residents are actively involved, more sustainable and resilient approaches can be developed that truly answer to their needs. One of the most important strategies is to develop policies that empower people through education, access to resources, and support for grassroots initiatives. In the case of Bima and Palankaraya in Indonesia, a disaster-risk-reduction programme called MASTANA, or ‘awareness’, was introduced with exactly this aim in mind. It has greatly enhanced community involvement in disaster management, thereby making people more resilient to disasters such as flooding, earthquakes, and forest fires15. Following the introduction of training, local committees, and sustainable practices, flood, earthquake, and forest-fire damage has reduced, and the number of fatalities has decreased. Only this kind of approach can ensure that people recover from disasters and are also prepared to face future crises.
15 Husein, Rahmawati. “Localizing Disaster Risk Reduction: A Case Study of Community-Based Disaster Preparedness in Bima and Palangkaraya, Indonesia.” IOP Conference Series: Earth and Environmental Science, vol. 989, University Forum for Disaster Risk Reduction Conference, September 29, 2021
Fig.7. Cyclone Disaster Management Phases: From Preparation to Rehabilitation
This diagram outlines the key phases of disaster management for cyclones, detailing the steps taken from initial preparation to longterm rehabilitation: Preparation (12-36 hours before): Involves issuing warnings and alerts to the population about an impending cyclone. Cyclone (1-2 days): The actual occurrence of the cyclone, during which evacuation of people to safer areas is crucial. Damage Assessment: Immediately after the cyclone, authorities assess the extent of damage to infrastructure, homes, and the environment. Emergency Aid (1 week - 1 month): Focuses on providing immediate relief such as food, clean water, temporary housing, and medical services to affected communities.
2.3.1 THE TRADITIONAL TOP-DOWN APPROACH
There are six main cyclone mitigation measures in Bangladesh: embankments, cyclone shelters, afforestation, early warning systems, awareness raising, and communication16 Some of these were adopted after the disastrous cyclone in Bangladesh in 1991, which killed over 138,000 people17. The guidelines adopted focused on standardized evacuation procedures, shelter construction, and resource allocation. These documents were primarily technical and came from the highest levels of government, and they were developed mainly from the top down. The highest authorities and experts created the basic rules and procedures.
2.3.2 MOVING TOWARDS COMMUNITY INVOLVEMENT
However, after Cyclone Sidr in 2007, which was the strongest in decades, it became clear that the system was losing its relevance and many outdated solutions needed to be modernized. Shelters were almost destroyed due to lack of maintenance. Early warning systems also failed to work as expected, with information not reaching people in time, especially in remote areas. The coastal zone had always been considered a “neglected zone” as described in the Fifth Five Year Plan of Bangladesh18 and this event increased the focus on these areas. Therefore, the revised strategy after this considered community needs more, tried to involve local people more in developing management strategies, and considered other approaches, both structural and non-structural. Instead of universal top-down solutions, local conditions and needs were considered. This helped to make the guidelines more tailored to specific regions.
Cyclones and devastating floods in Bangladesh can strike unexpectedly and vary in severity; An example of the successful application of this approach is establishing a network of multipurpose shelters that can be used not only as shelters during natural disasters but also as schools, health posts, and community centers during regular times. This contributed to improving infrastructure and raising the standard of living in the coastal areas16
Also, after the cyclone, emphasis was placed on developing early warning systems. This also included community engagement, and work began on training residents in emergency response and developing volunteer networks that could inform the public more effectively and promptly about impending disasters. The use of technologies such as telephone alerts has indeed played an important role in warning residents of disasters. Even if not everyone in the village has a telephone, people pass information to each other, and this method still works quite effectively. As a result, the number of people who did not have time to learn about the impending threat has significantly decreased. This led us to believe that more modern technologies can also play an important role not only in warning, but also in the rehabilitation process and community involvement.
Advanced technologies, like mixed reality (a hybrid of the physical and digital worlds), can be used to educate and involve the public in the planning of disaster preparedness, especially in high-loss areas. Interactive educational programmes can be created to better prepare residents to respond to emergencies and to teach them how to build safer, more resilient homes able to withstand devastating impacts and drastically shorten the time to return. This approach will not only improve the awareness of residents on how to act in emergency situations, but also help strengthen community interaction, because people will learn together and share experiences.
Relief Camp/Shelter
Overcrowding; Shortage of space
Inadequate facilities
Lack of sanitation
Lack of medical infrastructure
The evacuation process during a disaster unfolds as follows: residents are compelled to move to relief camps because their homes are not stable enough to withstand the impact. After enduring long periods of psychological stress from staying away from home in unsanitary conditions, they return to homes that are either partially or completely destroyed. The process of rebuilding is lengthy, often hindered by a lack of resources or money. After the exhausting effort of constructing a new home, it is ultimately prepared to be destroyed once again during the next disaster.
18
16 Islam, M Rafiqul.(2004). Water Resources Planning Organization (Bangladesh) Where Land Meets the Sea: a Profile of the Coastal Zone of Bangladesh.Dhaka, Bangladesh
17 Jia, Zheng. Cyclone Shelters and Cyclone Resilient Design in Coastal Areas of Bangladesh. Master’s thesis, Massachusetts Institute of Technology, 2010
Planning Commission. (1998). The Fifth Five-Year Plan 1997-2002. Dhaka: Planning Commission, Ministry of Planning, Government of Bangladesh.
Fig.8. Cyclone Impact and Recovery Process: From Damage to Reconstruction
The evolution of cyclone-resilient architecture in Bangladesh from 1970 to 1991 marks a shift from traditional building methods to more modern, structurally sound designs, driven by the need to withstand frequent cyclones.
Traditional Structures (Pre-1970): Before 1970, rural Bengali houses were typically constructed with bamboo and thatch, reflecting local culture but proving vulnerable to cyclone damage due to their fragile materials.
Damage Analysis and Adaptation: Repeated cyclone impacts led to a reassessment of these traditional methods. Observations revealed the structural weaknesses of bamboo and thatch, prompting efforts to reinforce frameworks, improve roofing, and secure building corners to better resist future storms.
Introduction of Modern Settlement Patterns (Post-1970): After the 1970 cyclone, new building practices emerged, emphasizing reinforced concrete columns and corrugated metal roofs to withstand severe winds. Elevated homes and public buildings became more common, reflecting a shift towards more resilient construction methods.
Transformation to Cyclone-Resilient Huts (1991): By 1991, architecture in cyclone-prone areas had further evolved to include pre-cast concrete elements and advanced roofing techniques, demonstrating a commitment to greater resilience against extreme weather.
2.3.3 THE BENEFITS OF COMMUNITY INVOLVEMENT
These examples show that integrating local experiences into disaster management makes disaster response more effective and helps develop sustainable community protection strategies. When residents participate in developing guidelines, solutions become more practical and relevant to their actual needs. Community participation helps create a sense of ownership, encouraging active participation in disaster preparedness and response. Such strategies, based on local experience, have a better chance of being sustainable, as they are built on practices that the community itself understands and supports.
Fig.9. Evolution of Cyclone-Resilient Architecture in Bangladesh: 1970 to 1991
2.3.4 TIME FRAME
However, despite all the improvements and better approaches to disaster management, the cycle of events before, during and after natural disasters in Bangladesh still faces significant challenges. Although the number of casualties has been significantly reduced compared to the first cyclones due to the measures taken, there has been a massive migration of people from the coastal areas to large cities such as Dhaka19. This is due to shortcomings in the relief and rehabilitation system. Reconstruction after natural disasters takes a long time; houses are destroyed, and roads and other infrastructure are washed away. The process of building new houses is delayed due to lack of resources and funds, and after intensive efforts to rebuild the house, it is soon destroyed again by the next disaster. The situation is further aggravated by the fact that many people refuse to evacuate. They fear that upon returning, their property and livestock will either be lost in the elements or stolen. The current strategy cannot cope with this process, which has become more frequent due to climate change, when the frequency of cyclones and their strength are becoming increasingly difficult to predict an floods inundate the area and can continue for weeks, making the situation worse. In the long term, a new strategy needs to be developed that considers the increasing pressure on houses and infrastructure. It is important to remember that barricades and isolation zones can save lives, but they do not work in the long term. Bangladesh is an agricultural country, and a more effective strategy is not to try to block disasters, but to learn to resist them.
19 Rahman, M. Hasinur, Md. Sadequr Rahman, and M. Mostafizur Rahman. Disasters in Bangladesh: Mitigation and Management
Fig.11. Formation of a Tropical Cyclone: A Step-by-Step Process10
Fig.10. Flow of Migration from Bangladesh over different period 10
2.4 STUDY AREA
Selecting appropriate research areas that are highly vulnerable to natural disasters is essential for developing effective strategies. In this regard, Bangladesh’s coastal zoning was analyzed to select the project site, focusing on flood and cyclone risks.
2.4.1
DISTRICT SCALE
Our research will focus on the Patuakhali district, located in the western coastal region of Bangladesh20. This district was chosen due to its high vulnerability to cyclonic activity and flooding, making it an ideal location for studying and implementing cyclone-resilient infrastructure. Patuakhali is divided into seven upazilas (sub-districts), with Kalapara being one of the largest located in the southern part of Patuakhali, Kalapara has a population density of 362 people per square kilometer, which is lower than the district average21. Despite its lower density, the area is highly susceptible to tropical cyclones and varying degrees of flooding due to its location and climate.
Fig.12. Cyclone Risk Area
This set of maps provides an overview of cyclone risk areas in Bangladesh, with a focus on specific regions vulnerable to cyclone impacts:
Cyclone Risk Area in Bangladesh (Figure 12): This map illustrates the regions most at risk from cyclones, highlighting areas of high risk, general risk, and specific wind risk. The southern coastal areas, including parts of the Bay of Bengal, are marked as high-risk zones due to their exposure to storm surges and high winds.
Map of Patuakhali District (Figure 13): A more focused map shows Patuakhali District, one of the regions within the high-risk zone, detailing its geographic features and proximity to the coastline, which increases its vulnerability to cyclone-related hazards.
Map of Kalapara Upazila (Figures 14-17): Zooming further in, this map provides a detailed view of Kalapara Upazila, highlighting local unions such as Lata Chapli and Dhulasar, which are highly susceptible to cyclone damage.
Fig.13. Map of Patuakhali District
Fig.14. Map of Kalapara Upazila
Fig.17. Map of Kalapara Upazila
Fig.15. Cyclone Shelter in Dhularsha Union
Fig.16. House in Dhularsha Union
2.4.2 UNION SCALE
Within Kalapara Upazila, we have selected Dhulashar Union as the specific for our project. Dhulashar Union is a newly established administrative region covering approximately 45 km² and is home to about 20,000 residents. Population are dedicated to either fishing or agricultural activities. The union’s direct exposure to destructive cyclones and storm surges makes it highly vulnerable to flooding. The limited infrastructure, with only five public shelters currently operational, highlights the urgent need for enhanced cyclone-resistant measures22
2.4.3 BIOME
The study area falls within a subtropical monsoon climate zone, characterized by moderate rainfall and four distinct seasons: pre-monsoon (March-May), monsoon (June-September), post-monsoon (October-November), and dry season (December-February). The monsoon season begins in late May, with an average rainfall of 183 mm, and continues until October, accumulating a total of 159 mm of precipitation. Peak rainfall occurs in August, reaching 445 mm, with an average annual precipitation of 1764 mm23
23
22 Haider, Mohammed Ziaul, and Md. Firoz Ahmed. Multipurpose Uses of Cyclone Shelters: Quest for Shelter Sustainability and Community Development. Economics Discipline, Khulna University, 2021
Climate Risk Country Profile: Bangladesh (2021). The World Bank Group, 2021
Fig.18. Average Monthly Mean, Max, and Min Temperatures and Rainfall in Bangladesh
Fig.19. Map of Dhulashar Union
Fig.20. Map of Cyclone Shelters in Dhulashar Union
2.5 VERNACULAR ARCHITECTURE
Statistically, the largest percentage of destruction occurs in residential buildings and infrastructure. Therefore, our proposal focuses on finding solutions to strengthen and improve these key elements within our architectural design.
2.5.1 COASTAL VILLAGES IN BANGLADESH
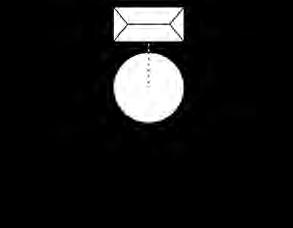
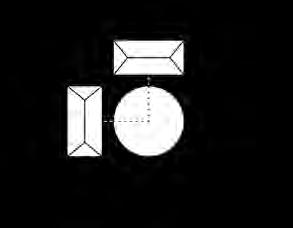
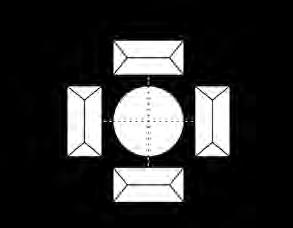
In coastal Bangladesh, village life is based on strong family and community ties. Houses are built in groups, with shared yards and spaces, forming the social fabric of the village. Over time, these groups coalesce into larger neighborhoods. The main sources of livelihood are agriculture and aquaculture, with collective fish and shrimp farming promoting efficient use of resources and strengthening community ties. A typical rural homestead in Bangladesh consists of several one- or two-room buildings (ghors) arranged around a rectangular open courtyard (uthan)24. The homestead begins with the main residential block and outbuildings. These courtyards are the central space in the house where all family members meet and spend time, and they also play an important role in ensuring good ventilation and adaptation to the climate. Buildings are erected on raised plinths to protect against flooding. Due to limited construction and ventilation technology, traditional building layouts have only one function. Such layouts do not meet the modern needs of people to live longer, more comfortably, and more conveniently. Therefore, we want to integrate and combine the functions of each building to create a new building that is in keeping with Bengali culture and use it as a basis for creating new settlements.
Village planning often takes natural protection from cyclones into account. Tall trees such as coconut palms are planted to act as wind barriers, with smaller trees closer to the houses for additional protection. Houses are often oriented to minimise exposure to prevailing winds, helping to mitigate the effects of cyclones. Important roads that connect settlements are often built on embankments. However, the main difficulties arise from the fact that they are often built on soft soils and seasonal fluctuations in the flood levels, which destroy embankments built on clay deposits. These difficulties have to do with the fact that the flooding phenomena are associated with excessive precipitation and slow compaction of the embankment material, which eventually results in the destruction of slopes and the loss of the bearing capacity of the foundation25
24 Ahmed, K. Iftekhar. “The Rural Bangladeshi Courtyard.” Department of Architecture, BUET, Dhaka 1000, Bangladesh, and Postgraduate Programs in Disaster Management, BRAC University, 66 Mohakhali C/A, Dhaka 1212, Bangladesh 25 Bhattacharyya, S. Highway Embankment Design in Bangladesh. Published December 1, 2009. Engineering, Environmental Science
Fig.21. Traditional House form in Rural Bangadesh
Fig.22. Principle of Uthan (Courtyard) Formation
Nowadays, there are two ways to reinforce the slope of an embankment: concrete blocks and traditional grass planting. Concrete blocks are subject to wear and tear, which leads to their destruction. In addition, they are not an environmentally friendly solution. At the same time, using ordinary grass does not bring the desired effect: the grass roots do not sufficiently strengthen the slope of the embankment and are often destroyed by heavy rainfall.
26 Ready4Adventure, Land Rover Defender Overland Adventure - Iceland Full Documentary, YouTube video, 50:02, September 5, 2023, https://www. youtube.com/watch?v=7oEpcMkbU8c&ab_channel=Ready4Adventure.
Fig.23. Domain Household ( foto)26
Fig.24. Domain Household
2.5.2 TRADITIONAL BAMBOO HOUSES
Traditional bamboo houses play an important role in coastal villages due to their flexibility and strength. These houses are built on bamboo frames reinforced with plaster and corrugated sheets and are placed on raised platforms or stilts to provide protection from flooding. During the dry season, the space underneath the house is used for storage27 Although these houses are stable, they are vulnerable to damage in severe weather conditions. A detailed analysis showed how these structures behave under different levels of cyclonic activity:
Deep depression: Wind speeds of 31-50 km/h. These storms cause minimal damage, affecting mainly poorly connected parts of the structure. The analysis showed small displacements, indicating the need to strengthen the connection points between bamboo elements.
Cyclonic storm: Wind speeds of 51-100 km/h. With stronger winds, these storms can cause moderate damage, especially to roofs and unattached metal sheets. The analysis showed significant structural displacements, suggesting the need to strengthen the structure with additional cross beams and stronger materials29
Severe cyclonic storm: Wind speeds of 101-165 km/h. These storms cause significant damage, with a high risk of collapse. The analysis showed that such structures are likely to completely collapse unless reinforced with more robust construction methods and materials22.
Very Severe and Super Cyclonic Storm: Wind speeds above 166 km/h. Extreme weather conditions cause widespread destruction, uprooting trees and damaging even the strongest structures. In such cases, bamboo houses will be severely damaged and will hardly withstand the destructive force of the cyclone27
27 Alam, M. R., A. B. M. Amrul Kaish, M. F. M. Zain, and Sadia Mahzabin. “Vulnerability Assessment and Construction Recommendations of Local Houses in the Cyclone Prone Coastal Areas of Bangladesh.” International Journal of Disaster Risk Reduction 21 (November 2016)
28 Guadua Bamboo, Joining Bamboo: Tips and Tricks, accessed September 20, 2024, https://www.guaduabamboo.com/blog/joining-bamboo
29 Ankush Agarwal, Cyclone Resistant Building Architecture (United Nations Development Programme and National Disaster Management Authority, India, 2007
Fig.25. Traditional Bamboo House
Fig.26. Structural Joint Design for Bamboo Architecture28
By Finite Element Analysis, the displacement of conventional building structures facing strong wind environments can be observed. This analysis highlights the importance of making buildings more resilient and comfortable so that people can stay in their homes during mild cyclones and have the ability and knowledge to rebuild them if they are damaged quickly, using local materials and understanding of how to restore their homes.
30 Architectural Review, Building with Bamboo: Trans-Himalayan Practices, accessed September 20, 2024, https://www.architectural-review.com/ buildings/bamboo/building-with-bamboo-trans-himalayan-practices
Fig.27. Traditional Bamboo-Stilt House in Rural Community30
Fig.28. Elevated Bamboo Structures in Rural Settlementmunity30
Fig.30. Bamboo Stilt House in a Rural Setting30
Fig.29. Traditional Stilt Bamboo House with Family in Rural Community30
Fig.31. Finite Element Analysis
Cyclonic Storm
Deep Depression
Severe Cyclonic Storm
2.5 MATERIAL
In the context of Bangladesh, where external assistance is limited, we decided to focus on using local resources. Our goal is to find ways to actively involve local communities in the implementation of the project and to make the most of the materials available in the region. We understand that in the context of frequent natural and economic challenges, such as resource scarcity and infrastructure constraints, it is important to rely on affordable and sustainable solutions. We considered their availability, environmental friendliness and ability to work in the local environment to ensure the best outcome.
We selected the materials based on the two main climate challenges facing our project: floods and cyclones. We initially focused on finding sustainable alternatives to cement blocks, which are often used to strengthen embankments, but which deteriorate over time and cause harm to the environment. The next step is to select materials that residents can use in their construction. These materials must not only be durable, but also resistant to the harsh weather conditions that are typical in the region.
Fig.32.Building Materilas in Bangladesh
2.5.1 BIO-BASED CONSTRUCTION MATERIAL
When selecting the components for our Bio-Based material, we were guided by several important criteria to define components:
1. Local Availability: We aimed to use materials that are easily accessible in the region. This helps reduce transportation costs and support the sustainable use of local resources, which also contributes to the economic development of local communities. 2. Stabilization of the Structure: Ensuring strength and stability was an important condition. We were looking for materials that would help maintain the integrity of the elements, preventing them from cracking and warping.
3. Biodegradability: An important aspect was that the materials could decompose under the influence of water over time.
4. Resistance to Moisture: But despite the ability to decompose, it was also important to us that the material system did not deteriorate very quickly.
Before choosing the right components for our material system, we conducted a research to find out which ones could be used to create biodegradable building elements. The basis for our choice was a study called “CoRncrete: A corn starch-based building material “31. It provided a clear idea of how starch could be an effective component for creating bricks that decompose over time. Starch is a common biopolymer that accumulates in plants and is a cheap and eco-friendly material. It can be obtained from renewable sources, which makes it attractive for various applications in both the food and non-food industries. Starch has long been used as a replacement for petrochemical components in plastics, detergents and adhesives32. However, the process of extracting starch from corn turned out to be quite complex and labor-intensive. Therefore, we decided to use potato starch, which is more readily available and easier to process, as the main binder for our project. Its use can bolster local agriculture, providing economic benefits to communities while ensuring a sustainable supply of materials.
In addition to potato starch, we will use clay and sand, both of which are readily available in the region. Clay offers excellent plasticity, aiding in structural stabilization and enhancing the moisture resistance of the bricks. Sand, on the other hand, strengthens the structure, preventing cracks and deformations. Together, these materials will ensure the production of durable and environmentally friendly bricks, capable of withstanding the region’s climatic conditions while effectively fulfilling construction needs.
31 Kulshreshtha, Y., E. Schlangen, H.M. Jonkers, P.J. Vardon, and L.A. van Paassen. 2017. “CoRncrete: A Corn Starch Based Building Material.” Geo-Engineering Section, Faculty of Civil Engineering & Geosciences, Delft University of Technology, 2628CN Delft, The Netherlands
32 Starch Europe Association. <http://www.starch.eu/starch/> (accessed on 25th June, 2015)
Fig.33.Starch Sand Mixture
2.5.2 CONSTRUCTION MATERIALS
Bamboo has become a popular and valuable building material in Bangladesh, with more than seventy percent of the population using bamboo for construction purpose for which it offers a sustainable and cost-effective solution. A bamboo cane has high resistance to tension, especially in its external layer. This layer can bear up to 40 kN/cm² (=400 N/mm² = 400 MPa), reaching the resistance of steel33 .Compare with wood, bamboo’s ability to regenerate quickly makes it a sustainable resource. Researchers state that bamboo can be sustainably harvested every 3-5 years without causing deforestation, promoting ecological balance, and reducing environmental impact29. The structural composition of a bamboo culm provides numerous possibilities for its utilization. The thickness of the culm wall varies significantly between genera and species. In Bangladesh, species such as Bambusa Balcooa is highly esteemed for their thick-walled, dense culms, with specific gravities ranging from 0.65 to 0.80, translating into exceptional compressive and tensile strengths essential for structural stability in construction projects. To better understand the material properties of this bamboo species, we collected the following data:
It is shown that this species is widely available in Bangladesh and grows rapidly. Its excellent mechanical strength performance and high durability make it the preferred choice of bamboo species for our project.
33 Walter Liese, Michael Köhl, Bamboo: the plants and its uses, (Switzerland: Springer Cham, 2015
Fig.34.Comparison of Bamboo and Timber: Carbon Capture, Harvest Cycle, and Material
Fig.35.Bamboo Species Comparison: Bambusa Balcooa, Dendrocalamus Strictus, and Dendrocalamus Giganteus
Treatment
When utilizing a straight bamboo piece for construction, meticulous attention to drying and curing processes becomes paramount. Bamboo contains a substantial amount of starch, making it vulnerable to insect infestation, particularly when sap levels are high. Additionally, moisture can lead to the growth of fungi and lichens34. Proper drying reduces moisture content, enhancing structural integrity, stability, and resistance to deformation and fungal decay. Curing methods, such as chemical treatments with borax-boric acid solutions or thermal techniques like flaming or smoking, are crucial for protecting bamboo against pests and ensuring long-term durability. These procedures collectively contribute to maintaining bamboo’s strength and stability throughout its lifespan, making it well-suited for robust structural applications. By rigorously adhering to these practices, the performance and longevity of bamboo sections in construction can be significantly optimized.
Fig.36.Bamboo Growth and Harvest Cycle: Carbon Capture and Material Extraction
Fig.37.Bamboo Treatment Process
34 Walter Liese, Michael Köhl, Bamboo: the plants and its uses, (Switzerland: Springer Cham, 2015
2.6 CASE STUDY
In selecting case studies, we prioritised projects that propose sustainable architectural and design solutions and the use of new technologies in the design and construction process. The projects highlighted in our case studies are design initiatives that innovate within these themes, and provide information on their application, advantages and disadvantages. We aim to analyse these case studies better in order to understand their relevance to architectural sustainability and resilience, and to consider how they can shape future design strategies.
2.6.1 DELTEC HOMES
Location: Florida, USA
Design by: Deltec Homes
Deltec Homes are innovative hurricane-resistant housing built mostly in Florida. These homes have a circular footprint and a radial truss system that distributes wind loads from a central hub to a pivotal point. Deltec Homes have an aerodynamic form that minimises pressure on any one part of the structure, with the circular shape dispersing wind pressure equally among the building. The homes have large suspension trusses and continuous metal straps that connect the roof, walls and foundation, so that wind forces are directed straight into the ground rather than merely pulling on walls35
Deltec Homes’ truss system is built with a series of large, pre-engineered components that radiate out from a single hub in an arrangement analogous to a wheel’s spokes. This force distribution directs destruction evenly outwards from the structure, distributing stress among most of the entire home. The continuous metal straps are located at the very perimeter of the tower, which is the most susceptible to conflicts such as roof uplift or wall failures under high-wind conditions. Moreover, under most circumstances, the strength and stability of Deltec Homes is further enhanced by the reinforcement offered by their reinforced concrete piers or deep foundation systems, which form the foundation that secures the building in the ground – resisting problems such as storm surge or flooding.
For our project, a key insight from the analysis of the Deltec Homes design was the realisation that the aerodynamic shape of the building significantly reduces wind loads by distributing them evenly throughout the structure, while a critical aspect of stability is the secure attachment of the roof to the walls and foundations. This approach is supported by our results from the Finite Element Analysis (FEA) conducted during the study of traditional coastal house architecture in Bangladesh. The use of continuous metal ties, as in Deltec Homes, or similar solutions made from local materials, can significantly improve the resilience of buildings to high winds and reduce the risk of structural damage. Despite these advanced features, the main drawback of the Deltec Homes design is the high cost of materials and manufacturing, which makes it difficult to widely use in our project. The use of traditional, locally available materials remains an important aspect of our region and the concept, which focuses on sustainability and affordability of solutions. Therefore, adapting such principles, such as aerodynamic shape and strong connections of structural elements, to local conditions and resources seems to be the most rational approach to increase the resilience of houses to extreme weather conditions while minimizing costs.
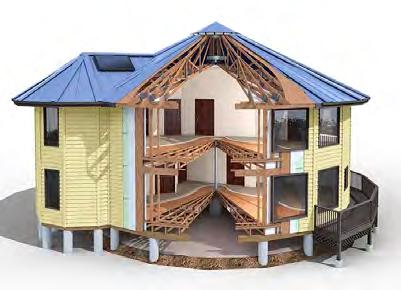
Fig.38.Construction and Design of Hurricane-Resistant Circular Houses (Deltec Homes)35
2.6.2 KHUDI BARI
Location: Bangladesh
Design by: Marina Tabassum Architects (MTA)
Khudi Bari designed by Marina Tabassum Architects (MTA)is a modular solution for floodprone areas of Bangladesh using lighweight, locally available materials including bamboo, steel connections and corrugated metal. The unit can be erected and dismantled quickly, and is adaptable to seasonal flooding (an essential imperative in these areas)36. Bamboo used for its lightness and available locally, is further reinforced with steel connections, elevated off the ground and is retained with the raised platform to cope with flood waters. However, a lightweight design can be disadvantageous particularly in high winds, which is why this is a critical consideration in coastal areas that experience cyclone loads. To enhance the wind resistance, additional bracing or stronger materials and construction types can be considered. Carrying out solutions such as reinforcement with wind ties or integration with local construction methods will increase a buildings’ resilience to high winds, while maintaining their flood adaptive management capability. This is an important aspect of our approach to creating resilient and affordable housing is effectively addressing the multiscale climate challenges that characterize the coastal areas of Bangladesh.
36 Marina Tabassum Architects. Projects.. https://www.marinatabassum.com/projects
Fig.39.Bamboo-Based Elevated Khudi Bari Home36
2.6.3 STEAMPUNK PAVILION
Location: United Kingdom
Design by: Gwyllim Jahn, Cameron Newnham, Soomeen Hahm, and Igor Pantic
The Steampunk Pavilion, a mixed reality (MR) experience developed by the collective of Gwyllim Jahn, Cameron Newnham, Soomeen Hahm and Igor Pantic, features a kinetic fádé and interior that users can manipulate in real time through MR interfaces, altering the spatial configuration and visual effects, thereby creating an immersive and responsive environment37
Its technical side includes the application of the most advanced MR technologies based on the use of sensors, actuators and interactive display systems, all made of lightweight and durable materials, which enable the appearance and spatial configuration of the pavilion to be changed in real time.
Despite the innovative nature of the approach, the high cost and technical complexity of MR technologies represent barriers to their widespread implementation. However, given that the technology does not require highly skilled specialists and is relatively easy to use, it represents a promising solution for implementation in educational programs. This will increase the involvement and responsibility of the local community in the construction process, as well as promote self-sufficiency and active participation of residents.
37 Steampunk Pavilion.” https://soomeenhahm.com/portfolio-item/steampunk-pavilion/
Fig.40.Steampunk Pavilion. Project Logic37
2.6.4 BISHAN-ANG MO KIO PARK
Location: Singapore
Design by: Ramboll Studio Dreiseitl
Bishan Ang Mo Kio Park in Singapore exemplifies urban design solutions that optimize people’s relationships with nature while also reducing flood risk. Initiated by the Singapore government’s Active, Beautiful, Clean Waters program, the project transformed an old concrete channel into a natural river, significantly improving stormwater management and promoting ecological restoration. The floodplain solution the designers implemented increases the river’s capacity by 40 percent, allowing all that water to be managed, ultimately reducing the likelihood of flooding38
Bioengineering techniques were also used to strengthen the riverbanks and native plants were planted, supporting biodiversity. The river now has a meandering course, improving its ecological functions and creating a habitat for wildlife. The park’s layout considers the local topography and hydrology, with low-lying areas acting as temporary reservoirs to store excess water. This slows its flow and allows it to gradually soak into the ground.
Although the project is successful in preventing floods and helping to restore ecosystems, regular maintenance is required to maintain its effectiveness. We plan to use the principles of this approach in our project, including the introduction of green spaces and landscape solutions such as water storage tanks and channels for its distribution. This will help us manage water resources more effectively and increase resilience to floods.
38
Mo Kio Park.” Designed by Ramboll Studio Dreiseitl. Accessed September 12, 2024. https://www.dreiseitlconsulting.com/bishan-ang-mokio-park
Bishan-Ang
Fig.41.Bishan ang Mo Kio ParK in singaPore38
2.6.5 REFLECTION
Each of the projects reviewed makes a valuable contribution to the discussion on sustainable and resilient architecture and highlights important gaps and challenges. Deltec Homes has demonstrated how aerodynamic design and robust structural elements can make an important contribution to making homes resilient to very high winds. However, the high cost of materials and technology limits widespread adoption. Meanwhile, while Khudi Bari’s modular solution has been an effective solution to a specific flood adaptation problem using lightweight, locally available materials, it still requires additional structural reinforcement to achieve high wind resistance. The Steampunk Pavilion demonstrates how mixed reality technologies can create dynamic, interactive architectural environments. But the cost and technical complexity of these technologies means that they are not readily available for mass use. Bishan-Ang Mo Kio Park integrates natural elements into urban infrastructure to manage rainwater and enhance biodiversity. However, regular maintenance is required to ensure its effectiveness. Combining the strengths of these projects can be a starting point for updated, more sustainable, cost-effective and durable solutions for our offering.
A combined approach that combines the aerodynamic efficiency of Deltec Homes with the modular adaptability of Khudi Bari and the advanced technologies of Steampunk Pavilion can yield ideas that are resilient in the face of disasters and cost-effective. Complementing these approaches with the green infrastructure strategies of the Bishan-Ang Mo Kio Park project – water management and other systems – can further optimize these solutions for sustainability and efficiency. Such an integrated approach can likely provide better solutions to multiple climate challenges while increasing community participation in the construction process.
Taking inspiration from the strengths of these projects offers a starting point for renewed, more sustainable, cost-effective and resilient housing solutions. A combined approach that marries Deltec Homes’ aerodynamic efficiency with Khudi Bari’s modular adaptability and Steampunk Pavilion’s advanced technologies could yield resilient, cost-effective and high-tech buildings. Matching these approaches with the green infrastructure strategies of the Bishan-Ang Mo Kio Park project – water management and other systems – can further optimise these solutions for sustainability and efficacy. Such an integrated approach can likely provide better solutions for multiple climate challenges while concomitantly increasing community engagement in the construction process.
03. METHODOLOGY
This chapter presents the methods and tools used in our research, analysis, and design. These methods are essential for analyzing data, obtaining experimental results, and planning the next steps in the design process. A key part of our research is to develop a fail-safe and fast assembly system and methodology designed for the construction of buildings in coastal regions. This study employs a combination of methods from different fields to create tests and document experiments to assess the rationality and effectiveness of the design.
3.1 FINITE ELEMENT ANALYSIS (FEA)
Finite Element Analysis (FEA) is a crucial numerical analysis method in architectural structural engineering that enables comprehensive evaluation and prediction of a building’s performance under various loading conditions. In this project, FEA will be used to analyze and optimize the structural system design to meet the challenges posed by cyclones in Bangladesh43.
We employ Karamba3D, a robust FEA software plugin specifically tailored for architectural structural analysis and design. This tool integrates seamlessly with modeling platforms like Rhino and Grasshopper, providing a powerful suite of features for structural optimization, parametric design, and automated workflows. This integration enhances both efficiency and precision in the structural analysis and design process.
3.2 COMPUTATIONAL FLUID DYNAMICS (CFD)
Computational Fluid Dynamics (CFD) is a key tool in analyzing aerodynamic resistance and fluid flow structures, which is crucial for enhancing the resilience and safety of architectural designs in cycloneprone areas.
In this project, Autodesk CFD will be employed to analyze aerodynamic resistance and airflow patterns. This will assist designers in reducing wind resistance and pressure, thereby improving the resilience and safety of structures39. Autodesk CFD allows for precise simulation of wind behavior around various architectural forms, enabling the optimization of building designs for wind resistance.
Additionally, Blender will be used to simulate water flow dynamics to understand how different canal designs interact with water forces. This helps in comprehending the behavior of water flow and its impact on various canal configurations.
By integrating Autodesk CFD and Blender, we can conduct a comprehensive analysis of how different shapes and contours influence both wind and water dynamics.
39
Fig.42. Finite Element Analysis
Fig.43. Computational Fluid Dynamics
3.3 AGENT-BASED MODEL (ABM)
Agent-based model (ABM) simulates the actions and interactions of autonomous agents to understand system behavior, integrating concepts from game theory, complex systems, and computational sociology. This ABM system aims to identify the most efficient and shortest water flow paths across the site while avoiding critical locations. By defining topological optimization methods for the terrain under various conditions, including agents’ starting positions and the urban layout, ABM provides personalized solutions for community water management.
3.4 MULTI-OBJECTIVE OPTIMIZATION GENETIC ALGORITHM
The multi-objective optimization genetic algorithm is instrumental in achieving optimal solutions that meet multiple design objectives40 Unlike single-objective optimization, this algorithm offers a variety of selection strategies to address different scenarios and target solutions. Utilizing Wallacei, for multi-objective optimization genetic algorithms integrated with Grasshopper, we can manage the entire optimization process. This includes defining gene ranges, setting genetic rules, and determining mutation probabilities to optimize the phenotype41. The analysis tools provided by Wallacei allow us to assess the performance of the design phenotype and refine the gene ranges further to meet the design objectives effectively.
3.5 MIXED REALITY (MR)
Mixed Reality is revolutionizing architecture by seamlessly integrating physical and digital environments, offering significant advantages for post-typhoon recovery and repair. Platforms like Meta Quest 3 provide immersive visualization, enhancing the understanding of spatial relationships and structural details, which is crucial for assessing damage and planning repairs47. In construction, MR merges digital models with real-world sites, utilizing holographic models to deliver precise visual feedback and improve template accuracy . This capability is especially beneficial for rapid post-cyclone repairs, as MR facilitates efficient damage assessment and supports dynamic adjustments to repair strategies. Additionally, MR aids in quickly guiding and training repair teams, reducing the requirement for high skill levels and enhancing overall efficiency in repair operations .
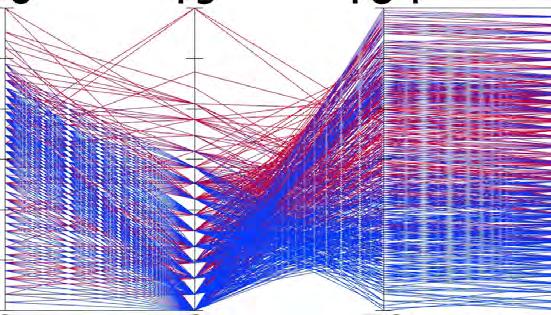
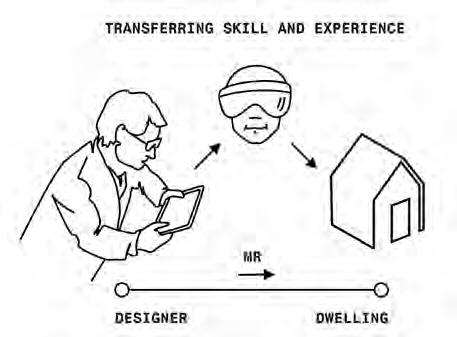
40 Deb, Kalyanmoy. 2015. “Multi-Objective Evolutionary Algorithms. Springer Handbook of Computational Inteligence, 995- 101
41 Showkatbakhsh, Milad, and Mohammed Makki. 2022. “Multi-Objective Optimisation of Urban Form: A Framework for Selecting the Optimal Solution
Fig.44. Agent Based Model
Fig.45. Multi-objective Optimization Genetic Algorithm
Fig.46. Mixed Reality
04. RESEARCH DEVELOPMENT
In order to inform our design approach to mitigate flood and wind risks, we conducted a series of tests based on the findings of the “Domain” chapter on vulnerabilities.
This section aims to explore, analyze, and synthesize the information that will be used to develop a sustainable and practical design. Four primary categories of study have been identified: Site Analysis, Adaptive Water Management, Material Testing, and Wind-Resistant Urban Planning. These tests will be undertaken to study unique site challenges such as flat terrain, limited natural drainage, and vulnerability to extreme weather events. Each category provides essential data and evidence that inform the proposed design solutions. The results will help establish the design solution undertaken at the design development stage.
Fig.47. Strategy
4.1 SITE ANALYSIS AND INTERVENTION SELECTION
The following section will present a comprehensive site analysis and intervention selection, being the first step in our design process. Based on the defined design directions, this chapter focuses on the defining criteria of vulnerability due to natural hazards and on the definition of the most suitable place for interventions to implement our flood mitigation strategies.
Based on the earlier analysis, one of the most vulnerable areas was chosen for the work - Kalapara in the Patuazali region. To select a site for our project, we identified key criteria affecting vulnerability to natural disasters: elevation, slope, proximity to shelters, distance from water bodies, and population density. They are vital for assessing the area’s risk and prioritizing where new proposals are needed.
Fig.48. Pixelated Vulnerability Map
Fig.49. Pixelated Elevation Map
Fig.50. Maps of Key Criteria
The Kalapara area was mapped using 100 m x 100 m grid maps, pixelated according to these criteria. The highest weight was given to elevation since, in the context of frequent floods and the threat of sea level rise, this factor is critical to reducing the risk of flooding and the long-term safety of residents. We chose distance from shelters as the second most important factor since it allows us to highlight areas where new protective measures are most needed. It was also essential to consider population density since the most vulnerable groups live in the most densely populated areas. Applying this set of criteria enabled us to draw up a list of targets of opportunity in which a balanced selection of criteria would minimize risk and maximize the effectiveness of protection measures related to a given intervention zone.
Fig.51. Map of Cyclone Shelters in Dhulashar Union
In analyzing Zone A, we sought to find the most suitable boundaries for further development, taking into account all possible natural risks. The area is located next to a river and is subject to regular flooding and strong winds due to frequent cyclones. Although coastal dikes provide some protection from storms, historical data shows that water levels in recent years have reached 2 meters, and even up to 4 meters in the last 50 years. This poses a serious risk to long-term construction projects and requires that this limitation be considered at all stages of planning.
For a more detailed analysis, we used historical flood and rainfall data to create a flood zone map. We also considered the terrain and distance from the nearest settlements. These factors helped us to identify areas where the risk of flooding is lower, but it is impossible to completely exclude the possibility of emergency situations. Even in the selected safe areas, problems may arise during extreme weather conditions, which remains a significant limitation of the project.
Another important criterion was the distance from roads and existing settlements, in order to facilitate access to the site and its subsequent development. Ultimately, the site marked on the map was selected, which, in our opinion, best combines accessibility, safety and resilience to natural disasters.
Fig.52. Flood Zone Map
Fig.53. Selected Area Map
Fig.54. Pixelated Elevation Map
4.2 ADAPTIVE WATER MANAGEMENT
Based on the site analysis, this chapter examines the critical vulnerabilities identified in Site A, including exposure to flooding and high winds from cyclones. Existing flood management approaches that have been studied previously often ignore local conditions and needs and their strategy works from the top down. Therefore, we propose to adapt the sponge city idea that we studied based on the Bishan Ang Mo Kio Park case study in Singapore, which combines water storage, distribution and absorption tailored to the specific site conditions. This involves creating ponds for holding water to reduce the effects of heavy rainfall, creating distribution channels or pathways where water can be controlled and distributed easily, and introducing terracing to increase the absorption of water and prevent soil erosion.
This approach follows the limitation of the previous discussion: natural drainage is hardly able to take place on flat terrain; hence, ponds and channels would be necessary for handling the water. Besides, the poor development of the embankment system requires a design that limits the diffusion of floodwaters as far as possible, minimizing the soil’s degradation processes. Our interventions have thus been designed to neutralize these onsite limitations and to make the local environment more resilient.
Fig.55. Spoonge City Strategy Diagram
Step 1: Water Storage
We calculated the volume of land that would be flooded at the selected site under the current rainfall conditions and considered possible future climate changes. Based on these calculations, we determined the required volume of water that needs to be distributed across the site for efficient water management.
In the first stage, we propose the creation of water storage ponds. The ponds are sized to accommodate the expected water volumes and can be used by residents for fishing and shrimp farming during normal times, adding socio-economic benefits to the project.
Fig.56. Spoonge City Strategy Diagram
Fig.57. Strategy Stage
Step 2: Water Distribution
The second stage involves the design of distribution channels to prevent the ponds from overflowing and to ensure uniform water distribution. The main goal is to reduce water flow velocity, increase infiltration, and minimize excavation work. We conducted a series of experiments to study the effects of meander angles, branch shapes, and number of branches on water velocity. Using computational fluid dynamics (CFD) and Blender simulations, we evaluated different methods of integrating channels into the drainage system.
We conducted six sets of single-variable experiments to elucidate the hydraulic principles governing our drainage system. Each experiment focused on isolating the effect of a specific variable:
Number of branches: The total number of branches in the drainage network
Number of branches at mouth: The number of branches converging at the mouth of the river
Branch position: The placement of branches along the main channel
Branch degree: The degree of curvature of each branch
Branch channel width: The width of each branch channel
Sincerity angle: The degree of sinuosity of the channel
The tests show that increasing the number of bends and branches of the channels dissipates the energy of the water flow, while minimizing the resolution of the banks. The choice of the final option was influenced by the fact that it was important for us to minimize the amount of excavation work and not allow a large division of the land into small areas. Therefore, for further work, the option with a meander shape was chosen.
Fig.58. Setting For Canals Simulation
Fig.59. Canals Simulation
Fig.60. Strategy Stage
Step 3: Water Absorption
Water absorption will be the third intervention through terracing around ponds and canals. We defined the slope, the radius necessary for the channels’ curvature (ranging from 3 to 5 meters), and other soil conditions, such as the clay and silt composition, which could give us the optimal distance between these terraces (spaced approximately 4-6 meters apart). Knowing these parameters ensures the terraces are better designed to improve the capacity of water absorption, reduce erosion, and stabilize the soil structure. The slope data (2-5%) helps to ascertain the appropriate gradient for the flow of water, while the type and condition of the soil guide the necessary radius for channel curvature (1.5 to 3 meters) to avoid excessive velocity of water and mitigate erosion risks. This will be useful in forming the basis of proposing a detailed design in the next chapter
However, to further prevent erosion of the newly created terraces, adequate reinforcement is essential to strengthen these structures against the ongoing impact of water flow and soil movement over time.
Fig.61. Strategy Stage
Fig.62. Terracing Design Parameters
Previously, in the materials section of the Domain chapter, we identified the key components for our building material. In this step, we plan to test how different amounts of water and starch, chosen as the main components, will affect the properties of the material. Our goal is to find the optimal ratio of these elements that will provide the following important characteristics:
1. Fast production of the material
2. Biodegradability
3. Ability to maintain structural integrity over time
4. Resistance to stress and external influences
Part of our approach also involves strengthening the embankments through the use of green tissue, in particular vetiver grass. Vetiver is a plant with a strong root system, whose roots can reach up to 3 meters in length in just one year. This plant can effectively prevent soil erosion, reducing it by 70%. However, the method of using vetiver has its limitations. The grass takes time to root and grow, and at the initial stage it is vulnerable to washing away in conditions of heavy rain or flooding.
To address this issue, the embankment will be temporarily supported by biodegradable bricks made from potato starch. These bricks will decompose over time without harming the environment and will give way to vegetation once the vetiver has sufficiently strengthened the soil. Using biodegradable bricks is also a more environmentally friendly solution compared to conventional concrete blocks, which can be destroyed by water and cause further damage to the soil and ecosystem.
Since a local material was chosen, it was important to calculate the amount of potatoes needed to produce the required amount of starch. An experiment showed that 80 grams of starch can be extracted from 1 kg of potatoes. This data will be used to plan the agricultural processes needed to produce the materials that will support the embankment until the plants are fully established.
Fig.63. Сriteria for Selection of Materials
Fig.64. Tests on Constituent Components
The first test was aimed at determining the optimal amount of water required to obtain a strong and durable material.
Aim: Find the optimal volume of water for the mixture so that the material remains strong, while the baking process is fast enough at 180°C.
Objective: Study how different volumes of water affect the strength of the material after baking. Find the ideal amount of water at which the brick will be stable and the drying time will be minimal. Analyze how the weight of the bricks changes after baking and whether they retain their strength.
Methodology: Three options for the amount of water were selected for the experiment: 10 ml, 30 ml and 50 ml, which were added to the starch-based mixture. The initial weight of each workpiece was 229 grams. The mixture with each volume of water was thoroughly mixed. The samples were baked at 180°C. The baking time was recorded for each sample. After baking, the weight of each brick was measured to assess moisture loss and strength.
Observations: The sample with 10 ml of water was too dry and difficult to form. The baking time was 3 hours, the final weight was 215 grams. Despite this, the structure was brittle and had cracks. The sample with 50 ml of water was too wet, which increased the baking time to 6 hours. The final weight was 205 grams, but the structure was unstable and had cracks. The sample with 30 ml of water showed the best result. The baking time was 4 hours, the final weight was 210 grams. This option provided good strength and drying speed with minimal defects.
The sample with 30 ml of water will be selected for further testing, as it demonstrated the best combination of strength and drying time. This amount of water, which is about 13% of the total weight of the mixture (229 grams), provides an ideal balance between the necessary moisture for forming the material and its ability to dry quickly, while maintaining strength.
Fig.65. Сriteria for Selection of Materials
Fig.66. Water Test 50 ml
Fig.67. Water Test 30 ml
Fig.68. Water Test 10 ml
The second test is aimed at studying the required amount of starch to ensure the integrity and strength of the brick.
Aim: To determine the optimal amount of starch in the mixture so that the brick is strong and durable enough, but still retains its biodegradability.
Objective: To study how different amounts of starch affect the rigidity and strength of the brick. Find the ideal amount of starch at which the material maintains its structure and resistance to external influences, without decomposing too quickly. To evaluate how bricks with different concentrations of starch behave under conditions of exposure to water.
Fig.69. Сriteria for Visual Evaluation
Methodology: Six bricks were tested for the experiment, each containing a different amount of starch. Each brick was formed with the addition of different amounts of starch, ranging from a minimum (3 grams) to a maximum (36 grams). The bricks were tested for rigidity and stability. Each brick was also placed in water for 24 hours to test its resistance to decomposition.
Fig.70. Comparison of Received Tests
Observations: Bricks with low starch content (less than 3 grams) were too soft and did not hold their shape, making them unsuitable for construction use. Bricks with high starch content (36 grams) decomposed too quickly when immersed in water, reducing their durability. Optimum stability was found with starch content between 3 and 9 grams. Bricks with this amount of starch were able to withstand 24 hours under water without disintegrating. Optimum stability was found with starch content between 3 and 9 grams. Bricks with this amount of starch were able to withstand 24 hours under water without disintegrating. Strength was assessed visually, and the bricks that showed the best results after the water test will be selected for further compression tests to finally confirm their mechanical strength and suitability for construction use.
After completing the previous tests with starch and water, the next step was to conduct a compression test to evaluate the strength of the material. For this, four samples were selected that had shown the best results in the previous stages. During the compression test, it was found that bricks with a starch content in the range of 9 to 18 grams outperformed the other samples in terms of strength. During the test, a force of 5 to 9 tons was applied.
Fig.71. Test of Material Resistance to Water
Based on this data, it was determined that the optimal composition for our embankment stabilization system is bricks containing approximately 17% potato starch and subjected to a long firing. This composition provides an ideal balance between strength and durability. These results will form the basis for prototyping in the subsequent stages of the project to ensure that the bricks can be effectively used to strengthen barriers in local conditions.
AND NEXT STEPS
The choice of this material is a kind of compromise between environmental sustainability and the required performance properties. Biodegradable bricks do have a minimal impact on the environment and can be an important support for the local economy. However, their ability to maintain strength in extreme weather conditions and long-term exposure to water remains a question mark. In the future, it will be important to consider adding additional components to improve the water resistance and durability of such bricks.
Fig.72. Testing Material for Compressive Strength
Fig.73. Test Comparison Graph
4.3.1 CONCLUSION
4.4 WIND-RESISTANT URBAN PLANNING
After developing the strategic framework for flood mitigation and selecting sustainable materials for embankment reinforcement, designing for wind resistance becomes another very important step in enhancing the resilience of coastal residential areas. Understanding the impact of wind on buildings and surroundings in cyclone-prone areas like Bangladesh, where strong winds and storms are common, is crucial for developing appropriate and sustainable design solutions.
For that, we have performed a set of Computational Fluid Dynamics experiments, which is crucial for assessing various layouts of buildings, their shapes, and spacing. Such an experiment is one of the optimizations of wind resistance while structural integrity is maintained and balanced with other critical design criteria of cost-effectiveness and practicality.
Wind load calculation method
Define Parameters:
Calculate Dynamic Pressure:
Calculate Effective Area:
Calculate Effective Area:
4.4.1 APPLYING FLUID MECHANICS PRINCIPLES TO DESIGN
To design residential areas capable of withstanding natural disasters like cyclones, it is vital to apply principles of fluid mechanics to understand how wind interacts with buildings. A clear comprehension of the principles gives one the necessary framework in which to interpret the CFD results for making informed design decisions to improve wind resistance in residential areas.
Wind Load Calculation: The assessment of wind load on a building is influenced by various elements, such as wind velocity, the angle of the windward facade, and the area subjected to wind exposure. The principal aim is to mitigate the effects of wind speed and alter the wind dynamics through thoughtful architectural design. Successful approaches include refining building geometries for enhanced aerodynamic efficiency and modifying the alignment and separation of structures to diminish wind-induced forces42.
Bernoulli Effect: The Bernoulli principle is essential in optimizing building forms in the fight against wind forces. According to this principle, increased velocity of airflow should subsequently result in a pressure decrease. This can be manipulated to lessen wind pressure upon buildings by adjusting the shape and curvature of building surfaces. For instance, wind flow can be accelerated on slanted roofs and facades, hence decreasing pressure on building surfaces- a connotation for downforce, improving structural stability and safety43
Wind Channel Effect: In highly built-up areas or lanes, the wind speed may increase due to the channeling effect, where the air must pass through confined passages. This leads to hard winds that could inflict injury on pedestrians and more stress on buildings. These negative effects can be minimal if strategic building layout design is considered; thus, all safety and comfort are guaranteed44
4.4.2 CFD EXPERIMENT SETUP
These principles of fluid mechanics then translate into a set of CFD experiments-wind at 35 meters per second under cyclone-like conditions to assess a wide range of building layouts, shapes, and spacing arrangements that would be efficient to reduce wind resistance and optimize environmental outcomes. The main objective was to identify those design strategies that could reduce wind velocities in the settlement to a safer range, ideally within 10 to 25 meters per second, while at the same time enhancing safety and livability in the residential areas.
42 T.G. van Engelen, Frequency Domain Load Calculation for Offshore Wind Turbines (TURBU Offshore), ECN-RX--04-127 (Energy Research Centre of the Netherlands, 2004), presented at the European Wind Energy Conference, London, 22-25 November 2004
43 V. Olenkov and P. Puzyrev, “Study of Wind Effects on Unique Buildings,” IOP Conference Series: Materials Science and Engineering 262, no. 1 (2017): 012154, https://doi.org/10.1088/1757-899X/262/1/012154
44 Biao, Li, Cunyan Jiang, Lu Wang, Weihua Cai, and Jing Liu. “A Parametric Study of the Effect of Building Layout on Wind Flow over an Urban Area.” Building and Environment 160 (2019): 106160. https://doi.org/10.1016/j.buildenv.2019.106160
Fig.78. Bernoulli Effect
4.4.2 CFD EXPERIMENT SETUP
These principles of fluid mechanics then translate into a set of CFD experiments-wind at 35 meters per second under cyclone-like conditions to assess a wide range of building layouts, shapes, and spacing arrangements that would be efficient to reduce wind resistance and optimize environmental outcomes. The main objective was to identify those design strategies that could reduce wind velocities in the settlement to a safer range, ideally within 10 to 25 meters per second, while at the same time enhancing safety and livability in the residential areas.
4.4.3 BUILDING LAYOUT CFD TEST
The first set of CFD tests analyzed various building layouts in detail. The results showed that linear building layouts could effectively reduce wind speeds between buildings by forming a barrier. This layout, however, increased the airflow velocity around the buildings making the external wind more turbulent. To solve this, a zigzag pattern was tested as well that broke up the stable airflow that the linear patterns exhibited. The zigzag pattern effectively blocked the wind around the building creating a uniform and pleasant wind environment thereby reducing wind concentration effects and improving the wind circulation and safety in the building.
4.4.4 BUILDING SPACING CFD TEST
The CFD analysis further explored how different building spacing intervals affected airflow environments. The results indicated that spacing buildings 5 to 10 meters apart created a low-wind, safe environment. Within this range, wind speeds were reduced, minimizing the direct impact on building facades and pedestrian areas. However, larger spacing increased wind speeds between buildings, creating unnecessary wind channel effects. Therefore, the optimal spacing not only improved wind resistance but also enhanced the comfort and livability of residential areas and urban complexes.
4.4.5 WINDWARD SHAPE CFD TEST
The final CFD tests explored various windward shapes that would yield the most productive strategies to optimize the immediate wind environment. The study showed that while aerodynamically rounded shapes could lower direct wind resistance, they sped up the surrounding wind velocities-maybe a little undesirable in a densely populated city. Furthermore, introducing streamed forms would add more loads to construction and raise costs. Hence, the selected shape should balance structural efficiency and wind effect, considering both functionality and economic viability. The analyses concluded that Type 3 can significantly reduce wind pressure without negatively impacting the surroundings and, thus, could be proven to be the most beneficial design.
4.4.6 CONCLUSION OF CFD FINDINGS
Based on the insight obtained from fluid mechanics, combined with detailed CFD experiments, we investigated and found the most appropriate building layout, spacing, and windward shapes that would minimize the effects of winds and further enhance the resilience of the residential areas against cyclones and strong winds. The obtained results form a sound basis for making a design proposal that balances wind resistance with costeffectiveness and practicality toward ensuring community safety and comfort in cycloneprone regions like Bangladesh.
4.5 CONCLUSION OF RESEARCH DEVELOPMENT
This chapter synthesized critical data and analysis from the following four key areas: Site Analysis, Strategic Framework for Mitigating Flood Risks, Material Testing, and Wind Environment to inform the development of an integrated and sustainable design strategy for the Kalapara region. By carefully selecting the intervention site based on vulnerability criteria and employing a “sponge city” approach for water management, we have developed a targeted plan to address the specific challenges of flooding and erosion in this coastal area. Moreover, biodegradable materials proposed for embankment reinforcements and the wind resistance analysis of buildings’ design show evidence of following sustainability principles, local involvement, and resilience against adverse natural events. These findings in each category create a strong base for the succeeding design development phase, which ensures that each proposed solution is practical and contextually appropriate by striving for effectiveness, costs, and environmental impacts. An integrated series of these research insights supports the development of an adaptive, resilient environment suited to needs and conditions in cyclone-prone regions like Bangladesh.
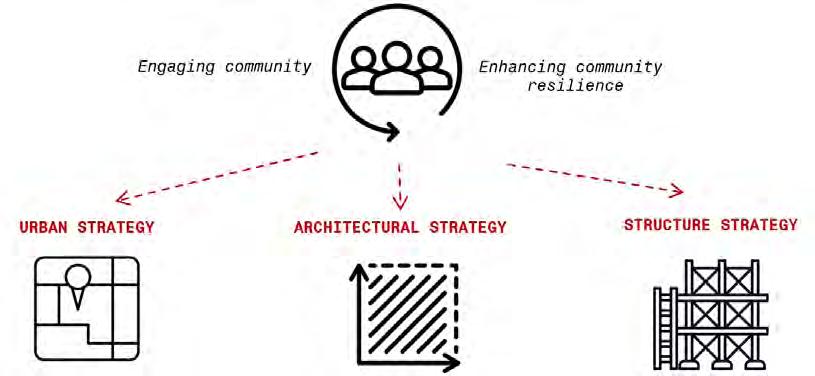
05. DESIGN CONCEPT
Based on the analysis conducted in the previous chapters, our concept aims to create a resilient and adaptive environment for the flood- and cyclone-prone coastal areas of Bangladesh. The concept includes three key areas: urban strategy, architectural strategy, and structural strategy, each designed to reduce the impact of natural disasters and enhance the resilience of local communities.
Urban Strategy
The urban strategy includes two key areas: landscape solutions and settlement planning. These areas are based on the results of the analysis and data obtained in the previous stages of the study and are aimed at solving two main problems: creating a water storage and distribution system, and reducing the impact of wind loads through optimal settlement planning that helps reduce wind speeds in residential areas.
Architecture Strategy
The architectural strategy is based on wind analysis and the use of affordable, sustainable materials such as bamboo. Our main goal is to create residential buildings that can withstand strong winds and cyclones typical of coastal areas. In addition, we plan to build the buildings on elevated platforms to protect them from flooding, especially in areas with frequent flooding.
Structure Strategy
The design strategy is focused on creating strong and flexible structures that can withstand extreme weather conditions. We use modern methods of connecting architectural elements to ensure maximum stability and flexibility. These connections are welded metal tributaries: they are easy to manufacture, but at the same time have high rigidity. The concept developed lays the foundation for sustainable design that considers the natural and climatic features of the coastal areas of Bangladesh. The next chapter, Design Development, will present detailed solutions for implementing these strategies. We will focus on the design and integration of architectural and structural elements into the overall structure of the settlements, which will ensure their long-term sustainability and adaptability.
06. DESIGN DEVELOPMENT
Previous chapters analyzed key vulnerabilities of the selected site in the coastal zone of Bangladesh, including flooding and high winds. These analyses resulted in proposed approaches to water management, sustainable building materials and urban planning that should minimise risks and enhance protection for local communities. Now, in the next chapter, we will focus on how these concepts will be implemented in practice. The Design Development section will offer detailed solutions that combine research findings and conceptual ideas to create resilient settlements adapted to the natural conditions of the region.
6.1 URBAN STRATEGY
1800 sqm 1500 sqm
6.1.1 STORAGE PONDS 3150 sqm
Based on the water management strategy proposed in the concept, the next step was identifying the most suitable locations for creating ponds. Based on the data and calculations made in the previous chapter, we have identified more appropriate places for the ponds that will serve as the main reservoirs for storing and accumulating water. The simulation of water flow has revealed areas where water accumulates most quickly, there are 3 such places on our site, using contour lines we have defined the boundaries of these ponds.
The storage ponds will serve not only as repositories for storage but also provide local people with the chance to fish and breed shrimp, thus bringing socio-economic benefits to the project and improving the overall quality of life in the community.
Fig.83. Rain Water Simulation
Fig.84. Calculations for Storage Pounds
The next stage is building a network of distribution cannels, which will help keep ponds from overflowing and disperse extra water evenly around the property. In the research development chapter, we examined the impact of different canal topologies on water flow and chose a more effective meander form.
A multi-objective evolutionary algorithm optimization method was used to explore the most efficient design, and four design objectives are summarized to establish the fitness criteria.
Design objectives
1. Reducing the costs associated with landscape alteration and construction.
2. the change in the slope of the canal should be gradual.
3. the canal should merge gradually into the main waterbody
4. changes in the curvature of the canal should not be overly significant.
Gene pool
Based on the design objectives, certain parameters in the parametric model are set as genes, i.e., variables, which affect the final shape. For example, the location and amount of control points used to generate the flow lines of a canal can be varied to affect the curvature of the canal as well as the magnitude of the bends. In areas of higher slopes, more bends can be generated by placing more control points to slow down the water flow. Afterwards, four fitness criteria are set to evaluate the performance of the optimization results.
Fig.85. Gene pool definition
6.1.2 DISTRIBUTION CANNELS
Fitness criteria
1. Minimize the entire length of the canal
2. Minimize the difference in the slope of each section of the canal
3. Minimize the elevation at the junction between the canal and the main river cannel
4. Minimize the curvature differences of each section of the canal
Fig.86. Parallel Coordinate Plot from Canal Optimization
Fig.87. Standard Deviation FC.1 Min Canal Length
Fig.88. Standard Deviation FC.3 Min Z-value of joint pt
Fig.89. Standard Deviation FC.2 Min Canal Slope
Fig.90. Standard Deviation FC.4 Min Curvature Difference
Fig.91. Pareto Front Canal
Fig.92. Average Criteria Result
Fig.93. The Seleted Result G42-28
Fig.94. Diamond Chart G42-28
Fig.95. Diamond Chart Average Criteria Result
The river optimization results were selected not only based on the performance of the optimization outcomes concerning the four objectives but also regarding to their actual morphology. By analyzing the morphology of the pareto front it is possible to identify some results that although performing well across the four optimization objectives, parts of the canal are too close to the main river or the spacing between the two canals is narrow, which can lead to potential risks. (figure) Therefore, In selecting the optimal individuals for the Pareto front, the distances between the river canals and the distance from the river canals to the main river of the site were also considered. Ultimately, g42-28 best met these criteriaand will be used as the final choice for the soft design proposal.
6.1.3 SOFT LANDSAPE DESIGN
Curvature analysis is essential for river canal design since the curvature of the alignment affects water flow and erosion control. Bends in the canal result in uneven forces on the water surface, with the convex side experiencing a rise in water level due to the superelevation. This creates significant erosion risks, especially at high velocities and large deflection angles, requiring additional protective measures. As noted in the principles of channel design, these effects are particularly pronounced at bends where erosion can undermine the stability of the channel banks45
Therefore, after achieving the optimal channel morphology, its curvature analysis was carried out at different locations. Circles were created at the analyzed positions to better visualize the erosion risk, With reference to the size of the area (7-10 meters) required for the soft landscape previously defined in the research development chapter, the radius of the circle obtained from the curvature analysis is remapped, and still corresponded to the curvature at that particular location, thereby illustrating the change in erosion risk at different points. The resulting distances were used as a basis for proposing soft scape terraces and create the final terracing design. Plants and grass that promote absorption were selected for planting on these terraces to improve absorption properties.
45 P.K. Swamee and B.R. Chahar, Design of Canals (New Delhi: Springer, 2015), http://dx.doi.org/10.1007/978-81-322-2322-1_2
Fig.96. Section A-A. Terrace Creation Process
Fig.97. Sections A-A / B-B
6.2.1 DESIGN STRATEGY OF BUILDING MORPHOLOGY
The design concept of Morphology incorporates four key elements, each addressing specific challenges associated with extreme weather conditions.
Cyclone-resistant roof design: The roof is designed to withstand cyclone winds and includes a central operable opening for rainwater collection and pressure balance between the indoor and outdoor environments during cyclones. This feature not only enhances the structure’s resilience but also promotes sustainability by incorporating a rainwater harvesting system. Therefore, a series of CFD experiments were conducted for roof morphology.
Elevated Building Structure: The building is 2 meters above ground level to mitigate the risk of flood damage, particularly in areas susceptible to rising waters. This elevation is designed to protect the structure and its occupants from floodwaters, providing an additional layer of security in flood-prone regions.
Lightweight Structural Components: Utilizing lightweight materials promotes rapid assembly and seeks to reduce the risk of injuries in the event of structural failure. This approach emphasizes ease of construction and occupant safety, enhancing the building’s adaptability to emergencies while preserving its structural integrity. Therefore, the structural strength behavior of the different species of bamboo that can be used for construction will be tested in the continuous optimization of the design.
Modular Design: The design employs a modular approach for as many structural elements as feasible. This strategy streamlines construction and enhances cost efficiency where applicable, while non-modular elements allow for necessary customization based on specific site conditions or design requirements.
Accordingly, formulated strategies regarding building morphology assessments are to be carried out to analyze the performance of structures when affected by the impact of high winds and cyclones. The most important part of the process is to enhance roof configuration with architectural planning of the central shaft for optimal stability. For this purpose, we conducted a series of CFD tests to evaluate how different roof parameters and wall heights affect the wind pressure and the structure’s resistance.
6.2.2
MORPHOLOGY CFD TEST
In order to clarify the effect of roof slope and height on the wind environment in typhoon conditions, models with different roof heights were tested to assess their performance under strong wind conditions and to analyse their specific impact on the surrounding airflow distribution.
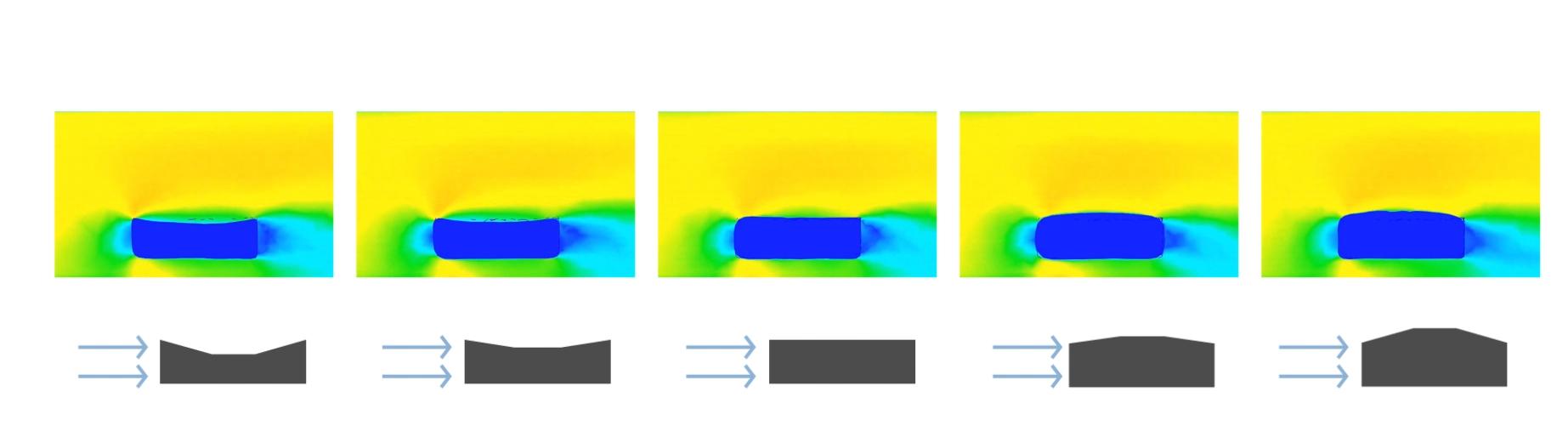
The experimental results show that when the roof is higher than the wall, the airflow velocity above the building increases significantly, leading to lower pressure and creating a more significant uplift force, which imposes an additional tensile force on the building structure and affects the stability of the roof and the overall structure, especially under solid cyclone conditions, where this tensile force may lead to the risk of the roof being torn apart or lifted, which may, in turn, compromise the building’s structural integrity. On the contrary, when the roof is lower than the wall, the airflow creates a low-pressure zone above the building, with lower wind speeds and uplift forces. This design effectively reduces the pressure on the roof and improves safety and stability. At the same time, due to the high velocity and low pressure of the airflow above, the higher wall can act as an additional barrier to help disperse and direct the wind force, avoiding excessive concentration of wind speed around the building.
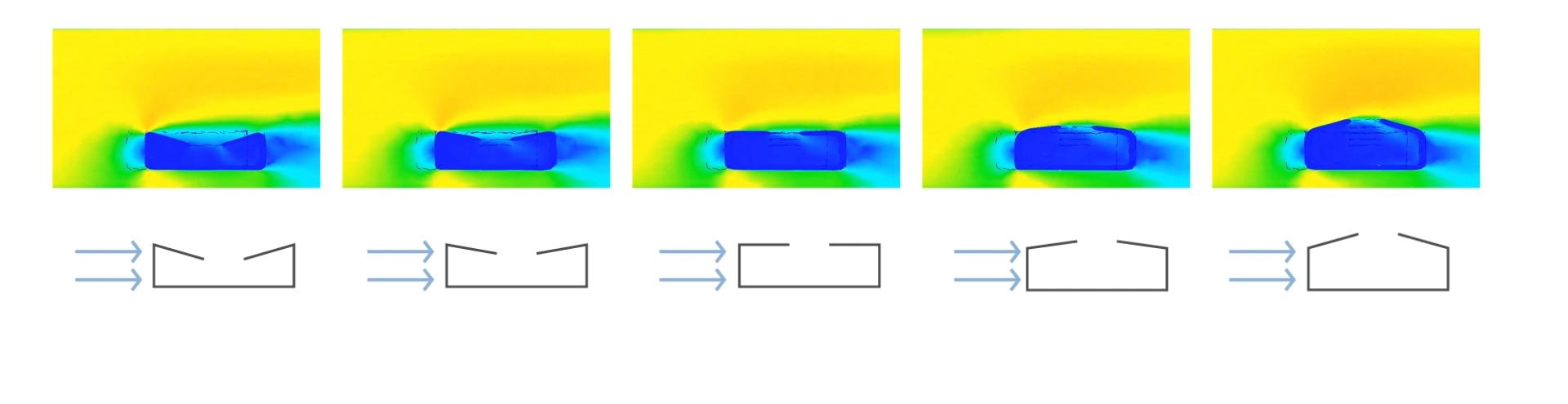
On this basis, we further tested the design principle of the central shaft. In a cyclone, the central shaft balances the pressure inside and outside the building. When strong winds strike, the windward side is subject to high pressure, while the leeward side generates low pressure, creating uplift forces that can damage the roof. The central shaft effectively balances the internal and external pressures by providing an airflow path that directs air from the interior of the building to the high-pressure areas, thereby reducing the net force exerted on the roof. This pressure balance reduces the risk of the roof being lifted and the potential for damage caused by negative internal pressures. The central shaft enhances the building’s resilience against strong wind loads during cyclones by managing airflow and pressure distribution. Therefore, a combination of roof height, wall design and the use of central shafts is essential to improve the overall wind performance of a building.
From the outcomes presented in the CFD tests, further optimization of the design and shape had to be considered in order to really improve the resistance of buildings against cyclonic winds and floods. Optimization here means to find an optimum between strength, material efficiency, and reduced solar radiation, which also enhances living comfort. For this purpose, we applied a multi-criteria optimization evolutionary algorithm which permits us to find the optimal form of a building, together with appropriate structural solutions.
Fig.127. CFD Analysis
Fig.128. CFD Analysis
6.2.3 EVOLUTIONARY ALGORITHM OPTIMIZATION FOR BUILDING
Corresponding to building design concepts, addressing extreme flooding involves elevating the structure to prevent water ingress. The corresponding strategy is to create a robust structural system to withstand strong winds from cyclones. The materials used for the structure will be locally sourced bamboo, and the design must facilitate quick assembly. This ensures that even if a severe weather event causes inevitable damage. Residents can have repairs made quickly. Consequently, this study employs a multi-objective evolutionary algorithm to optimize the structure and form of the building, aiming to achieve the best performance outcomes.
Three design objectives were identified before the optimization of the building.
Design objectives
1. Reducing wind damage to building structures
2. Reducing material usage while maintaining structural strength.
3. Maximize self-shading
Gene pool
Certain parameters, such as genes in the parametric model, can be adjusted to modify the building’s shape in order to identify the optimal form. Additionally, the performance parameters corresponding to three indigenous Bangladeshi bamboo species suitable for construction were incorporated into the model calculations to evaluate various structural strength performances.
Accordingly, a set of three fitness criteria (FC) were designated.
Fitness Criteria
1. Minimize the displacement of structure
2. Minimize the sum of the volumes of structure components
Fig.129. Changing the location of central opening
Fig.130. Changing the joinery point location
Fig.131. Changing the material size
Fig.132. Changing the slope of the roof
Parallel Coordinate Plot reveals the interrelation between individuals’ behaviors through the evolutionary simulation. as the evolutionary process continues, better individuals are observable in terms of FC1, FC2 and FC3 values. However, the FC.2 values reflects conflict compared to other FCs.
The standard deviation plots allowed the study of FC-specific domains for each generation; combining the diamond plot allows for a better view of the differences. Therefore, this requires a reassessment of the FCs and strategies to measure the potential impact of the goals on other criteria.
Fig.133. Parallel Coordinate Plot from Morphology Optimization
Fig.134. Standard Deviation FC.1 Min Displacement
Fig.135. Standard Deviation FC.2 Min Material Use
Fig.136. Standard Deviation FC.3 Min Solar Radiation
Fig.137. Diamond Chart Rank.0 for FC1
Fig.138. Diamond Chart Rank.0 for FC2
Fig.139. Diamond Chart Rank.0 for FC3
Consequently, different weights are assigned to the three fcs based on their significance, with a weight ratio of 6:3:1. By applying the new selection strategy to the top individuals in the Pareto front, the best-performing individuals that meet the design objectives can ultimately be identified, which is generation 47 individual 0.
Fig.140. Pareto Front Canal
Fig.141. Final Selection G47-0
Fig.142. Diamond Chart G47-0
From the result of FEA analysis, the maximum displacement of the chosen morphology is about 9.89 centimeters, and the average displacement is about 5.85 centimeters. Which is satisfied the safety requirement for building structure. In addition, the chosen results satisfy the requirement to reduce material use while maintaining high structural strength. the primary structure is by Bambusa Balcooa with 7.5cm diameter, the secondary structure and extra frame both by Denrocalamus Strictus with 5cm diameter.
Fig.143. FEA Analysis
Fig.144. Structure and Material Size
Fig.145. Solar Radiation
5.2.4
PLANTING
The computational fluid dynamics (CFD) analysis illustrates how trees can influence wind flow around a settlement exposed to a wind speed of 35 m/s. In the first scenario, without protection from trees, the wind directly impacts the buildings, creating high-speed wind zones and vortices behind the structures. This results in turbulence and uneven wind flow throughout the settlement. Conversely, the second scenario, which includes trees positioned according to the planting strategy, demonstrates how vegetation serves as a natural wind barrier, decelerating the wind and mitigating its effects on the buildings. Additionally, the trees help to reduce vortex formation, leading to a smoother and more uniform wind distribution. This underscores the significance of trees in urban environments
Fig.147. CFD Result with new planting and existing contexts
48 m/s
0 m/s 15m/s 30 m/s
45 m/s
0 m/s 15m/s 30 m/s
Fig.148. CFD Result without planting
Fig.149. CFD Result with new planting
Fig.150. Section
Fig.151. Settlement Top View 1
Fig.152. Settlement Top View 2
6.3 STRUCTURE STRATEGY
Following the architectural approach and refining building configuration to consider the aftermath of cyclones and floods, the next crucial step is the development of a structural strategy. This structural strategy must ensure that buildings are not only strong enough to withstand extreme weather but also adaptable, enabling quick repairs after natural disasters like cyclones and floods.
The structural joints we’ve developed, consisting of welded metal tube connectors designed to accommodate bamboo poles, offer enhanced stability and flexibility. These connectors are simple to fabricate yet robust enough to withstand external forces such as strong winds. The six joint types offer versatility for different structural needs, ensuring both strength and adaptability. This system allows residents to quickly replace damaged components and restore their homes, which is essential in regions where natural disasters strike seasonally.
The foundation system is based on a combination of traditional and modern methods to ensure the reliability and durability of the structure. The main components include:
Bamboo Poles: Act as the vertical load-bearing elements of the building, supporting the main load of the structure
Metal Connectors: Provide a strong attachment of bamboo poles to the supporting base, ensuring the durability of the connections
Metal Support Base: Distributes the load from the bamboo to the foundation, connecting the traditional material with a modern reinforced base
Concrete Foundation: Provides a stable platform for the building, protecting the structure from displacement and external influences
Concrete Filling of the Cavity: Increases the strength of the connection of bamboo poles to the foundation, improving the stability of the entire system.
This system effectively combines traditional materials with modern methods, creating stable structures that can withstand external loads.
Fig.153. Foundation Element for a Bamboo Structure
Four-Way T-Joint
Description: This connection uses a metal connector that joins 4 bamboo poles at right angles, forming a T-shape. The joint is secured with fasteners through pre-drilled holes in the metal connector. This design is ideal for vertical and horizontal junctions, supporting load distribution along the frame.
8-Way Radial Joint
Description: This connector allows eight bamboo poles to join at various angles around a central axis, creating a radial starlike structure. It is used to distribute the structural load equally in multiple directions and is suitable for domes or structures needing multidirectional support.
Four-Way Cross Joint
Description: The four-way connection utilizes a central metal connector to join bamboo poles in a cross-shaped pattern. It supports two poles horizontally and two vertically, distributing load in two opposite directions.
Fig.154. Four-Way T-Joint
Fig.155. 8-Way Joint
Fig.156. Four-Way Cross Joint
For the walls of the building, we decided to use traditional materials such as bamboo mats. This is one of the traditional construction methods in Bangladesh, and its effectiveness has been proven for centuries. The weaving of bamboo mats can vary in shape and density. Some types of weaving create a strong tie effect, which significantly improves the resistance of the structure to wind loads. Thus, walls made using such materials and techniques are not only durable, but also adapted to local climatic conditions.
The use of bamboo mats has another important advantage: in case of damage to the building, for example, after a strong wind or cyclone, the walls can be easily restored or replaced. Since the building’s core - a frame reinforced with strong connecting joints - remains stable, repairs are limited to replacing or restoring the external walls, which significantly speeds up the restoration process.
In addition, an important part of the design is the open shaft in the center of the building. It promotes improved natural ventilation, which is especially important in the hot and humid climate of Bangladesh. The shaft helps maintain a comfortable temperature in the premises by creating air flow and reducing the need for artificial cooling.
Fig.157. Section of the Proposed House
BAMBOO ROOF
BAMBOO RAFTER SYSTEM
WOVEN BAMBOO EXTERIOR WALL
METAL СONNECTOR
BAMBOO POLE
PLANK FLOORING
RAILING
BAMBOO POLE
CONCRETE FOUNDATION
STAIRS
Fig.158. Exploded Axonometric View
Fig.159. Settlement View 1
Fig.160. Settlement View 2
6.4 MIXED REALITY ASSISTED PLATFORM
To test the effectiveness of integrating mixed reality (MR) technology into construction training, we conducted a series of experiments to understand how this technology could improve the training of local residents to build and renovate the homes designed as part of our project. The basic idea is that by training a group of people, they will be able to build and renovate homes on their own and earn money by providing services to other communities.
MR can provide an environmentally aware overlay of digital content in the physical world, with users able to interact with both the digital and physical worlds simultaneously. It can also provide visual feedback to the user during manufacturing.
INTERACTIVE LEARNING
Interactive Learning MR technologies overlay digital content on the physical world, allowing users to interact with both simultaneously. In the context of construction, MR can provide visual feedback at each stage, allowing users to learn the techniques for constructing new homes. This training will not only improve the level of construction skills of local residents, but will also enable them to renovate homes quickly and efficiently in the future, minimizing their dependence on external specialists.
Fig.161. Learning About Connections Through MR
When using MR for construction training, quality control at each stage of the process is a critical part. With MR technology, users can see a digital model of the building at a 1:1 scale, which helps them accurately follow the instructions. During construction, the systems can collect data on the actual condition of the elements and compare it with the digital model. This provides real-time feedback that allows errors to be corrected early, ensuring that all architectural components are accurately aligned and meet standards.
Community Perspectives Training local residents through MR technologies not only helps in creating a sustainable and efficient construction system, but also provides them with new economic opportunities. Having acquired the knowledge and skills to build using new technologies, residents will not only be able to build and repair their own homes, but also offer services to other communities, creating new jobs and sources of income.
This approach demonstrates how MR technologies can not only improve the quality of construction, but also play a key role in the long-term sustainability and self-sufficiency of local communities.
QUALITY CONTROL AND GUIDELINES
Fig.162. Installation of Foundations Assisted by MR
6.5 CONCLUSION FOR DESIGN DEVELOPMENT
The Design Development chapter develops our initial ideas into workable plans that are suited to the unique difficulties encountered by Bangladesh’s flood—and cyclone-prone areas. In addition to reducing the risk of flooding, our adaptive water management plan— which includes storage ponds, terracing, and canals—offers socioeconomic advantages like shrimp farming, enhancing the project’s emphasis on resilience and community well-being. In terms of materials, the introduction of biodegradable bricks made from local potato starch represents a sustainable solution. These bricks demonstrate our dedication to environmental care since they are made to break down gradually and encourage the growth of flora. While their use integrates natural systems and improves embankments, continuous monitoring of their longevity is still essential.
The CFD study guides settlement design, which balances livability improvements with wind load reduction. By fusing traditional bamboo materials with contemporary structural technology, we guarantee flexibility and ease of repair in post-disaster scenarios, enabling local populations to actively participate in sustaining their homes.
Lastly, using Mixed Reality (MR) in the building process improves accuracy and promotes community involvement and training, demonstrating a progressive approach to sustainable development. When combined, these tactics offer a robust, flexible solution that considers the requirements of both people and the environment.
PROTOTYPE OF THE BRICK
The next step in our project was to create a physical prototype of the brick based on the materials tested in the previous experiments. To do this, we developed a mold using CNC technology, which allowed us to accurately calculate the volume of material needed to produce the brick. The brick was successfully produced, confirming the viability of our approach.
However, we encountered certain limitations during the work. The mixture for making the brick requires baking, as it is not strong enough to maintain the shape without heat treatment. In the future, it is worth considering the possibility of developing a mold that can withstand high temperatures, which will improve the manufacturing process. In addition, studying additional components that could accelerate the hardening of the mixture or increase its strength may allow the brick to be removed from the mold faster, thereby speeding up the production process and reducing the need for a large number of molds for assembly.
This prototype was an important step in our research, and its creation opens up opportunities for further improvements in the production technology. It will form the basis for subsequent analysis of the efficiency and possible improvements to the system.
07. PHYSICAL
Fig.163. Mold for Brick Fabrication: Proposed Design
Based on preliminary material tests and experiments, we have identified the optimal percentage ratios of the components to create a brick measuring 30 cm × 21.5 cm × 5 cm:
Water: ~679 ml (13.6% of the total weight)
Sand: ~4526 g (90.7%)
Dry clay: ~453 g (9.1%)
Starch: ~204 g (4.1%)
These proportions were determined through a series of strength and biodegradability tests, which allowed us to create a material that meets our requirements for durability and environmental friendliness.
Mixing the components:
All components are placed in a container and mixed thoroughly. This initial step is important to achieve a homogeneous mass, which will ensure that all materials are evenly distributed in the finished product
Kneading the mixture:
After adding all the ingredients, the mixture is thoroughly mixed until a homogeneous consistency is achieved. This is necessary to ensure that the mixture is sufficiently plastic and easy to process
Filling the mould:
The prepared mixture is placed in a mould created using CNC technology. At this stage, it is especially important to fill all corners and voids of the mould to avoid defects in the final product
Compacting and moulding:
After filling the mould, the mixture is manually compacted to avoid air pockets and ensure the future strength of the brick
The final stage involves baking the brick at 180°C for 4 hours. The result is a strong, structurally stable brick that retains its shape and properties
Fig.164. Brick Making Process
Fig.165. Physical Prototype
Fig.167. 3d Printed Model
Fig.168. 3d Printed Model
Fig.166. Physical Prototype
07. POST-ANALYSIS
Our post-analysis aims to assess how successfully our strategies have been implemented and what the prospects are for them in the event of further deterioration of climate conditions. The focus is on water, wind load and the overall impact of the implemented solutions on the resilience and adaptation of the settlement.
Water distribution
Based on the previously collected data, we were able to calculate the amount of water that can be effectively distributed by our drainage and storage system. The results show that the current strategy will work as long as the water volume does not exceed the calculated limit. However, in the event of a significant increase in precipitation or more frequent floods, more serious interventions will be needed, both in terms of infrastructure and water management. Until that point, our system demonstrates resilience, and experience shows that such strategies based on nature-based solutions without significant engineering interventions can effectively cope with the challenges of climate change. This is also confirmed by the results of a study of similar solutions applied within the framework of a case study, where nature-based measures helped to mitigate the effects of natural disasters.
Fig.169. Water Distribution Calculation
Wind load impact
As a follow-up to the analysis, we conducted CFD modeling taking into account all the interventions implemented during the project. The results show that the wind speed inside the settlement was significantly reduced in the early stages due to the correct placement of buildings and the use of natural landscape elements. Additional tree planting and consideration of existing vegetation showed that these measures also effectively protect the buildings from strong winds. Choosing a site with existing trees on the windward side turned out to be the right decision. This not only reduces wind loads, but also potentially reduces the dependence on strict building placement in the future. However, one of the difficulties in the analysis remains predicting how wind conditions may change with further climate change.
Prospects and conclusions
In conclusion of the post-analysis, it can be said that our strategic decisions based on a combination of natural and architectural interventions show good prospects under current climate conditions. However, further deterioration of the climate will require an expanded approach to water management and wind loads. These findings highlight the importance of flexible and adaptable solutions that can be scaled and modified to meet emerging climate challenges.
Fig.170. Wind Load Impact
DISCUSSION
Our research aims to develop resilient and adaptable architectural solutions for coastal areas of Bangladesh, which are under constant threat from climate change, particularly floods and cyclones. We used a multi-layered approach, combining urban planning, architectural solutions, structural strategies and modern computational methods. All this aims to create an integrated system that can cope with both current and future climate challenges.
The use of CFD (computational fluid dynamics) helped us analyze air flows and find optimal building shapes and locations to reduce wind loads. However, there were limitations. Although CFD is effective in modeling wind conditions, it only provides a partial understanding of how buildings interact with their environment, especially in extreme weather conditions. More research is needed to obtain a more accurate picture. In particular, it is important to study how air flow distribution affects infrastructure and how this data can be used to improve the resilience of settlements to strong winds.
There was also a need to delve deeper into the water absorption capacity of soil and its role in flood prevention. Although we touched on this topic, the research was superficial. Flood management requires a detailed analysis of the soil condition, its water retention capacity and how it will respond to climate change. To do this, we will need to engage experts in hydrology and soil science to develop more effective water management strategies. Without this data, our project remains vulnerable in terms of long-term sustainability.
One of the key elements of the project was the biodegradable potato starch bricks that we used to strengthen the embankments and stabilise the soil. This innovative material shows promise in terms of environmental sustainability and integration with natural processes. However, at the moment, these bricks are only used to strengthen embankments. To make them more versatile and mass-produced, their formulation needs to be improved. This will open up new opportunities for job creation in the region and will be a major contribution to the economic development of local communities.
Improvements to the brick formulation should allow them to be adapted for use in different types of landscapes and in wider construction processes. At this stage, our tests were not comprehensive enough to understand how these bricks would perform in the extreme climate of Bangladesh. We also did not yet test how the decomposition of the bricks would affect the growth of plants such as vetiver and how this would affect the soil stabilization. These issues require further research to ensure their sustainability and environmental effectiveness in the long term.
Greenery plays an equally important role in flood management and soil stabilization. Plants, especially trees and grasses with strong root systems, help retain water in the soil and prevent erosion. We have started to develop this idea, but the potential of vegetation has not yet been fully explored. For example, planting trees along banks or on high ground can act as a natural barrier, slowing down the flow of water and promoting its absorption. However, the interaction of vegetation with engineering solutions such as biodegradable bricks requires further analysis. We need to understand how to integrate green spaces into architectural and engineering measures as effectively as possible to increase the resilience of settlements to changing climate conditions.
We also aimed to create structures that are easy and quick to erect, but in practice this turned out to be more difficult than expected. Despite the use of six types of connections, assembling these structures still requires significant effort, especially for people with limited construction experience. The possibility of using mixed reality (MR) technologies to simplify the process was considered, but the high costs and complexity of these technologies make them difficult to access for poor regions. Even if MR is introduced into educational programs, the question remains about its real applicability and accessibility for the local population.
Long-term resilience of settlements is another important aspect of the project, especially in the context of increasing vulnerability of coastal areas to climate change. We considered various scenarios related to worsening climate conditions, including sea level rise, but the question of how the settlements will be able to adapt in the next 15 years requires further study. Strategies need to be developed that will allow settlements not only to survive, but also to successfully adapt to frequent extreme weather events and possible resource shortages.
Overall, our project demonstrates significant advances in and integration of traditional knowledge and modern technologies such as CFD and MR, which helped optimize the placement of buildings, water flow management and construction processes. However, there are still issues such as insufficient analysis of wind conditions, difficulties in using biodegradable materials and high costs of MR technologies. These issues require further research and development.
In conclusion, the project offers a holistic approach to creating resilient settlements that can adapt to changing climate conditions. We have proposed short-term measures to reduce risks and long-term strategies to ensure sustainability. However, to fully realize our vision, further research is needed on the durability of materials, improving wind flow management and exploring the application of MR technologies under economic constraints. This will help lay a solid foundation for sustainable development in the coastal areas of Bangladesh.
BIBLIOGRAPHY
• Ahmed, K. Iftekhar. “The Rural Bangladeshi Courtyard.” Department of Architecture, BUET, Dhaka 1000, Bangladesh, and Postgraduate Programs in Disaster Management, BRAC University, 66 Mohakhali C/A, Dhaka 1212, Bangladesh.
• Alam, M. R., A. B. M. Amrul Kaish, M. F. M. Zain, and Sadia Mahzabin. “Vulnerability Assessment and Construction Recommendations of Local Houses in the Cyclone Prone Coastal Areas of Bangladesh.” International Journal of Disaster Risk Reduction*21 (November 2016).
• Ali, Anwar. Climate Change Impacts and Adaptation Assessment in Bangladesh. 1999.
• Architectural Review. Building with Bamboo: Trans-Himalayan Practices. Accessed September 20, 2024. https://www.architectural-review.com/buildings/ bamboo/building-with-bamboo-trans-himalayan-practices.
• As-Salek, J. A. “Coastal Trapping and Funnelling Effects on Storm Surges in the Meghna Estuary in Relation to Cyclones Hitting Noakhali–Cox’s Bazar Coast of Bangladesh.” 1998.
• Baten, Abdul, Pedro Arcos González, and Rafael Castro Delgado. “Natural Disasters and Management Systems of Bangladesh from 1972 to 2017: Special Focus on Flood.”
• Bhattacharyya, S. Highway Embankment Design in Bangladesh.*Published December 1, 2009. Engineering, Environmental Science.
• Biao, Li, Cunyan Jiang, Lu Wang, Weihua Cai, and Jing Liu. “A Parametric Study of the Effect of Building Layout on Wind Flow over an Urban Area.” Building and Environment 160 (2019): 106160. https://doi.org/10.1016/j.buildenv.2019.106160.
• CEGIS. “Risk from Major Cyclones during 1960-2009.” 2010.
• Climate Risk Country Profile: Bangladesh (2021). The World Bank Group, 2021.
• CH. Climate Change and Child Health Case in Selected Cities of South-western Nigeria. The New York Academy of Medicine, 2010.
• Dasgupta, Susmita, et al. “Vulnerability of Bangladesh to Cyclones in a Changing Climate: Potential Damages and Adaptation Cost.” World Bank (Policy Research Paper no. 5280, April 2010).
• Deb, Kalyanmoy. “Multi-Objective Evolutionary Algorithms.” Springer Handbook of Computational Intelligence (2015): 995-1015.
• Dutilly, C., and E. Hainzelin. “Conclusion: Food System Adaptation and Mitigation: Managing Trade-Offs.” 2019.
• Guadua Bamboo. Joining Bamboo: Tips and Tricks. Accessed September 20, 2024. https://www.guaduabamboo.com/blog/joining-bamboo.
• Get Bengal. “The Cyclone Which Became a Trigger for the Birth of a Nation.” Accessed September 20, 2024. https://www.getbengal.com/details/the-cyclonewhich-became-a-trigger-for-the-birth-of-a-nation.
• Government of Bangladesh (GOB). Patuakhali District. 3rd ed. Dhaka: Surveyor General of Bangladesh, Survey of Bangladesh Offices, 1997.
• Haider, Mohammed Ziaul, and Md. Firoz Ahmed. Multipurpose Uses of Cyclone Shelters: Quest for Shelter Sustainability and Community Development. Economics Discipline, Khulna University, 2021.
• Hanif, Abu, and Md. Hossain. “A Study on Planning of Rural Settlement: A Case Study of Baidyerbazar Union, Bangladesh.” European Academic Research 3 (2015): 1693-1722.
• Husein, Rahmawati. “Localizing Disaster Risk Reduction: A Case Study of Community-Based Disaster Preparedness in Bima and Palangkaraya, Indonesia.” IOP Conference Series: Earth and Environmental Science 989 (2021).
• Islam, M. Rafiqul. Where Land Meets the Sea: A Profile of the Coastal Zone of Bangladesh. Water Resources Planning Organization, Dhaka, Bangladesh, 2004.
• Jia, Zheng. Cyclone Shelters and Cyclone Resilient Design in Coastal Areas of Bangladesh. Master’s thesis, Massachusetts Institute of Technology, 2010.
• Lectures Bureau. “A Hurricane Is Indeed a Machine of Inconceivable Power.” Accessed September 15, 2024. https://www.lecturesbureau.gr/1/hurricane-is-indeed-a-machine-of-inconceivable-power-1538.
• Liese, Walter, and Michael Köhl. Bamboo: The Plants and Its Uses. Switzerland: Springer Cham, 2015.
• Marina Tabassum Architects. Projects. Accessed September 12, 2024. https://www.marinatabassum.com/projects.
• Planning Commission. The Fifth Five-Year Plan 1997-2002. Dhaka: Planning Commission, Ministry of Planning, Government of Bangladesh, 1998.
• Rahman, M. Hasinur, Md. Sadequr Rahman, and M. Mostafizur Rahman. *Disasters in Bangladesh: Mitigation and Management.
• Ready4Adventure. Land Rover Defender Overland Adventure - Iceland Full Documentary. YouTube video, 50:02. September 5, 2023. https://www.youtube. com/watch?v=7oEpcMkbU8c&ab_channel=Ready4Adventure.
• Shidore, Sarang. Climate Security and Instability in the Bay of Bengal Region.* Discussion Paper Series on Managing Global Disorder No. 13, April 2023.
• Showkatbakhsh, Milad, and Mohammed Makki. “Multi-Objective Optimisation of Urban Form: A Framework for Selecting the Optimal Solution.” 2022.
• Swamee, P.K., and B.R. Chahar. Design of Canals. New Delhi: Springer, 2015. http://dx.doi.org/10.1007/978-81-322-2322-1_2.
• The Report. “Cyclones of Bangladesh: What You Need to Know & How to Stay Safe.” Accessed September 20, 2024. https://thereport.live/bangladesh/cyclones-of--bangladesh-what-you-need-to-know-how-to-stay-safe/30156.
• The World Bank Group. Climate Risk Country Profile: Bangladesh. 2021.
• Van Engelen, T.G. “Frequency Domain Load Calculation for Offshore Wind Turbines (TURBU Offshore).” ECN-RX--04-127 (Energy Research Centre of the Netherlands, 2004), presented at the European Wind Energy Conference, London, November 22-25, 2004.
• WTVY News 4. “Hurricane Categories.” Last modified June 4, 2021. https:// www.wtvy.com/2021/06/04/hurricane-categories.
• Xie, Y., Ma, J., and Li, Y. “Optimization of Building Form and Layout Based on CFD Simulation.” Journal of Architecture and Civil Engineering 36, no. 2 (2020): 106-112.