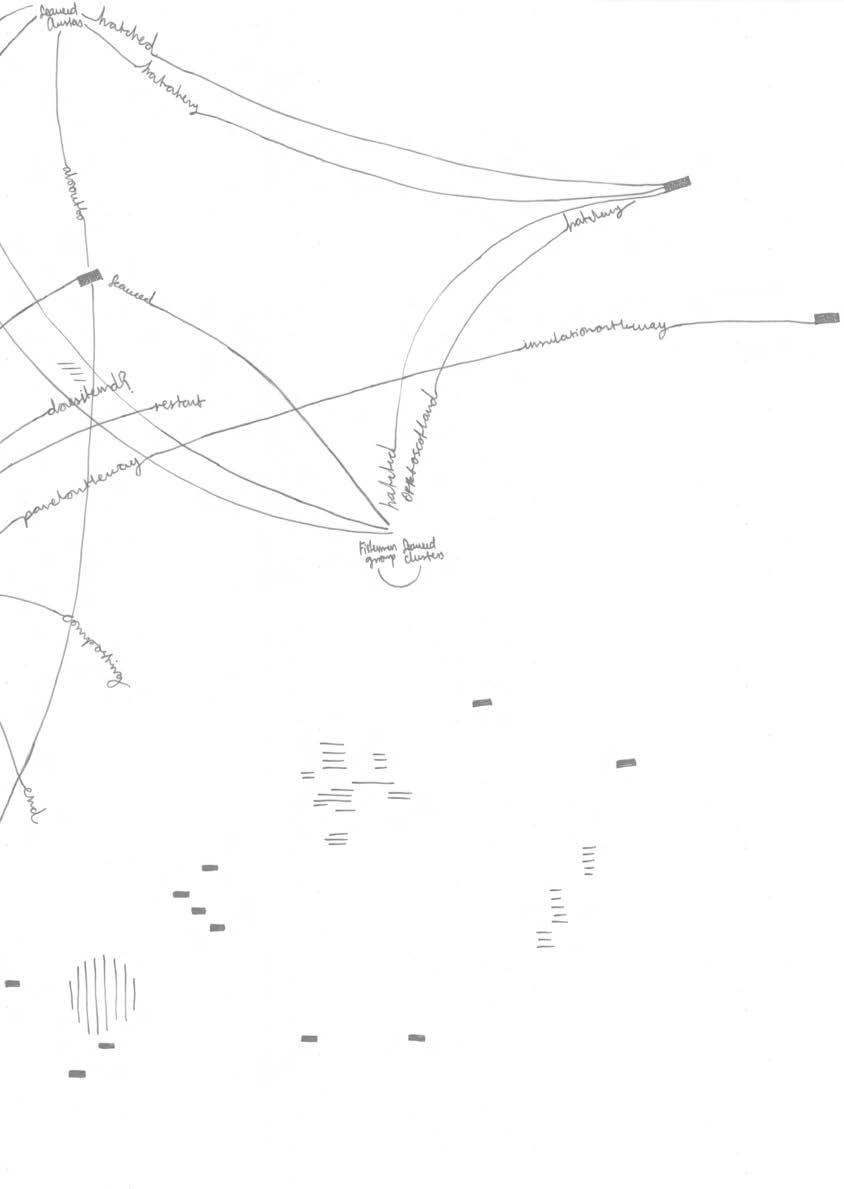
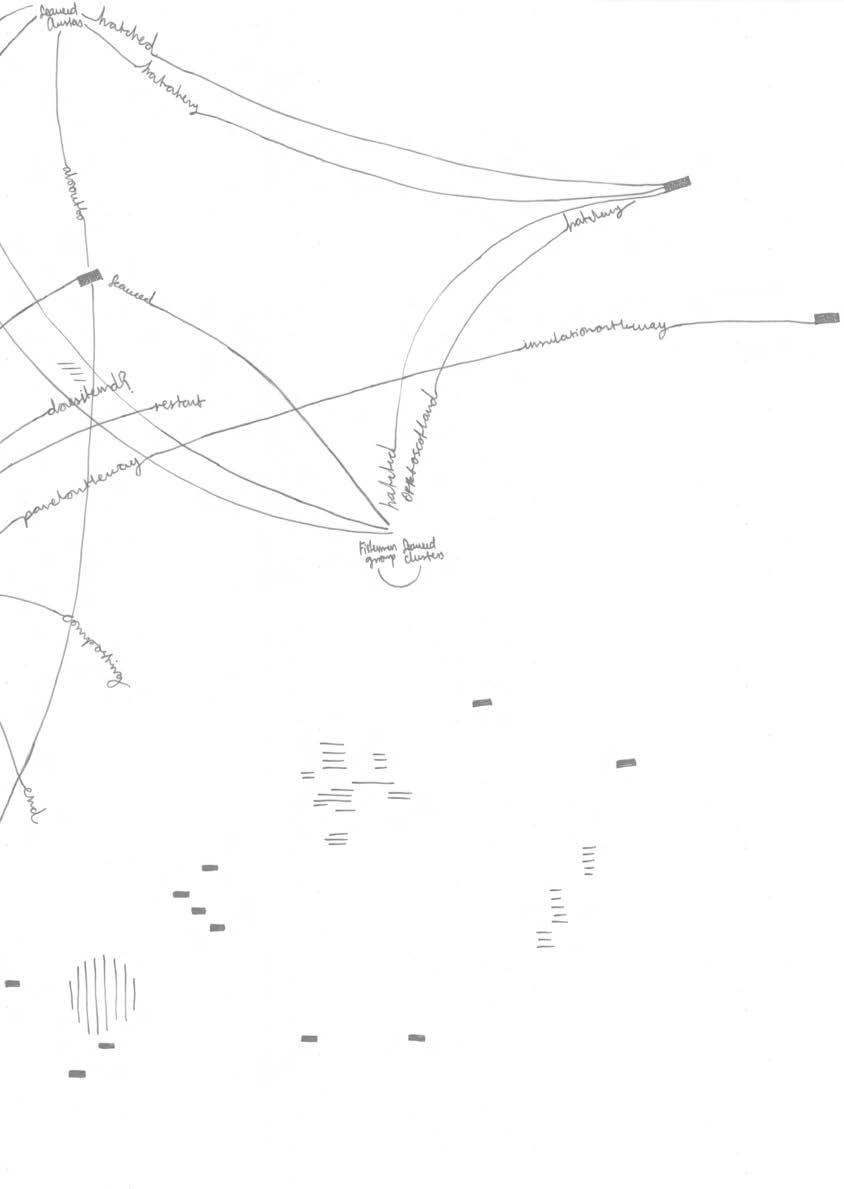
CULTIVATING COMMONS
Submission
Alejandra Iturrizaga Andrich
In collaboration
Priyanka Awatramani
Emily Bowerman
CULTIVATING COMMONS
Biobased Retrofitting for Public Institutions
Architectural Association School of Architecture
Landscape Urbanism Msc 2023-2024

CULTIVATING COMMONS
Thesis Supervisor
Clara Olóriz Sanjuán
Programme Directors
Jose Alfredo Ramirez
Eduardo Rico
History & Theory Seminar Tutor
Elena Luciano Suastegui
Technical Tutor
Daniel Kiss Huang Sheng-Yang
Acknowledgements
This thesis was developed during terms 2 and 3 in collaboration with my teammates, Priyanka Awatramani and Emily Bowerman. I completed term 4 on my own as part of the MSc programme, while they will continue to develop this thesis in term 4 of the MArch programme. This submission is solely by me, and any maps or writing completed as a group in previous terms will be properly credited.
would like to express my deep gratitude to our tutors, especially our thesis advisor, Clara Oloriz, who guided us with her generous support and expertise in achieving this thesis.
am also grateful for the opportunity provided by the British Geological Survey (BGS) to explore new ways of rethinking their approach to achieving Net Zero through their much-needed refurbishment plan. A sincere thank you to all the professionals we were able to interview, as well as the external jurors, whose insights and experience in their respective fields shaped and expanded our understanding.
Lastly, would like to express my immense gratitude to my family for their constant support and for being a source of inspiration in many ways.
Cultivating Commons
A radical proposal to mobilise biobased materials production in the East Midlands, UK through Public Common Partnerships
by Priyanka Awatramani
CULTIVATING
[kal.ti.veit.ing] verb.
The intentional and continuous process of nurturing and developing landscapes of production. It embodies the act of fostering growth, not only in agricultural terms but also in the broader sense of encouraging regenerative practices, sustainable resource management, and community-led initiatives. Cultivating involves actively shaping and transforming environments -both natural and built- towards a more resilient and ecologically balanced future.
COMMONS
[kam.ens] noun.
Shared resources and benefits that emerge from collective stewardship and fair management of landscapes of production. It represents the surplus generated by regenerative practices, which is not owned by any single entity but is instead available to all members of a community. The concept of commons underscores the importance of local ownership, collaboration, and the balanced allocation of resources, aiming to create a sustainable and inclusive economy that serves both people and the planet.
by Alejandra Iturrizaga Andrich
As we grapple with the urgent need to address climate change and foster sustainable development, innovative solutions are emerging to reshape our relationship with the environment and economy. Our project, Cultivating Commons, seeks to explore the intersection of environmental stewardship, economic prosperity, and community well-being. Cultivating Commons recognises the interconnectedness of landscapes of production—encompassing both the natural and built environment—and their potential to shape regional ecologies while strengthening local economies.
By reimagining traditional models of production and consumption, Cultivating Commons aims to harness the power of regenerative practices and biobased materials to mitigate carbon emissions and reduce environmental impact. Cultivating Commons is dedicated to transforming the construction industry by advocating for the transition from extractive materials to biobased alternatives.
Cultivating Commons acknowledges the pivotal role of agriculture in shaping landscapes and livelihoods, particularly as biobased materials are cultivated and harvested within these environments. In recognising this connection, the project advocates for a transition from monoculture farming to regenerative practices. This shift not only facilitates carbon sequestration in the soil but also opens avenues for economic diversification and community empowerment.
At the heart of Cultivating Commons lies the principle of community wealth building, emphasising local ownership, equitable distribution of resources, and collaborative decisionmaking. Through engaging stakeholders at all levels – from farmers and builders to policymakers and community members – the project aims to foster a shared sense of stewardship over landscapes of production.
Cultivating Commons is a collective effort to “cultivate” landscapes of production that not only sustain people and the planet but also contribute to building a surplus shared as “commons” for a more equitable future. It aims to forge a path towards a more regenerative economy, where landscapes of production serve as catalysts for environmental regeneration, economic vitality, and community resilience.
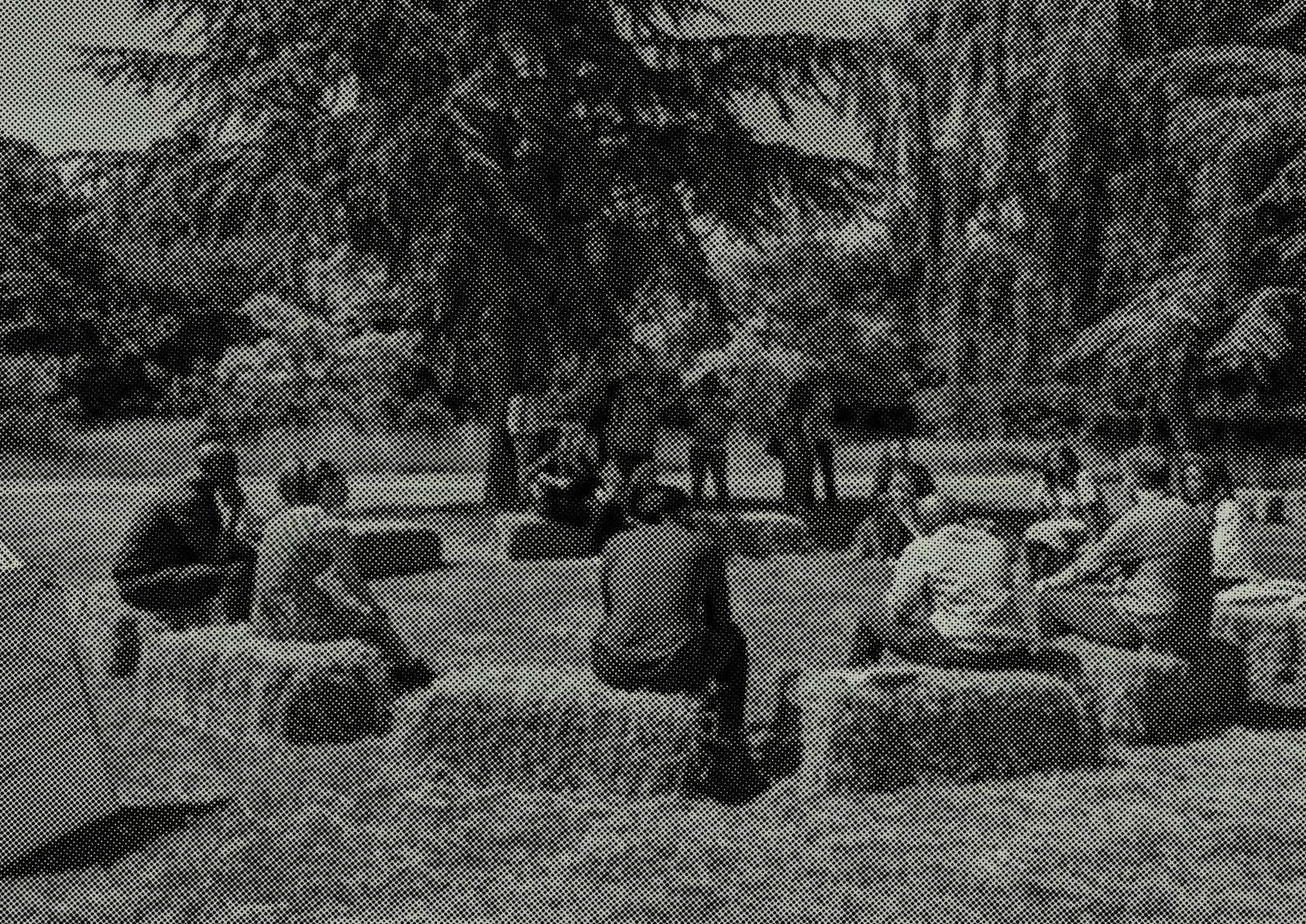
Study Overview, Methodology & Methods
BGS serves as a case study example to employ biobased material retrofitting; key-informant interviews draw expert advice and perspectives to inform the foundation of our work.
by Emily Bowerman
Approach
The research follows a case study approach to develop an in-depth analysis of the opportunities for retrofitting the British Geologic Survey campus in Keyworth, UK with bio-based materials. The research involves a mixed-method approach2 and will be undertaken over the period of February 2024 to January 2025 within the UK – our case being specifically within the bioregional boundaries of the East Midlands region. 3
Methods
Critical Discourse Analysis
Critical Discourse Analysis defined by Fairclough (1995) as, will be performed to understand the status of knowledge and policies related to decarbonisation initiatives in the UK; biobased material research, development, and use; and existing evidence of Community Wealth Building initiatives in the UK.4 The document analysis will contribute to initial literature reviews about decarbonisation, bio-based materials, transition to bio-economies andcommunity wealth building through public common partnships. The literature will inform a policy map to document the current pathways for achieving net zero, using bio-based materials in practice, and opportunities for alternative economic models through community wealth building approach.
Key-Informant Interviews
Existing conditions and status of current policies and bio-based material production industries will be graphically represented and mapped through visual representation methods. To understand the status of A. bio-based material production in the UK, use in the building industry and B. understand the status of agricultural industry in East Midlands we need to speak with experts in the field. We have identified 6-15 key informants who will be contacted for open and semi-structured interviews on the respective fields. We are exploring six core bio-based materials including clay, timber, straw, hemp, mycelium, and seaweed. To ensure a balanced understanding of each material, we intend to engage with the same number of experts for each material. The purpose of selecting keyinformants who are already engaged in the topics mentioned, is to avoid any knowledge extraction from local communities, farmers, manufacturers, contractors, or stakeholders who may not benefit from the project; key Informants will be selected based on their prior engagement in research projects or initiatives. Participation will be entirely voluntary (this is elaborated in the following section). We anticipate that the interviews may be conducted intermittently throughout the project development phase (February 2024-June 2024), based on participant availability. Some may be re-contacted (if they are willing) to further develop ideas discussed in preliminary interviews. Moreover, as the project develops, iterative feedback and guidance from experts will be beneficial to ensure the project reflects the current status of knowledge in the field.
The study involves site visits to contextualise academic and grey literature from the Critical Discourse analysis and capture existing conditions with use of camera and video. The purpose of the project is to propose a policy for bio-based materials that have land-based implications, therefore, conducting a landscape inventory analysis in the east midlands region is essential. Similarly, several organisations across the UK and Europe have tested and are testing bio-based materials, therefore, site visits could involve visiting test sites or workshops to better understand material use.
Data Analysis
Following the Critical Discourse Analysis, Key-Informant interviews, and Site Visits will perform triangulation to gain a comprehensive understanding of phenomena.5 We will be evaluating the potentials for each bio-based material in the UK and the East Midlands through a viability assessment. This will account for factors including material production, manufacturing, use in buildings, architectural potentials and most critically, opportunities for local communities to engage in the transition toward a bioeconomy.
Upon triangulating the data, it will be combined with visual research methods to represent the policy implications within the East Midlands region. Moreover, a design strategy will be proposed for the BGS campus in Keyworth. This will be reviewed and iteratively revised with members of the BGS to ensure the design reflects their current needs.
Sampling
The Key-Informants will be identified through purposive sample according to their expertise in the following three topics: decarbonisation of the construction industry in the UK; biobased material use or research and development; and knowledge of Community Wealth Building, Public Common Partnerships (PCP) or bioregional economics. Key-Informants will be approach via email and will be asked for their willingness to engage in a brief conversation regarding their expertise on a particular topic. Participation will be entirely voluntary with no incentive or deception. Consent to participate in the open and semi-structured interviews will be acquired prior to participation.
Data Dissemination
The information will be disseminated in two AA Landscape Urbanism Thesis documents. Firstly, the MSc in Landscape Urbanism by Alejandra Iturrizaga Andrich in September 2024, and by Priyanka Awatramani and Emily Bowerman, January 2025. If the information within the thesis were to be used for publication or for a conference, we would ask permission from the British Geologic Survey and the Key-Informants to ensure all information is represented as per their intent.
1. Wang, David, and Linda N. Groat. Architectural Research Methods. Hoboken, NJ: John Wiley & Sons, 2002; Robert K. Yin, Case Study Research: Design and Methods (Thousand Oaks, CA: Sage Publications, 2013).
2. Creswell, John W, and David Creswell. “Research Design: Qualitative, Quantitative, and Mixed Methods Approaches,” n.d.
3. Robert E. Stake, The Art of Case Study Research (Thousand Oaks, CA: Sage Publications, 1995); Robert K. Yin, Case Study Research: Design and Methods (Thousand Oaks, CA: Sage Publications, 2013).
4. Fairclough, Critical Discourse Analysis: The Critical Study of Language (London: Longman, 1995), page number.
5. Michael Q. Patton, “Enhancing the Quality and Credibility of Qualitative Analysis,” Health Services Research 34 (1999): 1189-1208.
Achieving Net Zero in the UK
Addressing Operational and Embodied Carbon Emissions and the British Geological Survey (BGS) as a case study.
by
In 2018, the UK emitted 1511 million tonnes of carbon dioxide equivalent (MtCO2e) Green House Gas (GHG), encompassing territorial, production, and footprint emissions as per the Office for National Statistics. With the Climate Change Act 2008 the UK government in 2019 set a target for achieving a 100% reduction in Greenhouse Gas emissions by 2050 known as the Net Zero Target. 2
The construction industry plays a significant role in these emissions, accounting for approximately 40% of the UK’s total carbon footprint, as estimated by the UK Green Building Council. However, most decarbonisation efforts within this sector have primarily focused on reducing “operational carbon,” which pertains to emissions from a building’s energy consumption for heating, cooling, and lighting. However, the clean energy transition is questionable. As cited by Dark Matter Labs in their New European Bauhaus lighthouse project, “solar and wind facilities require up to 15 times more concrete, 90 times more aluminum, and 50 times more iron, copper, and glass than fossil fuels or nuclear energy”.3 It is also estimated by the Mining Watch Canada that approximately three billion tons of metals and minerals will have to be mined to facilitate the energy transition. Additionally, inefficiencies in insulation and design contribute to high energy consumption for heating and cooling in buildings, particularly in the UK.
There are 80 UK Research and Innovation (UKRI) institutions that may require retrofitting to enhance energy efficiency, improve interior conditions, and achieve Net Zero, with the highest concentration of them in the southeast of England. The British Geological Survey (BGS) is among these institutions and has approached us to explore new alternatives for meeting these goals. In their efforts to align with the UK’s target of a 100% reduction in greenhouse gas emissions by 2050, BGS is focusing on retrofitting four of its buildings and has engaged the consultancy firm Pick Everard to provide a comprehensive analysis of the refurbishment. This analysis evaluates factors such as cost, function, and carbon emissions but does not address emissions related to the materials used and their sourcing. This thesis aims to complement this work by incorporating material-related emissions into the evaluation. Additionally, it can serve as a case study that other institutions can reference to address these crucial aspects in their own retrofitting efforts and the path towards Net Zero.
80 UKRI institutions retrofitting for Net Zero emissions 100% reduction in Green House Emissions. Net Zero target set in 2019.
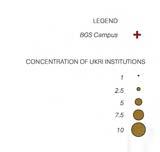
1. “Emissions,” accessed April 16, 2024, https://climate-change.data.gov.uk/dashboards/ emissions.
2. “Net Zero Government Initiative: UK Roadmap to Net Zero Government Emissions,” n.d.
3. “New-European-Bauhaus-Economy_Digital-version_DML.Pdf,” accessed February 18, 2024, https://www.irresistiblecircularsociety.eu/assets/uploads/20230707-New-European-BauhausEconomy_Digital-version_DML.pdf.
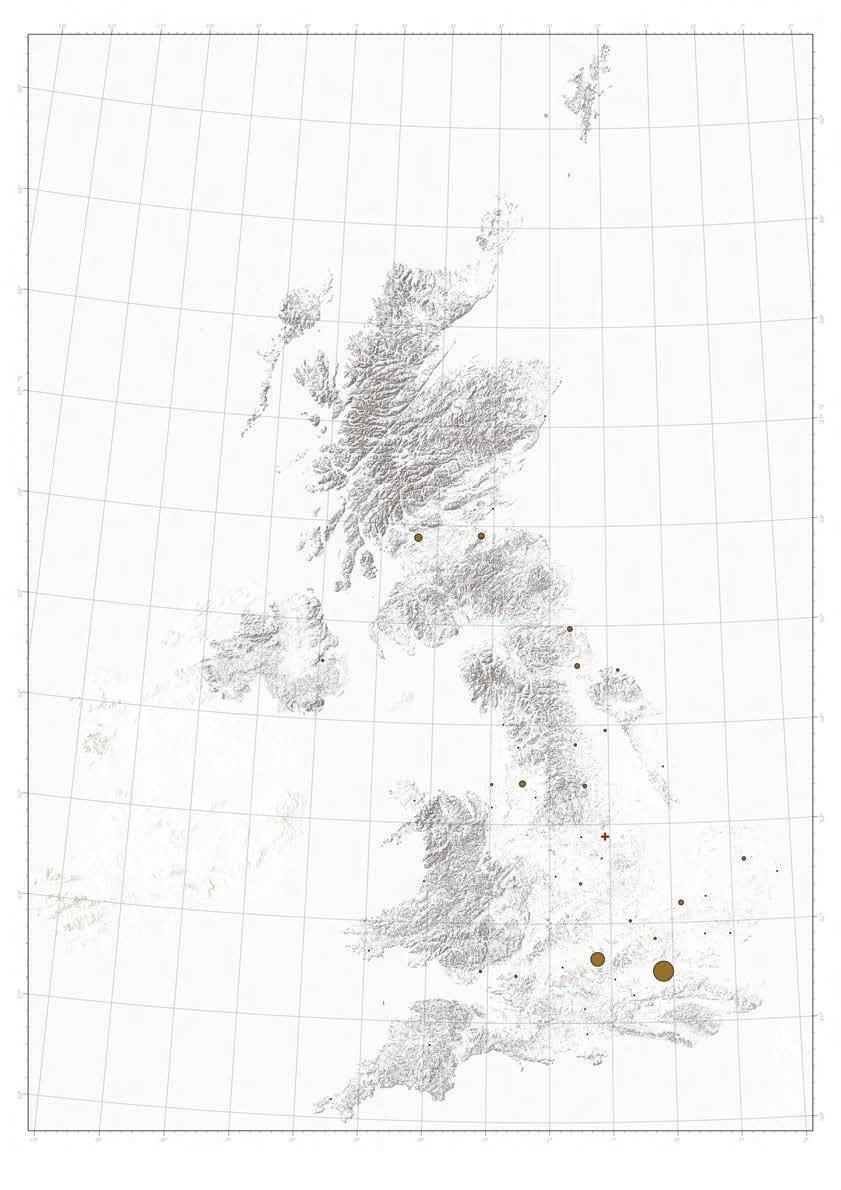
Alejandra Iturrizaga Andrich and Priyanka Awatramani
100km
defining regional scale
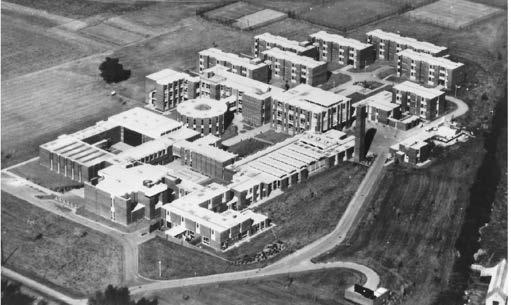
British Geological Survey (BGS)
6.
British Geological Survey (BGS)
by Alejandra Iturrizaga Andrich
The British Geological Survey (BGS) is a pillar of geological research and scientific advancement. It is the world’s oldest national geological survey, founded in 1835 as the Ordnance Geological Survey by Thomas F. Colby and Henry Thomas de la Beche, the BGS mission was to systematically survey and understand the geological landscape of the United Kingdom. This culminated when the Museum of Economic Geology was created in 1835, which served as a hub for collecting and studying geological specimens.6
Over the years, the BGS expanded its scope and influence, playing a crucial role in shaping the scientific landscape of the UK and beyond. The Geological Survey Act in 1845 provided the legal framework necessary for the survey’s continued growth and development. Afterwards, the establishment of the School of Mines in 1851 and the separation of the Geological Survey and Museum from the Royal School of Mines in 1872, underscored the BGS’s commitment to advancing geological education and research. 6
In 1965, the BGS underwent a significant transformation with the passage of the Science and Technology Act, leading to the creation of the Institute of Geological Sciences (IGS). This evolution marked a new chapter in the BGS’s history, solidifying its position as a world-leading geological survey and research institution. In 1984, the organisation was officially renamed the British Geological Survey, reflecting its expanded mandate and growing influence in the scientific community. 6
Today, the BGS continues to uphold its legacy of excellence and innovation, with cutting-edge technologies and interdisciplinary approaches to address geological and environmental challenges. As part of UK Research and Innovation (UKRI), the BGS remains at the forefront of public-good science, providing invaluable geoscientific data and expertise to support informed decision-making and sustainable resource management for all. With a constant commitment to scientific excellence and public service, the BGS remains at the top of geological knowledge and exploration, shaping our understanding of the Earth’s past, present, and future.
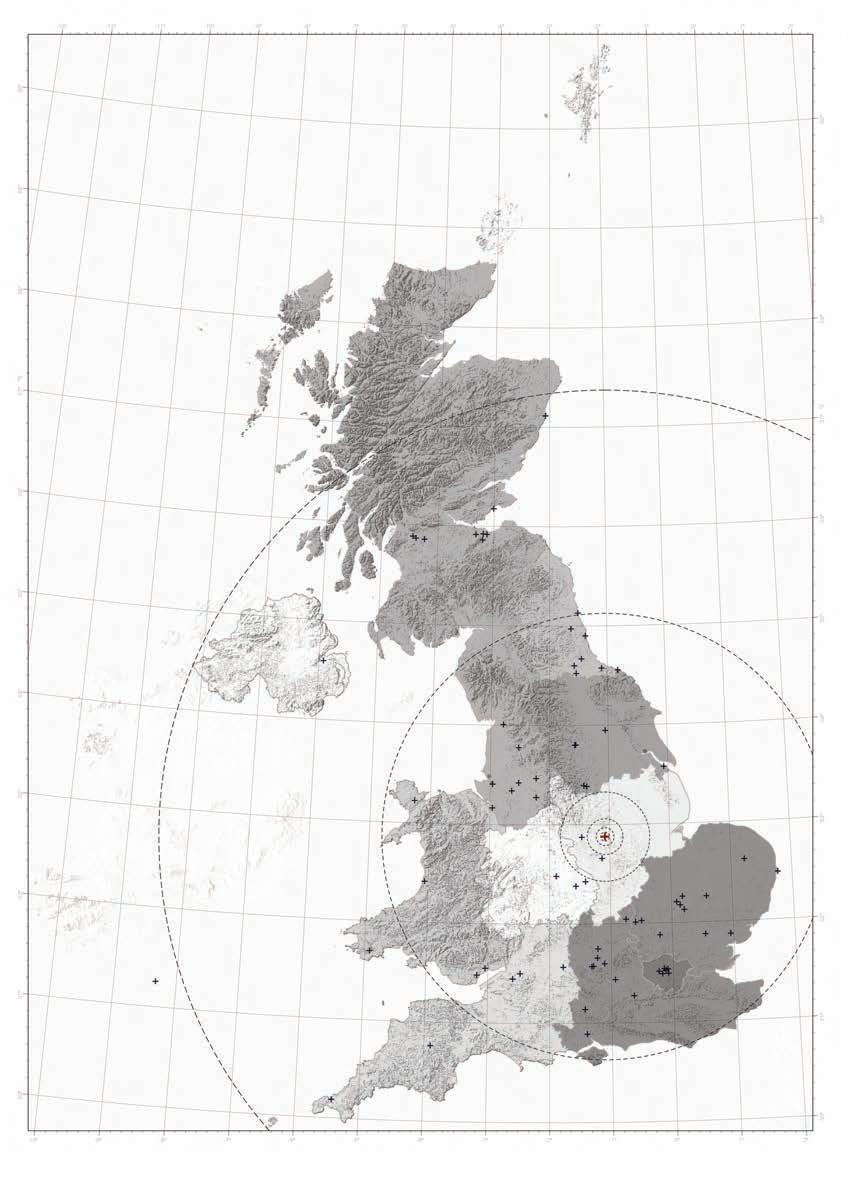
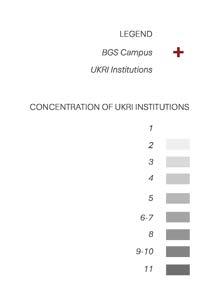
Pick Everard’s Retrofitting Proposal
The proposal only accounts for reducing carbon emissions of the operational energy through maintenance and alternative energy sources.
by Alejandra Iturrizaga Andrich
Pick Everard was commissioned to conduct a thorough review of the British Geological Survey (BGS) building, focusing on potential refurbishment or new construction options for the laboratory facilities. In response to BGS’s requirements for short and long-term maintenance, net-zero aspirations, energy decarbonisation, and adaptable working methods, Pick Everard proposed distinct outcomes. These options were meticulously analysed to address the need for phased strategies, storage solutions, and improvements to workspace design. With most of the existing building fabric requiring replacement within the next decade, Pick Everard’s assessment also considered the structural integrity and longevity of the facilities. Following a comprehensive evaluation, they identified five viable paths forward for the BGS building.7
Cons: Long-term maintenance impacted.
flexibility. Limited improvement in workspaces. Option 2: Partial Refurbishment
Pros: Less schedule impact. Achieves degassing of site. Low impact on electrical capacity.
Cons: Limited long-term benefits. Disruptions during refurbishment. Limited scope for new science.
Option 5: Hybrid
Pros: Flexibility of spaces. Position as world-class leaders. Positive impact on
Sites of Conventional Building Materials
Decarbonising the construction industry is essential to reduce UK’s overall emissions
by Priyanka Awatramani
IRECIPROCAL LANDSCAPES OF UK BUILDING INDUSTRY
Bridging the gaps between biobased construction materials (non-sites) and landscapes of production
Addressing the challenges of the construction industry requires a focus on reducing our energy consumption and adopt practices that make our buildings more resilient to the climate. This would entail critically addressing the contribution of the “embodied” carbon emissions in the construction industry. As stated by ACAN in the Briefing Note of Carbon Footprint Construction, embodied carbon emissions account for up to 75% of a building’s total emissions over its lifespan.4 These emissions are associated with materials and processes throughout the entire lifecycle of a building, including extraction, processing, and manufacture of materials; transportation; assembly and installation on-site; as well as replacement, refurbishment, maintenance, demolition, and disposal.
Most conventional building materials like cement and stone are non-recyclable materials, meaning that at the end of their life-cycle they just go into the landfills, creating pressures on waste management. These extractive and disposal processes not only contribute to carbon emissions but have a detrimental impact on the environment including natural resource depletion and waste generation, harming ecosystems. The global resource extraction and processing currently accounts for more than 35% of global biodiversity loss and water stress.5 Despite their substantial contribution to overall emissions, strategies aimed at reducing embodied carbon have often been overlooked. This stems from traditional linear approaches to building design and operation which overlook the interconnectedness of various systems within a building. Building materials and structures, often perceived as durable, are actually “designed for obsolescence.” Rapid urban development exacerbates these challenges, involving significant land use changes and extraction of natural resources, leading to habitat destruction and increased carbon emissions.
Shifting towards a holistic view that considers interactions between material, energy, water, waste, and occupants is essential. Decarbonisation efforts must extend beyond solely
reducing carbon emissions to encompass broader environmental factors such as water usage, material sourcing, and waste generation. This propels an urgency to “transition” towards regenerative materials, prioritising ethically produced, low-carbon, biobased building materials that can significantly reduce dependence on conventional materials such as concrete, steel and aluminum. These curb carbon intensive extraction and production systems, advancing in decarbonisation efforts.
Improved building standards and retrofitting existing structures can further aid in reducing energy reliance and enhance building resilience. Embracing circular economy principles, such as resource reuse, recycling, and regeneration, is crucial. By integrating these considerations into building design and construction practices, we can mitigate environmental degradation and promote sustainable development within the construction industry. Moreover, decarbonisation efforts offer numerous benefits beyond mitigating climate change, including better health, improvement in quality of life, shifts towards circular economies, biodiversity and habitat preservation, and disaster resilience. Ultimately, this holistic approach enables the creation of healthier, more resilient communities while addressing the urgent challenge of climate change.
UK Landscapes of Extraction
by Priyanka Awatramani
“The sites and non-sites were linked by material displacement from one to the other, but also through their differences. Sites were peripheral, overlooked spaces that supplied materials for urban development, while non-sites were central concentrations of cultural capital.”7
Through the lens of Jane Hutton’s Reciprocal Landscapes, we examined the material supply chains within the UK’s construction sector. In this framework, the construction sector’s reliance on material extraction sites as “sites” and the built environment as “non-sites” becomes apparent. UK’s construction sector is a critical component of the nation’s economy; the industry contributes significantly to carbon emissions and landscape degradation due to heavy reliance on fossil fuels and machinery.
We mapped these extraction sites in two categories: bedrock reserves reflecting geological resources and stone quarries along with superficial deposits representing available aggregate resources. According to the BGS’ Mineral Planning factsheet, there are 439 active building stone and slate quarries, with concentrations particularly evident in regions such as the Midlands, South-West, and Northern Ireland. The East Midlands hosts an abundance of limestone resources, with numerous cement factories spread around these resources.
Sand and gravel, essential components for construction, are sourced through various means, including crushing sandstone and extraction from superficial deposits. These aggregates, comprising approximately 85% of non-energy minerals extracted in the UK, are procured from over 1300 quarries across the UK, as well as through marine aggregate dredging. The heatmap reveals the highest concentration of quarries in regions like the South-East, Midlands, and East Scotland highlighting an abundance of reserves in these areas. Due to the scale of this industry, the production totalling approximately 260 million tonnes annually, remains labour-intensive, providing employment for an estimated 88,000 individuals, both directly and indirectly.
This insight into the scale and spatial dynamics of material extraction and production in the UK, within the framework of reciprocal landscapes becomes crucial for informed decisionmaking regarding resource management and environmental sustainability.
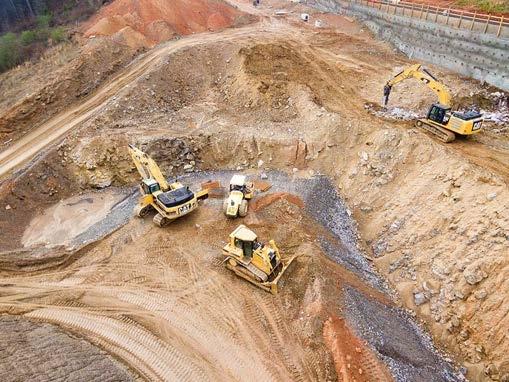
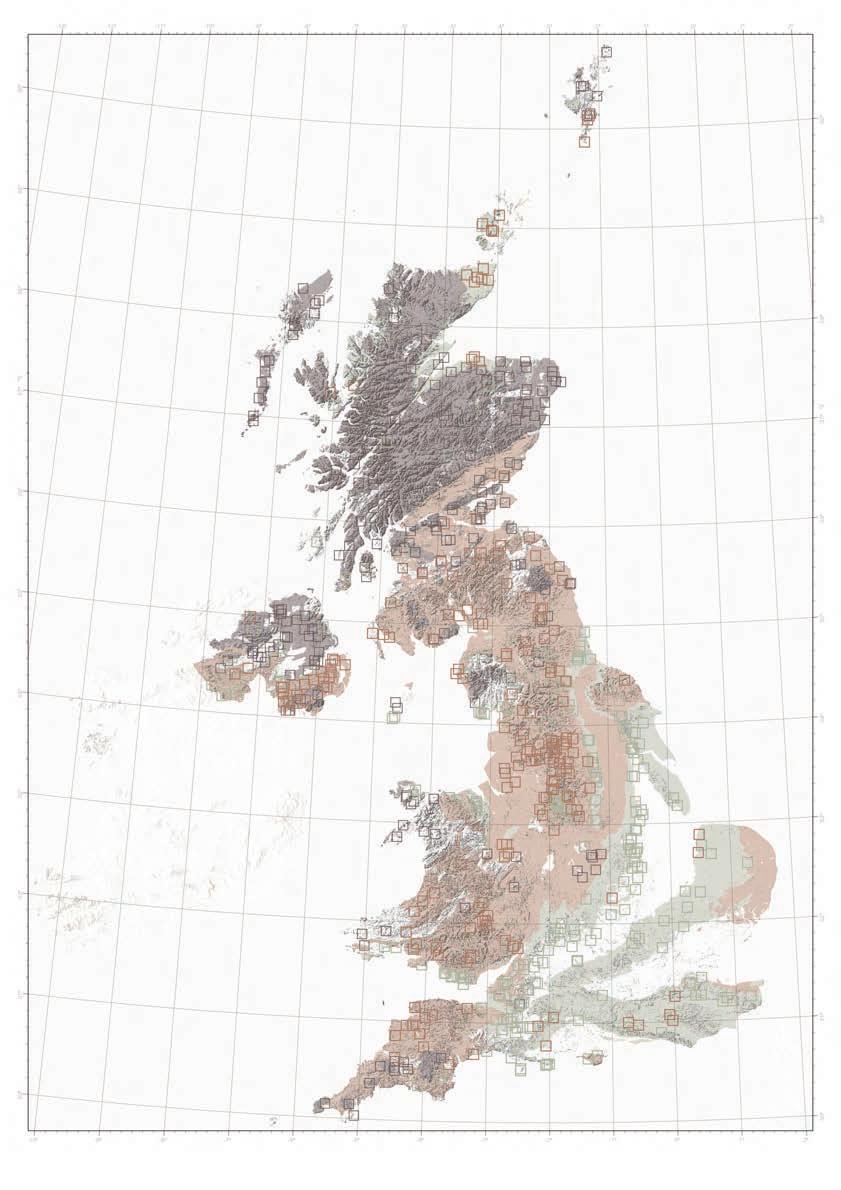
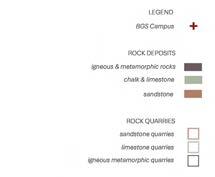
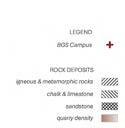
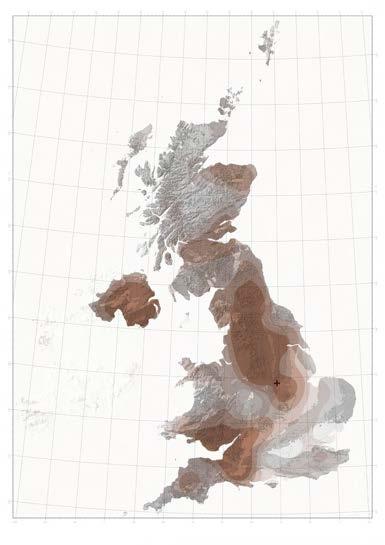
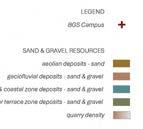
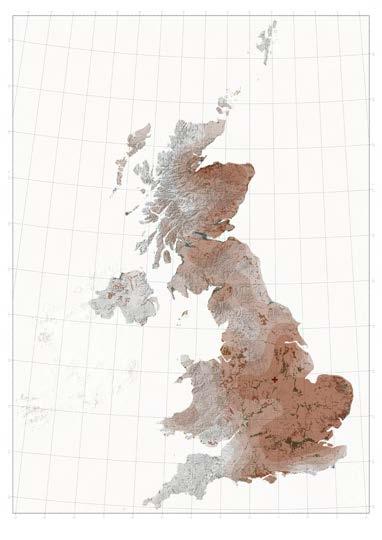
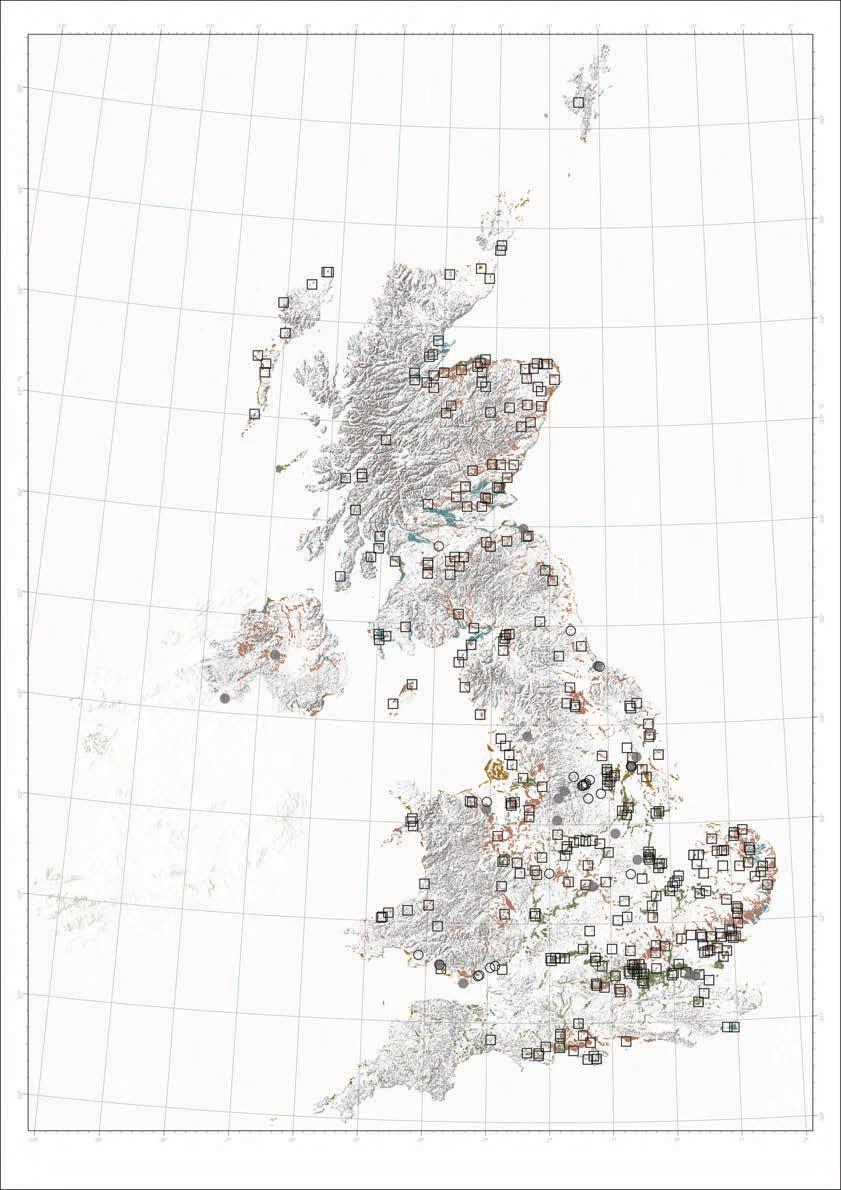
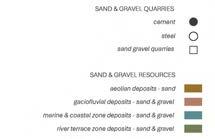
Construction Minerals Global Imports
by Priyanka Awatramani
Despite the significant extraction of construction materials within the UK, a substantial portion of the carbon footprint associated with the construction industry stems from the imports of materials. The UK is a net importer of crucial construction materials such as cement, iron, and stone from various regions worldwide.9 This reliance on imports introduces complexities into the carbon accounting of the construction sector, particularly as these materials traverse vast distances, often from disparate continents.
Iron ore, for instance, is sourced from five different continents, highlighting the global nature of material supply chains and the interconnectedness of the construction industry with international markets. This dependence on imported materials is visually represented through the depiction of emission rings surrounding the globe, illustrating the total emissions linked to the imports of these materials into the UK.
While the UK’s extraction sites contribute to domestic supply, the industry’s increased demands and globalisation have normalised the use of materials produced from across the globe. The design and construction sectors, driven by factors such as cost considerations, availability, quality standards and aesthetics, have resulted in the sourcing of construction materials from across the globe. This trend has led to the establishment of complex networks of extraction, transportation, and distribution, amplifying the carbon footprint associated with the construction industry.
Moreover, the imports of construction materials affect additional environmental and social considerations beyond carbon emissions. Issues such as habitat destruction, land degradation, and labour exploitation are often linked to the extraction and production of materials in other countries, making it crucial to incorporate holistic assessments in construction material procurement. To demonstrate where these impacts might be most severe, the globe displays the domestic extraction of construction materials by each country along with locations of major extractive sites. It is therefore critical that the addressing the carbon footprint of imported materials adopts a multifaceted approach that considers not only the direct emissions associated with extraction and transportation but also the broader environmental and social impacts throughout the supply chain.
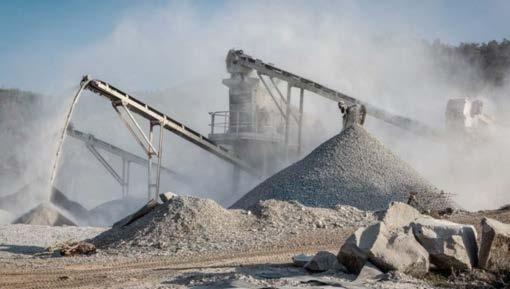
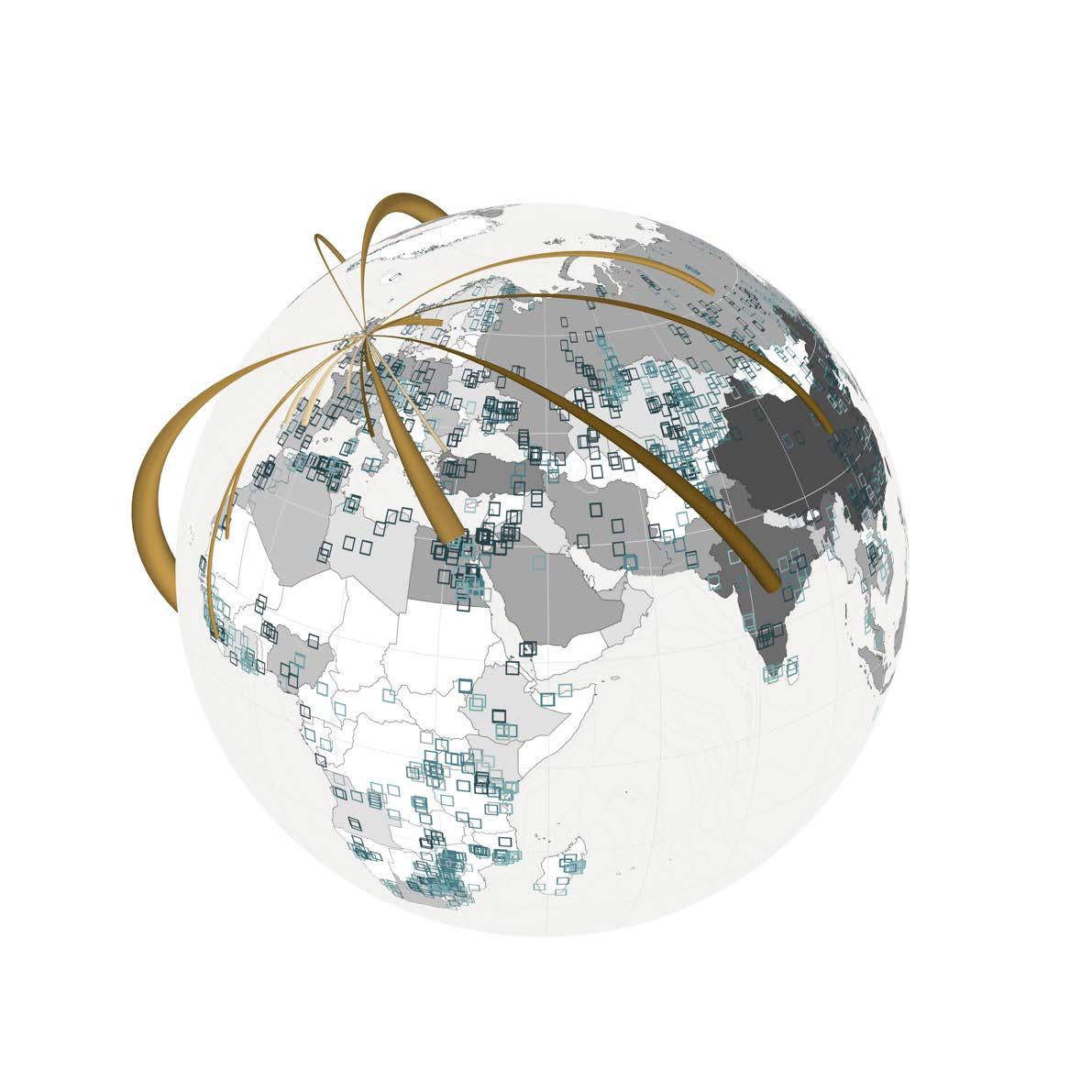
Waste in the construction industry
Construction and Demolition wastes end up in landfills contributing to landscape and soil degradation
by Alejandra Iturrizaga Andrich
The UK faces a critical challenge, with 42% of its landfills already reaching capacity,10 emphasising the urgency for sustainable waste management solutions. As per DEFRA’s statistics of 2016, 62% of all UK waste comprises demolition and excavation waste,11 highlighting the need for a shift towards a cradle-to-cradle approach. This approach aims to ensure that materials harvested can return to the land through composting sites, rather than ending up in a landfill.
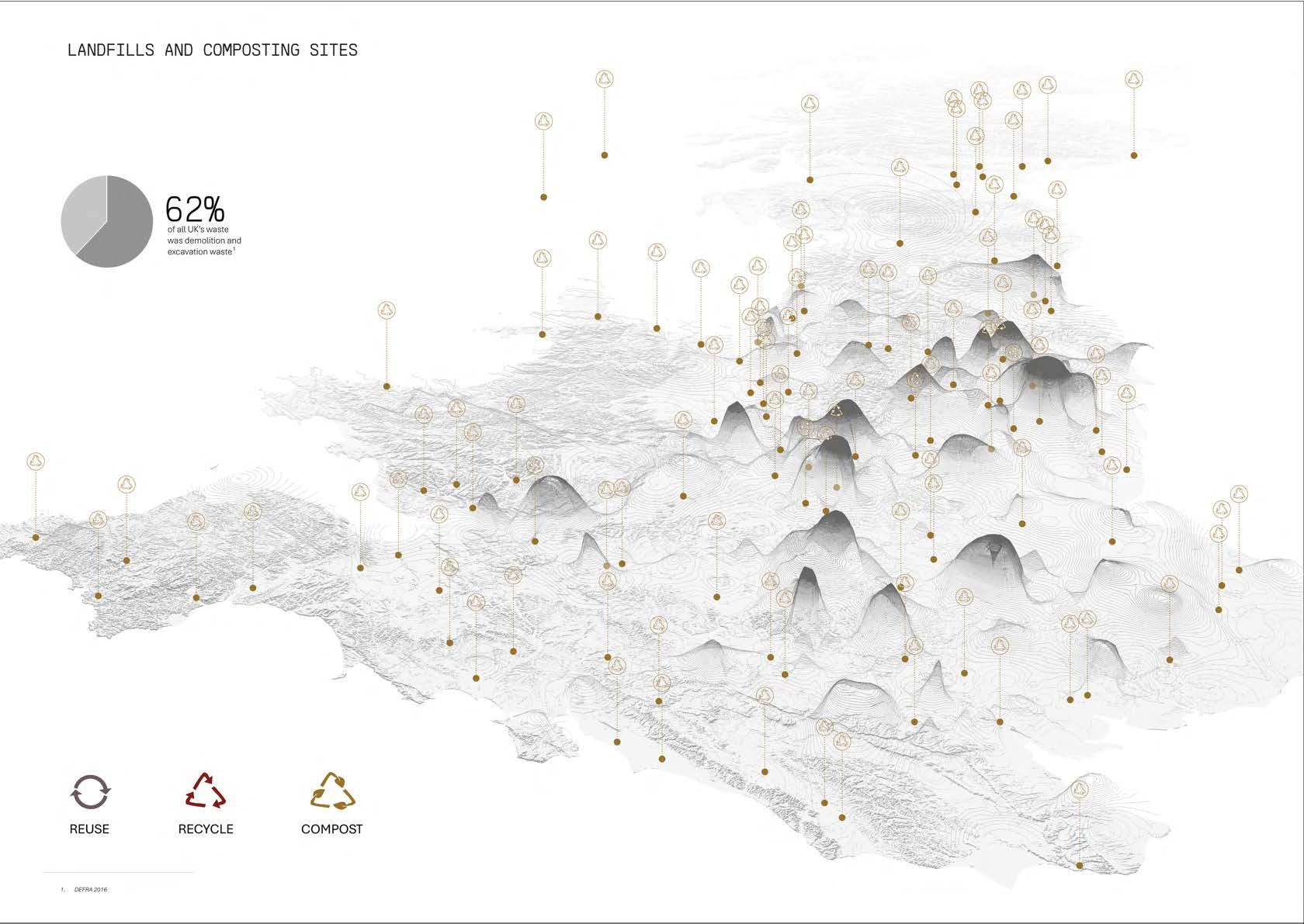

Biobased materials
Biobased materials which are responsibly sourced from the earth, have regenerative benefits, have low embodied energy , a continuous lifecycle and some offer carbon sequestration.
by Alejandra Iturrizaga Andrich
BIOBASED MATERIALS
Buying into biobased; how building materials can be designed for broader landscape sustainability
The landscapes of extraction and architecture are intricately linked, prompting a need to reconnect design with landscape and agricultural practices. Building on the concept of Material Cultures, the initial drawings when designing a building should carefully consider the selection of materials and their potential impact on people, the economy, and environmental factors such as climate and ecology.1
Around the world, landscapes are being shaped by extraction activities. Understanding this is crucial, especially considering that the typical lifespan of modern buildings is around 50 years.
As said earlier, 62% of all UK waste consisted of demolition and excavation waste. 2 In response, contemporary construction should aim to be “designed for a post-waste economy where all components can be reused, recycled, or composted,” as proposed by Material Cultures in their book Material Reform.
These resources must be regenerative. Materials harvested from forests or agricultural lands should be managed sustainably, considering the quantity and characteristics of each material regionally to prevent depletion and soil erosion.
Biobased materials offer sustainability throughout their lifespan. From planting to use in construction, and even after demolition, they can be composted back into the land. Additionally, they contribute to carbon sequestration and can act as carbon sinks when planted, grown, and used as a material.
The performance and benefits of materials should not only be based on their behaviour in the built environment but also on the footprint they leave before arriving on site. 11% of worldwide carbon emissions come from their manufacturing.3 Locally harvested biobased materials lend identity to the design of buildings, aligning them with their surroundings and connecting them with the unique qualities that compose each region.
France has been at the forefront of promoting bioconstruction practices, exemplified by the “Guide matériaux biosourcés et commande publique” published in April 2020. It reflects the
country’s leadership in promoting environmentally friendly building practices. This guide reveals France’s commitment to encouraging the use of bio-sourced materials in public buildings, aligning with sustainability goals and regulatory frameworks. While not mandatory, the integration of bio-sourced materials is actively encouraged and supported through various regulations, guidelines, and incentives, emphasising their environmental performance, economic benefits, and contribution to sustainable construction practices. Public authorities are urged to prioritise these materials to align with environmental objectives, promote local economic development, and enhance the overall sustainability of construction projects. Incentives such as subsidies, regulatory support, financial assistance, capacity-building programs, and networking opportunities further promote the adoption of bio-sourced materials in public procurement and construction projects. France’s support for specific farming practices that promote the production of bio-sourced materials enhances the availability, quality, and environmental performance of such materials. The collaborative effort behind the guide, led by the French Ministry of Territorial Cohesion and the Ministry of Ecological and Solidarity Transition, reflects the government’s dedication to promoting sustainable construction practices and bio-sourced materials in public procurement strategies. 4
France’s proactive stance on bioconstruction serves as a valuable example for nations aiming to promote sustainable construction practices and the use of bio-sourced materials.
The UK can learn from France’s initiatives and collaborative efforts in advancing environmentally friendly building solutions. By adopting similar measures and leveraging lessons learned from France’s experience, the UK can enhance its own efforts to promote sustainability in construction and public procurement. This exchange of knowledge and best practices contributes to a global movement towards a more sustainable built environment, emphasising the importance of cross-border collaboration in addressing environmental challenges.
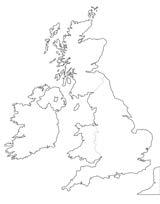
Biobased Materials Viability Framework
by Priyanka Awatramani
Shifting from conventional materials to biobased requires a strategic approach. How do we know which material to choose? And if it is feasible? Understanding existing opportunities of biobased materials in the UK, cultivation or sourcing processes, licensing requirements, investment needs and production capacities is vital. This entails assessing growing conditions, regulatory frameworks, and logistical aspects. This is essential to leverage the potential of alternative building materials effectively.
This framework comprises of five key studies:
1. Biobased Material matrix
This matrix lays out the logistical aspects of setting up a biobased industry in the UK in a zine format. This includes factors such growing conditions, required labour and resources, process of cultivating sourcing, the expected yield, and key policies and stakeholders that affect the industry. These insights are crucial to assessing the feasibility of biobased industries in the UK.
2. Biobased Industries and Opportunities
Mapping the current landscape of biobased industries in the UK involves identifying farmed areas, producers, distributors, and processing facilities. Additionally, factors such as favourable areas and protected areas are recognised for expansion or adoption of biobased industries. This highlights the opportunities existing within the biobased sector.
3. Building Material Performance Table*
The performance characteristics of biobased building materials is assessed in a simple tabular format. Factors such as compressive strength, thermal and acoustic performance, fire and moisture resistance, and pest resistance are laid out for available or potential forms of building materials developed out of biobased materials. This informs decision-making regarding material selection while ensuring compliance with existing codes and guidelines.
4. Embodied Carbon for Potential Building Materials*
As stated before, the embodied carbon of building materials is often overlooked in decarbonisation efforts. A visual summary of embodied carbon of biobased building materials versus conventional building materials highlights the environmental benefits of biobased alternatives, aiding in informed decisionmaking.
5. Biobased Regional Summary Map
This map ranks each biobased material based on their availability and the exapansion opportunities they present for each region in the UK. This serves as a valuable tool for the UKRI institutions in decision-making, offering insights into material availability and potential for retrofitting.
These studies and summaries highlight two significant things for the project. Firstly, due to its unique conditions, UK demonstrates vast potential for most types of biobased materials. Secondly, certain biobased industries like that of clay and timber are more established compared to emerging industries like seaweed and hemp. Thus, a nuanced approach tailored to each industry’s maturity level is essential to effectively drive biobased initiatives forward.
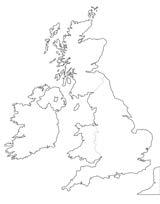
Biobased Material Evaluation
As part of our material evaluation for the retrofitting project at the British Geological Survey (BGS), we created six Zines focusing on biobased materials: Seaweed, Straw, Mycelium, Timber, Clay, and Hemp. Each Zine provides comprehensive information on the growing conditions, relevant policies, stakeholders involved, harvesting processes, and raw materials required for each type. Additionally, they cover environmental benefits, yield, and species available in the UK. This exercise aims to support the decisionmaking process for stakeholders involved in sustainable building practices, including architects, builders, policymakers, and researchers.
Find them in the pockets on the back of the book.
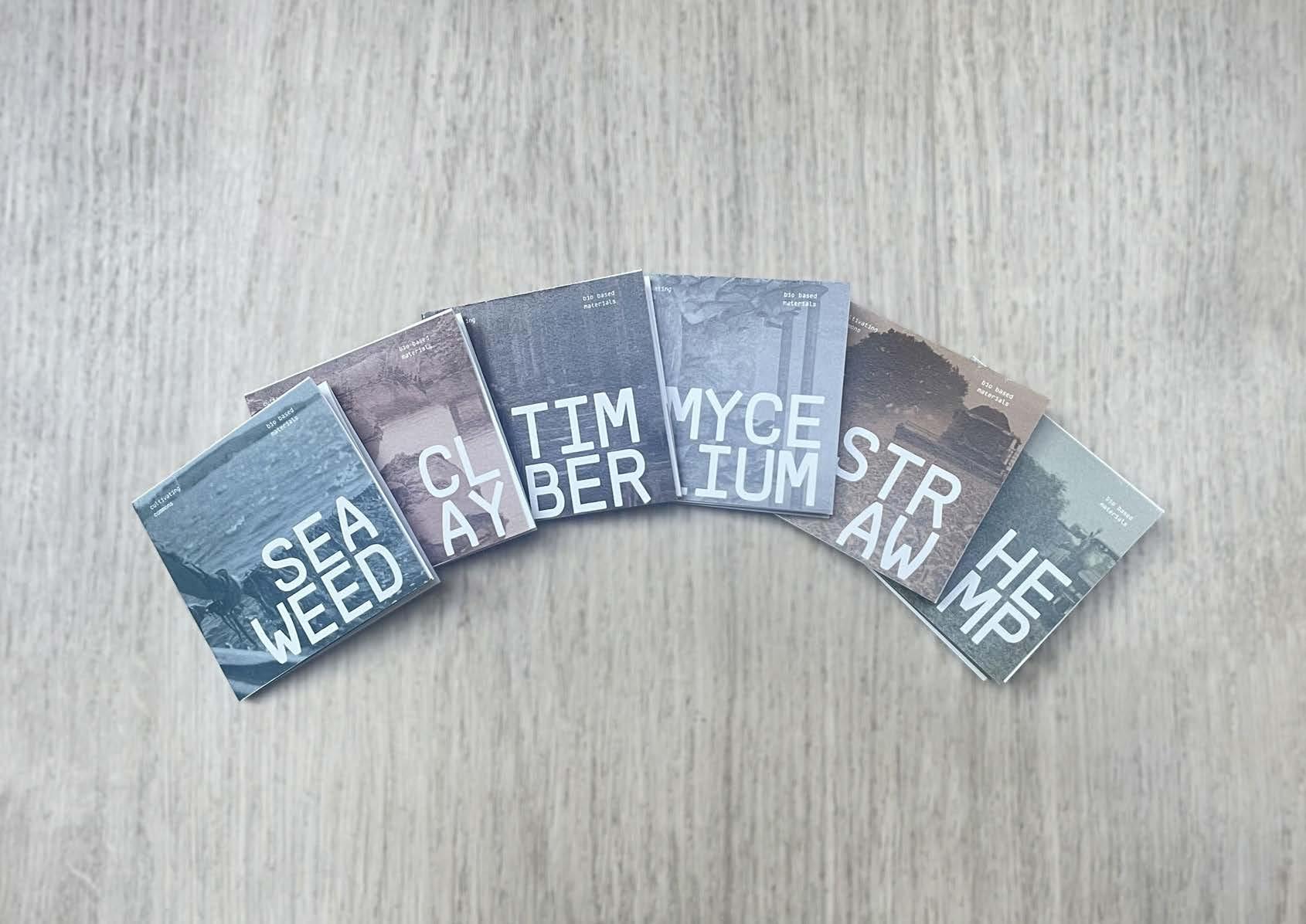
SEA WEED
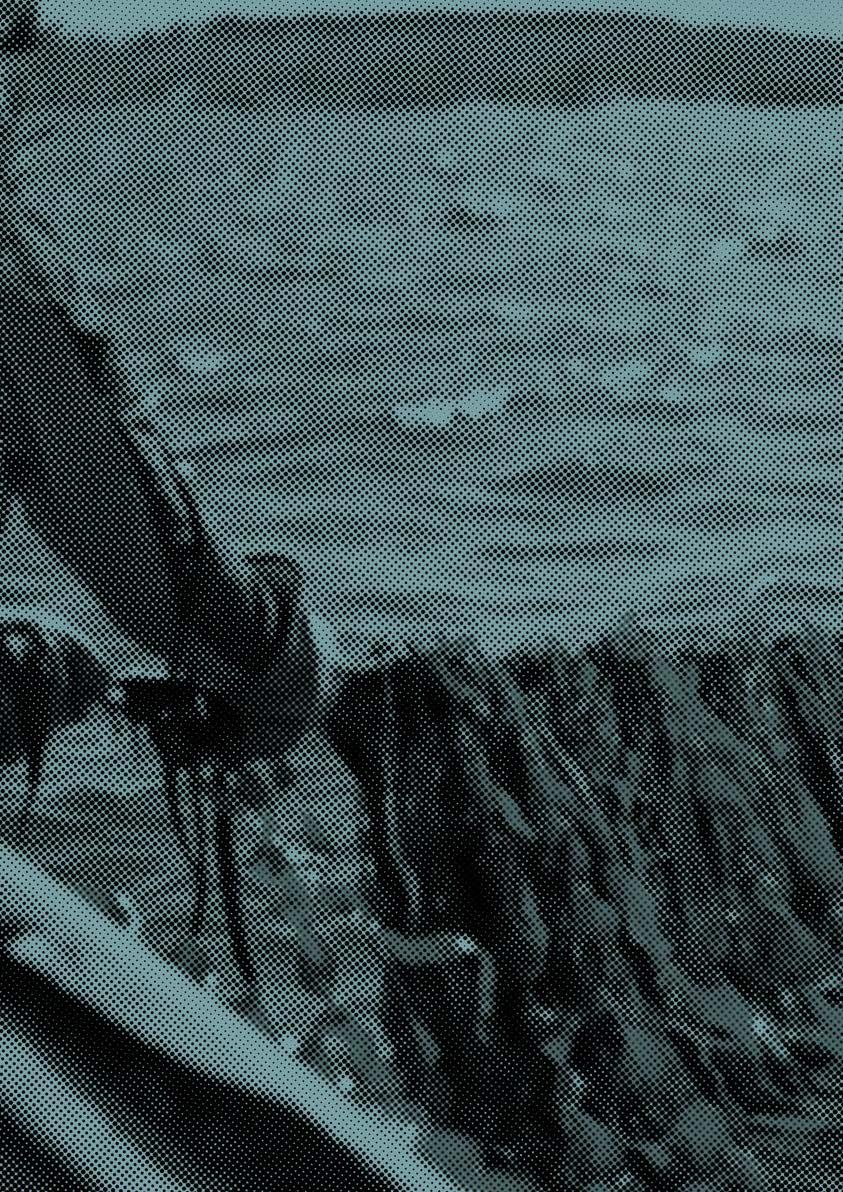
Biobased material matrix
13 marine licenses
issued for commercial seaweed farming in the UK
15,000 tonnes
(wet weight) of seaweed was harvested in the UK by some estimates
Seaweed
Industry & Opportunities
by Alejandra Iturrizaga Andrich
A new wave of sustainable acquaculture is emerging in the UK - Seaweed farming. While historically dominated by shellfish and fish farming, recent attention has shifted towards seaweed cultivation due to its rich nutritional content, pharmaceutical potential, and versatility in applications such as fertilisers, animal feed, and potential use as a construction material.
Insights gathered from industry experts like Allie Wharf from Norfolk Seaweed shed light on the progress of pilot seaweed farming projects along UK’s coast. Combining this firsthand knowledge with our desktop research, we have mapped potential opportunities for scaling up seaweed cultivation in the UK.
Our mapping process considered various environmental and social factors influencing the feasibility and sustainability of seaweed farming. Data included wave patterns, port accessibility, ocean floor depths, fishing vessel traffic, marine protected areas, and synergies with offshore wind farms. By analysing these factors, we identified regions with optimal conditions for seaweed cultivation while minimising potential conflicts with existing marine activities and conservation efforts.
For instance, the presence of ports in regions like the East Midlands signifies logistical advantages for seaweed farming operations, facilitating transportation and market access. Coastal communities were mapped as potential hubs for the workforce, recognising their proximity to seaweed farming sites and the potential economic benefits for local people. Furthermore, our mapping efforts aim to illustrate the importance of selecting sites with favorable wave patterns and minimal erosion susceptibility to ensure the viability and resilience of seaweed cultivation ventures. Eroded areas were targeted for seaweed cultivation to potentially attenuate waves and mitigate erosion, contributing to coastal resilience.
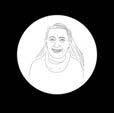
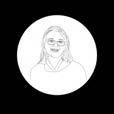
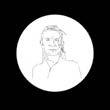
Pairing seaweed farming with planned offshore wind farms offers benefits, maximising resource utilisation and sharing ocean space. Mapping marine protected areas aimed to understand both the benefits and limitations of seaweed cultivation within these designated zones, ensuring alignment with conservation objectives. By considering these spatial dynamics, we aim to support the sustainable growth of the seaweed sector while conserving marine ecosystems and coastal communities. Ultimately, our efforts contribute to advancing the UK’s transition towards a more resilient and environmentally conscious aquaculture landscape to propel bio-construction.
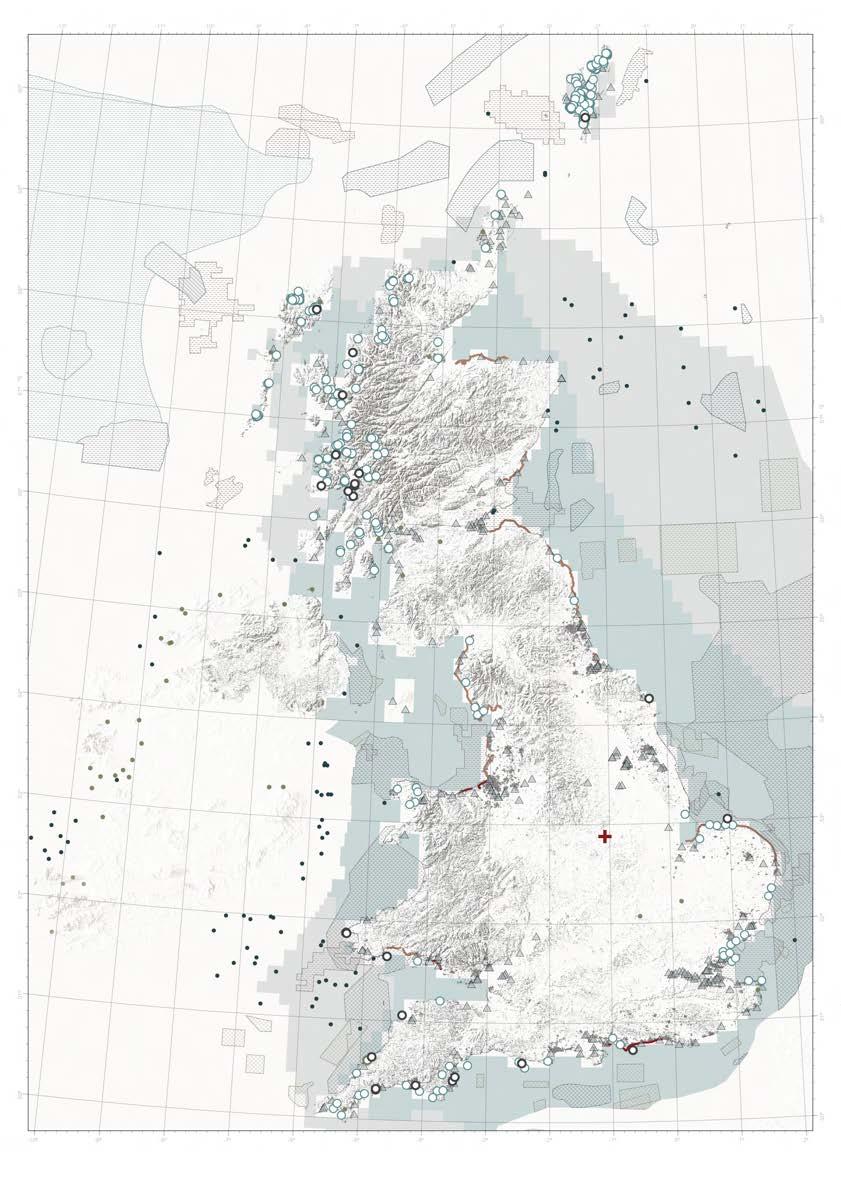
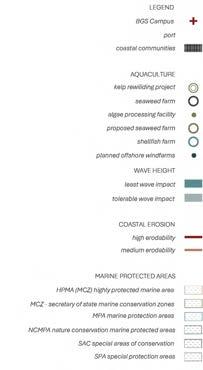
On February 23rd, 2024 we attended a site visit and interview with Allie Wharf
of Norfolk Seaweed. We visited her at the Morston Quay where her offshore seaweed farm is located in Norfolk.
Allie is a former producer and director at the BBC and is now a passionate and devoted seaweed farmer.
The following outlines some of the key takeways from our conversation.
Production
*NS = Norfolk Seaweed
- Seaweed farming has high costs of entry due to the licencing requirements, boats and infrastructure required.
- Norfolk Seaweed uses long-line offshore kelp farming techniques.
- Kelp is ready to harvest within 5 months of being set and some farms harvest twice a year.
- NS harvests in the spring, as it is best before the waters get warmer.
- The current farm is 25ha and NS has succesfully secured a license to expand to 50ha next year.
- They currently only have four people who manage the 25ha plot.
- They are exploring the use of remote sensing and monitoring tools to observe the offshore kelp farms.
- Kelp likes clear water with low wave action. Their farm is located nearby to a rock shelf that mitigates wave action.
- Hatching kelp is one of the major limitations as the only hatchery in the UK currently is in Scotland. This results in high transportation costs.
Environmental
- Kelp is claimed to sequester carbon. Allie Wharf explained that once harvested, any sequestered carbon is released back into the atmosphere. However, online reports state otherwise *To be further investigated in Term 3
- Combining kelp farms and oyster farms can establish integrated multi-trophic aquaculture systems that create more resilient crops and shellfish, and promotes habitat creation.
- Farming in a protective area is restrictive. They are trying to prove the ecological benefits of kelp to make expansion along the Norfolk coast more desirable by policymakers.
- Kelp farms can contribute to wave attenuation depending on their location. This can reduce coastal erosion.
Economic
- Seaweed farming is a costly endeavour. NS’s established oyster farm made seaweed farming more accessible since they already owned boats, had prior experience in aquaculture, and were familiar with the Norfolk coast farming sector.
- Selling raw kelp is not that profitable. It is critical to monetise every aspect of the algae.
- Kelp for use in fertilisers is the most popular application. Pharmaceutical industries have expressed interest in their product.
- Monetising the extracted or dried kelp is advantageous.
- No one has currently inquired about scaling kelp production for use in construction. However, Kathryn Larsen, seaweed architectural researcher, Netherlands has requested raw fibres for experimentation.
* We are also awaiting a fibre sample from Allie.
- Looking ahead, the farm wants to construct its own hatching and processing facility to monopolise all aspects of processing and distribution on site.
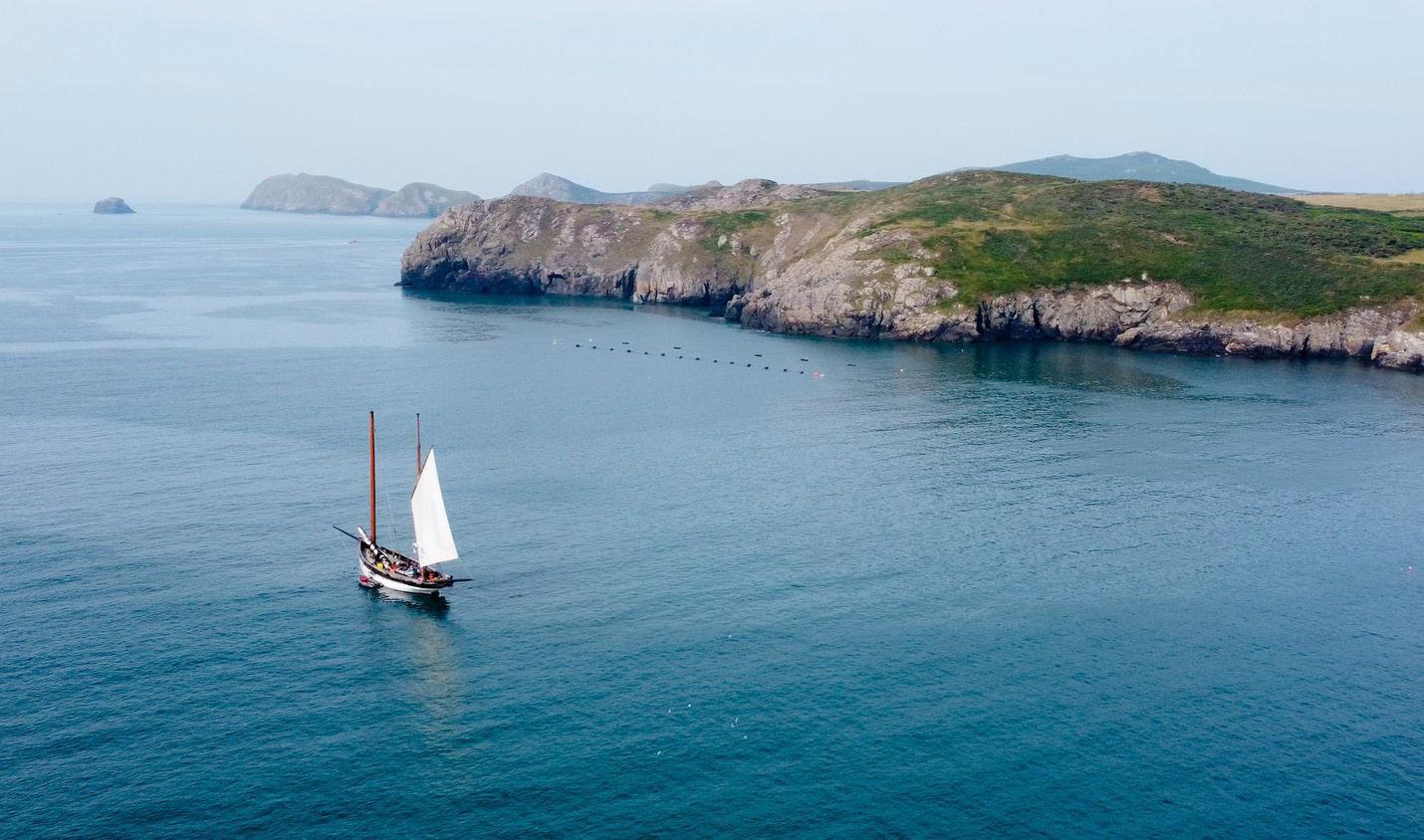
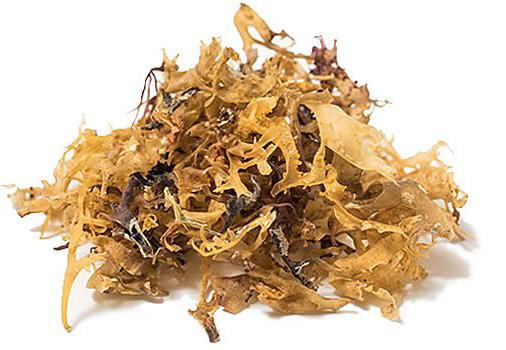
Seaweed for BGS
by Emily Bowerman
The proposed strategy for procuring materials for the BGS considers factors beyond its use in construction, such as how the materials are farmed, produced, and manufactured. Therefore, based on the geography of the East Midlands, there is an extensive coastline with an existing Seaweed farm just beyond a 100km radius from the BGS as noted (the potential of Seaweed is further explored at the East Midlands scale, forthcoming in this report). Moreover, desktop research of additional farms across the UK reveal a drive to expand the industry and accelerate innovation in farming, production and the breadth of products being manufactured from Seaweed.
Although Seaweed is a relatively novel practice in the UK, globally, it is a multi-billion-dollar industry, and some Asian countries produce tens of millions of tonnes a year. Seaweed farmers are seeing the potential of kelp not only for its commercial use, but also its contributions to ecosystem health.
As Seaweed farming begins to expand, opportunities exist to prescribe sustainable farming and ecological marine management agendas; mitigation measures need to be in place to reduce potential for disease ridden monocultures or acceleration of erosion in vulnerable coastlines due to over sedimentation.
Opportunities exist for seaweed to contribute immediately to more sustainable cereal crop production, of which straw is a by-product, as an alternative to synthetic fertilisers. Kelp is fast growing and requires no pesticides or water for production. Moreover, kelp fertiliser is bioavailable for plants, contains natural growth hormones like cytokinins, auxins, and gibberellins that help plants sustain harsh climatic conditions like drought, heat, or disease, and it doesn’t contain harsh chemicals that pollute waterways or ecosystems.6
Upon extracting nutrients from the Seaweed for use in fertiliser, the by-product could potentially be used for acoustic panels, exemplified by innovation companies such as BlueBlocks in the Netherlands. As Seaweed farming expands in the UK, opportunities exist to be at the forefront of circular supply chain thinking. 7
Overall, proposing Seaweed-based fertilisers as an immediate input to the straw supply chain could catalyse growth of the Seaweed industry. Scaling of a nascent coastal industry could create jobs in farming, research & innovation, machine-learning and more jobs that target younger demographics.
1
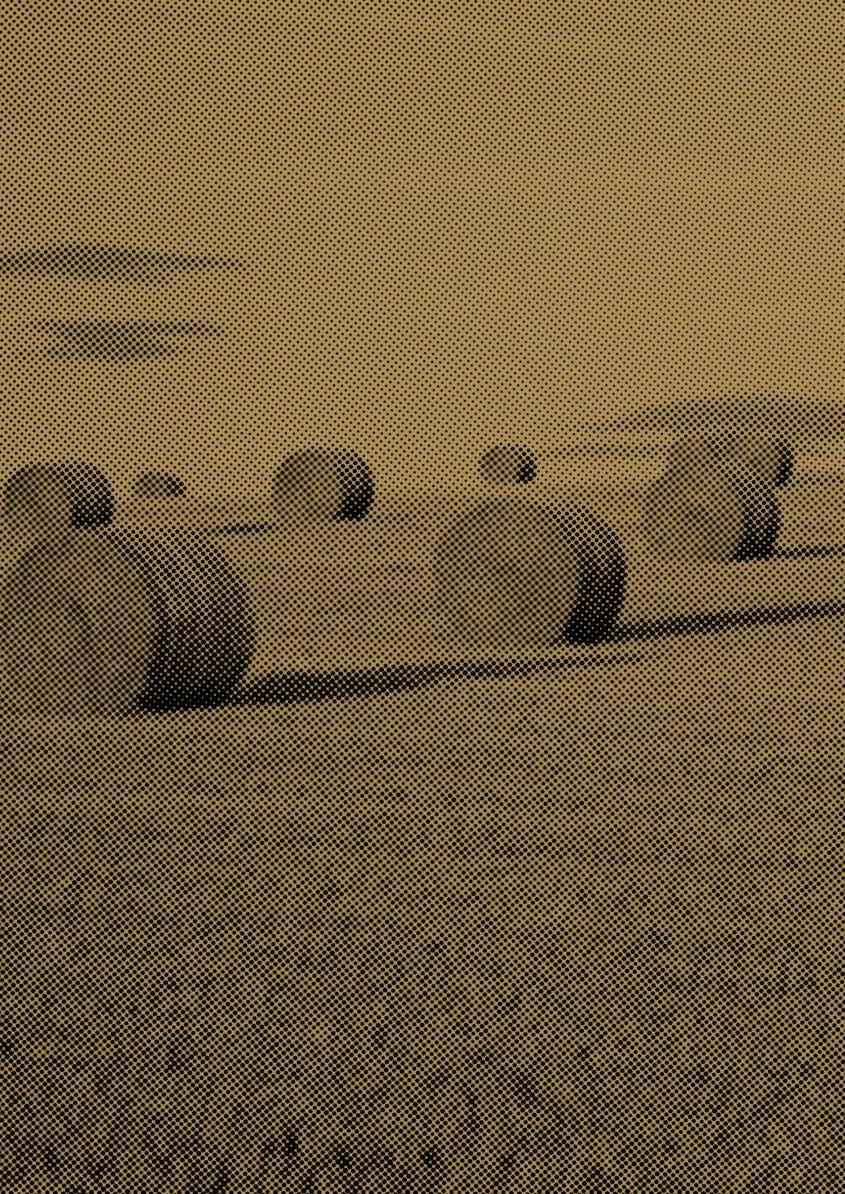
Biobased material matrix
8.
Straw is a promising biobased material for construction in the UK, given its abundant availability and favourable properties for sustainable building practices. With approximately a quarter of UK land already dedicated to cereal crops, from which straw is a natural byproduct,8 there is potential for meeting material demands. Moreover, straw exhibits excellent insulation properties,9 making it an attractive option for enhancing building energy efficiency and reducing carbon emissions. Its conversion into a building material is relatively straightforward, leading to lower carbon footprints and simplified construction processes.10
In our mapping efforts, we focused on identifying areas with significant and possible straw-producing croplands across the UK. Additionally, we mapped out key stakeholders involved in the straw supply chain, including members of the British Hay and Straw Merchants Association (BHSMA), who play an important role in straw procurement and distribution. We also identified European Straw Building Association (ESBA) certified straw bale producers. We found out there are only three producers in the whole of UK that adhere to ESBA’s quality standards.
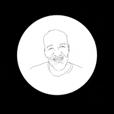


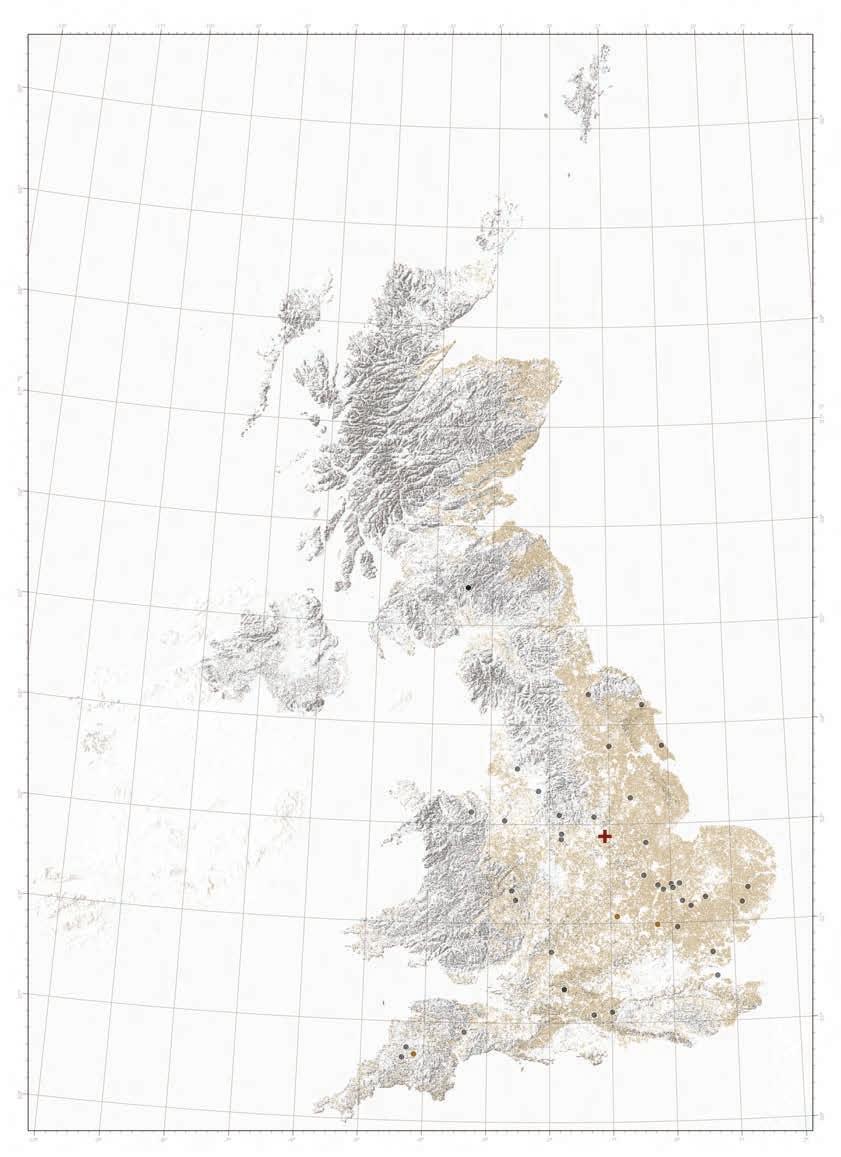
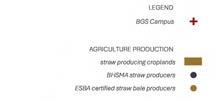
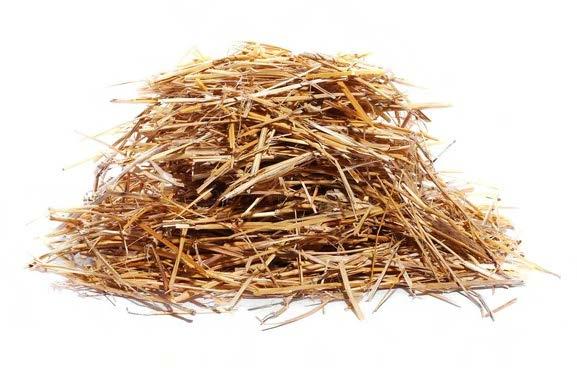
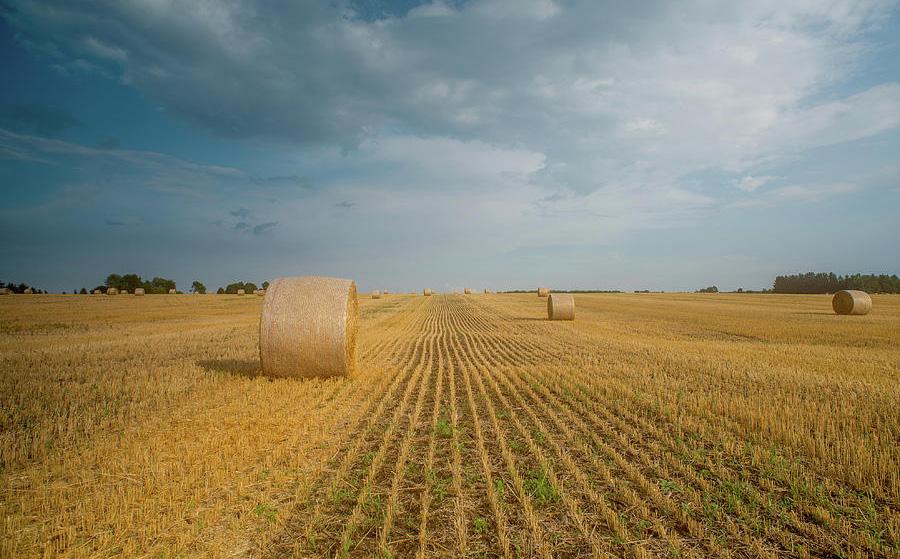
Straw
by Alejandra Iturrizaga Andrich

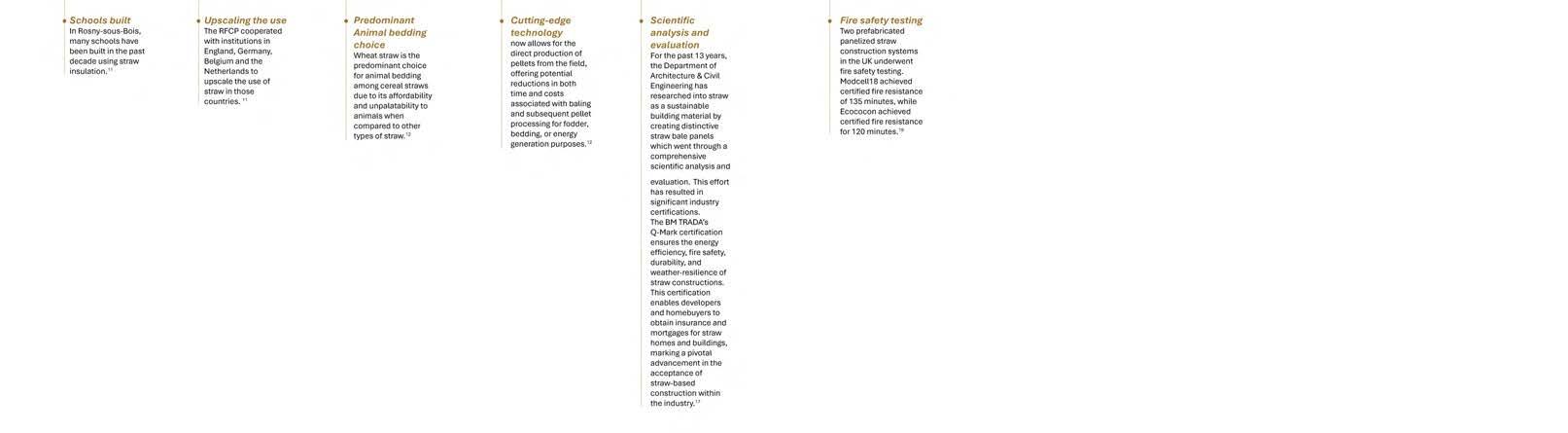

HE MP
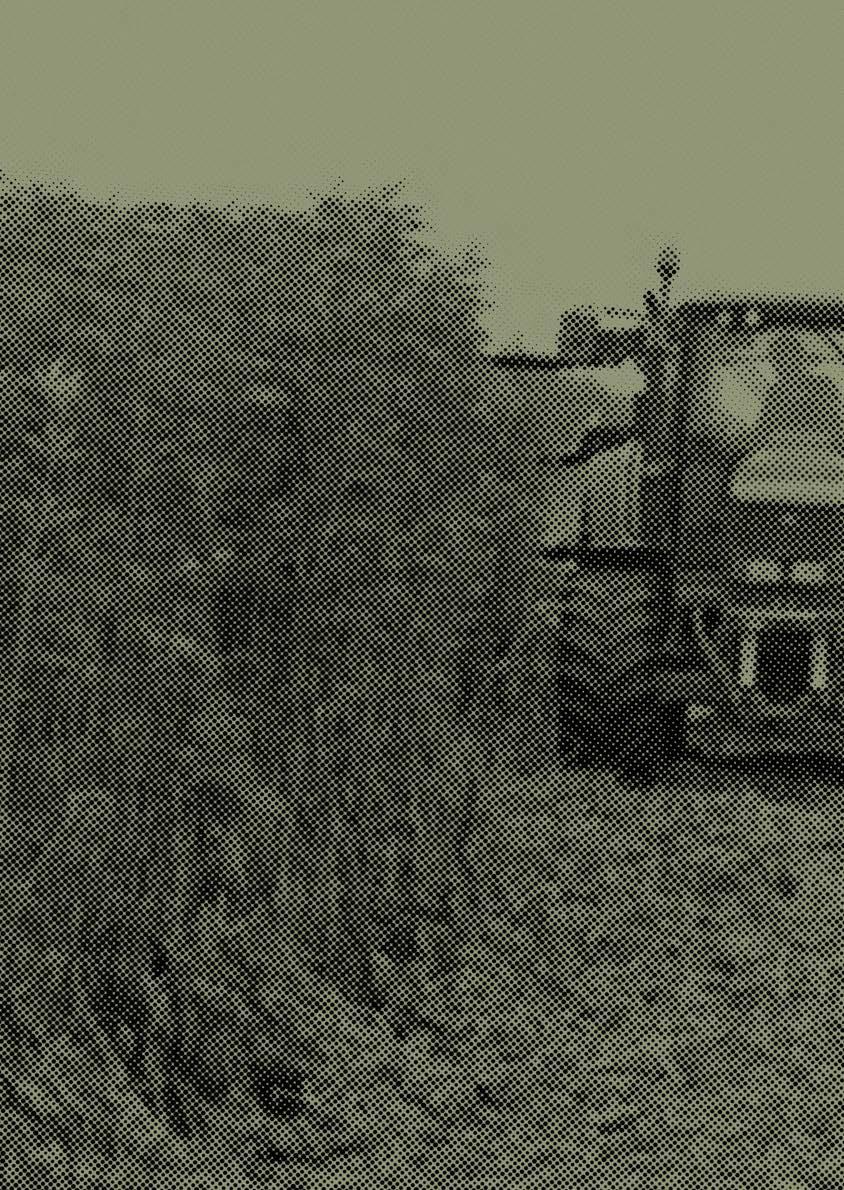
110 licences
issued for a validity period of three growing seasons
*Detailed information unavailable
Area under Hemp cultivation
UK: 810 ha
Europe: 33,000 ha
110 kg of C02
Construction with hemp and lime can lock up to 110 kg of CO2 per cubic meter of wall 1
31. “Hemp Lime Spray,” Hemp Lime Spray, accessed March 2024 https://hemplimespray.co.uk
32. “Hemp Lime Spray,” Hemp Lime Spray, accessed March 2024 https://hemplimespray.co.uk
33. “UK Hempcrete,” UK Hempcrete, accessed March 2024, htttps://ukhempcrete.com
34. “UK Hempcrete,” UK Hempcrete, accessed March 2024, htttps://ukhempcrete.com
35. “Hemcore Ltd,” Business Weekly, accessed March 2024, https://www.businessweekly.co.uk/ business-directory/hemcore-ltd
36. “Hemp Fuel,” WAMA Underwear, accessed March 2024, https://wamaunderwear.com/blogs/ news/hemp-fuel
37. “Where Is Hemp and Cannabis Grown?” Vitality CBD, accessed March 2024, https://vitalitycbd. co.uk/pages/where-is-hemp-and-cannabis-grown
Industry & Opportunities Hemp
by Alejandra Iturrizaga Andrich
Hemp is gaining popularity across many sectors in the UK, particularly in biobased industry as a concrete alternative. Hempcrete, is a mixture of hemp shiv and lime which serves as a high-performance, low-carbon building material.31 It has excellent insulating properties and breathability, hempcrete reduces the energy required to heat buildings while preventing issues such as dampness, condensation, and mold.32 Moreover, hemp has the capacity to absorb approximately 110 kg of CO2 per cubic meter of wall, contributing to carbon sequestration.33
The historical significance of hemp in the UK, dating back to Queen Elizabeth I’s decree mandating hemp cultivation for naval use, underscores its durability and versatility as a construction material.34 Modern innovations in hemp farming and processing have led to the revival of hemp-based industries, with companies like Hemcore Ltd pioneering hemp cultivation for various applications, including biobased construction materials.35 Hemp’s rapid growth cycle, low water requirement, and ability to prevent soil erosion further enhance its appeal as a sustainable agricultural crop.36 Additionally, hemp-based biofuels offer a renewable alternative to traditional fuels, requiring less water and pesticides for cultivation.37 As the hemp industry continues to expand and innovate, its role in biobased construction and sustainable agriculture grows.
In mapping the potential for hemp cultivation and its integration into biobased construction practices in the UK, several factors were considered. First, we identified areas of suitable soil composition for hemp cultivation, focusing on regions where cereals are predominantly grown. This approach leverages existing agricultural infrastructure and practices while diversifying crops to enhance soil health and resilience. Additionally, existing hemp farms were mapped to understand the current landscape of hemp cultivation in the UK. However, it is important to note that the information on hemp farms is not disclosed publicly, indicating a need for transparency and data sharing within the industry. Furthermore, we mapped hemp producers and hem-based pharmaceutical distributors to identify key stakeolders in the industry.
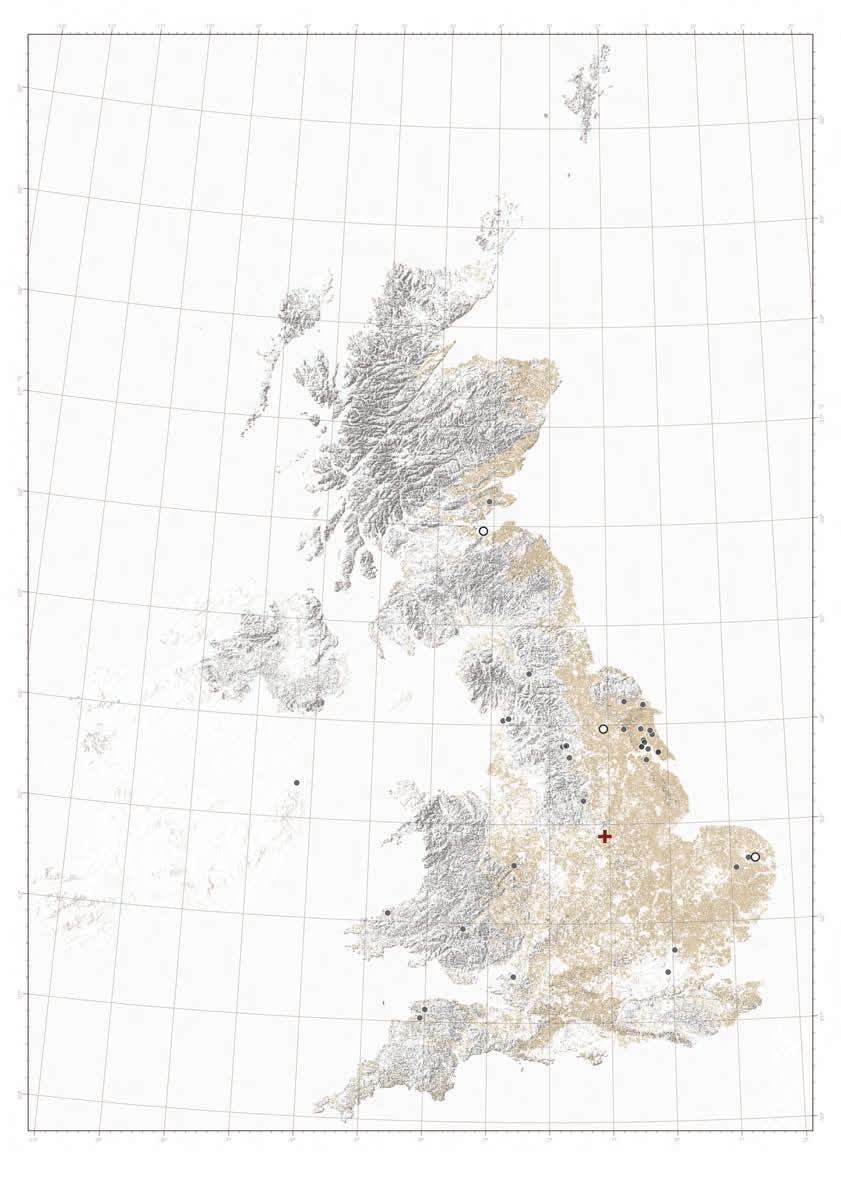
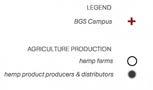
MYCE LIUM
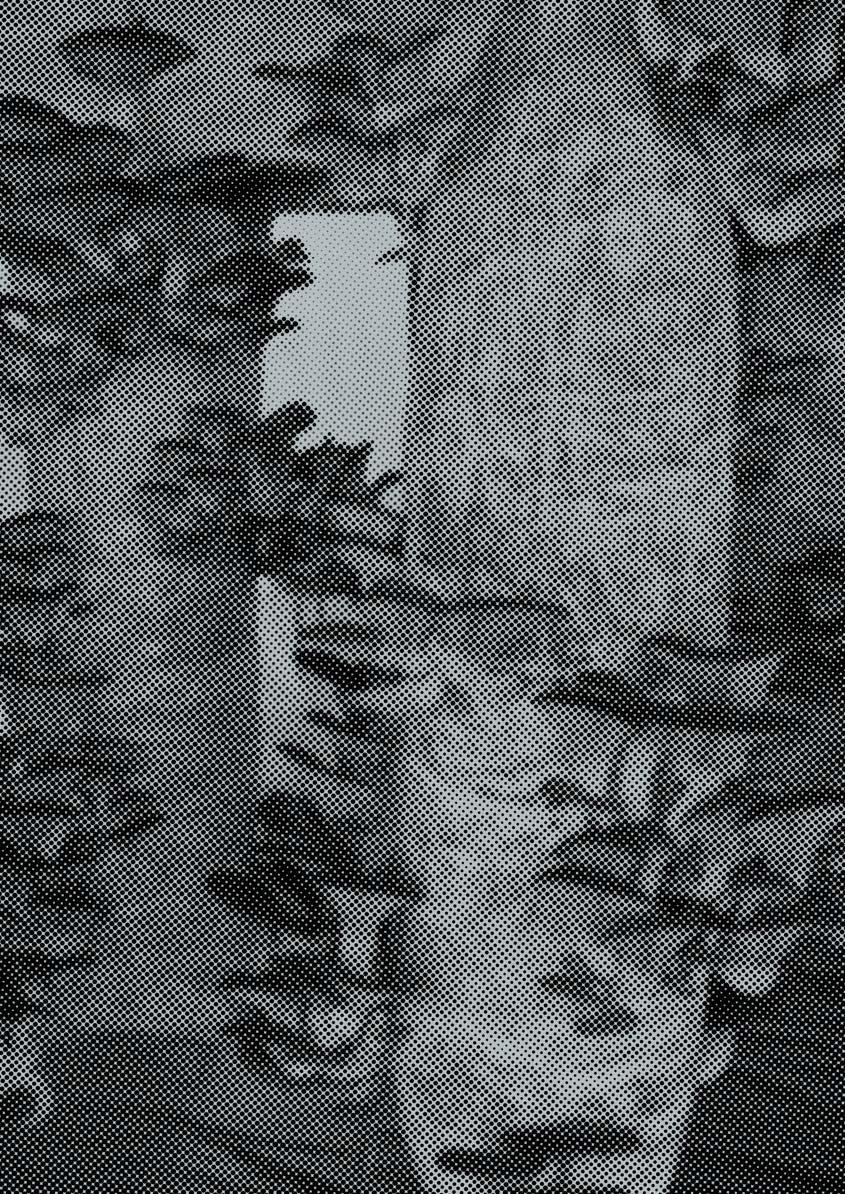
MYCE LIUM
38. Mykor Limited, “Mycelium Materials: Fantastic Fungal Innovations & Will Fungal Composites Take Over the World?” Mykor, accessed March 2024, https://www.mykor.co.uk/news/myceliummaterials-fantastic-fungal-innovations-will-fungal-composites-take-over-the-world
39. “Mycelium Insulation,” UK Green Building Council, accessed March 2024, https://ukgbc.org/ resources/mycelium-insulation/
40. Mykor Limited, “New UKRI Funding Aims to Enhance UK Sustainable Biomanufacturing,” Mykor, accessed March 2024, https://www.mykor.co.uk/news/new-ukri-funding-aims-to-enhance-uksustainable-biomanufacturing.
41. Mykor Limited, “Mykor Secures £825k Pre-Seed Funding Round” Mykor, accessed March 2024, https://www.mykor.co.uk/news/dj5kgcutix4gq9oi14v0xhj1h15151.
Industry & Opportunities
by Alejandra Iturrizaga Andrich
Mycelium is lightweight and versatile nature make it an attractive option for building material as insulation, providing both acoustic and thermal performance while offering superior fire resistance and moisture regulation properties.38 Mycelium-based products require minimal water and energy compared to conventional alternatives,39 sometimes combining it with paper waste and repurposing it. Additionally, mycelium composites have impressive durability and resistance to pests and mould, ensuring longevity and structural integrity in building applications. The rapid growth of mycelium presents opportunities for efficient production and scalability, potentially revolutionising the construction industry. High-profile projects such as the HY-Fi Installation in New York and the Mycelium Hayes Pavilion at the Glastonbury Festival demonstrate the architectural possibilities and aesthetic appeal of myceliumbased structures. Mycelium biobased material research has been funded up to date 40 by Innovate UK and the EU41 which proves interest in this material and acceleration in certification processes.
Mycelium is versatile fungi that can grow in relatively small spaces, therefore, we mapped urban areas as potential grounds for mycelium production. This focus on urban environments aligns with the trend towards sustainable, high-density living and offers opportunities for repurposing under-utilised spaces for mycelium cultivation. Furthermore, the proximity to paper factories was mapped, recognising the symbiotic relationship between mycelium producers and paper waste as a key ingredient in mycelium insulation production. By situating mycelium production facilities near paper factories reduces transportation distances and associated carbon emissions. Additionally, existing mushroom farms and dedicated mycelium producers were mapped to identify regions with established infrastructure and expertise in fungal cultivation. However, we understand that there might be labs and other places which could be cultivating mycelium for which we were not able to find information. By analysing these spatial dynamics, we aim to support the growth of the mycelium industry.
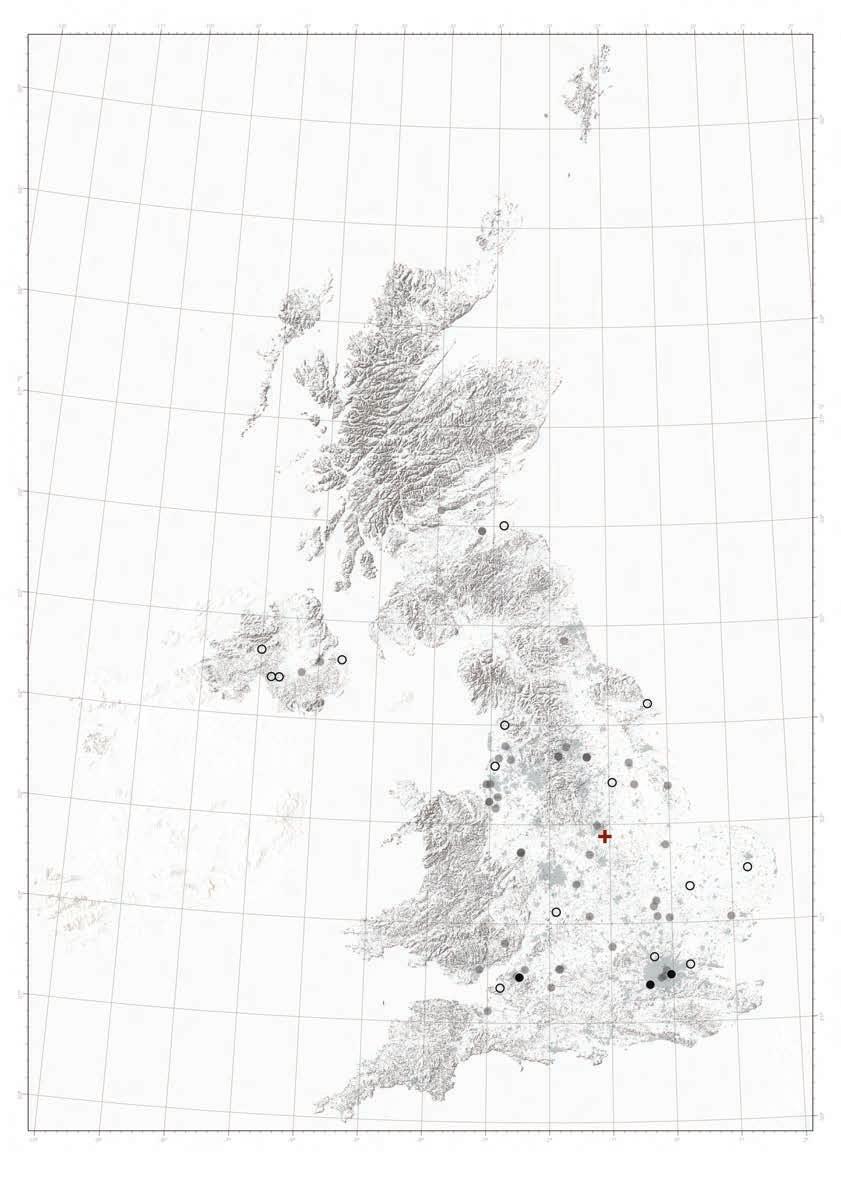
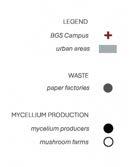
Mycelium
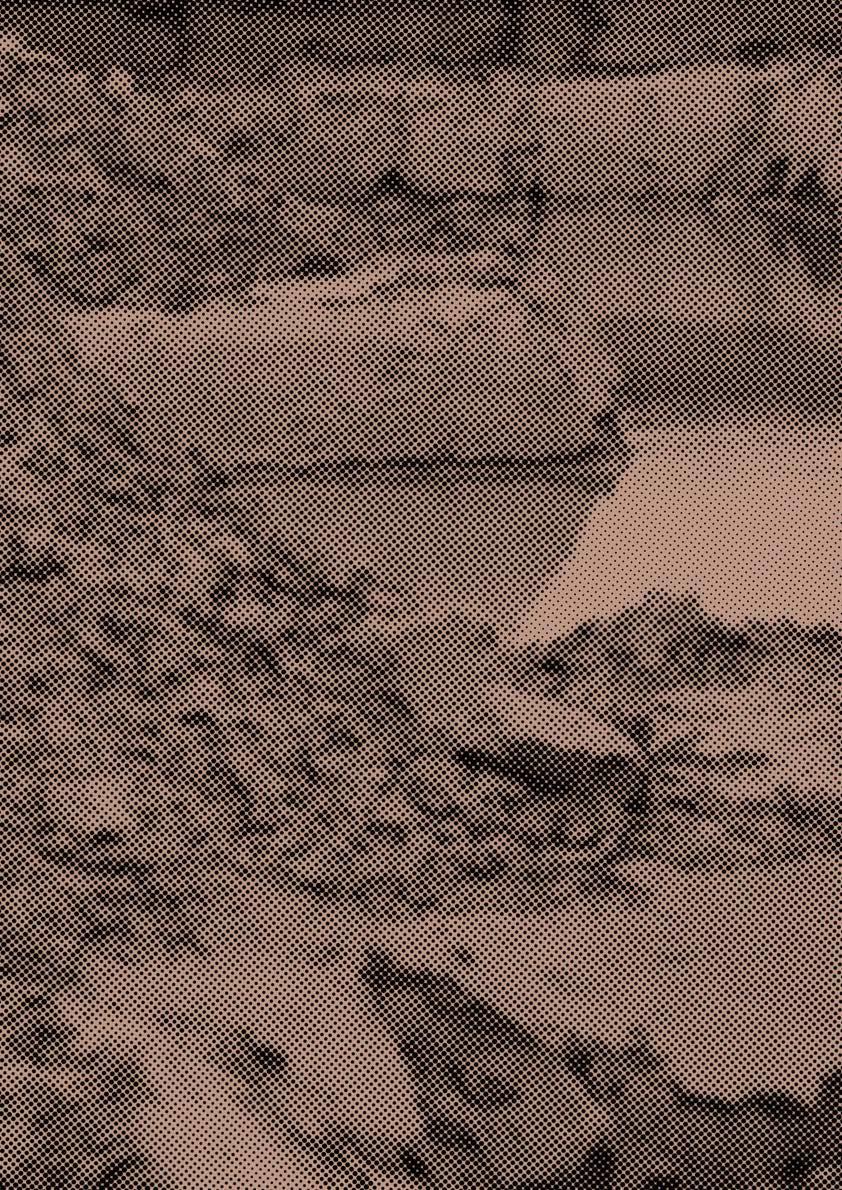
SEA WEED
4 million tonnes
extracted clay and shale
42. “Brick Clay Mineral Planning Factsheet” BGS, 2022. accessed April 17, 2024, https://nora.nerc. ac.uk/id/eprint/532490/1/Brick%20Clay%20Mineral%20Planning%20Factsheet.pdf.
43. Wienerberger. “What Are the Benefits of Building with Clay?” Accessed March, 2024. https:// www.wienerberger.co.uk/tips-and-advice/sustainable-building/what-are-the-benefits-of-buildingwith-clay.html#clay-building-a-better-quality-of-life
44. Build Naturally “Clay.” Accessed March, 2024. https://buildnaturally.com/Clay.html
Industry & Opportunities
by Priyanka Awatramani
Brick clay comprises clay—a topsoil, shale and mudstone.42 It holds significant importance in various industries, including construction. Its limited availability makes it a valuable resource, particularly for its regenerative properties and ecological benefits. Clay deposits are alive with microorganisms and ecosystems, contributing to soil health and biodiversity. In our exploration of the UK’s clay industry, we mapped the spatial distribution of clay reserves across the country along with extraction sites and brick manufacturing facilities. These are mainly concentrated around the Midlands and southern England. In 2021, approximately 4 million tonnes of clay and shale were extracted, highlighting its indispensability as a construction material.44
Clay finds versatile applications in construction, serving as the foundation for bricks, tiles, and raw materials for cement and plasters. Despite the challenges posed by its limited availability, the use of clay in construction offers numerous advantages. Its structural integrity and insulating properties make it invaluable, ensuring that buildings remain thermally comfortable by absorbing and storing heat, then gradually releasing it as temperatures fluctuate.43 Moreover, clay’s innate ability to regulate humidity further enhances indoor air quality, fostering healthier living environments conducive to human well-being.45
While most conventional bricks are fired, rendering them non-biodegradable, more ecological alternatives like adobe bricks and compressed earth blocks need to be prioritised. These biobased building alternatives align with growing environmental consciousness, as viable options for reducing the ecological footprint of construction activities and building resilience.
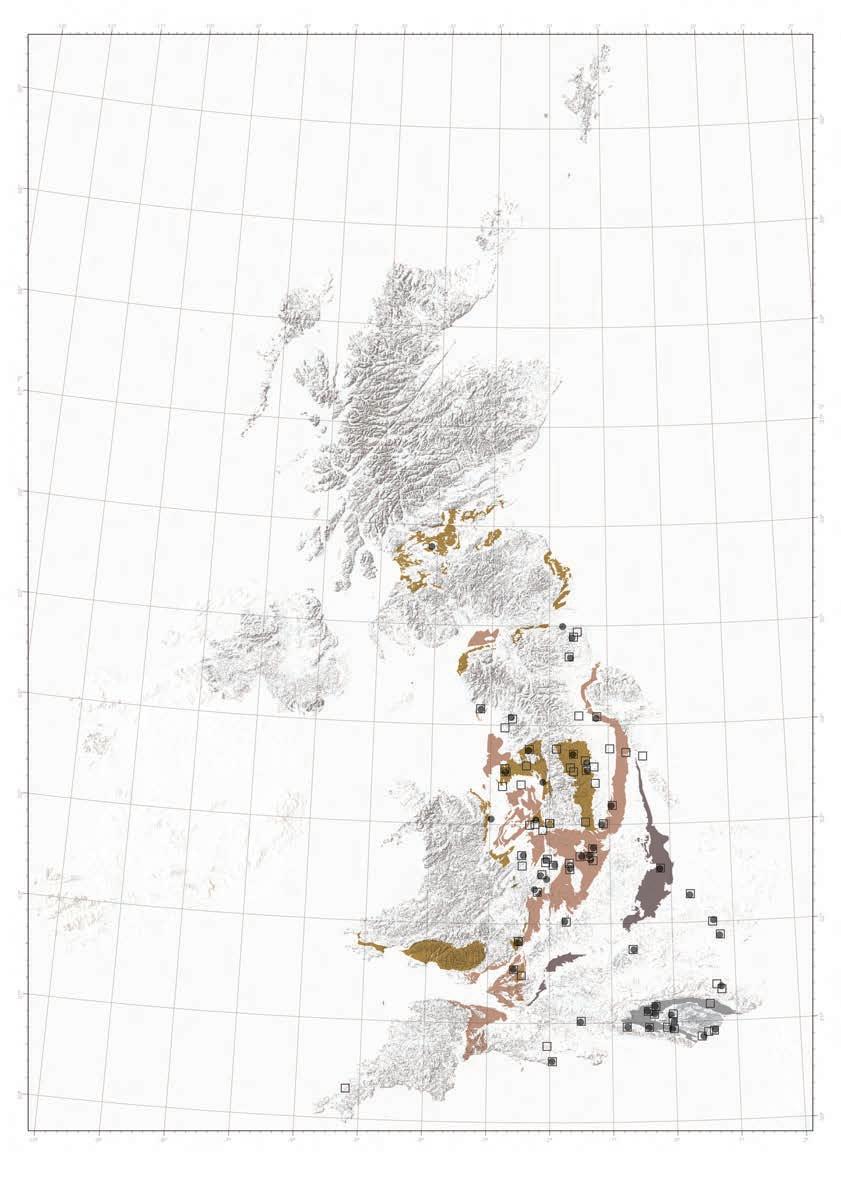
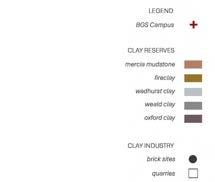
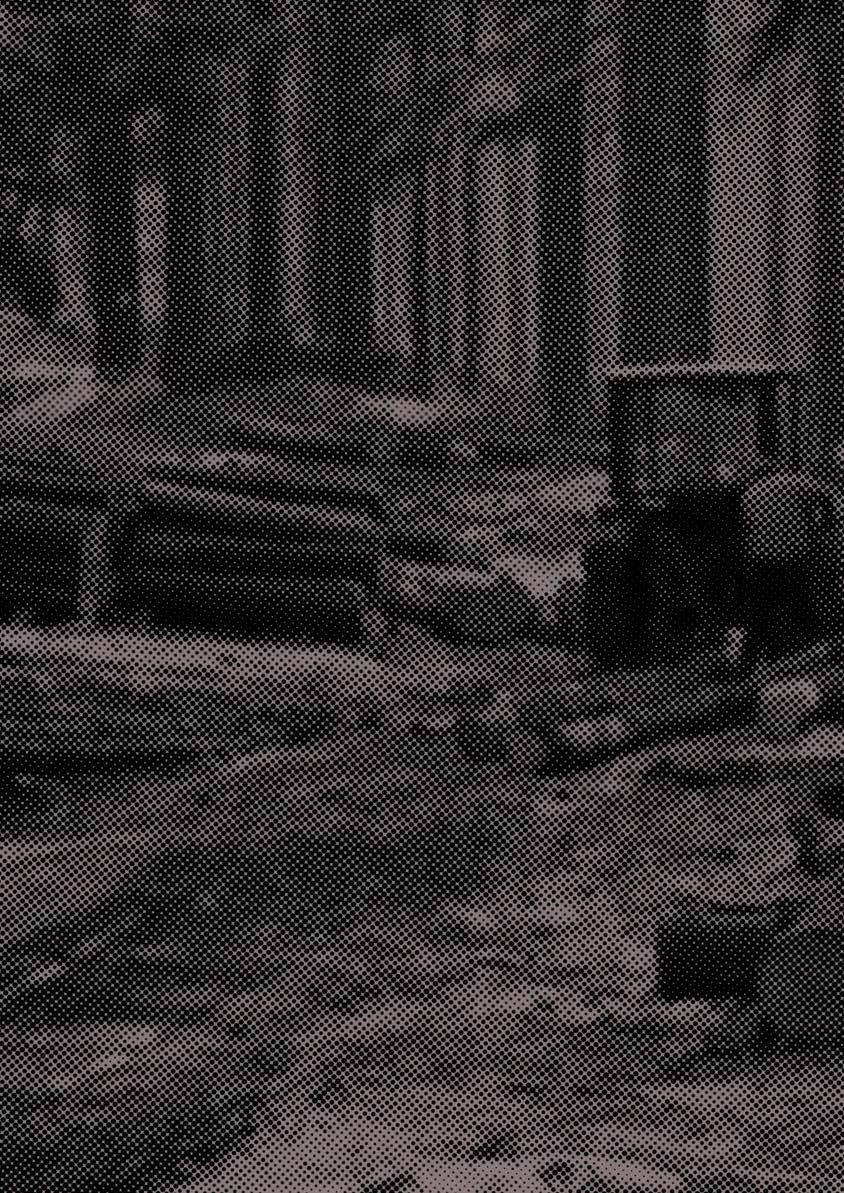
2.5% Increase
in Softwood availability in the UK 2046
12.3% Increase
in Hardwood availability in the UK 2046
45. “Forestry Statistics 2021 Chapter 2: UK-Grown Timber,” Forest Research, 2021. Accessed April 17, 2024, https://cdn.forestresearch.gov.uk/2022/02/ch2_timber_fs2021_mlulwth-1.pdf
46. Exova Trada, “Specifying British-Grown Timbers,” 2017.
47. “Timber in Construction Roadmap,” GOV.UK, accessed April 17, 2024, https://www.gov.uk/ government/publications/timber-in-construction-roadmap/timber-in-construction-roadmap
Industry & Opportunities
Timber by Priyanka Awatramani
The timber industry plays a significant role in the UK’s environmental strategy. According to estimates from the Forestry Commission, approximately 10.9 million tonnes of roundwood was harvested from the UK forests in 2020, with softwood accounting for the majority (92%) of these removals.45 Softwood, known for its faster growth rates in the UK, comprises the bulk of timber harvested, with hardwood making up the remaining portion. However, the reliance on softwood presents challenges, as UK-grown softwood is typically graded as C16, necessitating the imports of higher-grade timber from Europe.46
While mapping the timber industries in the UK, it was difficult to obtain precise data on active woodlands in the UK complicating efforts to assess the industry’s spatial distribution. However, spatial datasets indicating possible harvested areas, such as coppice or ground preparation sites were mapped, suggested high concentrations in regions like Scotland and Wales. Additionally, mapping privately and publicly managed woodlands and timber processing facilities provided further insights into the industry’s infrastructure and distribution across the country. It is important to note that the data for Northern Ireland is lacking hence the insights might be more applicable for Britain scale.
Despite these challenges, the UK’s abundant timber resources offer opportunities for utilising timber as a biobased material. Biobased materials such as hempcrete blocks or mycelium blocks, which require supports at frequent intervals, can complement traditional timber usage. Leveraging timber as a biobased material therefore has the potential to align with the nation’s net-zero strategy, offering environmentally sustainable alternatives to conventional construction materials. Furthermore, as the UK aims to increase tree canopy and woodland cover to 16.5% by 2050, opportunities arise for expanding the timber industry sustainably.47
Sustainable forestry practices, including selective logging and reforestation, are pivotal in enhancing woodlands’ capacity as carbon sinks, aiding in greenhouse gas mitigation and climate change adaptation. The timber industry is crucial in supporting broader environmental goals by promoting biodiversity, mitigating climate change impacts, and safeguarding ecosystem health.
In summary, the timber industry in the UK occupies a crucial role in balancing economic prosperity with environmental stewardship. Through sustainable management practices and innovative approaches to material utilisation, the industry can contribute significantly towards building ecological and economical resilience.
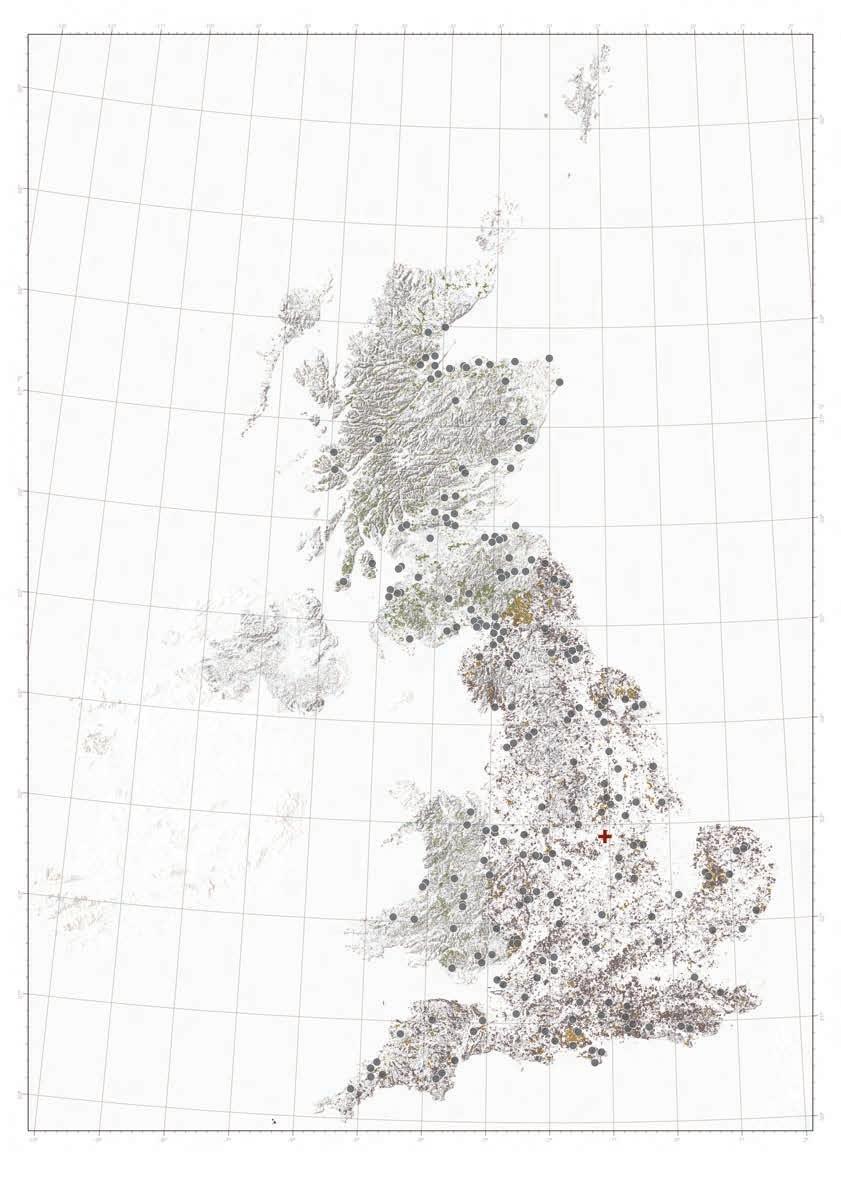
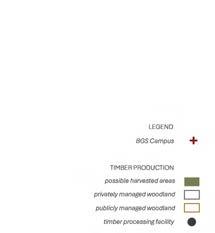
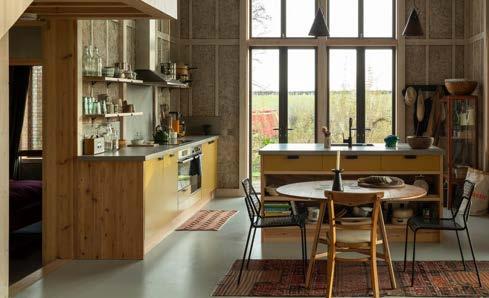
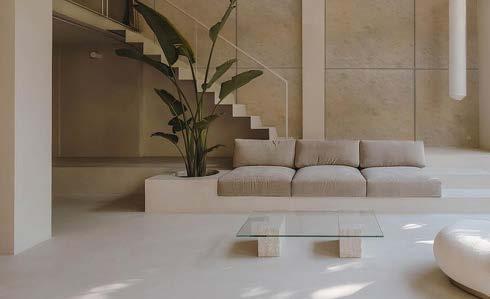
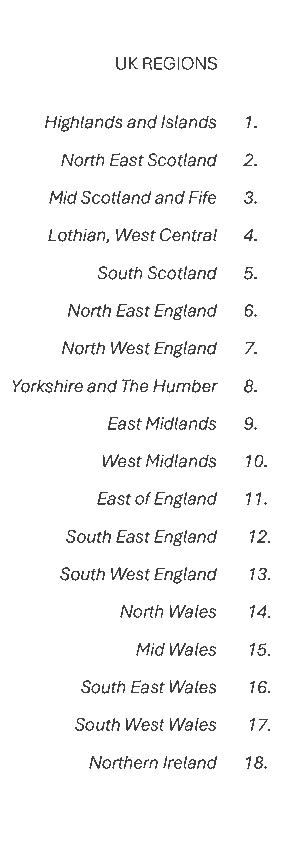
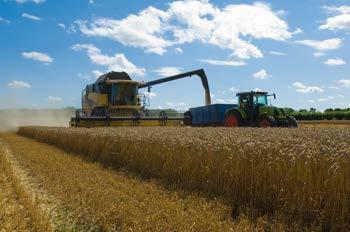
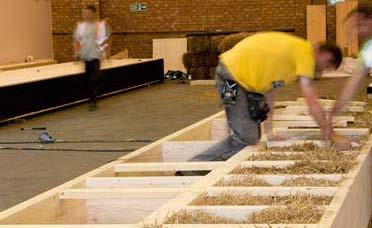
Biobased material procurement for UKRI institutions
Awatramani
To bring awareness to the UKRI and inform decision-making, a summary for each region’s biobased potential was prepared. Since Cultivating Commons recommends a regional procurement of materials, each biobased material is ranked based on their availability in each region and the opportunities they present, for retrofitting projects in the UK.
Combined with the viability study for biobased raw materials and building materials, this map acts as a tool for 80 UKRI institutions, enabling them to identify suitable materials for retrofitting projects. However, these rankings are not deterministic; they have the potential to evolve based on various factors such as strategic investments, research advancements, and long-term development plans.
For instance, a region may currently rank high in availability of certain biobased materials, but with targeted investments and research efforts, another region might surpass it in potential. While regions may compete for investments and opportunities, collaboration and knowledge sharing among UKRI institutions can foster innovation and maximise the collective potential of the biobased industries nationwide.
In
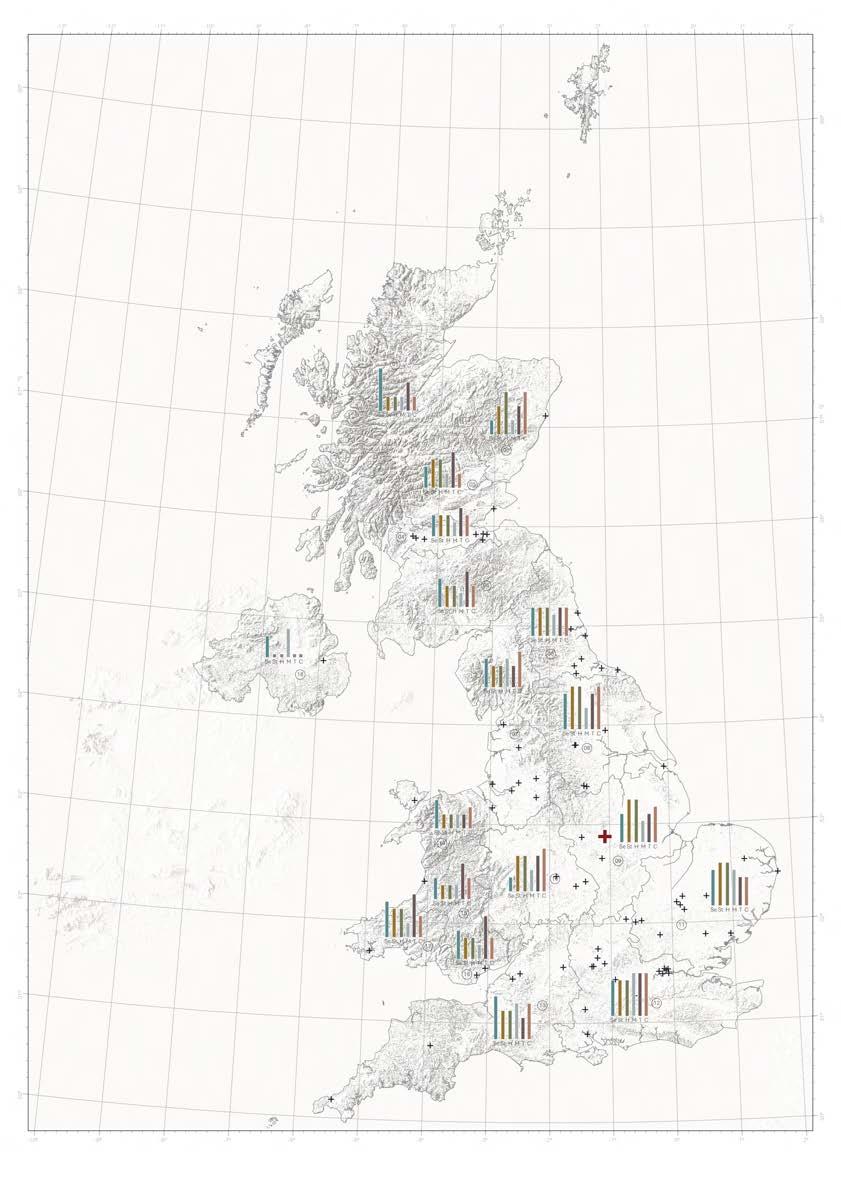
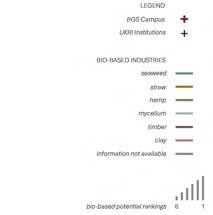
TRANSITION TO BIOBASED INDUSTRY
Transition from extractive industries to biobased industries; monoculture farming to regenerative farming; through a cradle to cradle approach
Just Transition
Transitioning from extractive and carbon intensive industry toward a biobased economy
by Priyanka Awatramani
The 2015 Paris Agreement on climate change incorporated the concept of a ‘just transition’ for workers and communities, which was later signed by more than 50 countries in the COP24 climate conference in 2018, including the UK. This declaration recognised the need for equitable measures as the global economy adapts to the challenges of climate change.1
In the construction sector, a just transition from extractive materials to biobased materials in the UK needs a holistic approach towards environmental, social, and economic aspects of the sector. Transitioning industries away from environmentally harmful practices towards more sustainable alternatives requires deliberate and equitable strategies to mitigate potential negative impacts on workers, communities, and stakeholders. This aligns with the principles of just transition in following ways.
Environmental impact
Shifting from extractive materials, which often involve destructive mining practices and contribute to carbon emissions, to biobased materials represents a significant step towards reducing the environmental impact of the construction sector. Biobased materials offer lower carbon emissions, reduced waste generation, and improved resource efficiency.
Social Justice
Encouraging new opportunities for workers in the construction industry, particularly those affected by transitions away from conventional practices. By providing support for reskilling, upskilling, and job transition programs, as well as prioritising investment in areas with higher concentrations of unskilled workers, the transition can create new opportunities and ensure inclusivity for all stakeholders.
Economic Viability
Investing in research and development for innovation can stimulate economic growth, particularly in poor areas. By targeting investment in regions with potential for biobased materials and aligning it with workforce development initiatives, the transition can foster long-term economic viability and prosperity.
Policy Support
National, regional, and local governments can implement supportive policies and incentives to encourage the adoption of biobased materials, such as tax incentives, grants, and subsidies, and promote adoption of transition toolkits for ensuring transparency and accountability. The transition from extractive materials to biobased materials is crucial for the UK on multiple fronts. Environmentally, it is a significant step towards reducing carbon emissions, minimising waste generation, and preserving natural resources, aligning with global sustainability targets. Economically, it presents opportunities for innovation, job creation, and fostering longterm economic growth and competitiveness. Socially, it promotes equity by providing new opportunities for workers affected by the shift away from extractive practices. A just transition to biobased materials is critical in enhancing global leadership in sustainable practices.
Transition Opportunities for the UK
From Extractive to Biobased Industry
by Priyanka Awatramani
Identifying areas for investor action is a crucial step in facilitating the transition from extractive materials to biobased materials in the UK. We targeted regions with significant potential for biobased materials and aligning investment efforts with the needs of local communities and workers.
Mapping Extractive Material Sites (A & B)
Utilising geological surveys and databases, we identified sites across the UK where extractive materials like stone and sand are mined. These sites may include quarries, gravel pits, and sandbanks, which are sources of raw materials for construction and manufacturing industries. In addition to this, we also identified the industries involved in these processing of materials, such as steel and cement production. These industries are major consumers of extractive materials and play a significant role in driving demand for these resources. Material extraction has severe environmental impacts associated, such as habitat destruction, land degradation, and water pollution and by identifying areas where extractive activities are particularly intensive or where environmental vulnerabilities are high, such as the 10km zones around these sites. These areas can be prioritised for investment in transitioning these areas towards biobased materials to mitigate negative environmental impacts and promote ecosystem restoration.
Overlapping Biobased Potentials (B & C)
Identifying overlapping biobased potentials involves mapping regions in the UK where biobased material production intersects with high environmental vulnerability zones. By considering factors such as crop production, active woodlands, and aquaculture industries, these maps pinpoint areas where the transition to biobased materials is both feasible and promising. This strategic approach ensures that investments in biobased material production contribute to ecological viability while fosterin economic development.
Prioritising Areas of Socio-Economic Vulnerability (D) Within these identified zones, prioritising investment in areas with a higher concentration of unskilled workers can maximise the socio-economic benefits of the transition. We identified regions where communities reliant on extractive industries may be facing job displacement or economic challenges, such that investors can help facilitate a smoother transition by creating new employment opportunities in the biobased sector. This targeted approach ensures that the transition is not only environmentally sustainable but also socially equitable.
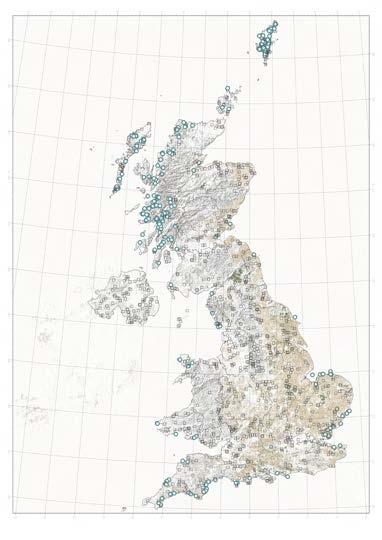
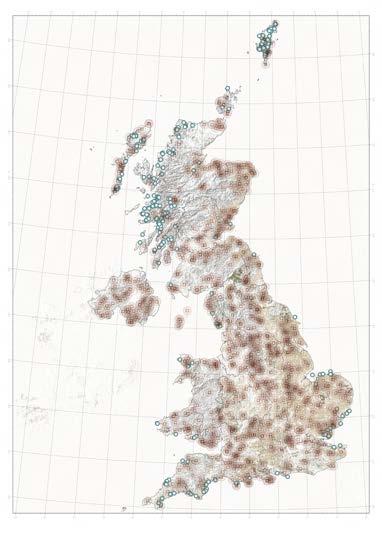
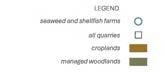
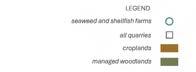
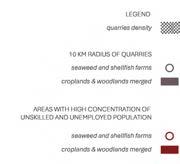
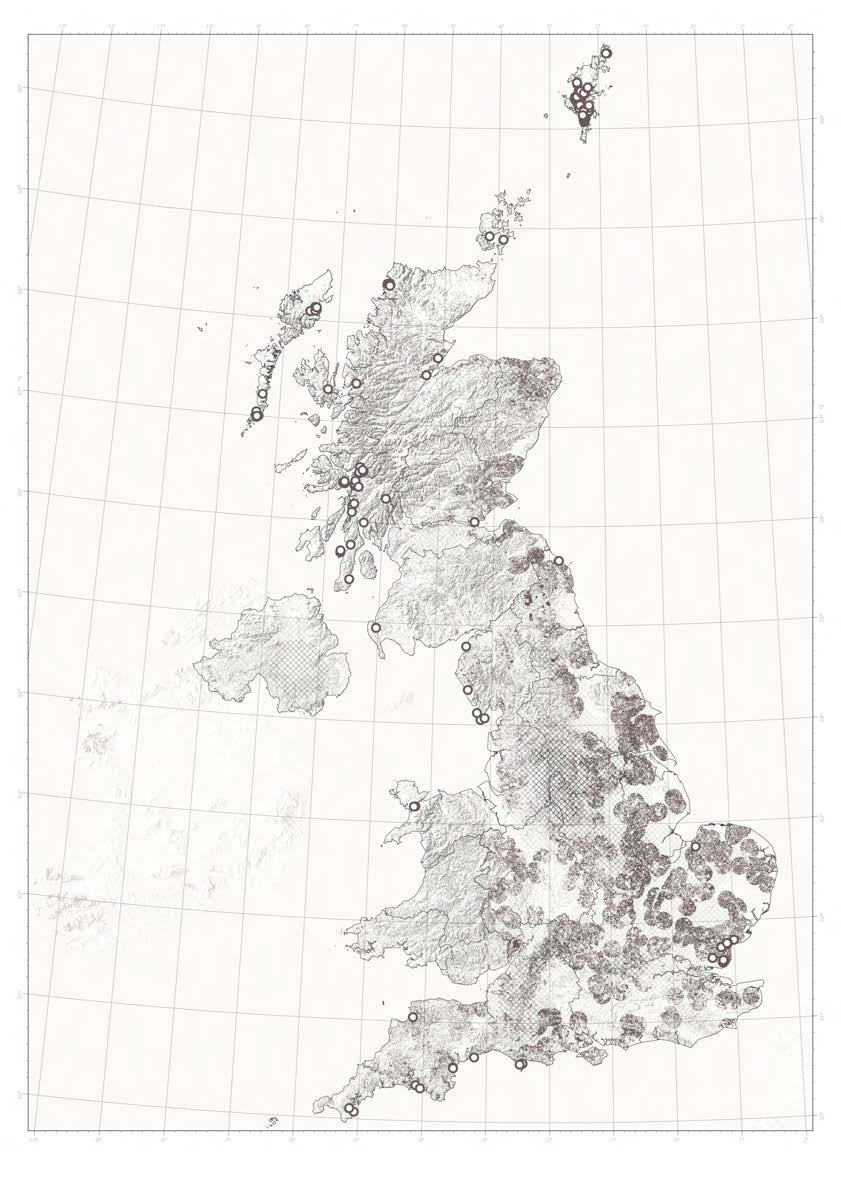
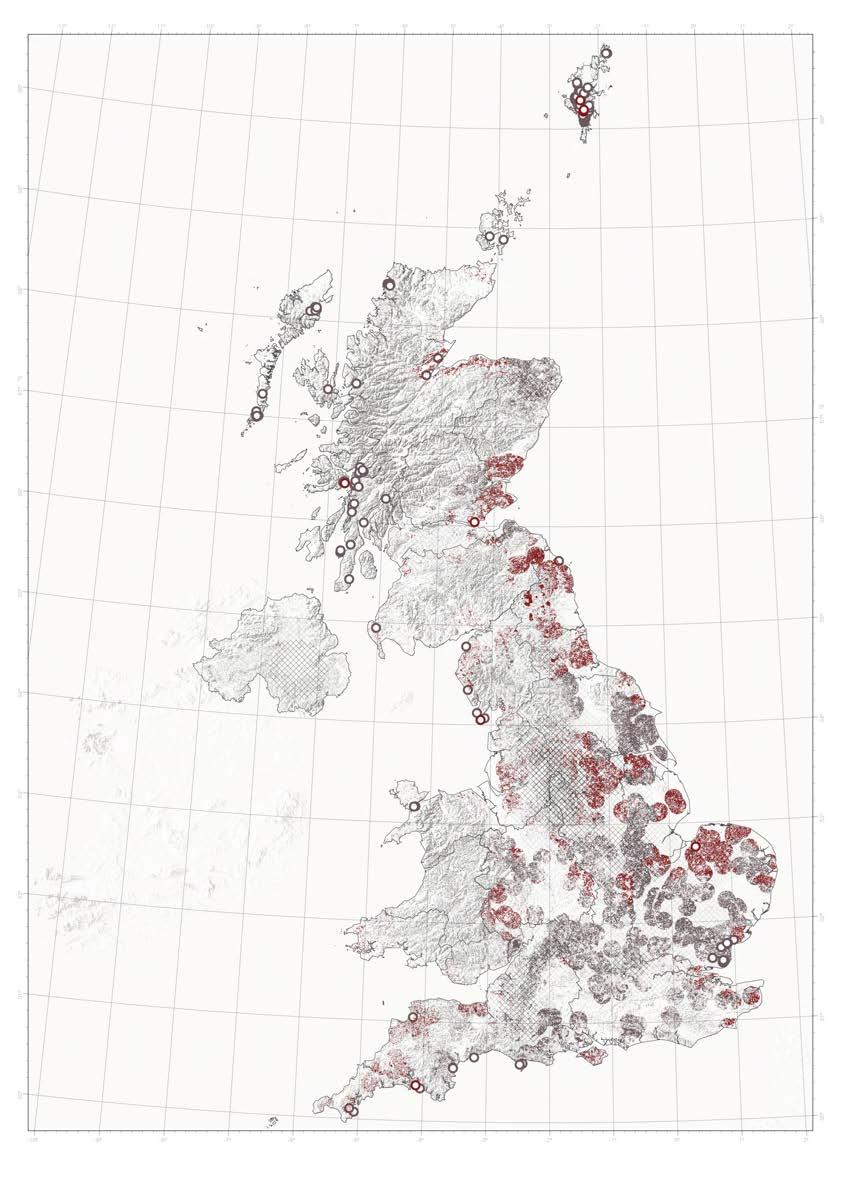
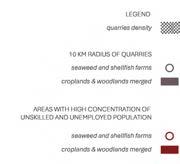
BGS RETROFIT
assessing retrofitting needs based on interviews and site observations
Need for Retrofitting BGS
Exploring opportunities to imrpove building insulation, interior working conditions and improve acoustics.
by Alejandra Iturrizaga Andrich
We had the opportunity to visit the British Geological Survey (BGS) campus located in Keyworth, Nottingham, UK in March 2024. The visit provided us with valuable insights into the existing infrastructure and highlighted areas for potential improvement.
One notable aspect of the campus is the predominance of older buildings, with only two structures being recently constructed. This mix of old and new presents challenges and opportunities for retrofitting efforts. During our exploration, it became evident that the older buildings, while having historical charm, require significant upgrades to meet contemporary standards of energy efficiency and functionality.
While materials like brick and concrete are durable, it was evident that the buildings lacked adequate insulation, as evidenced by the thin windows and the necessity to use curtains to minimise heat loss. This inefficiency in thermal performance points to the need for retrofitting measures to enhance energy efficiency and occupant comfort.
The campus boasts various green areas interspersed between buildings, offering a refreshing outdoor environment. One feature is the Geological walk, showcasing three billion years of Earth’s history along its path, providing both educational and recreational value to the campus community.
During our exploration of the campus facilities, we encountered small, closed cubicles that were implemented during the COVID-19 pandemic for e-meetings. However, it was apparent that these spaces were not adequately ventilated, resulting in uncomfortably warm conditions. Given the shift towards remote collaboration, there is a clear need for designated, properly ventilated spaces to accommodate virtual meetings effectively.
Additionally, we were made aware of the requirements of additional storage space in the core storesto accommodate the growing volume of samples received on campus. Addressing this requirement is crucial to maintaining the integrity and accessibility of the BGS’s extensive sample collection.
The laboratories, which were the focus of our analysis for retrofitting, presented specific challenges. While these spaces serve as critical hubs for scientific research, their internal arrangement was often not optimal, reflecting their adaptation from older buildings. This configuration poses constraints on usability and efficiency.
Two primary concerns emerged during our assessment of the laboratories: acoustics and thermal conditions. The presence of loud noises in some labs significantly detracted from the work environment, while inadequate insulation and outdated windows compromised thermal comfort and energy performance. Addressing these issues emerged as the top priorities for retrofitting, as they directly impact occupant productivity and well-being.
In conclusion, our assessment demonstrates the pressing need for comprehensive retrofitting measures to enhance the functionality and sustainability of the BGS laboratories. We identified two potential paths: retrofitting the existing lab buildings to improve environmental quality and reduce their ecological footprint or repurposing these structures as offices and constructing a new, biobased material building specifically designed to meet the unique requirements of modern scientific research. By embracing these initiatives, the BGS can ensure its facilities remain at the forefront of geological research while promoting environmental stewardship and occupant satisfaction.

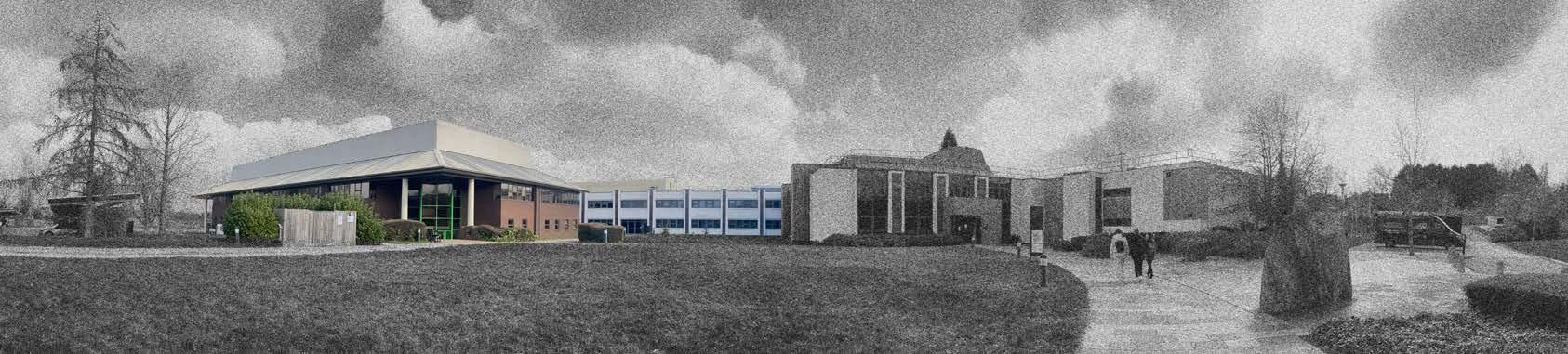
Andrew Barkwith Director of Operations at BGS
BGS Laboratory
What BGS staff say about the laboratories
By Emily Bowerman
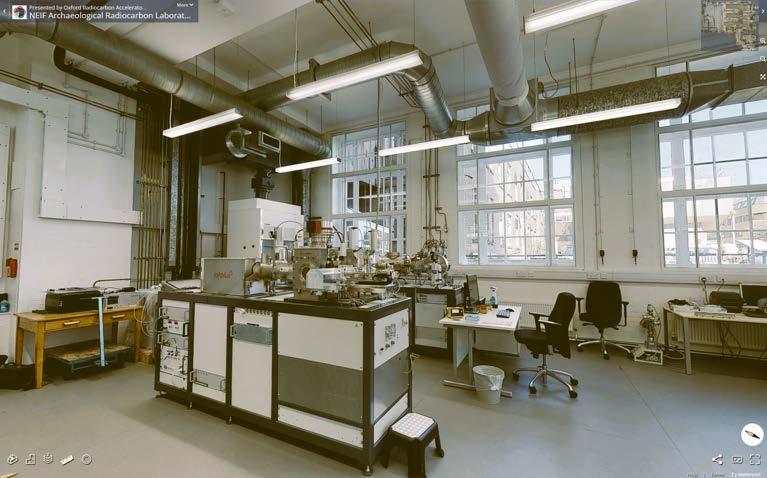
Noise from large machinery and ‘chillers’ causes an unpleasant working environment. *Acoustics are a primary issue.
Some tests and machinery require staff to work directly in the labs for polonged periods to time to run tests and studies. Appropriate desks and workspaces are required.
Appropriate solar control is required to better regulate interior temperatures.
BGS management recognise the interconnection between employee well-being and design of physical space. They want to ensure the building architecture and spaces within the BGS promote employee well-being and research excellence.
Interior lab layouts should encourage collaboration and comraderie. The BGS labs are currently spread apart, making it difficult for teams to meet, collaborate and share knowledge.
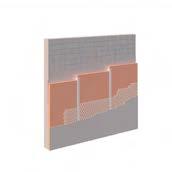
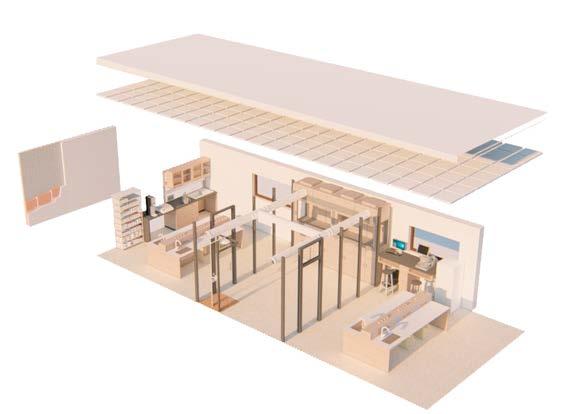
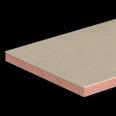
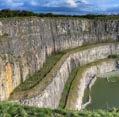
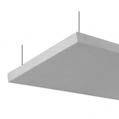
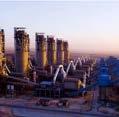
Inadequate ventilation and noisy air circulation vents make it difficult for employees to concentrate and work without noise cancelling headsets. *Acoustics are one of the major considerations for employee comfort.
Labs are dated, isolated, and do not reflect the innovative research being undertaken by the BGS.
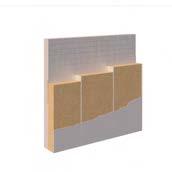
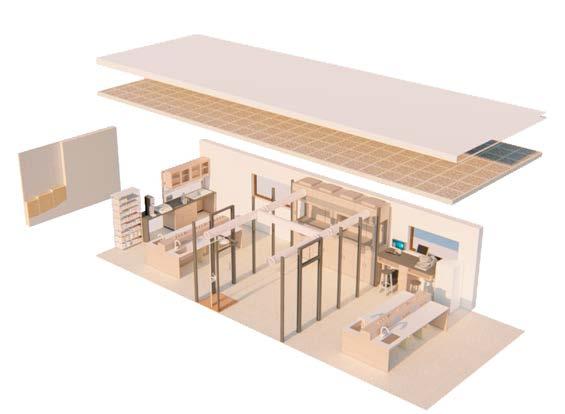
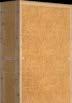
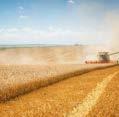
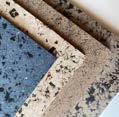
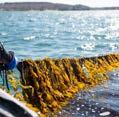
V BGS PROCUREMENT OF BIOBASED
MATERIALS
proposal for regional procurement of biobased building materials building off of Material Cultures case study
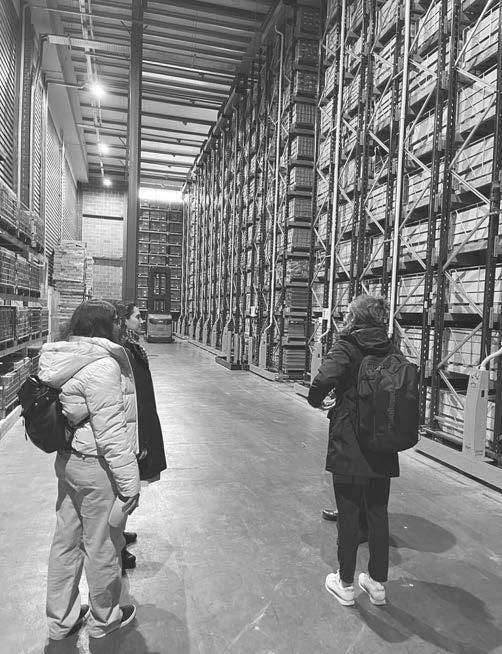
Case Study: Arup & Material Cultures
A recent study by Material Cultures and Arup highlights the potential of biobased materials in new housing developments in the Northeast and Yorkshire region. They exemplify how the materials could bolster local industries, support craftsmanship, and benefit farmers and suppliers on a regional scale.
by Emily Bowerman
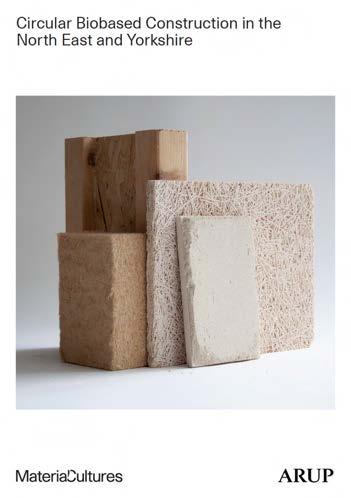
Material Cultures, a London-based architecture and research practice, is a leader in biobased material use in the UK. 2021, they published the report ‘Circular biobased Construction in the Northeast and Yorkshire, documenting their work with Arup in the Yorkshire region. The project targets the new construction of 500,000 new homes in NEY region by 2038 using biobased materials. It addresses biobased materials for structural applications, insulation and lining in buildings; building elements are linked to potential biobased materials and addresses potential procurement networks within the region. The report also presents opportunities of using biobased materials to develop a robust regional network of producers, suppliers, and manufacturers. Overall, the report offers a case example for the potential of biobased materials by addressing environmental impact, economic potential, resource management opportunities, skills development, policy, and funding. This has been a foundational resource for our study.
Upon critical review, we have identified the successes and gaps associated with the MC report. The following section outlines the key accomplishments of the Material Cultures strategy, and it addresses areas that the Cultivating Commons project differs from and advances existing knowledge.
1. REGIONAL NETWORKS & LANDSCAPES OF PRODUCTION
From the MC report, it is estimated that transitioning to biobased materials in housing construction in the NEY region could save up to 2.88 megatonnes of CO2 over the next 17 years. Similarly, the use of biobased construction materials can contribute to lower embodied carbon and reduced environmental impact compared to traditional materials. It is clear from the MC report that biobased materials have low-embodied carbon and have regenerative potentials. The MC report emphasises the importance of evaluating sustainability more holistically, thinking not merely about the use of the material, but also the quality of the landscape from which the material derived.
Opportunities for Cultivating Commons
The report considers the site-specific landscape benefits that biobased materials can provide; the study suggests that best-management practices can be employed on the farms or productive lands to improve biodiversity and produce in a way that is mutually beneficial for the crop/biobased crop and the landscape. Despite this notion, the report does not mention Landscape Architects or Planners within the proclaimed systems-based approach. Cultivating Commons explores a more holistic approach to regional landscapes of production, with an emphasis on establishing a robust green infrastructure network that considers the individual productive landscapes as part of the regional matrix. Scaling-up and adopting a regional landscape perspective and planning policy lens ensures an understanding of how site- specific interventions contribute broadly to the regional landscape health. This is the most critical gap in the MC study.
Why are biobased materials not yet readily used in conventional building practice?
Material Cultures explain that biobased materials can be more expensive than traditional carbon-based materials. Efforts are needed to reduce costs through increased production and economies of scale. The study highlights that start-up costs for housing systems using biobased materials require proportionately smaller demand. For example, a representative of a modular straw housing system in the UK reported that demand for 30 homes or more per year would support a factory in the UK, reducing the need for imports and transportation costs. Moreover, as demand for biobased materials increases, economies of scale can be leveraged to reduce labour costs and make biobased construction more cost-effective.
To accelerate uptake of biobased materials, the report proposes a Biobased Construction Materials Working Group to build awareness, network, exchange skills and knowledge. To meet the growing demands for biobased construction materials, the study also emphasises the need for more growers, manufacturers, and contractors with the necessary skills. The report suggests that funding is essential to allow innovators to access prospective markets, conduct necessary testing, and attract investment. Scaling up the biobased industry will require investment in assets, forming relationships across the supply chain, and providing education and training to meet the demand for a specialised workforce. By investing in skills development and training, regions can ensure that they have the necessary workforce to support the transition to biobased construction practices.
2.TRANSITIONING FROM EXTRACTIVE INDUSTRIES TO BIOBASED
Material Cultures is successful in considering not only the environmental benefits of using biobased, but the economic opportunities associated with establishing a regional biobased supply chain. The report states that building over 500,000 new homes could generate between £0.5 billion to £1.9 billion per year, contributing 10-36% of the region’s total economic output in the housing sector. They also estimate that the total value of output generated through the supply and installation of biobased materials in the region could range from £4.3 billion to £14.8 billion over the specified time. It is evident that regionally produced and procured biobased materials could inject significant amounts of funds and invite investment in agriculture, manufacturing, and construction sectors and create new jobs.
Opportunities for Cultivating Commons
The report identifies many opportunities associated with scaling the biobased material industry, however, they do not address the implications this could have to existing extractive industries. In the case of East Midlands specifically, the region is highly industrialised and many communities are economically reliant on quarries, mines, and other industry. Therefore, Cultivating Commons considers the potential implications of scaling biobased industries and that could result in the potential downscaling of extractive industry. Our project will target upskilling opportunities for the workforce and consider policy implications associated with the closure of industrialised sites in relation to the regional landscape network.
3. MOBILISING AND SCALING BIOBASED
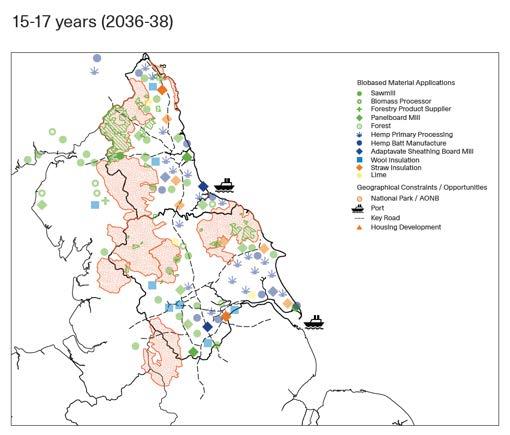
Opportunities for Cultivating Commons
The report successfully identifies the need for a strong network of producers, manufacturers and installers and they enforce the need for funding. Who is willing to invest and what is the recommended funding strategy? Further developing the network conceptualised by MC, Cultivating Commons proposes a tangible framework for mobilising the biobased industry through public common partnerships embedded within a community wealth building framework. The CC project aligns directly with the MC in intent; however, it leverages the BGS, a permanent Government Institution as a springboard for funding upskilling, prototyping, research, and development; innovation and technologies; and optimising their network. The CC project proposes a rigorous policy framework to identify anchor institutions, stakeholders and actors, roles, and responsibilities to fund, facilitate and support the uptake of biobased materials at all stages. Not only will the project provide a supply chain analysis, but also identify who, what, where and how the individuals and organisations involved contribute and benefit from participating. This builds on the work of MC by prototyping examples of funding streams and what roles council, local government, and UK institutions play.
4. POLICY & PROCUREMENT
What is the role of designers and policymakers for encouraging biobased materials?
Material Cultures identify some of the major barriers to procuring and using biobased materials including lack of standardisation of material performance and insurability. Moreover, the study
notes that competitive tendering processes challenge contractors to limit costs to win bids. In a labor market where costs are rising and skills are in short supply, cutting material costs can be a strategic compromise for contractors. Until legislation and regulation promote greater use of biobased materials and market prices adjust, contractors may face challenges in assuming the risk of higher cost, lower carbon materials.
Opportunities for Cultivating Commons
Cultivating Commons is focused on the application of biobased materials in institutional scale projects. Therefore, these projects follow a standardised tendering process.
Acknowledging that many biobased contractors are small, privately owned businesses at this stage, it would be critical to reform the UKRI tendering process to divide tenders into smaller contracts – enabling smaller-scale businesses to access the tendered projects from the BGS. Moreover, the BGS will serve as a prototype for biobased material use. If successful, the demand for biobased could increase, therefore more workers and contractors will be needed; the industry demand could exceed the available skilled-labour. These are considerations that will be further explored in Term 3.
According to the RIBA – Plan of Work, we will be identifying the roles and responsibilities of actors in specifying, sourcing and constructing with biobased materials. This will build on the works of MC to demonstrate how biobased can be integrated and the implications within a conventional project development framework.
INSURANCE
The report also identifies three key insurance scenarios that restrict the use of biobased materials including Professional Indemnity Insurance that protects design professionals, including architects and their clients on design projects. The availability of insurance for using biobased materials is essential for designers to specify and design with these materials. Data, testing, and legislative support are necessary to ensure insurance coverage for designers working with biobased materials. Secondly, manufacturers may struggle to provide warranties and technical data to ensure compliance with building codes and regulations. Designers rely on this information to demonstrate compliance and meet the requirements of home insurers and mortgage providers. Supporting small and medium enterprises (SMEs) in accessing funding for testing and compliance can help address this challenge. Lastly, insurance costs can be prohibitive for buildings constructed with biobased materials.
Opportunities for Cultivating Commons
We currently understand that insurance remains a barrier to using biobased materials in conventional construction. The example of Nottinghamshire University Campus building that uses straw-bale construction will be further investigated to understand how they were insured, the process for acquiring insurance, and cost if information is available.
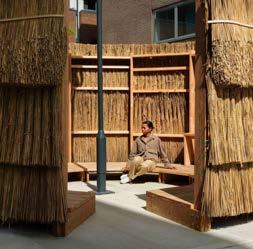
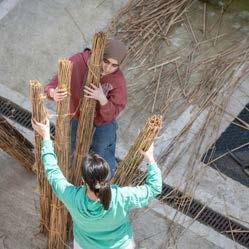
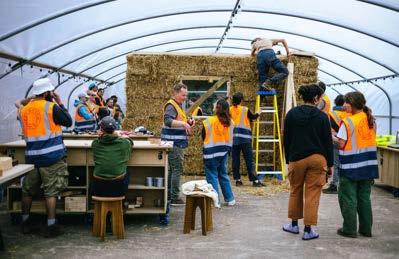
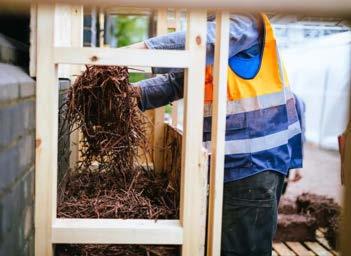
Building Community Wealth through Public Common Partnerships (PCPs)
Circular supply chains, Cradle-to-Cradle,
by Emily Bowerman
Procurement of conventional building materials follows a linear economic model opposed to the proposed bioregional economic model outlined in the previous section. Conventional economic models, such as capitalism, prioritise economic growth, are profit driven and strive for market integration. These models often overlook environmental limits, social equity, and long-term sustainability in favor of short-term gains and market efficiency. The neglect of local ecosystems negatively impact community resilience and cultural heritage in favor of economic expansion. Ultimately, conventional economic models prioritise growth and expansion with limited acknowledgement for environmental limits or ecological capacities of the landscapes they influence.
Cultivating Commons explores alternative economic models that counter conventional linear economics driven by extractive and exploitative. According to the Doughnut Economics model by Raworth (2017), social well-being and environmental sustainability are highly interdependent.1 This means that human well-being and environmental well-being are explicitly linked –therefore, our approaches to meeting social and economic needs must account for the impacts on environmental systems that sustain us. To implement this, material supply chains such as those developed by Material Cultures (2021) follow a Cradleto- Cradle approach that considers the means and methods of producing and using a material as equal importance to its disposal, composting, or reuse. 2
Aligning with Raworth’s social foundation, to promote distributive economic development, UK initiatives such as Community Wealth Building and Public Common Partnerships (PCPs) offer progressive methodologies for achieving equitable development regimes and mobilising a Cradle-to-Cradle strategy. Community Wealth building looks at opportunities for forming partnerships with anchor institutions so project goals can be realised in a way that benefits local community economies more broadly such as providing employment, upskilling. For example, the retrofitting of public institutions seeking to lower emissions can procure materials within the local region, supporting local producers, craft and business –the public project propels local industry and invests more broadly in the community whilst achieving project goals.3
To mobilise the community wealth building approach, Public Common Partnerships (PCPs) can be established between local councils, educational institutions, and government institutions such as the BGS. PCPs are not a strict regimen but rather a series of principles and processes that need to be designed and implemented on a largely case-by-case basis. Conventional procurement models typically involve Public-Private Partnerships (PPPs) that combine “the deployment of private sector capital and, sometimes, public sector capital to improve public services or the management of public sector assets”.4 In the case of the BGS retrofit, a PPPs would involve the procurement of materials and services from a private sector firm who would tender the project, a builder would be appointed, and materials would be procured from conventional suppliers of concrete, insulation etc. (oversimplified for the purpose of this summary). Alternatively, to redistribute wealth so government asset improvement projects benefit communities more broadly, a Public Common Partnership model could form networks between government institutions, research institutions and councils. These partnerships could forge way for communities, businesses, and local suppliers and crafts people to benefit from the procurement of biobased materials whether as suppliers, manufacturers, research and development personnel, skilled-contractors or upskilled employees.
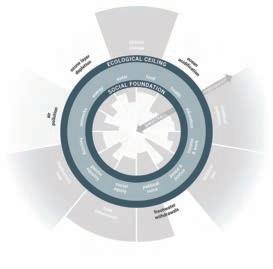
From speaking directly with Kai Heron of ABUNDANCE on April 15, 2024 we understand that PCPs require a shift in values; whereby the acquisition of building materials needs to equally consider the broader impacts production and procurement of materials has to the landscape and community at large.
What would incentivise anchor institutions such as local Universities or UKRI to promote a Cradle-to-Cradle approach and engage in PCPs for the procurement of materials?
From our interview with Kai Herton, it is understood that councils may engage in PCPs to incentivise jobs and taxes that improve the councils’ image and economic viability. Furthermore, PCP’s might fulfil universities or educational institution’s sustainability agendas; universities may also partner to provide field courses related to biobased materials or develop training and internship programs associated with the R&D or construction of biobased materials.
Mobilising alternative economic models for material procurement from a Cradle-to-Cradle lens is complex. The novelty of biobased material use in the UK presents challenges related to uptake and interest in investing. Because of this, prototyping the BGS retrofit project becomes even more critical to demonstrate the feasibility and opportunity that comes from engaging in a biobased economic system of production for both communities, contractors, councils, educational bodies and government Institutions.
1 Hill-Hansen, Dani, Guldager Kasper Jensen, and Kate Raworth. Doughnut for Urban Development: A Manual. Copenhagen: Danish Architectural Press, 2023.
2 Material Cultures, and Arup. “Circular Biobased Construction in the North East and Yorkshire, 2021.” Material Cultures. Accessed April 21, 2024. https://materialcultures.org/2021-circularbiobased-construction-in-the-north-east-and-yorkshire/.
3 Radcliffe, Eleanor, Laura Williams, Victoria Bettany, Amy Clancy, Matt Fawcett, and Amanda Stevens. “Part 1: Why Community Wealth Building?,” 2021.
4 PMC, Europe. “Promoting Urban Farming for Creating Sustainable Cities in Nepal.” Europe PMC, January 9, 2023. https://europepmc.org/article/ppr/ppr594002.
5 Kai Heron, interview by Priyanka Awatramani, April 15, 2024.
Supply Chain Diagram
As addressed in the section on biobased materials, we understand that materials have a carbon footprint from propagation through to disposal. Building on the works of Material Cultures, we have identified opportunities for integrating strawbale insulation with timber framing, and kelp acoustic panels. This approach optimises all aspects of the supply chain to serve either an environmental or economic benefit to the local community through production, manufacturing, construction, and disposal processes. In term 3, we will further develop the
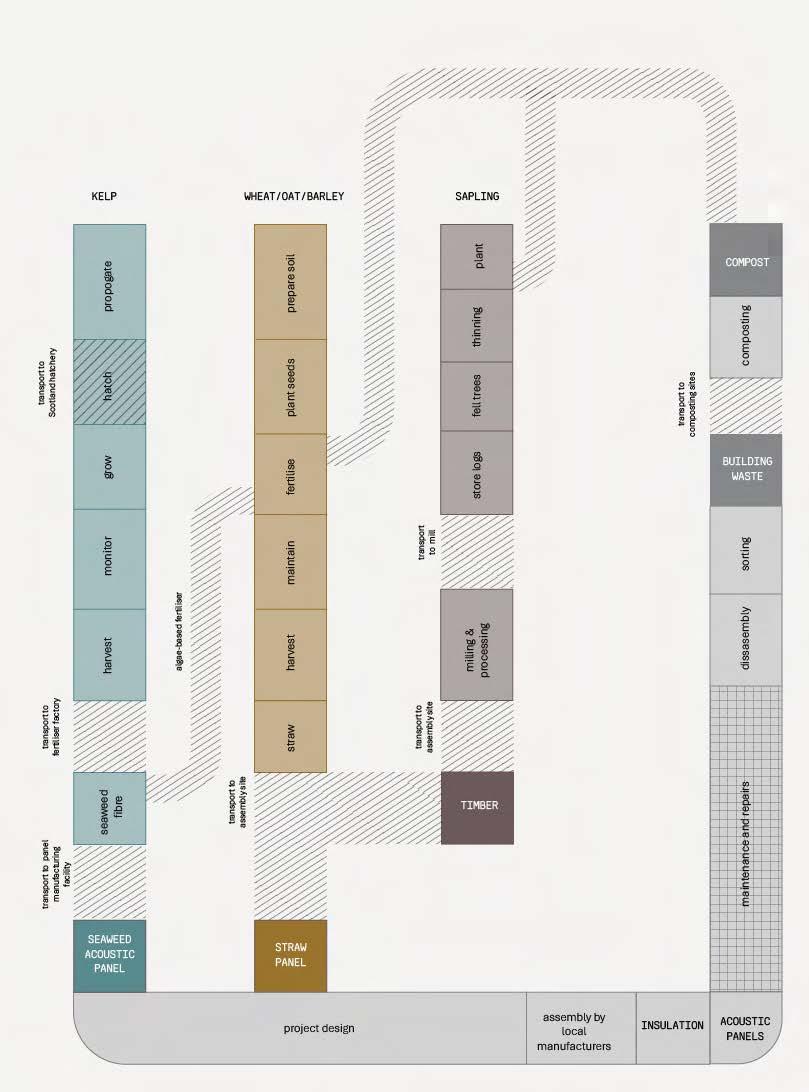
Supply Chain Network for BGS
To spatialise the potential supply chain network for the BGS, we mapped the existing cereal croplands, the existing seaweed farms nearby to the region, and the shellfish farms with potential for expanding to farm seaweed. In the short-term, seaweed farms could supply fertilisers for the production of cereals, of which straw is a by-product. Also identified on the map are suppliers, manufacturers, and contractors with existing biobased construction knowledge. This map provides foundational understandings of how the materials could be procured currently this reflects the methodology employed by Material Cultures.
How can landscapes of production be considered at a regional scale to ensure they provide economic benefits whilst integrating holistically with the existing ecosystems and landscapes?
Building on this network, we will explore how the sites of production and procurement can form a regionally regenerative land management system.
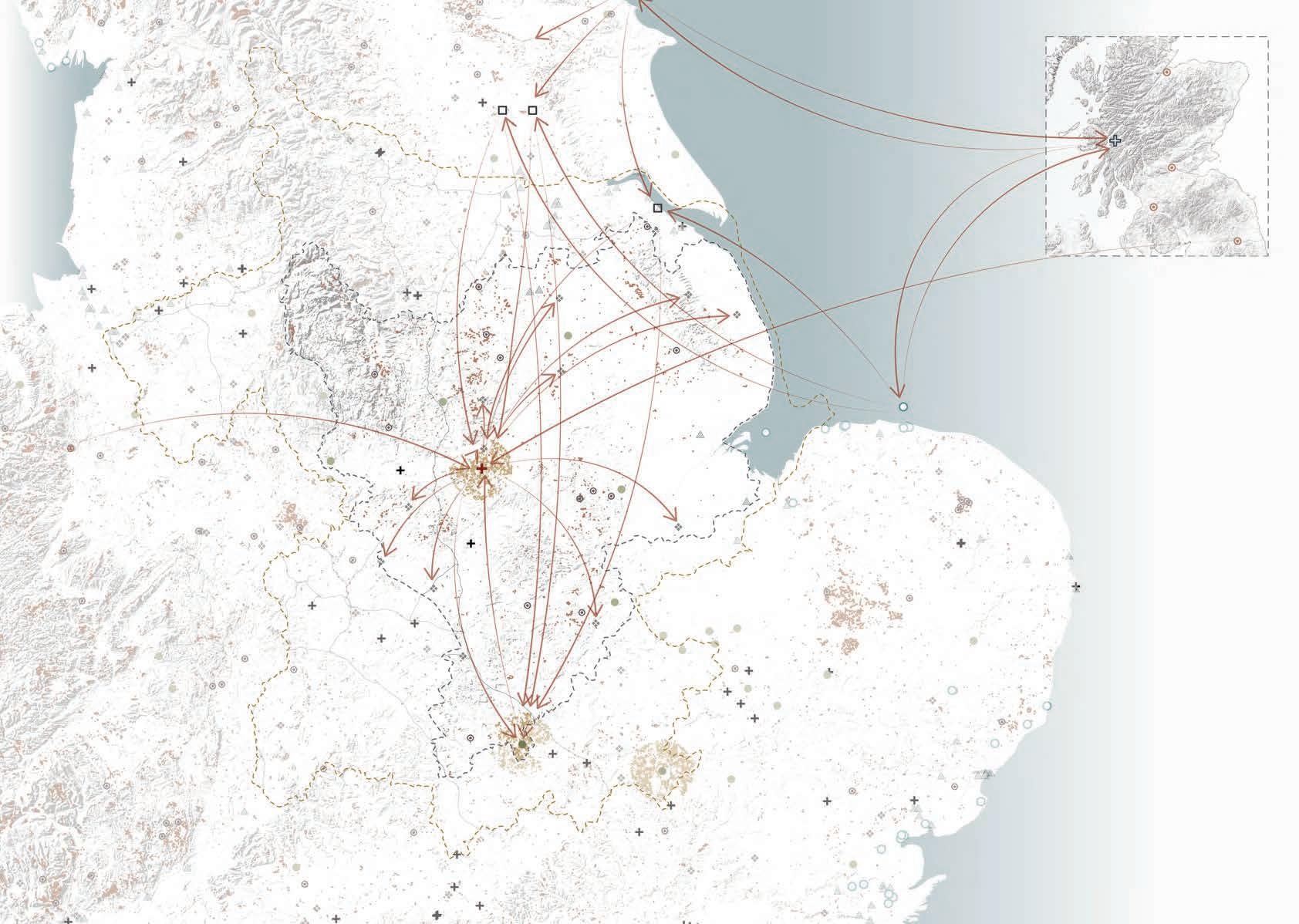
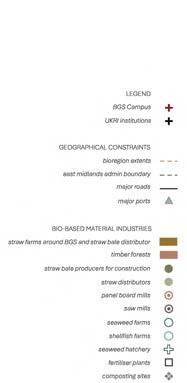
Establishing a local bioeconomy through Public Common Partnerships (PCPs)
“Public-Common Partnerships are a way for organised communities, working in partnership with relevant public bodies, to gain ownership and control over the assets and resources (buildings, enterprises, farms) which impact their lives” (Abundance, 2024).
by Alejandra Iturrizaga Andrich
The local workforce, aiming to transition from traditional mining jobs to roles within the green economy, is at the forefront of a new initiative to form partnerships with regional producers, including farmer clusters, fishermen, and seaweed and shellfish groups. This coalition, supported by the British Geological Survey (BGS) advances through an extensive retrofitting project. Together with local councils and universities, BGS acts as an anchor institution, offering government-owned land, upskilling and education opportunities, and leveraging grants and incentives to foster local businesses that supply biobased construction materials. In our efforts to understand how collaborations between BGS, local councils, and institutions could be formalized, we consulted experts in Public-Common Partnerships (PCPs), including Kai Heron from Abundance. Our proposal extends the PCP model to include designers and consultants already active in these fields, ensuring comprehensive guidance and support throughout the transition.
Find the BGS Handbook in the pocket on the back of the book.
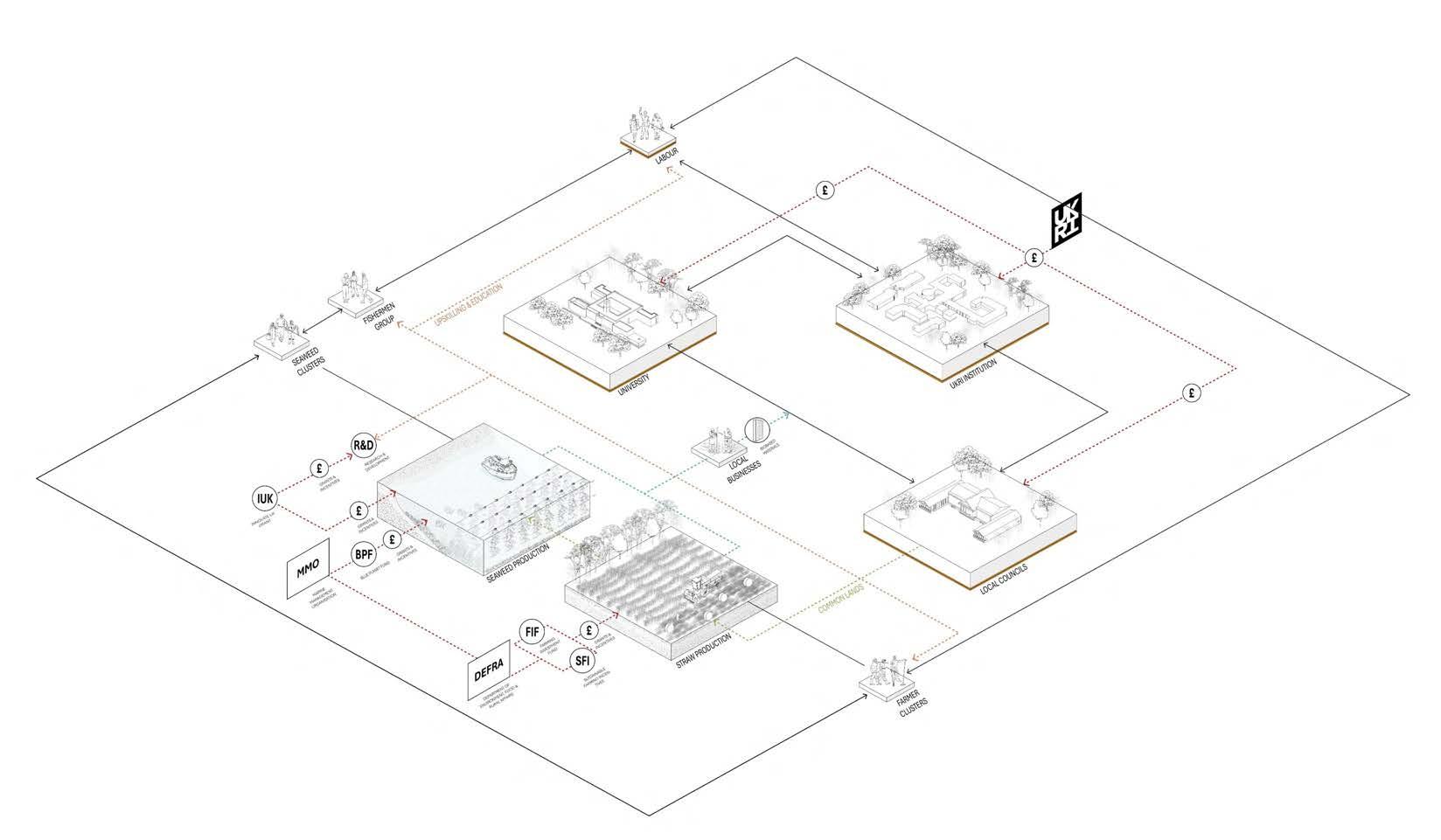
Bio based Retrofitting of UKRI Institutions through local
Public Common Partnerships
(PCPs)
Executive Summary
In 2019, the UK government set a target to achieve a 100% reduction in greenhouse gas emissions by 2050. (ref. Climate Change Act 2008). This necessitates retrofitting campuses of public institutions such as the British Geological Survey (BGS) of UKRI. However, conventional retrofitting often depends on carbon-intensive building materials, contributing to 40% of global emissions, according to the UK Green Building Council. Consequently, emissions from material extraction and production have adverse effects on local well-being and ecologies.
To better this goal, this policy mandates the use of locally produced biobased materials, which offer low embodied energy and in some cases, even contributes to carbon sequestration or soil regeneration. This approach not only supports UKRI’s net-zero targets but also benefits the regional communities (of East Midlands) by promoting a transition away from traditional production and extraction practices.
This paper highlights policy gaps in decarbonisation efforts, inadequacies in building codes, and challenges in retrofitting practices, emphasising the need for holistic approaches to construction. It also identifies disparities in the biobased materials industry and proposes strategies to address them, including promoting biobased materials in building codes, investing in research and capacity building, leveraging financial resources, and utilising public lands for research and development, through Public Common Partnerships (PCPs). These recommendations aim to enhance awareness, support funding, overcome resistance to change, and ensure sustainable procurement practices, ultimately advancing the transition to biobased construction materials and achieving broader environmental and social goals.
The Issue
1. Policy Gaps in Decarbonisation
The drive towards decarbonization in the UK, with a target of achieving Net Zero by 2050, highlights the urgency of addressing the gaps in existing policies. While policies predominantly focus on reducing carbon emissions, they often overlook the intricate interplay between the construction sector, environmental preservation, and human health.
2. Inadequacies in Building Codes and Sustainable Practices
The inadequacies in building codes become apparent when considering the incorporation of bio-based materials. Existing frameworks including non-governmental actors such as BREEAM, predominantly prioritize carbon reduction. evaluating environmental performance of a while overlooking its broader social and economic impacts.
3. Retrofitting Challenges
Despite its potential to mitigate environmental impact, the prevalent approach to retrofitting primarily focuses on reducing operational energy. However, this approach leaves significant room for improvement and fails to address the issue of construction materials ending up in landfills, necessitating a more comprehensive strategy.
4. Neglect in material procurement
In retrofitting projects, material sourcing practices often ignore factors such as embodied carbon, production methods and manufacturing locations. This narrow focus obstructs the holistic advancement of the construction industry towards conscious practices.
5. Failure to address the implications of extractive Construction Materials
The issue of extractive construction materials remains largely unaddressed in both decarbonization efforts and existing policies. This oversight perpetuates the environmental impact of material extraction and exacerbates challenges in achieving sustainable construction practices.
6. Assessing Variation in Biobased Material Industries
(Bio-based materials studied: Timber, Clay, Straw, Hemp, Seaweed and Mycelium) Each material presents unique requirements in research and development, with some necessitating restrictive licensing for scaling up production. And hence, specific policies are required for each material, to address the challenges hindering the integration of these materials into the construction industry as mainstream building materials.
The Challenge
1. Limited Awareness and Understanding of PCPs
Lack of awareness and understanding among stakeholders regarding the concept and potential benefits of Public Common Partnerships (PCPs) could impede successful implementation. [Addressed in Recommendation #2]
2. Securing Funding Sources
Relying only on public funding may pose challenges in ensuring adequate financial resources for large-scale biobased retrofitting projects. [Addressed in Recommendation #2]
3. Overcoming Resistance to Change, Research and Verification
Resistance from stakeholders, including institutions and local communities, towards adopting biobased retrofitting methods and transitioning from traditional practices is prevalent. Additionally, there is a problem with lacking research in materials, which impacts the ability to obtain insurance coverage as builders need verified materials to comply building codes and insurance companies checks. [Addressed in Recommendation #2 and #3]
4. Balancing Local Procurement and fair bidding process
Establishing a fair and transparent bidding process for the procurement of biobased materials without imposing restrictive distance radius requirements. [Addressed in Recommendation #1]
5. Technology and Innovation gaps
Limited technological advancements or innovations in the
This policy initiative focuses on leveraging biobased materials to transition from carbon-intensive construction practices aiming to promote sustainability and support regional economies. This policy facilitates local production, fabrication and procurement of biobased materials for retrofitting public institutions through Public Common Partnerships (PCPs). These partnerships enable the adoption of biobased materials in retrofitting projects, contributing to both regional community economies and ecologies. The policy mandates the formation of PCPs, fostering collaboration between the UKRI, local councils, universities, and communities to mobilize the transition to biobased materials. A PCP serves as a joint enterprise that in this context would include the BGS as an anchor institution local council, local businesses and community groups to catalyze industry advancements. The BGS retrofitting project is pivotal to implement the initiatives under this policy that include funding upskilling programs for local workers and farmers, supporting education, and facilitating research and development initiatives on common lands. Common lands include council farms, protected landscapes and brownfields are utilized as test plots for biobased material production. This not only showcases the uptake potential to local farmers and producers but also fosters innovation and sustainability in regional economies.
[After mapping and analyzing the potentials of six bio-based materials for each region of the UK, we have chosen to focus on seaweed and straw for prototyping the case of BGS. Straw was selected due to its easy availability in the East Midlands region, while seaweed presents a promising opportunity as a long-term industry that can transition from a fertilizer to a construction material with further research and development.
It is important to note that the choice of materials may vary for other institutions depending on regional production capabilities and future interests in scaling up industries. Therefore, the selection of seaweed and straw for the BGS’s prototype does not imply that these materials are universally applicable or that they alone can transform the construction industry. This allows us to acknowledge the potential of specific materials within our context while recognizing the dynamic nature of regional production and evolving industry interests.]
Policy Recommendations
»
»
development and prototyping of biobased material production and use.
» Make county farms available for testing farming methods of biobased materials.
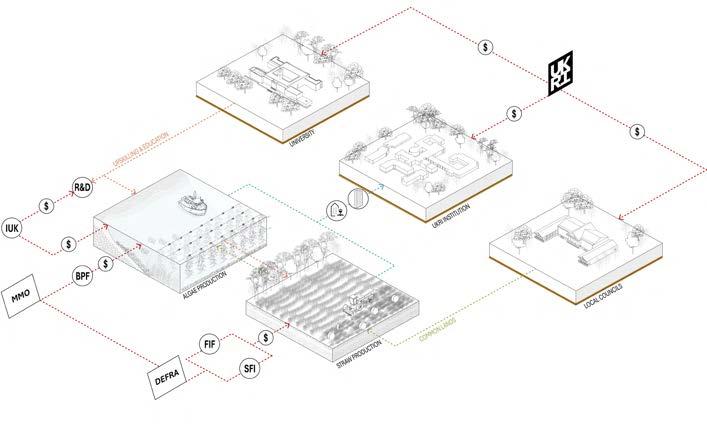
1. Promotion of Biobased Materials in
DEVELOPING A REGIONAL PRODUCTIVE LANDSCAPE STRATEGY
proposal for East Midlands bioregion for propelling biobased initiatives as a phased strategy
Bioregional Economics
Adopting a bioeconomic approach to establish biobased material production in the East Midlands
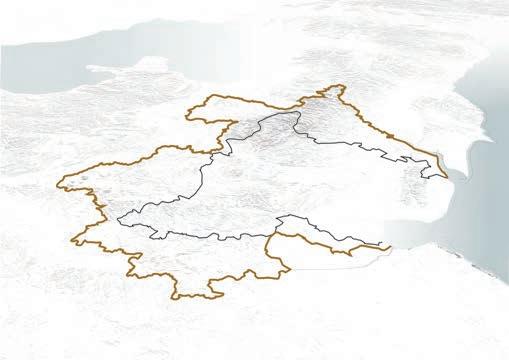
Building on the works of economist Molly Scott Cato, we have followed a bioregional approach to advance our understanding of regional systems beyond mere administrative boundaries.
Bioregions are defined according to watersheds, climate patterns, and biodiversity to acknowledge the interconnectedness of ecosystems and communities. 1 Following bioregional boundaries acknowledges the interrelationships of human activity and natural resources within a particular area; administrative boundaries often do not reflect the ecological and hydrological characteristics of a region.
Bioregional frameworks promote a holistic understanding of the region, emphasising the importance of sustainable resource management, preservation of ecosystem services, and adaptation to environmental changes accelerated by climate change. This project acknowledges the unique inland and coastal conditions that shape the East Midlands region; these landscapes are key indication of the opportunities and potential limitations of producing biobased materials. The study defines the East Midlands region according to the bioregional boundary that reaches from sea to land, crossing varied topographies and micro-climates.
Why transition to a Bioregional economy?
Bioregional economies support a more harmonious relationship between community economics and natural resources. Aligning human activities with the region’s inherent natural resources and ecological functions promote sustainability and resilience. For example, regenerative agriculture could provide materials required for construction whilst considering the health and longevity of soils and lands. This approach contrasts existing extractive practices of mines and quarries, relied upon for conventional building materials. Focusing on regional sustainability lessens reliance on global supply chains for goods and services.
Bioregional economies also encourage a sense of pride and community identity, whereby individuals within a particular
region adopt stewardship responsibilities and identify with the landscapes that they rely on. Rather than resources coming from afar, disconnected from their sites of production and the labour involved, individuals are more integrated within the production and use of resources directly within the area they inhabit. Ultimately, embracing bioregional boundaries not only acknowledges the interconnectivity of human and ecological activities but also offers an approach towards sustainable, resilient, and culturally rich communities.
What are some of the limitations of a Bioregional approach?
The transition to bioregional economies is not without challenges. Due to the incongruencies with political boundaries and bioregional extents, political resistance could ensue due to conflicting interests in governance strategy of natural resources. Moreover, coordinating actions across various jurisdictions within a bioregion could present logistical difficulties – this demands a reformed understanding of responsibilities around natural resources and collective agreements for managing lands and resources. This will be further explored in Term 3, specifically to overlay political actors and bioregional resources for the prototyping of the BGS.
Adopting a Bioregional approach in the East Midlands
Adopting biobased material production, manufacturing, and processing for use in the BGS retrofitting project follows a two-phase approach at both the East Midlands scale and the Nottinghamshire scale. The phased approach is as follows:
by Emily Bowerman
East Midlands boundary Extents of bioregion
Upscaling industries in East Midlands: Phase 1
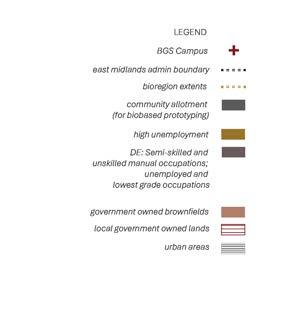
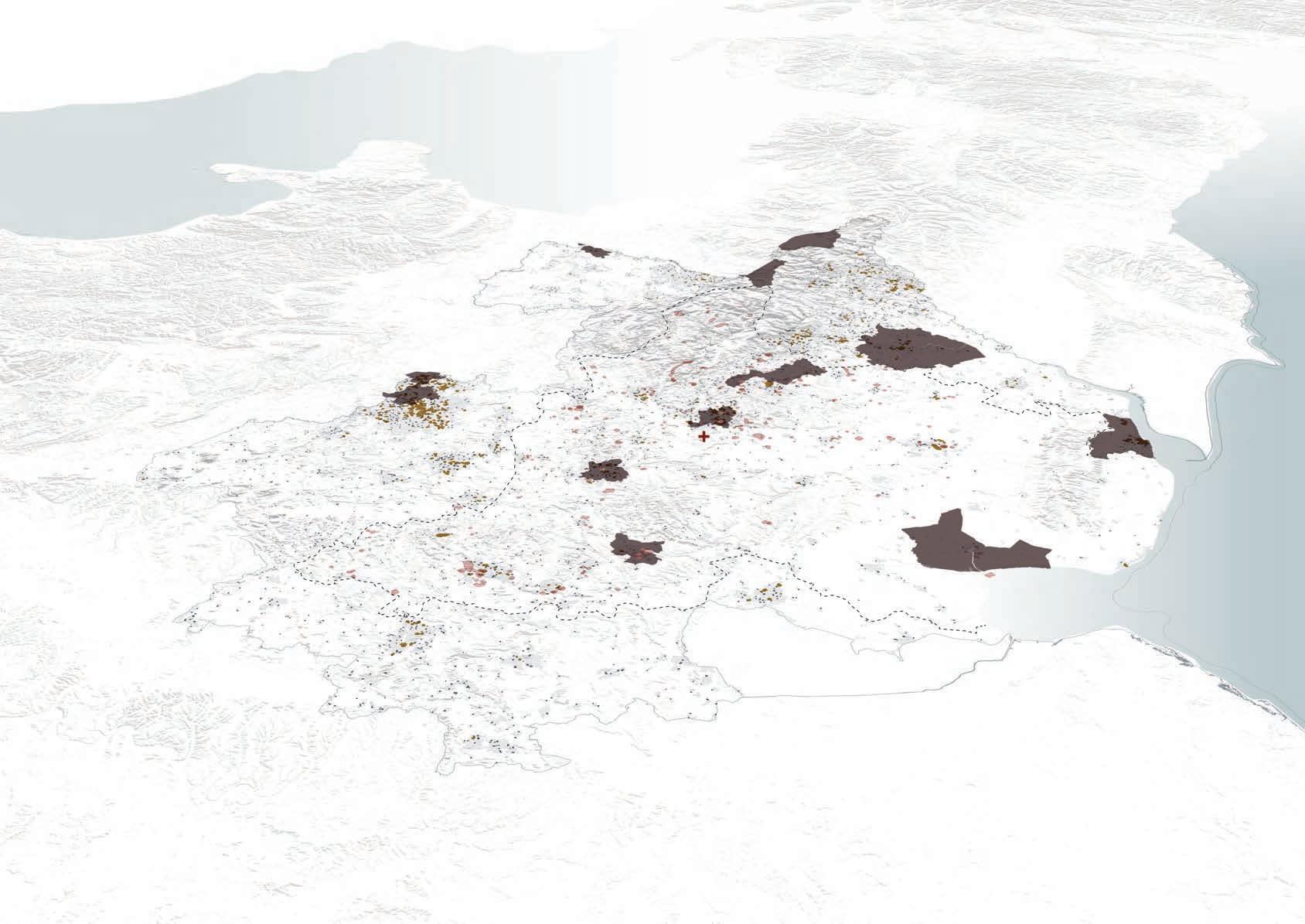
Upscaling industries in East Midlands: Phase 2
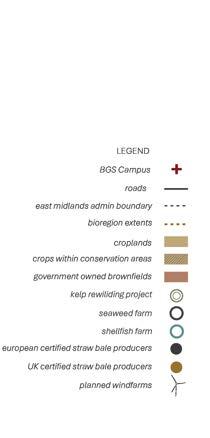
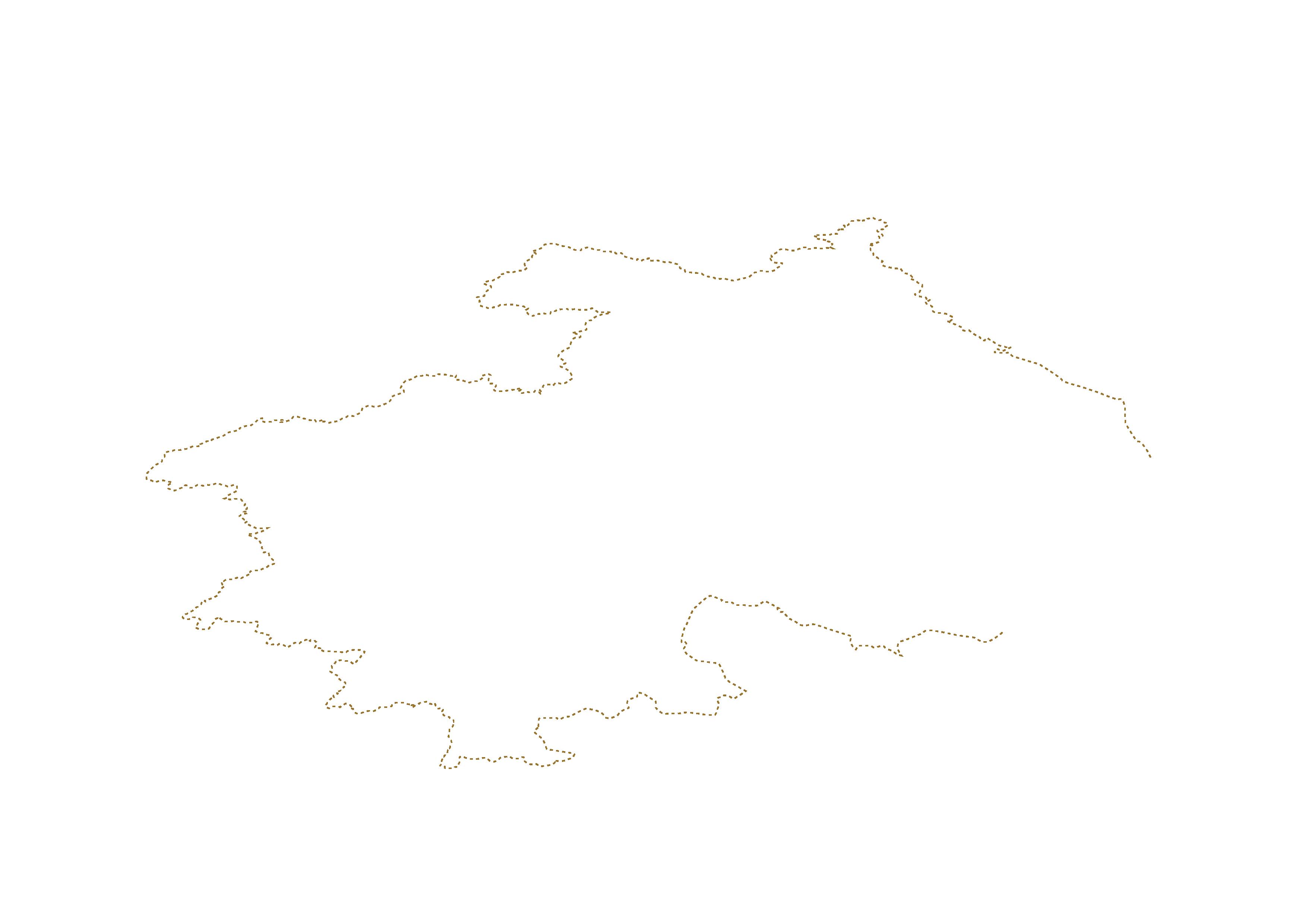
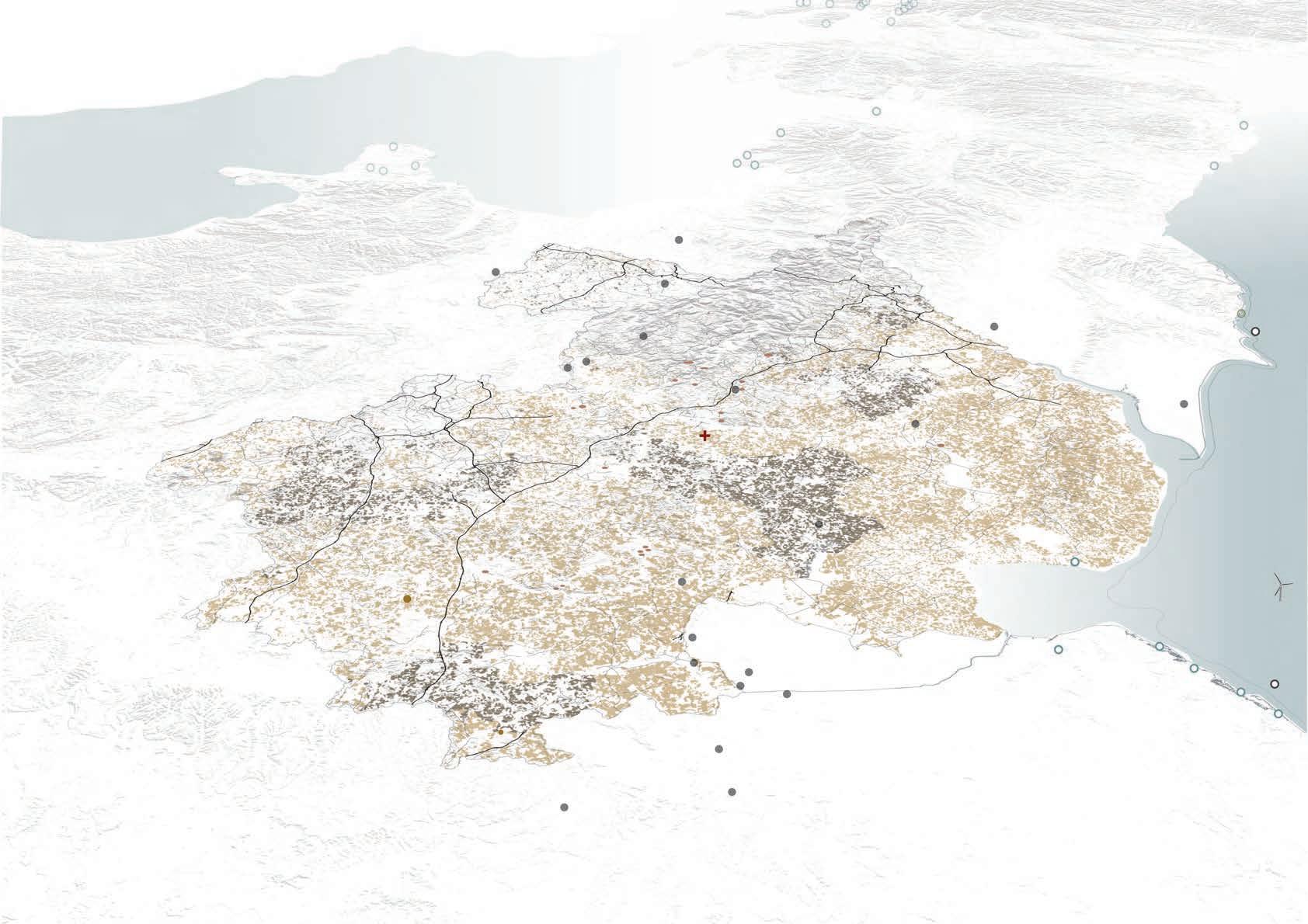
Nottinghamshire: Phase 1 & 2
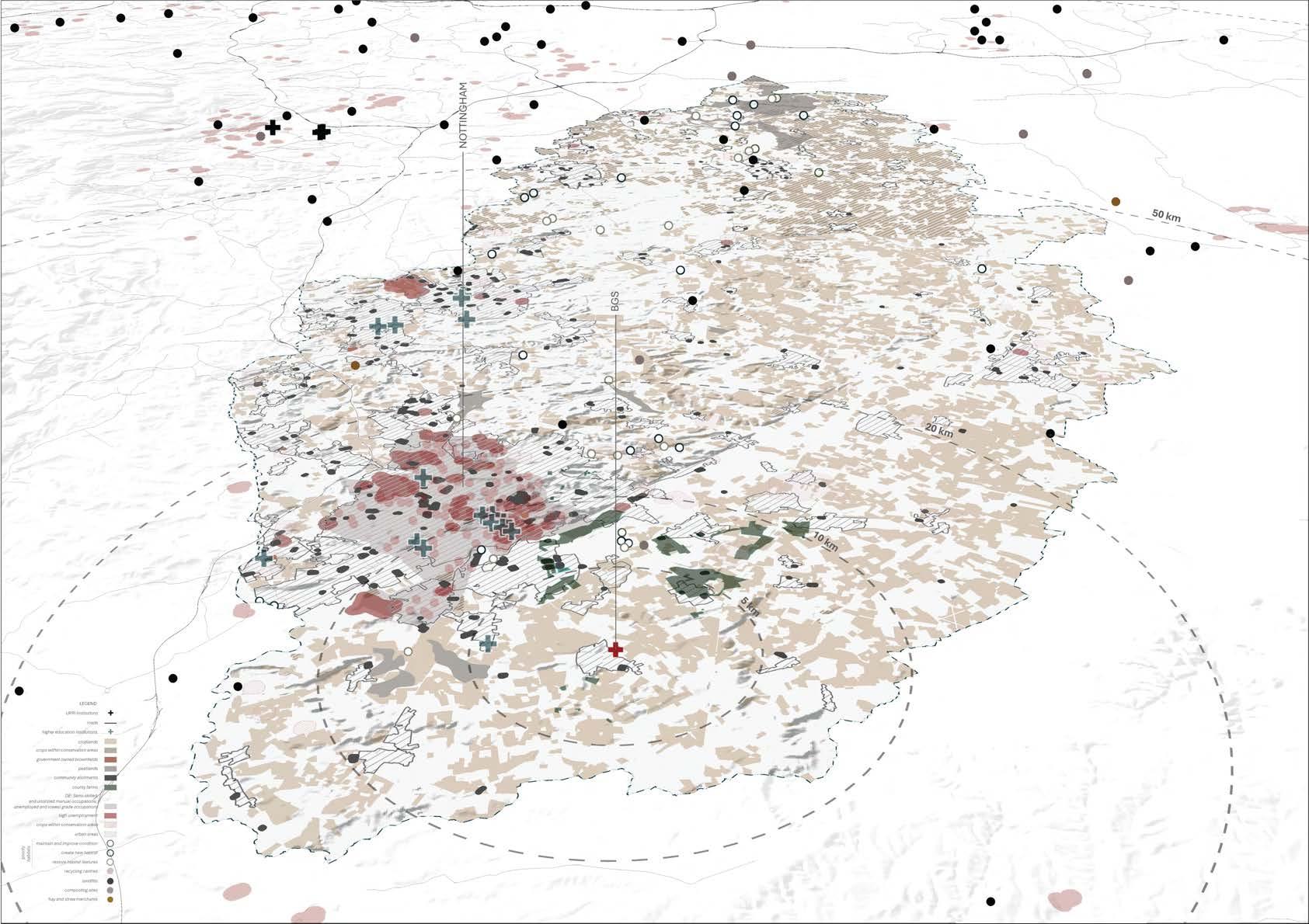
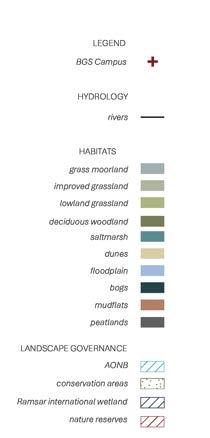
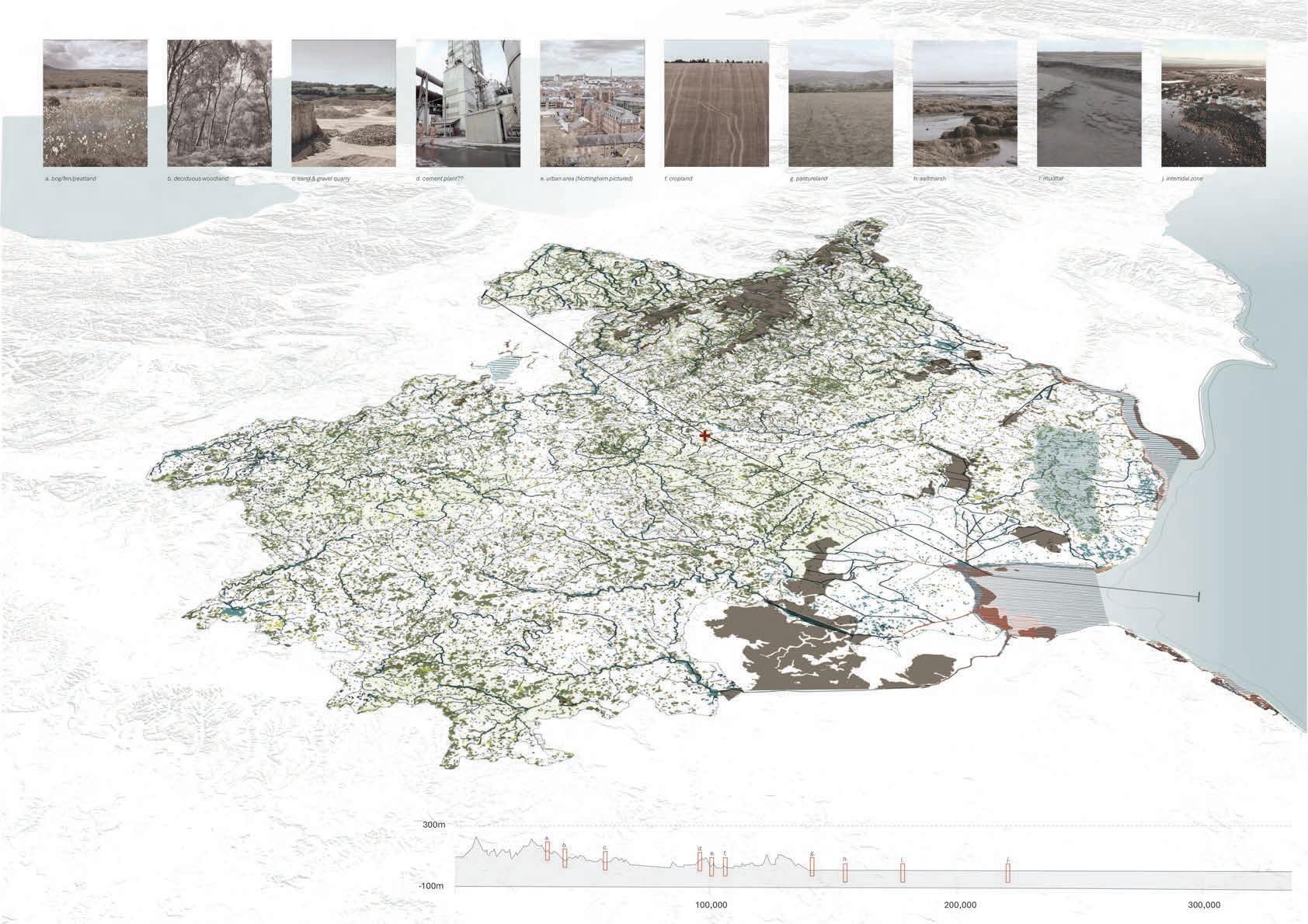
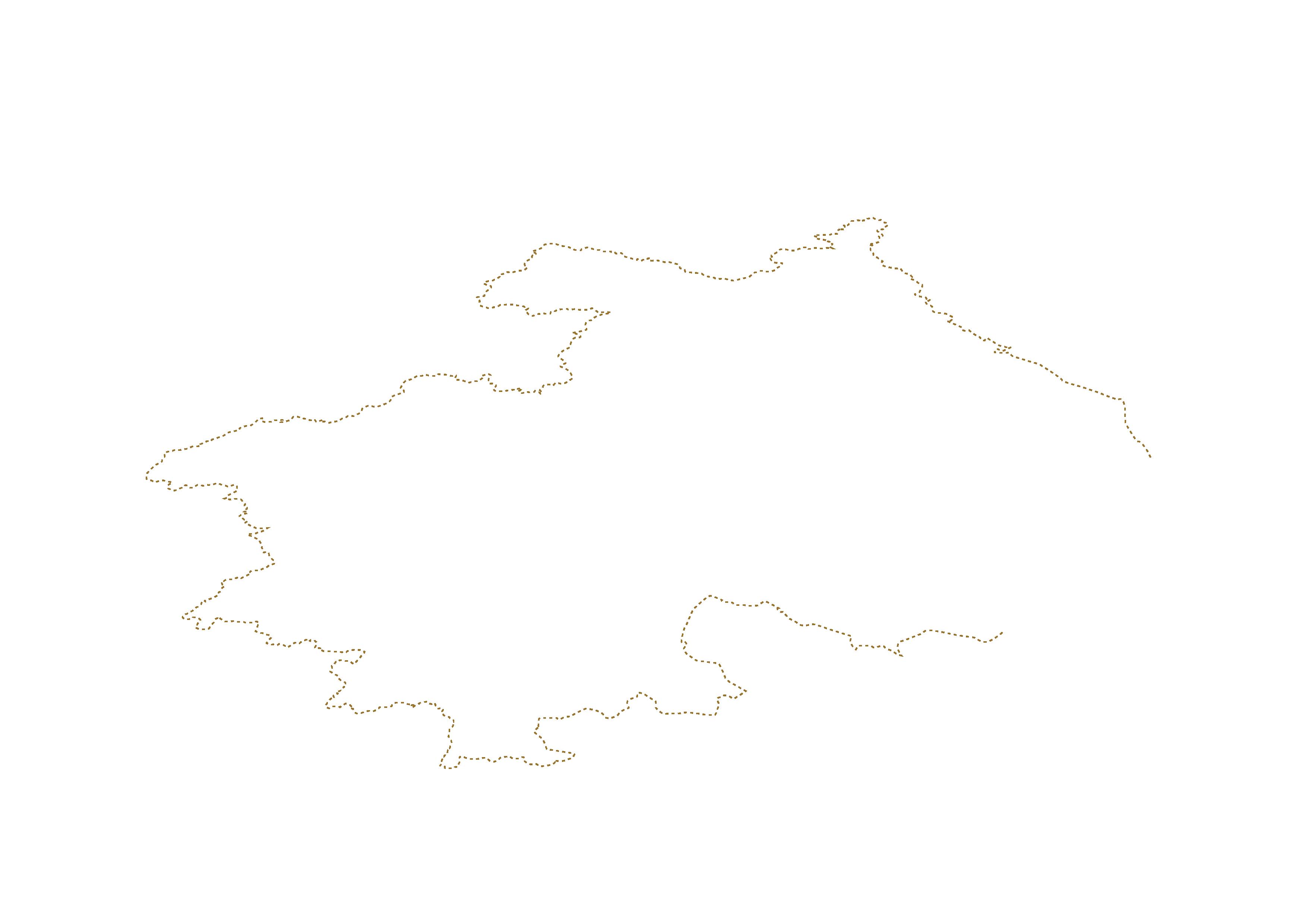
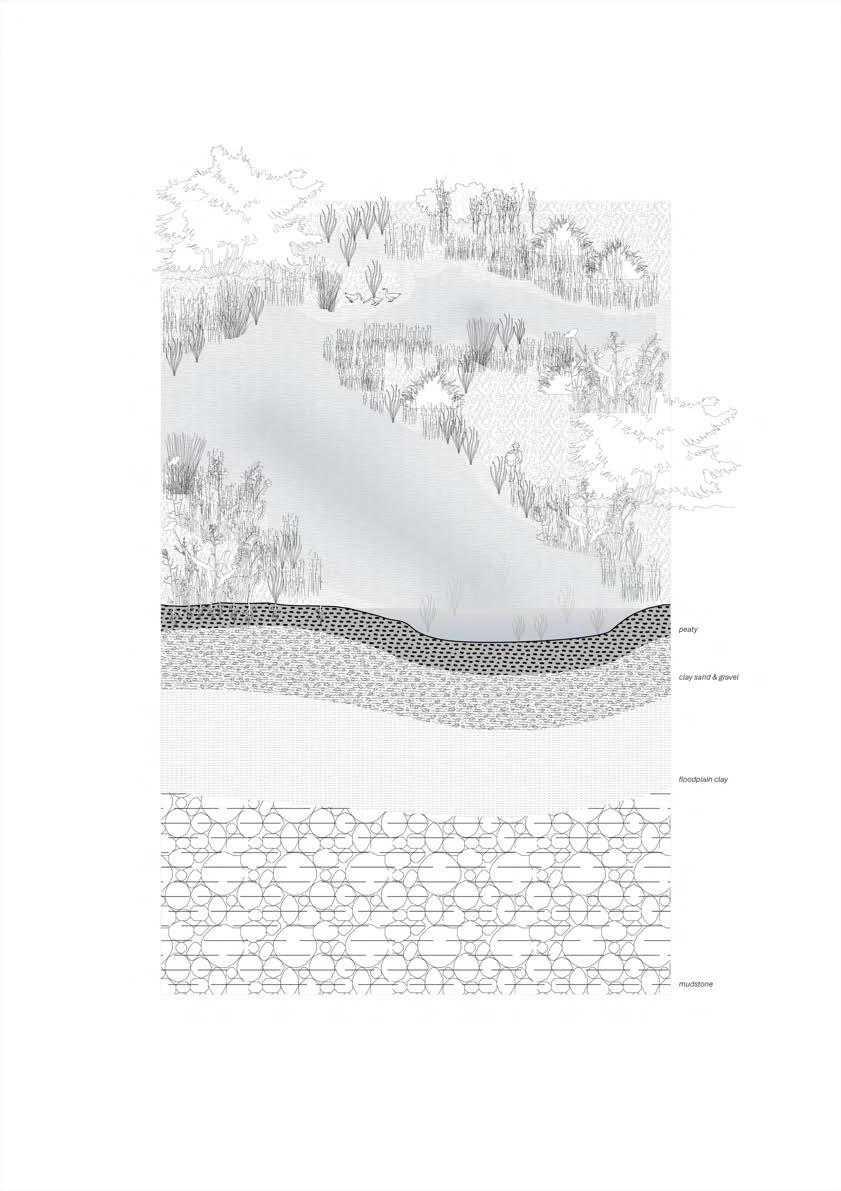
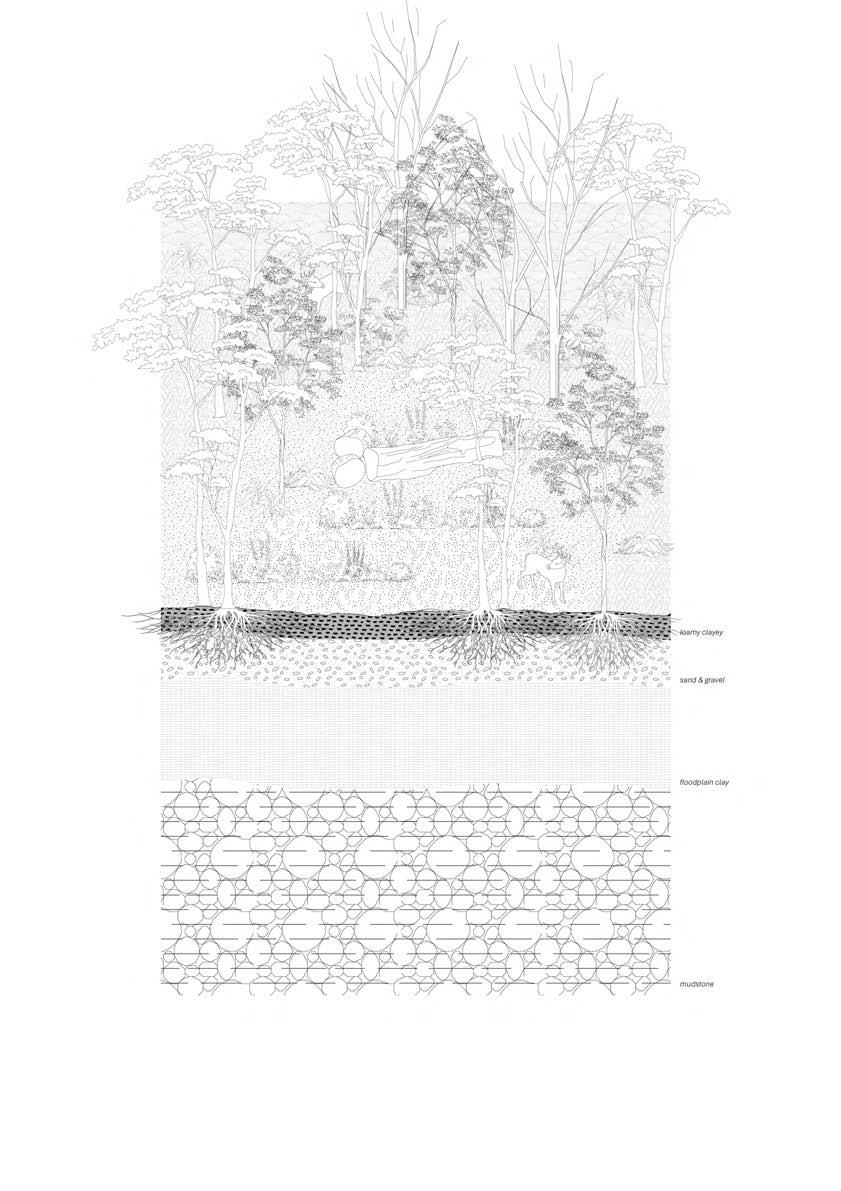
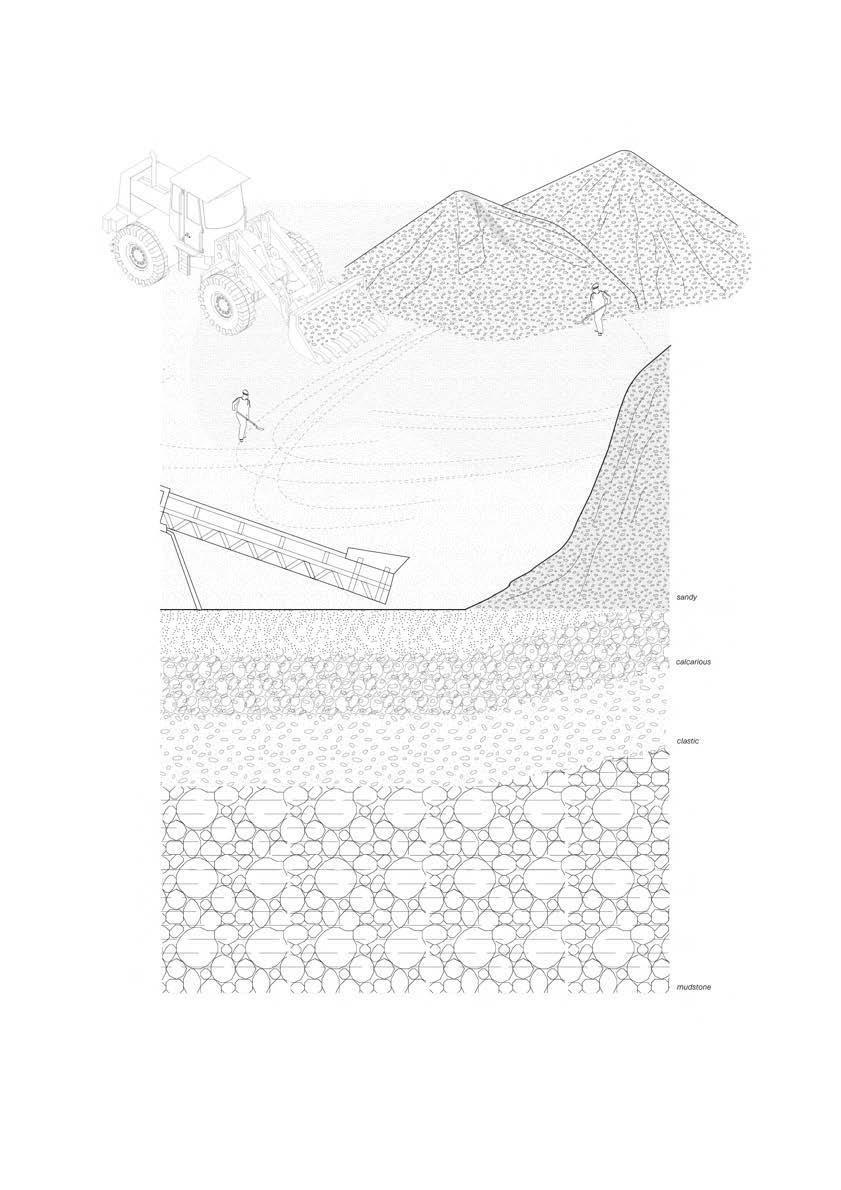
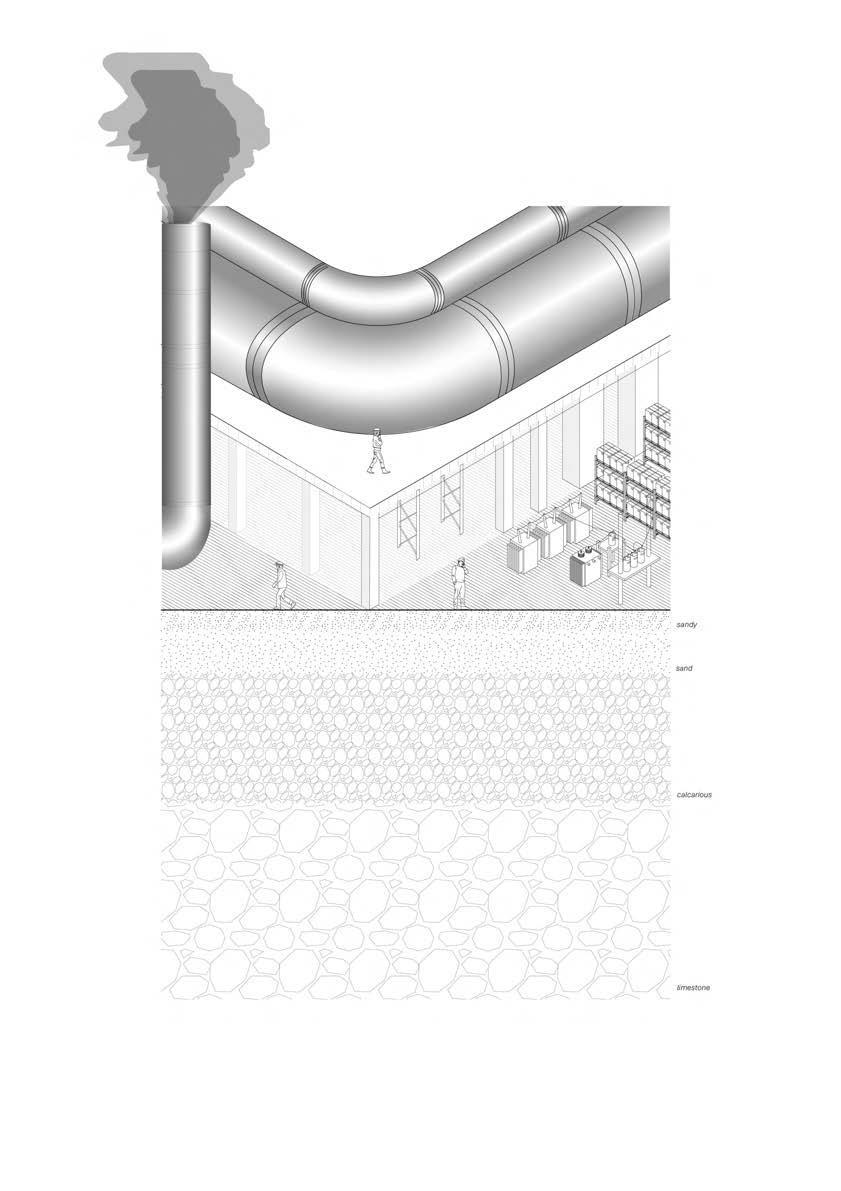
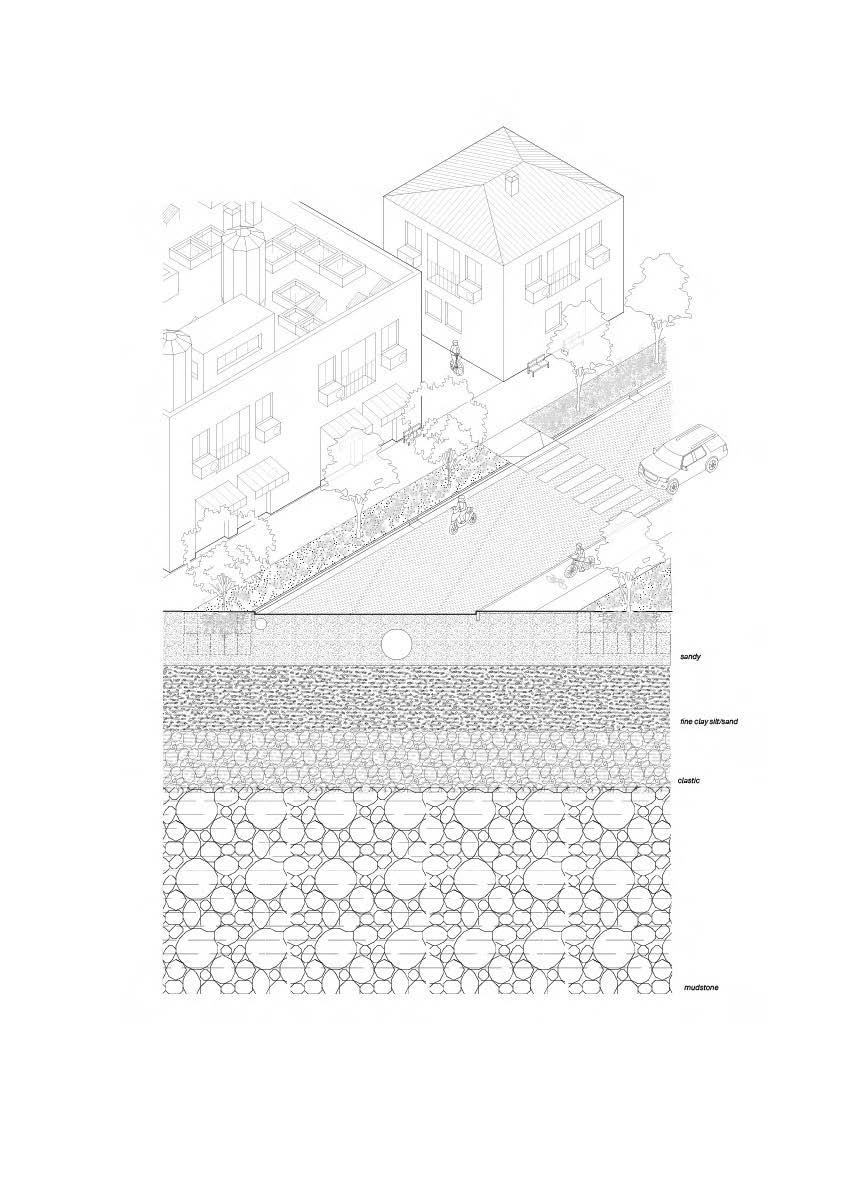
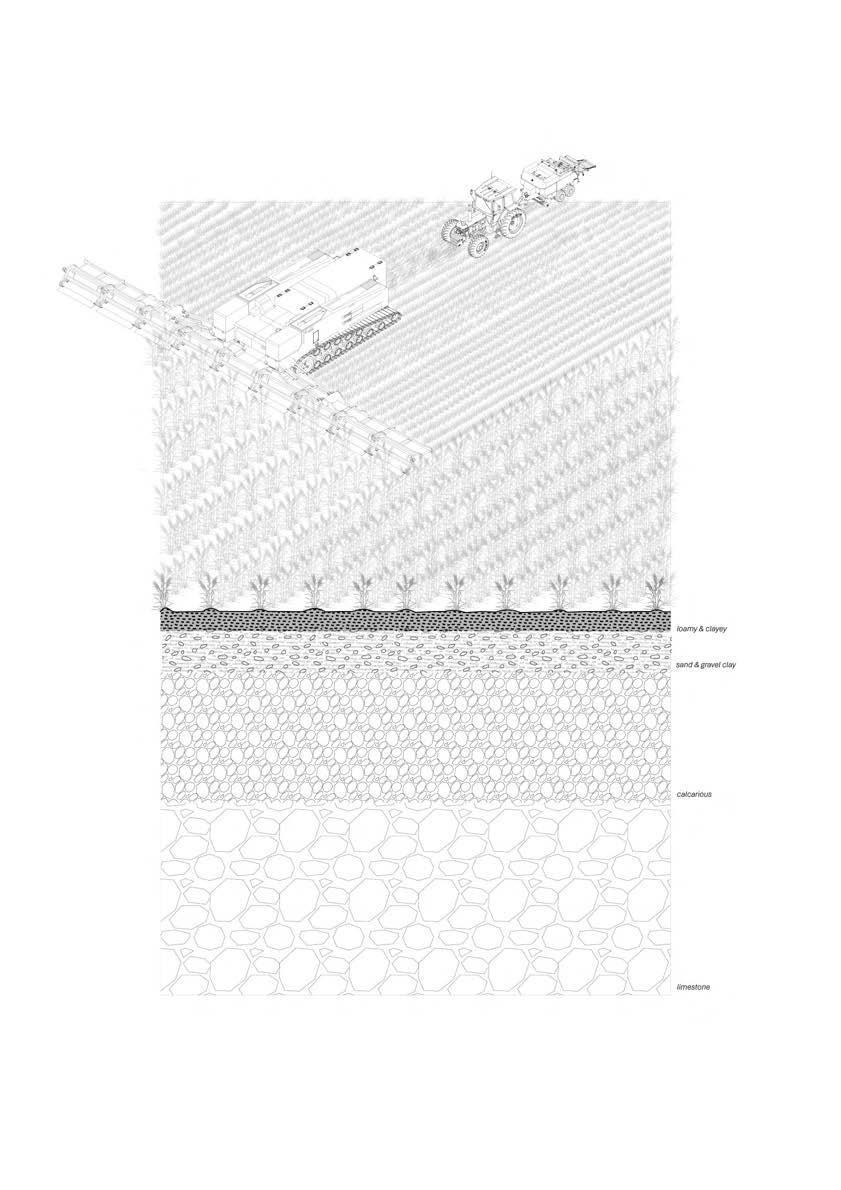
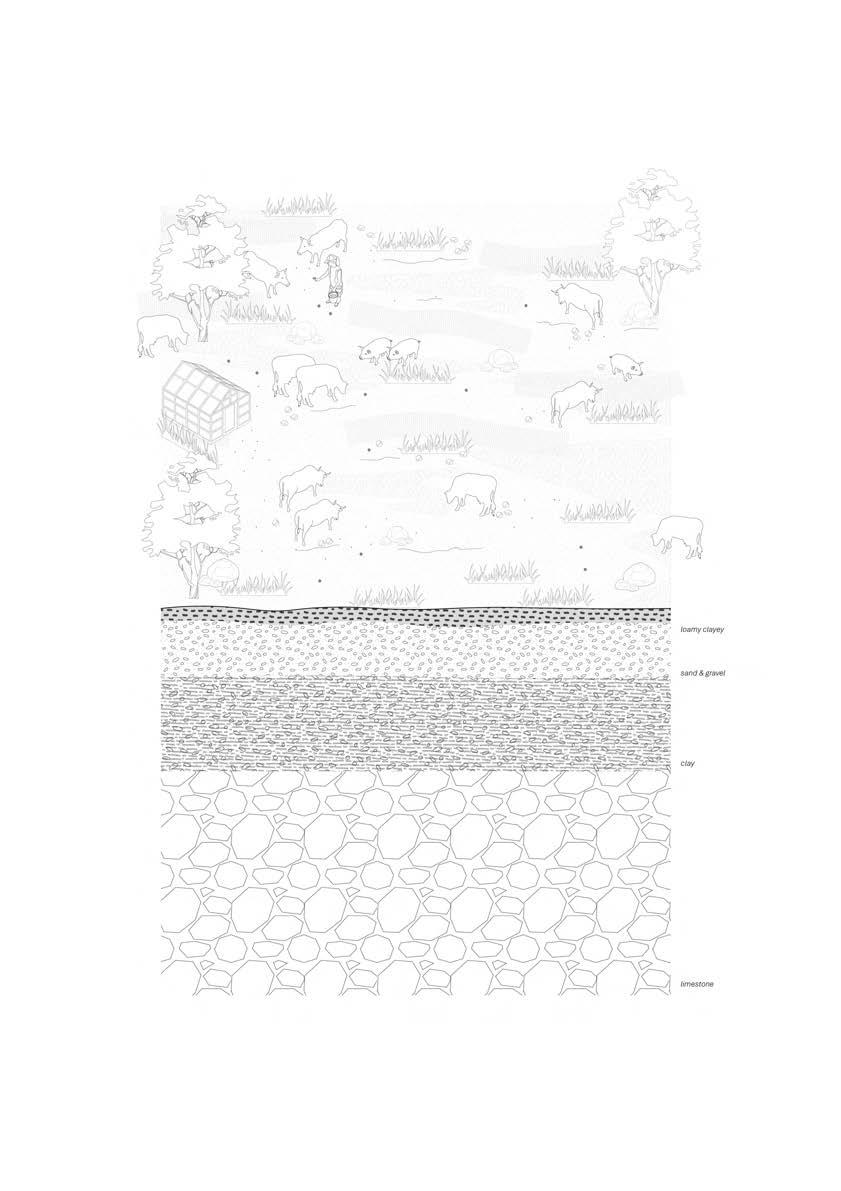
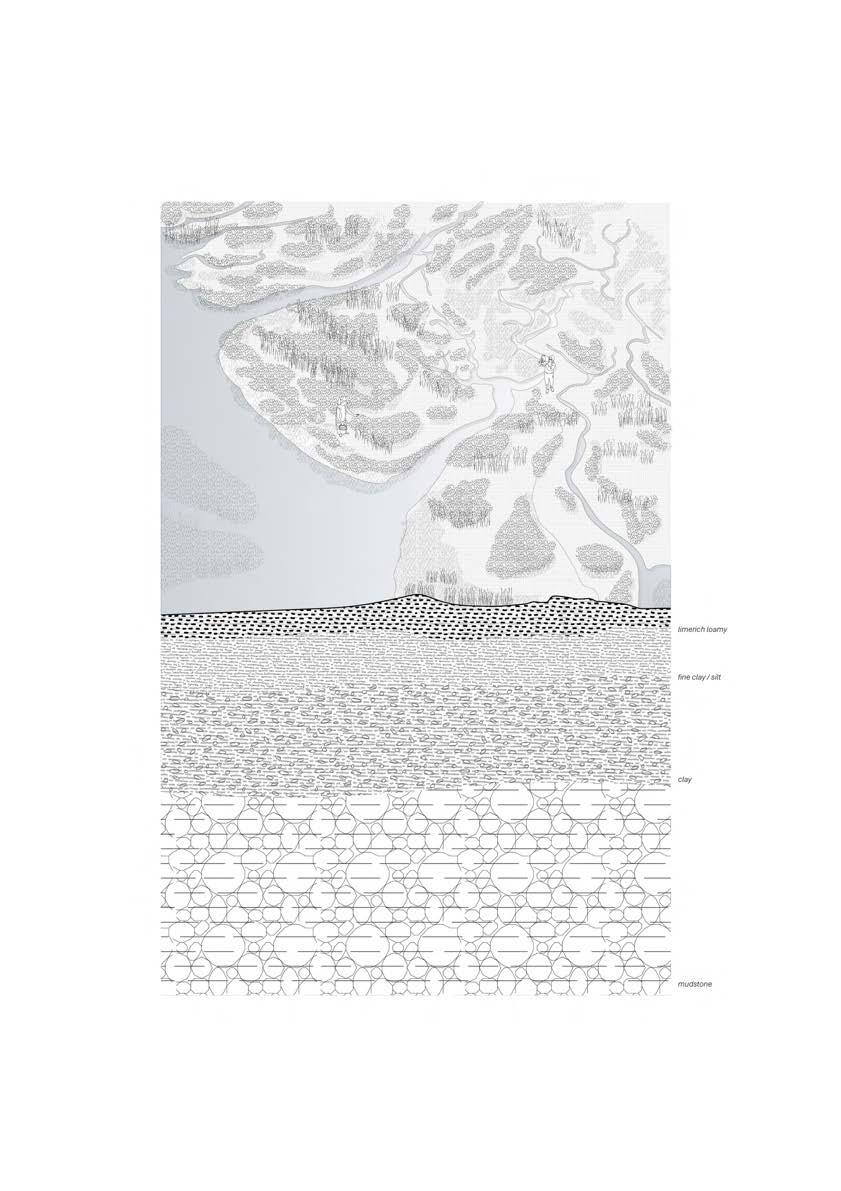
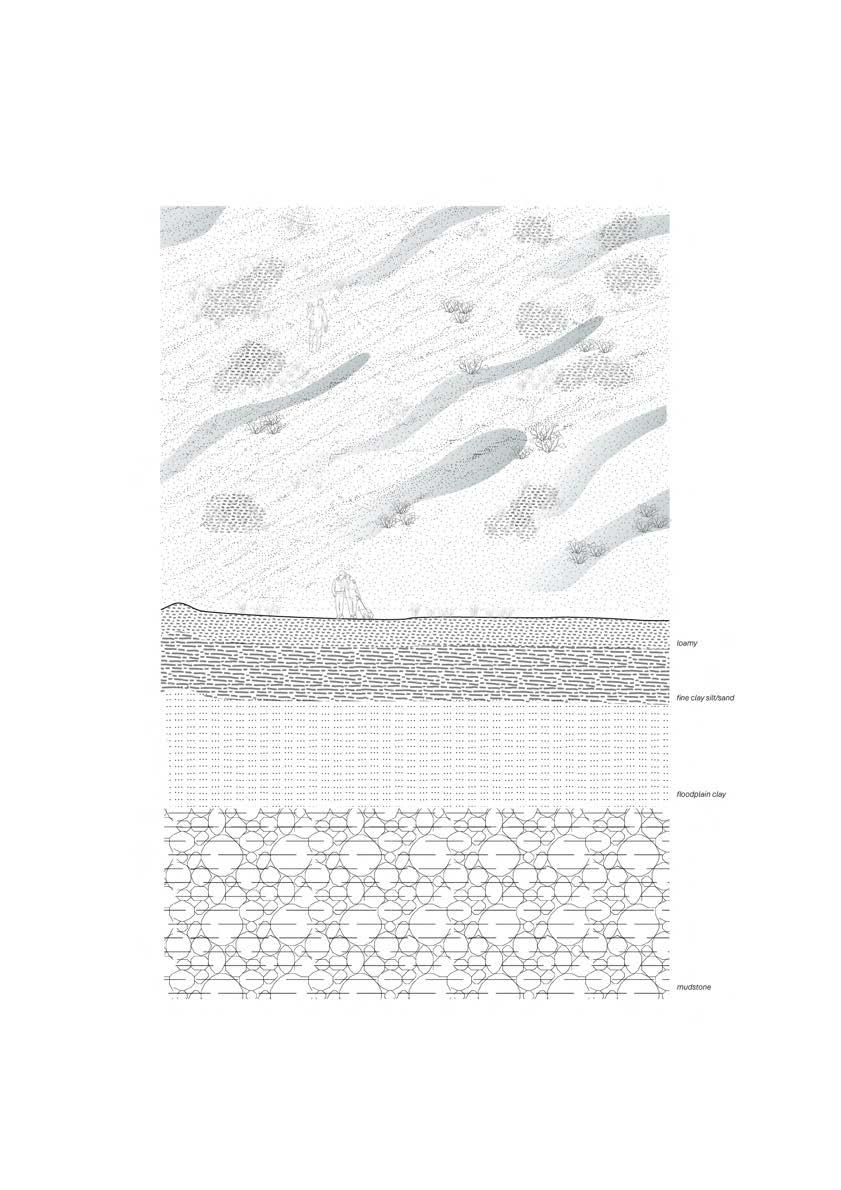
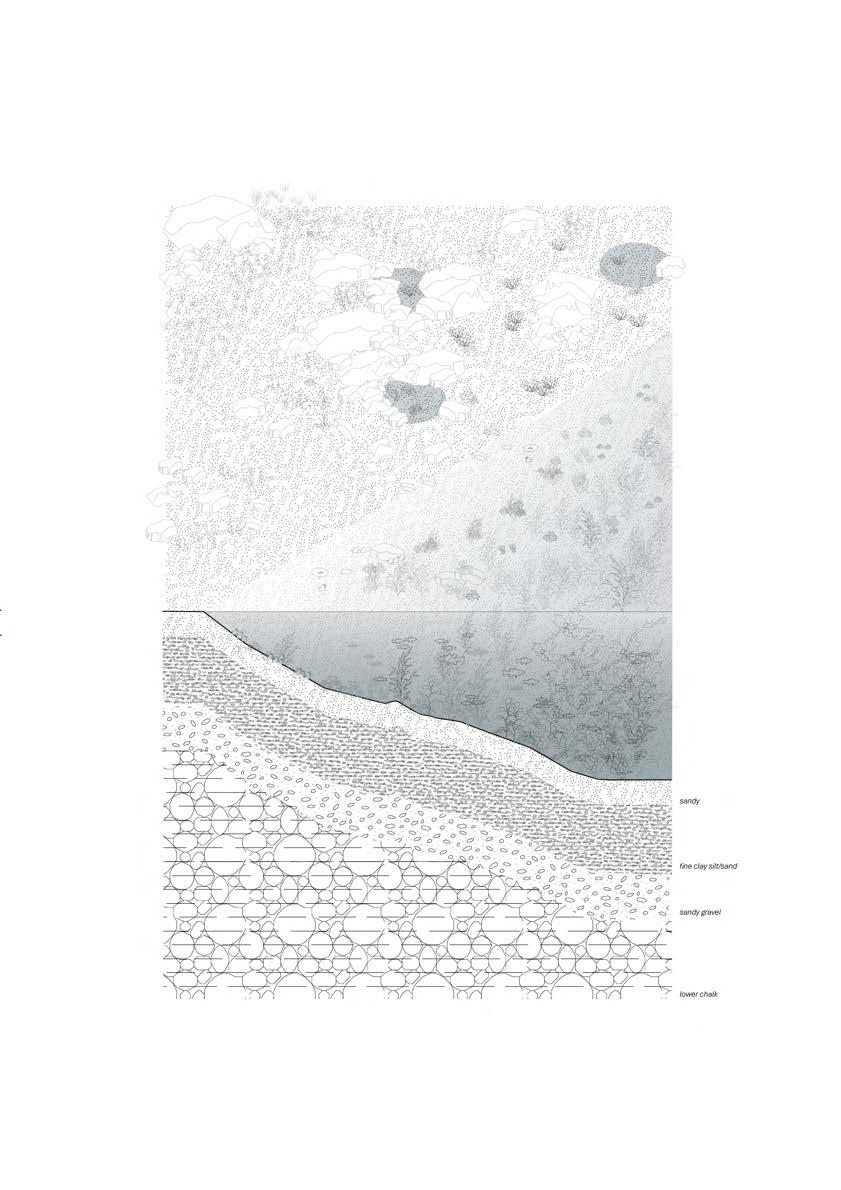
PROTOTYPING THE BGS AND LANDSCAPES OF PRODUCTION
Envisioning the BGS, straw farms, seaweed and seashell farms, and prototyping sites.
Prototyping the British Geological Survey
Retrofitting the BGS: Integrating biobased materials and enhancing the campus landscape
by Alejandra Iturrizaga Andrich
The British Geological Survey (BGS) campus presents an opportunity to address existing limitations and implement advanced sustainable solutions. The current 3D render illustrates the campus’s existing conditions as seen on the BGS visit, featuring smaller labs, inadequate insulation, and limited natural light, with the outdoor space largely undeveloped. This chapter focuses on the proposed retrofitting strategies designed to enhance both the building’s functionality and its interaction with the surrounding environment.
Our proposal aims to address these deficiencies through a comprehensive retrofit approach, incorporating biobased materials and design solutions. By focusing on external facade insulation and outdoor space, we align our strategy with principles established in previous chapters, such as sustainable land use and environmental impact reduction. The goal is to create a cohesive environment that supports both the building’s performance and its integration into the broader landscape by utilizing native and pollinator-friendly plants. We focused not only on the building itself but also on an integral retrofit of both outdoor and indoor areas.
The British Geological Survey (BGS) plays a key role in the PublicCommon Partnerships (PCP) model by integrating biobased materials like straw and shellfish renderings in its retrofit project. Collaborating with local councils and universities, BGS will foster research, provide workshops for upskilling workers, and create sustainable supply chains. As a demonstration site, BGS supports the PCP model’s goals of promoting biobased building practices, strengthening local economies, and advancing sustainable construction techniques throughout the region.
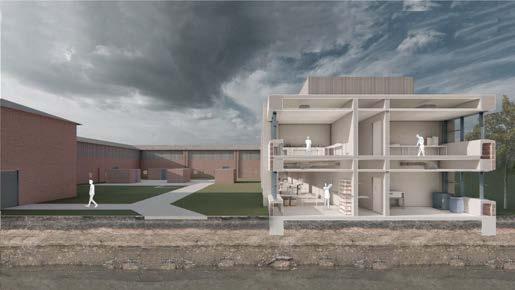
ill.27 BGS current status by Alejandra Iturrizaga Andrich
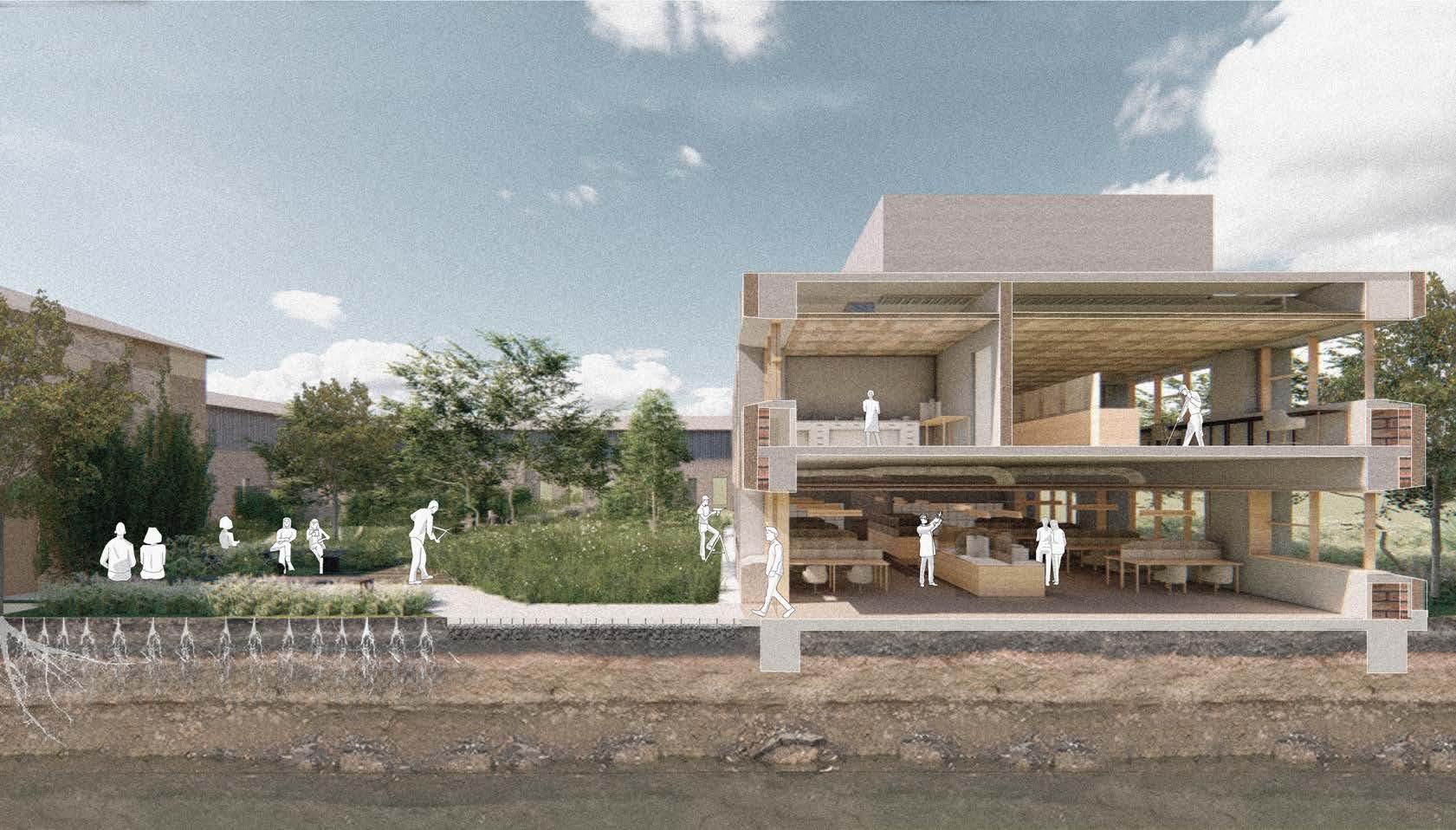
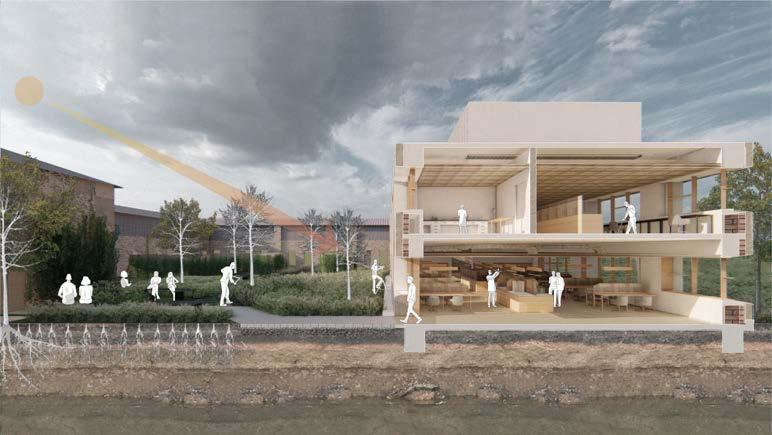
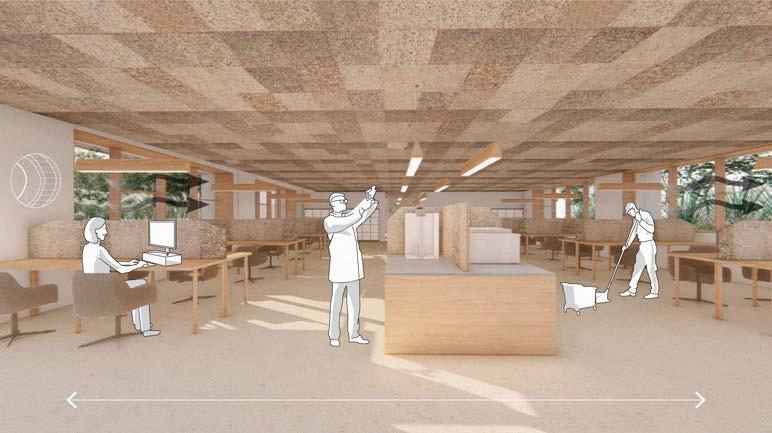
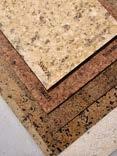
The 1:10 scale showcases a section of the building with the proposed straw panels and shellfish render applied. This physical model brings people closer to the proposal and the materials themselves, offering a tangible experience that deepens understanding and appreciation. The model has been crafted to facilitate dialogue between all stakeholders involved in the project, ensuring that everyone has a clear and concrete vision of the proposed solutions.
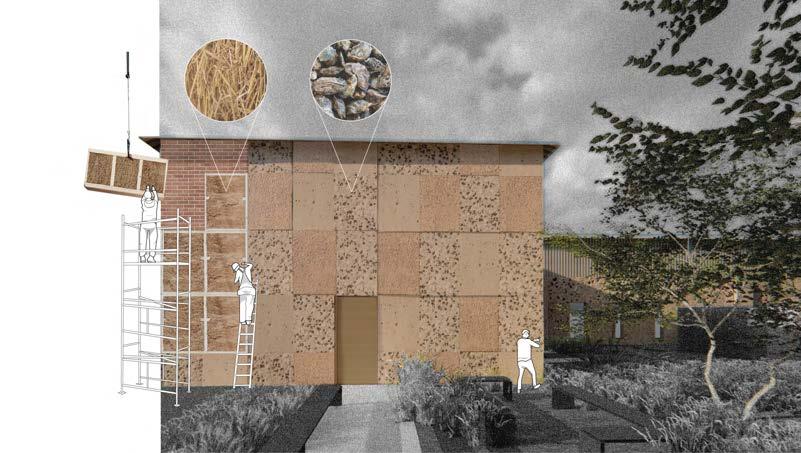
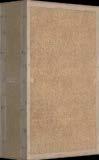


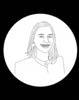
To address the insulation problem, we propose the use of straw panels on the facade. These modular panels, sourced from regenerative farms in the East Midlands region, offer an efficient and sustainable solution. The straw panels are designed for easy maintenance, allowing for individual panels to be mounted, demounted, and replaced as needed over the years. In developing this approach, we consulted with Ciaran Malik from Environmental and Technical Studies at the Architectural Association (AA) to gain a deeper understanding of biobased assemblies and construction methods. His insights helped to refine our approach to using straw insulation effectively.
For the facade rendering, we recommend a shellfish-based material, inspired by the innovative work of architects Katya Briskina and Nataly Nemkova. We had the opportunity to interview both Katya and Nataly where they provided us with a comprehensive understanding of their experimental work with shellfish in construction, which informed our decision to incorporate varying sizes of crushed shellfish across the facade.
This rendering approach not only makes it aesthetically pleasing but also follows a modular grid pattern that mirrors the internal structure of the wall, making it easier to identify and maintain. The variation in shellfish sizes across the panels provides a textured, dynamic appearance that enhances the building’s character while contributing to its environmental performance.
The BGS, known for being a government institution at the forefront of resource innovation in the UK, serves as an ideal experimental ground for these materials. Not only the immediate needs of the building can be adressed, but also there could be a contribution to the broader field of sustainable construction practices. In line with this, conducted my own shellfish rendering tests to explore the practical application of this innovative material.
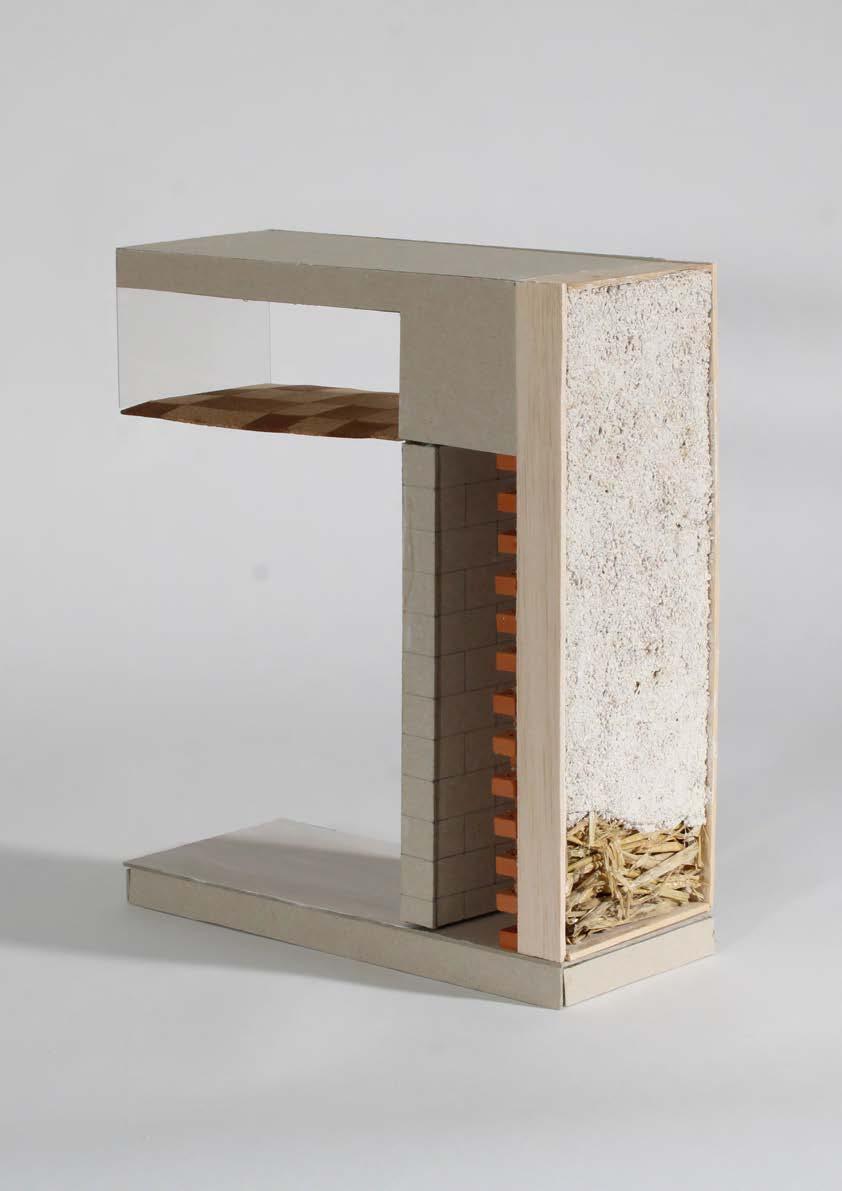
Straw panel / Constructed from straw bales encased in timber frames, these panels are renowned for their excellent acoustic and insulation properties. The natural materials not only enhance energy efficiency but also contribute to a sustainable building approach by using renewable resources.
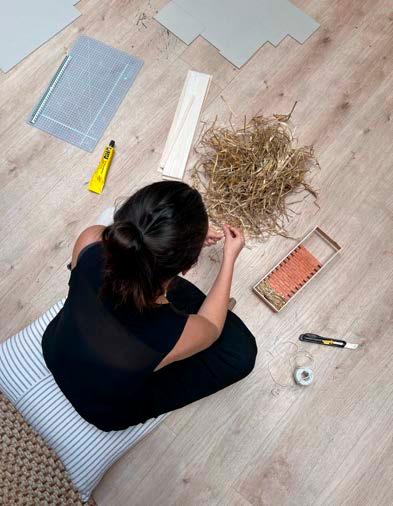
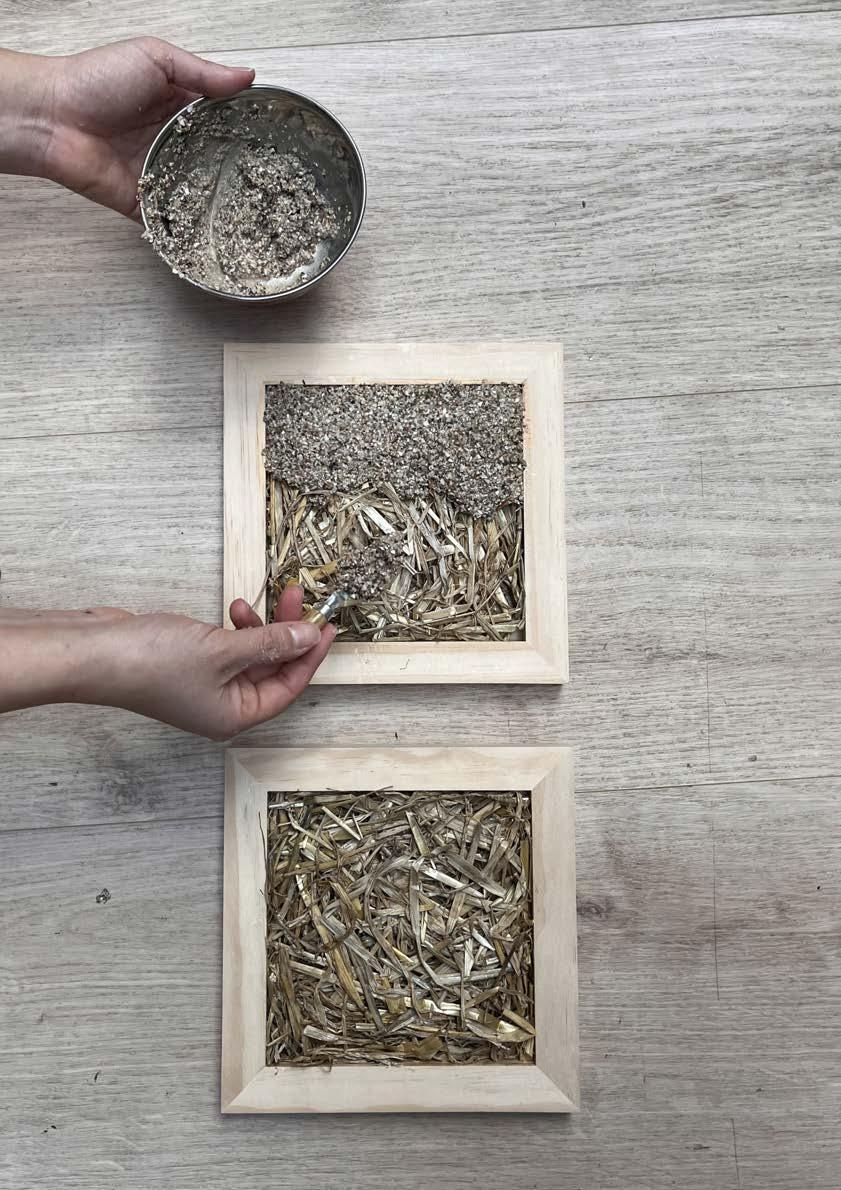
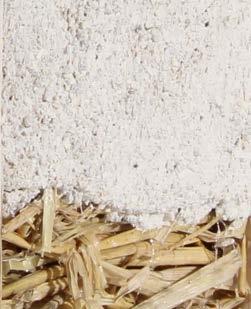
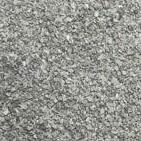
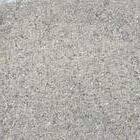
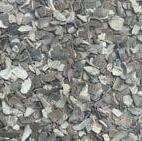
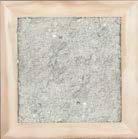
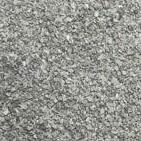
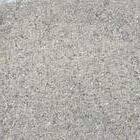
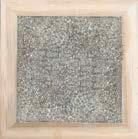
Shellfish rendering presents a promising alternative to traditional lime rendering. Particularly, in the case of BGS, seashells could come from the East Midlands coast. Traditional lime rendering typically uses a mix of lime and water, sometimes combined with sand. In this approach, I substituted lime with shellfish powder, experimenting with different types of crushed shellfish to determine which combination would give the best results.
The shellfish powder is crucial because it solidifies as it dries, providing the necessary structural integrity. The crushed shellfish, while primarily decorative, offers the opportunity to create various textures and patterns. However, they do not contribute significantly to the rendering’s strength. When using smaller crushed shellfish, it’s essential to ensure that a sufficient amount of shellfish powder is present to hold the shell fragments in place as the render dries.
Although the shellfish powder showed great potential, observed that the mixture might require an additive to improve its durability in rainy environments and is something that would need further testing. Ultimately, found that a mix of shellfish powder, small crushed shellfish, and lime provided the most durable rendering (fourth row on the right). In this case, lime is not entirely replaced, but its demand could be significantly
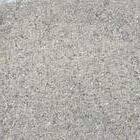
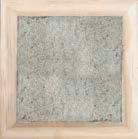
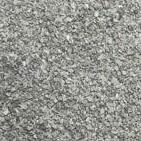
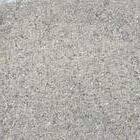
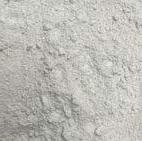
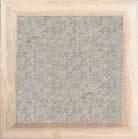
SMALL CRUSHED SEASHELLS SEASHELL POWDER RESULT
SMALL CRUSHED SEASHELLS SEASHELL POWDER BIG CRUSHED SEASHELLS RESULT
SEASHELL POWDER RESULT
SMALL CRUSHED SEASHELLS
SEASHELL POWDER
SOLID less more
fig.48 Shellfish and lime rendering on straw
Photo: by Alejandra Iturrizaga
Transforming traditional practices by integrating strategic grazing, soil regeneration, and ecological connectivity
by Alejandra Iturrizaga Andrich Straw Farming
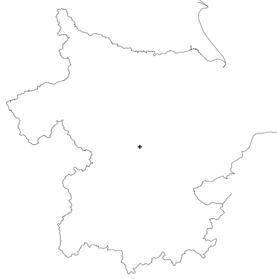
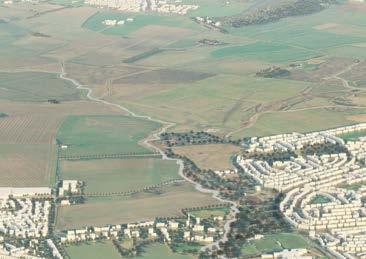
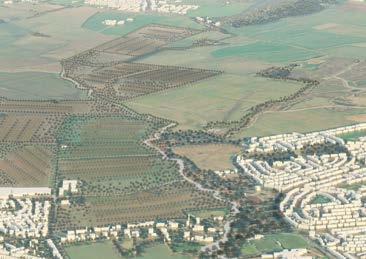
Many existing farms in the East Midlands are characterized by conventional monoculture cereal production. The area under consideration which is located on the outskirts of Clifton town in Nottingham, along Fairham Brook and approximately 7 km from the BGS, exemplifies this traditional approach. This landscape, marked by isolated ecological parcels, presents an opportunity to enhance ecological connectivity and resilience through a comprehensive agroecological farming prototype centered on straw. By establishing wildlife corridors, hedgerows, and buffer zones, the prototype would link these fragmented habitats, allowing for the movement of species and the flow of ecological processes across the landscape.
The Straw Farm Prototype envisions a transformation from conventional practices to a more sustainable and ecologically integrated farming system. A key part of this prototype is the use of strategic animal grazing. By adopting rotational grazing practices, the farm will improve soil health, control weeds, and enhance nutrient cycling. This method supports soil regeneration and integrates livestock into the farming system in a way that complements and sustains other practices.
Tree buffers will be planted around fields and along waterways to provide shade, reduce soil erosion, and create valuable wildlife habitats. These buffers will also help in controlling wind and water flow, further contributing to soil regeneration and overall ecosystem health. The farm will incorporate the collection and utilization of straw bales. These bales will serve multiple purposes, such as mulch for soil health improvement, construction material, or as part of a closed-loop system to enhance farm sustainability.
Additionally, the prototype includes a recreational trail that will appear along the farm, providing public access and educational opportunities. This trail will facilitate deeper engagement with the farming practices and biobased material production, promoting awareness and fostering community connection as seen on the agroecological farming section on the next page.
By integrating strategic grazing, tree buffers, perennial crops, and public engagement through recreational trails, the aim is to create a resilient and interconnected agricultural landscape. This comprehensive approach will contribute to a more sustainable and ecologically rich farming system.
The Straw Farm Prototype will be part of the Public-Common Partnerships (PCP) model, focused on producing constructiongrade straw while supporting local economic growth through job creation in sustainable agriculture. Collaborating with local councils and universities, the farm will serve as a hub for biobased material production. Universities will play a key role in providing research, workshops, and education to upskill workers and community members in biobased building techniques. Local councils will assist by offering policy support and grants, facilitating the adoption of agroecological practices across the region.
Through its collaboration with other farms in the PCP network, including seaweed farms that can provide organic fertilizers, the Straw Farm Prototype will foster an interconnected and regenerative local economy. This integrated system will strengthen regional resilience, enhancing both environmental sustainability and economic opportunities.
Perennial crops, including perennial wheat, will be central to restoring soil fertility efforts, offering long-term benefits by reducing soil disruption and promoting longer soil health. The integration of wildflower buffers along field margins and within corridors will further support pollinators, increase biodiversity, and enhance the aesthetic value of the landscape. These buffers also play a crucial role in soil health and water management.
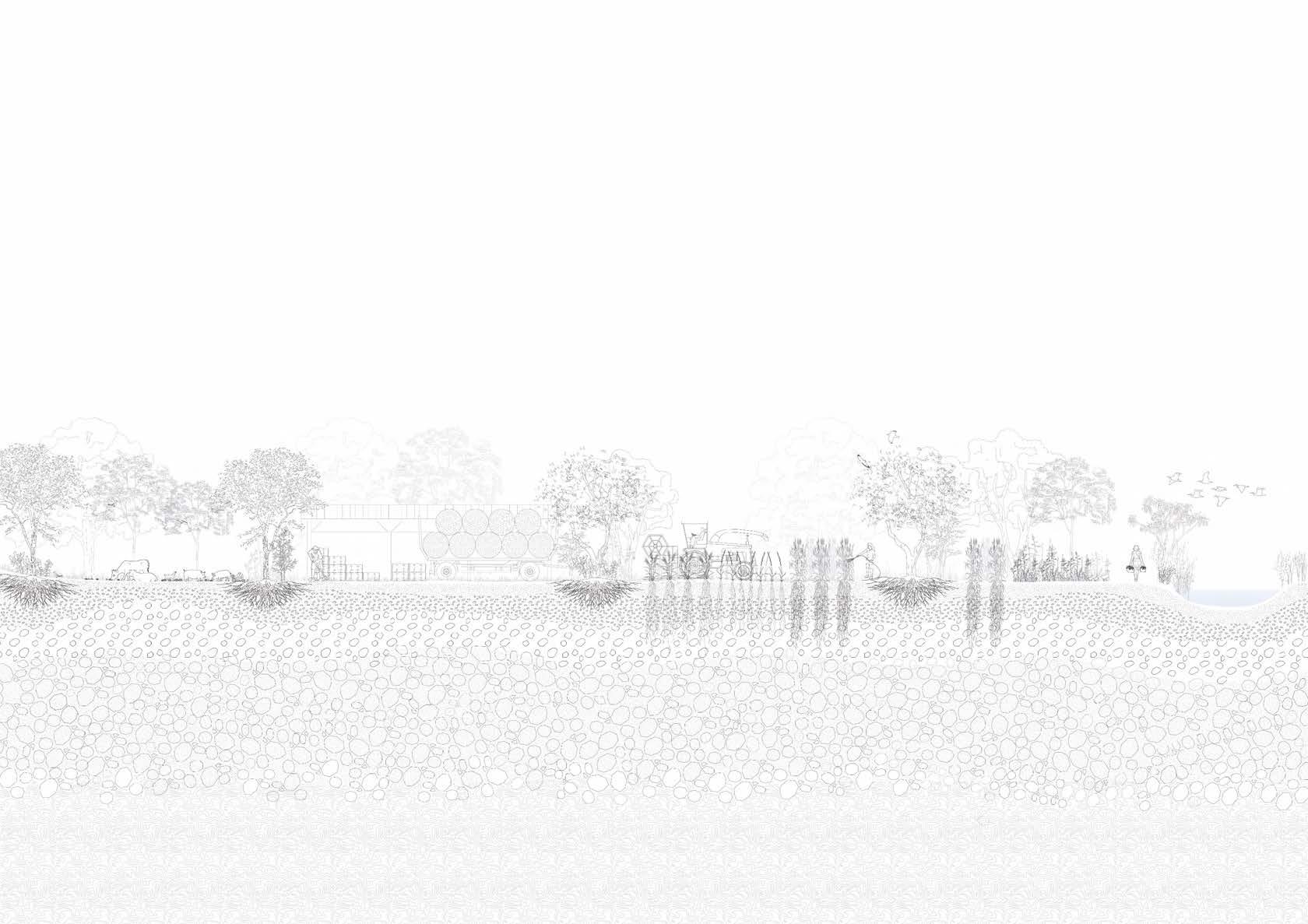
Perennial
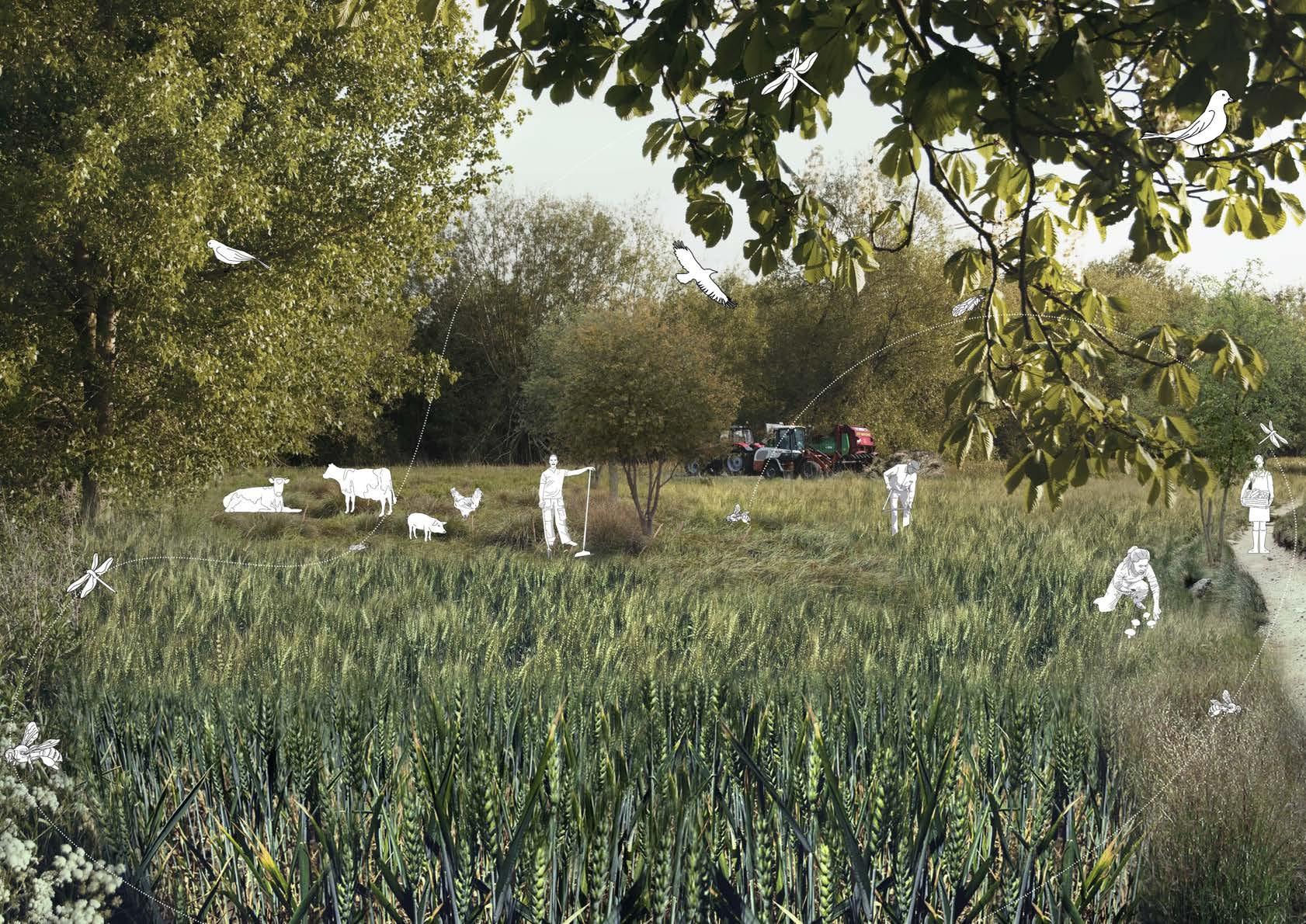
Prototyping site
Transforming underutilized spaces to testing and training for biobased construction and regenerative practices
by Alejandra Iturrizaga Andrich
The prototyping sites play a crucial role in the transformation towards more sustainable and regenerative practices. These sites are envisioned as spaces where empty public lands, brownfields or abandoned structures are repurposed for a range of testing and educational activities. By adapting these areas, we can explore and refine innovative approaches in both construction and agriculture.
At these sites, we will test various straw panel assemblies to evaluate their effectiveness and durability in real-world conditions. This practical testing is essential for optimizing the design and performance of straw-based building materials. Additionally, soil sampling will be conducted to assess and monitor soil health, ensuring that regenerative practices are having the desired impact on soil fertility and ecosystem health.
The sites will also serve as laboratories for growing straw and experimenting with different cultivation techniques. This allows
us to explore the best methods for producing and using straw in a variety of applications. Furthermore, these prototyping sites will host educational events aimed at training and upskilling workers and farmers. By engaging in hands-on activities and learning about biobased construction and farming methods, participants can better implement these practices in their own work.
Ultimately, the goal is to enable upskilled workers and farmers to adopt biobased construction and regenerative farming practices across the East Midlands. Through these prototyping sites, we aim to demonstrate the viability of these approaches, support the transition to more sustainable practices, and foster a broader adoption of regenerative methods in the region.
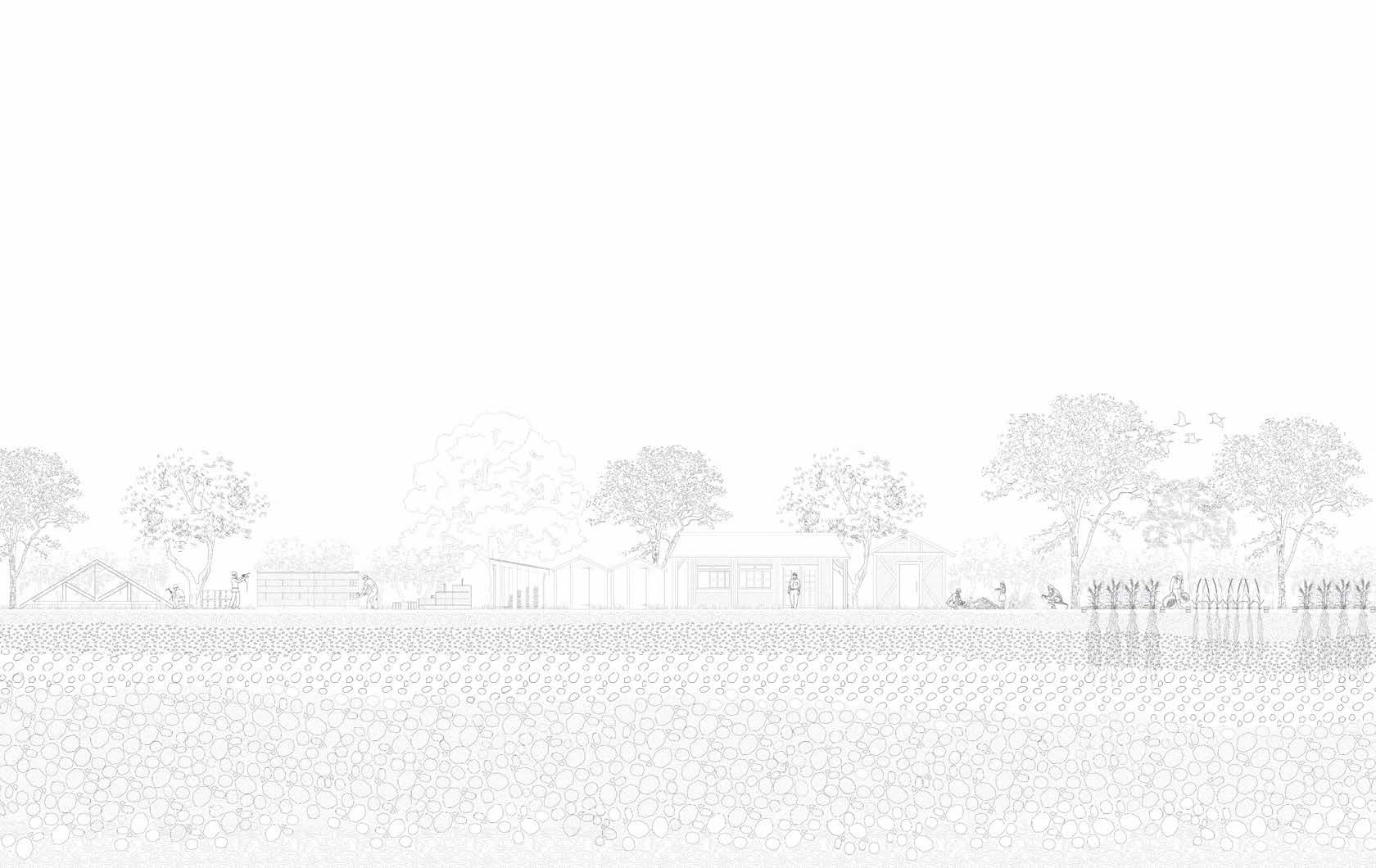
Repurposing old structures
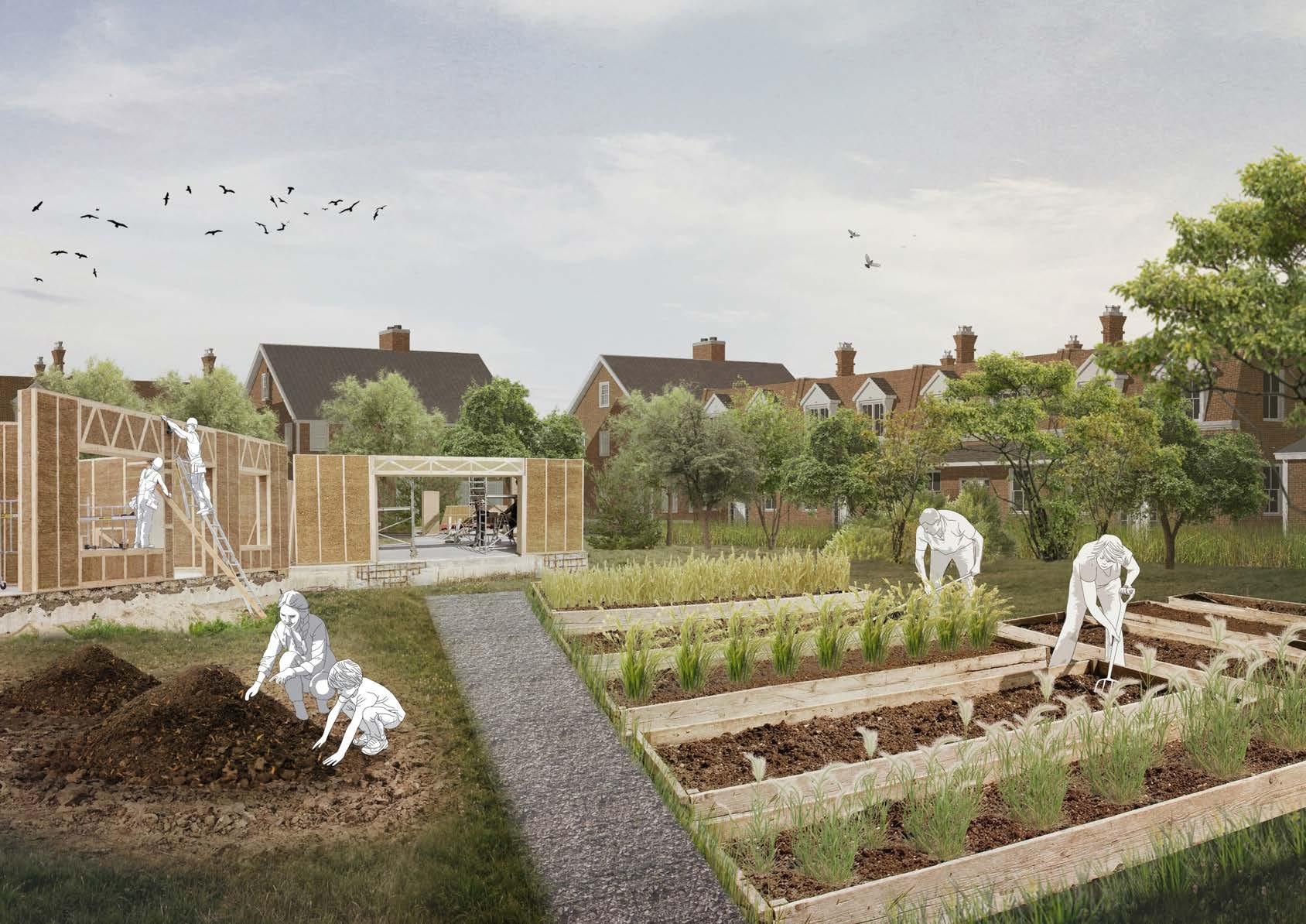
by Alejandra Iturrizaga Andrich Seaweed
Farming
Sustainable Farming and Marine Rewilding in Skegness
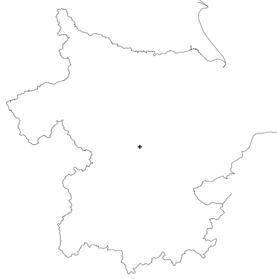

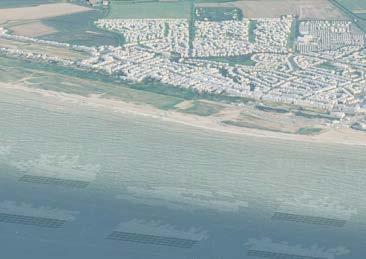
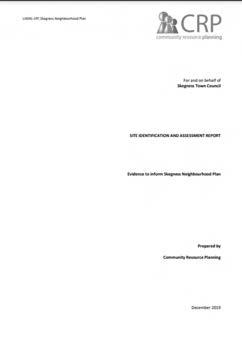
The Seaweed and Shellfish Farm Prototype envisions a transformative approach to coastal and marine resource management, particularly for communities like Skegness. This coastal area depicted, faces challenges such as flooding and economic dependency on tourism. Recent plans and news highlight a growing interest in diversifying into alternative industries, with seaweed farming emerging as a promising opportunity.
Seaweed farms could be established along the Skegness coast, integrating farming, recreational activities, and marine rewilding. These farms, marked by black hatches on the visualizations to the left, are designed not only to provide economic benefits but also to contribute to environmental restoration. The white irregular patterns indicate areas where habitat rewilding would evolve alongside the farms, enhancing ecological health and biodiversity.
Kelp and seagrass rewilding efforts aim to restore seabed biodiversity that has been degraded by trawling and eutrophication. Ensuring that the seabed composition and water clarity are suitable for restoration is crucial to the success of these initiatives. To address issues related to light penetration, some rewilding efforts might need to be situated in nearby bay areas where the water is shallower and light penetration is more favorable.
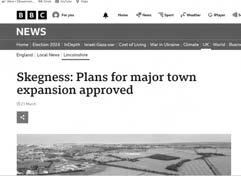
In addition to seaweed farming, we propose integrating multitrophic aquaculture systems, which involve cultivating kelp, mussels, and oysters together. These systems are beneficial for diversifying production, filtering pollutants from the water, and reducing the spread of diseases among marine species. Integrated multi-trophic aquaculture can significantly enhance the overall health and productivity of marine environments.
Key components of this prototype include drying facilities for seaweed processing, conservation efforts for nearby dunes, and the development of a recreational trail similar to those in the straw farm prototype. Wharfs would facilitate the handling and transportation of seaweed and shellfish. This prototype will actively collaborate with the Public-Common
BGS
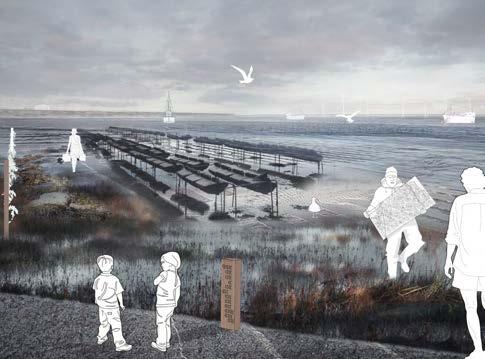
Onshore activities would encompass drying facilities, recreational trails, and small ports dedicated to monitoring and maintenance operations. Additionally, there is potential to upskill existing shellfish farmers to transition into kelp farming, leveraging their current expertise and infrastructure to support this emerging industry.
Integrating recreation and educational opportunities is a top priority to enhance community buy-in. The image on the right depicts recreational floats situated near kelp farms and diverse habitats. These floats serve multiple purposes, including community upskilling, field research, and monitoring areas. They also provide spaces for community recreation and eco-tourism, all within proximity to the sustainable aquaculture site such as the seaweed farm. Additionally, these areas could be designed to support migratory bird habitats, further enriching the local ecosystem.
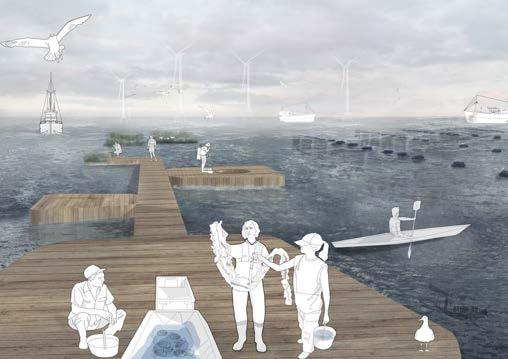
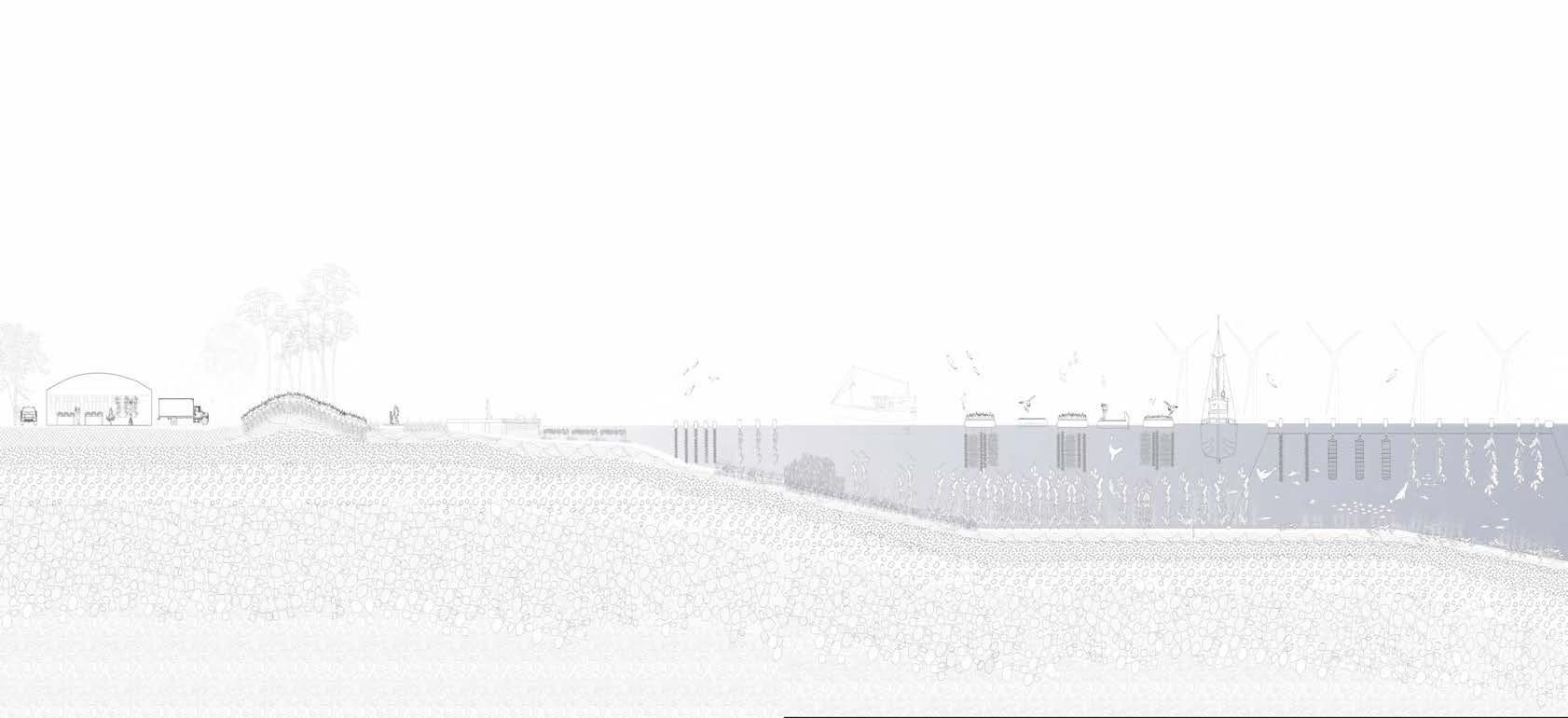
Drying facility Dune Trail Wharf Oyster farm Mussel farm Kelp prototyping ill.38
Recreation floats Kelp Farm Integrated multi-trophic aquaculture
Seabed rewilding
Oyster reef
REGIONAL NETWORKS AND LANDSCAPES OF PRODUCTION
Connecting Landscapes and Enhancing Biobased Production
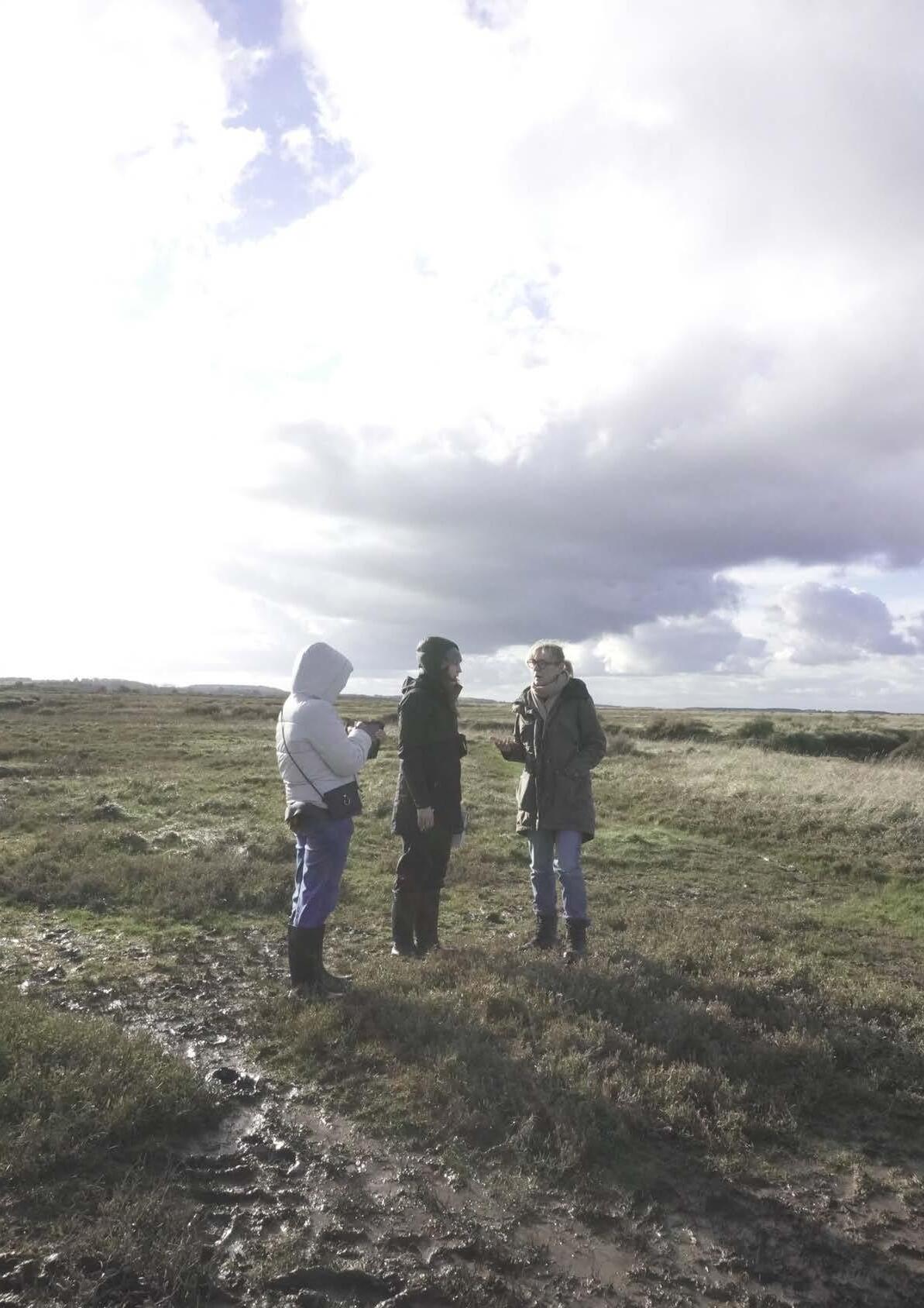
Uptake of Biobased Materials
by Alejandra Iturrizaga Andrich
Uptake of biobased materials
The first phase focuses on leveraging community allotments, government-owned lands, and brownfields situated near areas with high unemployment and unskilled labor. These locations would serve as sites for prototyping construction assemblies, testing agricultural methods, and providing opportunities for workforce upskilling. In parallel, existing shellfish farms, represented by the blue crosses, could serve as pilot sites for seaweed-based material prototyping.
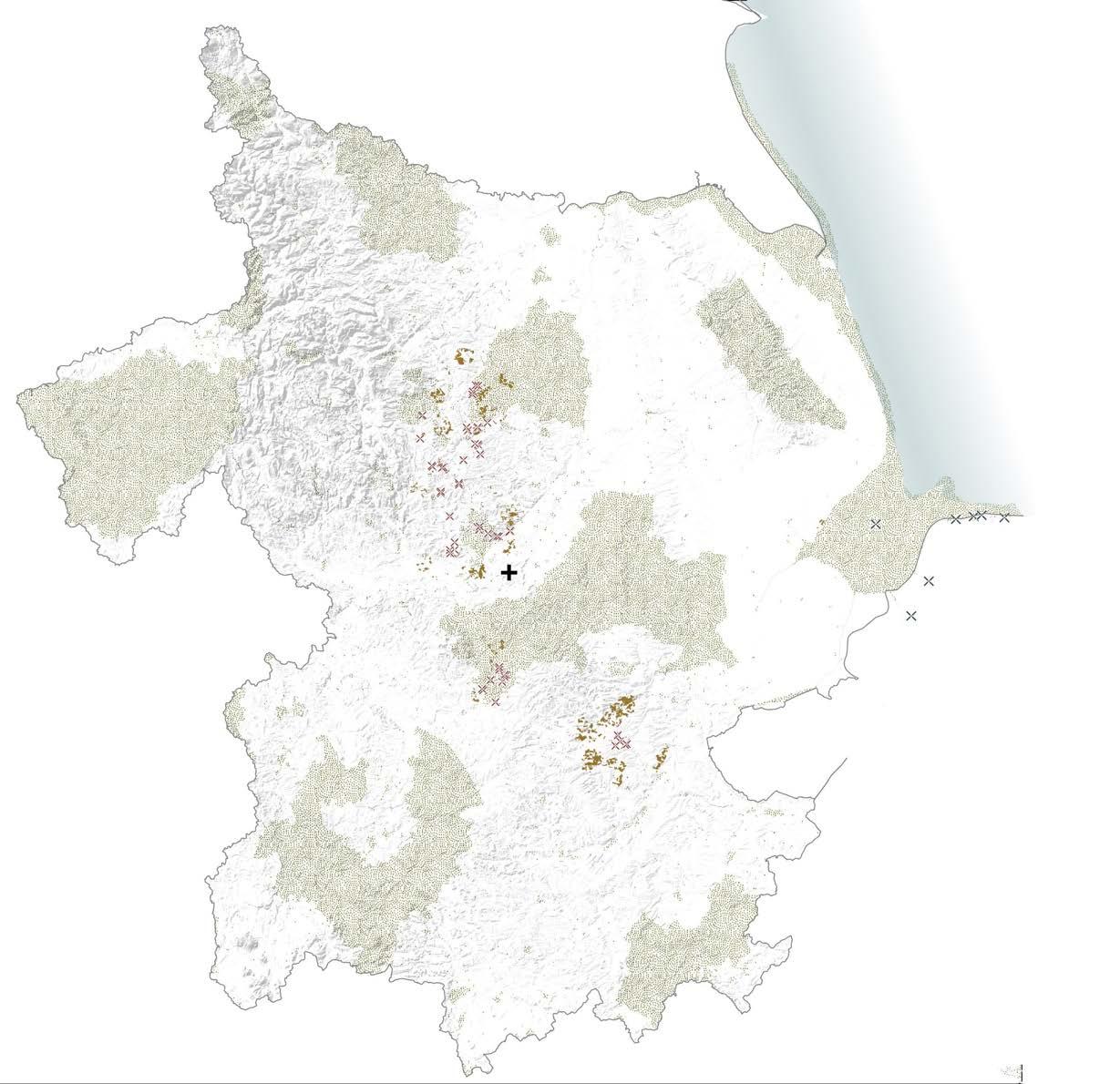
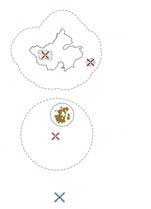
by Alejandra Iturrizaga Andrich
Phase 2
Uptake of biobased materials
by Alejandra Iturrizaga Andrich
In Phase 2, as production of construction-grade straw increases, the network can broaden its reach to include a larger amount of under-skilled workers across the region. Additionally, seaweed farms can either expand along the coast or potentially integrate with new offshore wind farms, creating synergies between these two emerging industries. The preliminary selection for siting seaweed farms considers factors such as bathymetry, wave intensity, turbidity, fishing vessel traffic, proximity to ports, and Marine Protected Areas. This siting strategy is informed by reports from CEFAS, Hethel Innovation, and Norfolk Seaweed, which detail the placement of seaweed farms in the East Anglia region. As straw farms develop inland and potential seaweed farms are established, a noticeable degree of fragmentation within the network matrix is anticipated.
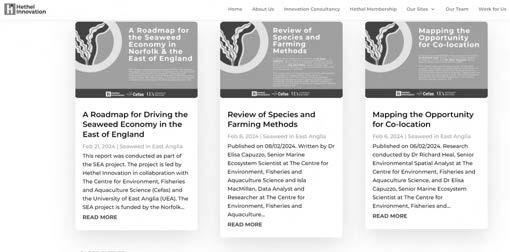
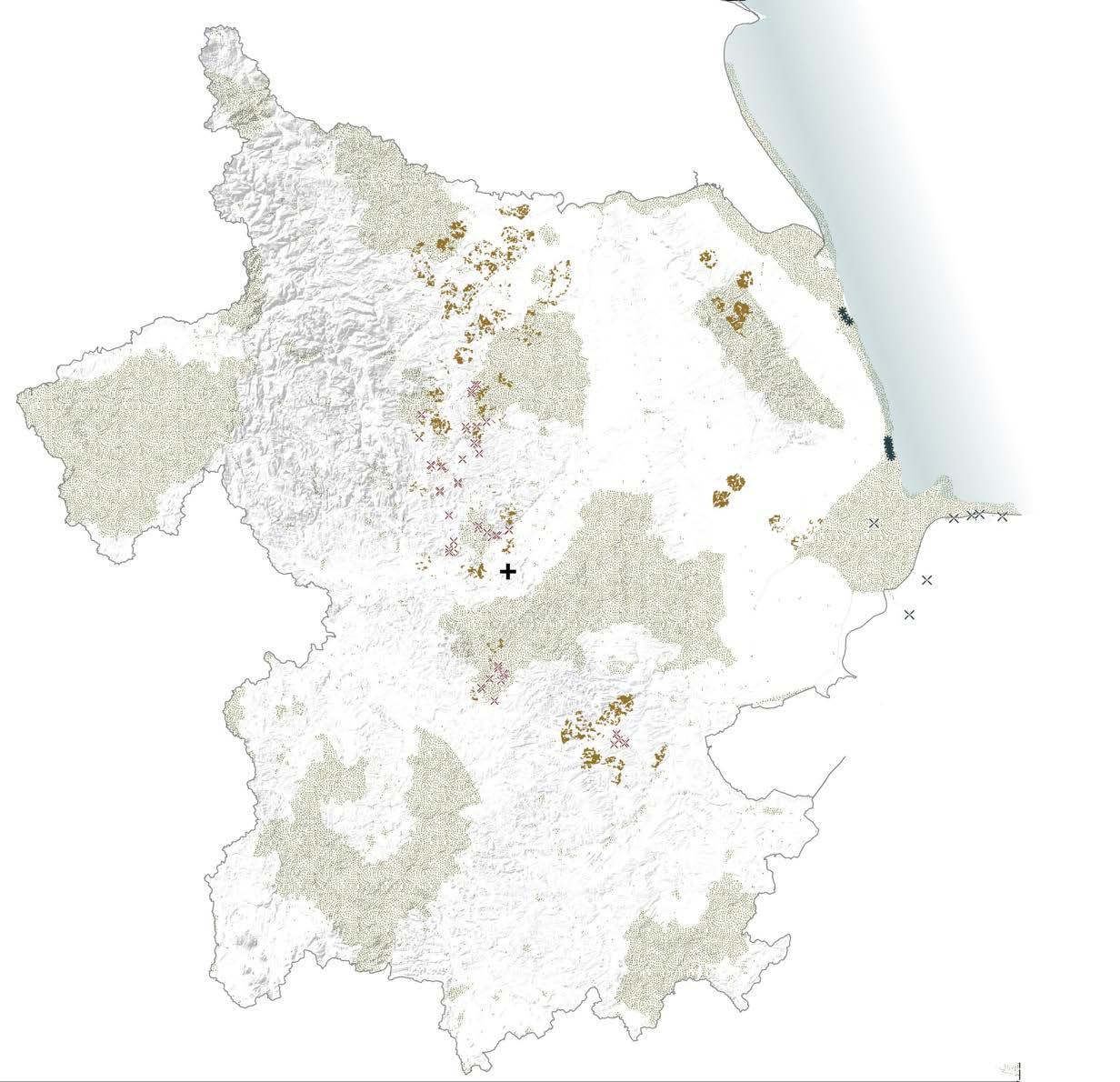
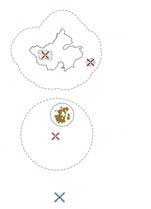

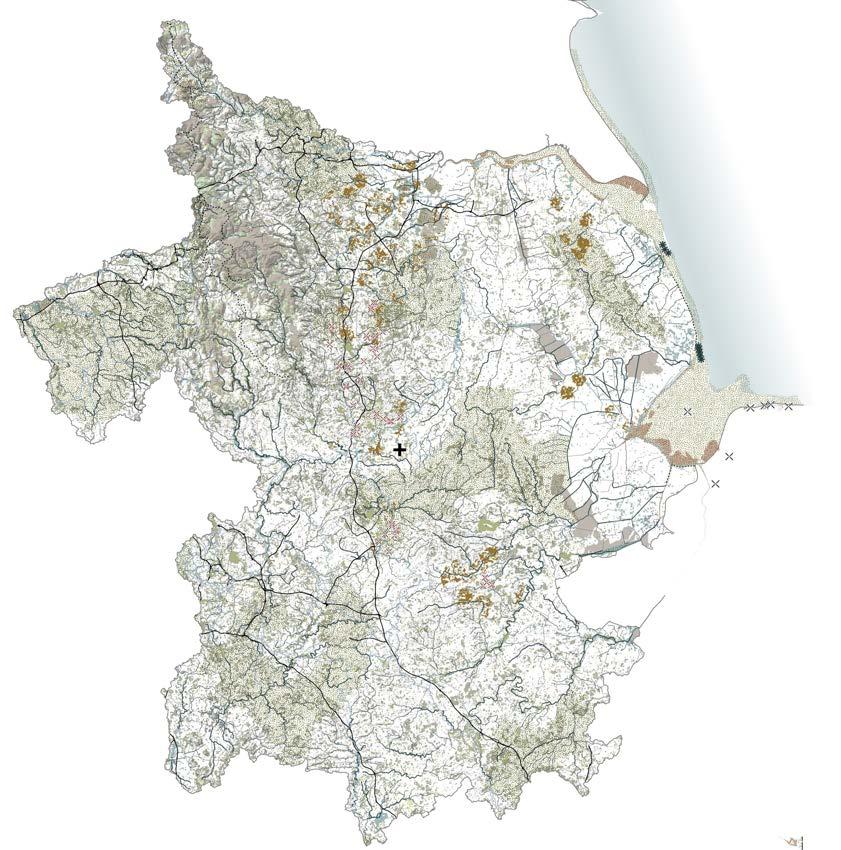





Patches and corridors in the East Midlands Matrix
Integrating Biodiversity and Connectivity in Biobased Production Landscapes
by Alejandra Iturrizaga Andrich
As the network of biobased materials production expands across the East Midlands, the landscapes dedicated to this production can play a pivotal role in enhancing regional biodiversity. When biodiversity enhancement measures are thoughtfully implemented at individual farms or across multiple farms, these productive landscapes can function as critical ecological patches. Practices such as planting wildflower buffers, establishing tree lines, and integrating rotational grazing or agroforestry systems contribute to creating habitats that support a diverse range of species. Over time, these measures help to regenerate soil health, promote pollination, and foster a balanced ecosystem within the agricultural landscape, making these areas not just zones of production but also ecological focal points.
In addition to the on-site biodiversity initiatives, it is crucial to consider the broader landscape connectivity facilitated by existing recreational networks. Greenways and greenspaces,
already established within urban and suburban contexts, can be leveraged as ecological corridors, linking isolated patches of biodiversity across the region. These corridors enable the movement of wildlife, support plant dispersal, and allow for the flow of essential ecological processes between different sites. Canals and waterways further enhance this connectivity by serving as natural conduits that link various habitats, providing both ecological benefits and opportunities for community engagement through recreation and education.
Moreover, traditional agricultural features like hedgerows and windbreaks, which historically served to protect crops and manage soil erosion, can be revitalized to act as linear connectors between farms. These features not only improve landscape connectivity but also contribute to the visual and ecological richness of the region.
Strengthening
Bridging
by Alejandra Iturrizaga Andrich
Trails and roads, if designed with ecological considerations in mind, can similarly function as connectors, linking productive landscapes and recreational areas while ensuring minimal disruption to wildlife.
By integrating these biodiversity patches with a network of ecological corridors, the East Midlands can create a mosaic of interconnected landscapes that support both agricultural productivity and environmental health. This approach not only strengthens the resilience of local ecosystems but also enhances the quality of life for communities by providing accessible green spaces, recreational opportunities, and educational experiences that foster a deeper connection to the land. As the region transitions to a biobased economy, these interconnected landscapes will be essential in balancing the needs of production with the imperative of ecological stewardship.
The concept of a “corridor of production” begins to take shape as we integrate the various elements of biobased material production across the East Midlands. This corridor visualized on the map, represents a continuous link between inland agricultural zones and coastal seaweed farms, effectively connecting land-based and marine environments. By strategically aligning these production sites, we not only enhance the flow of resources and knowledge across the region but also create a network that supports
between different landscapes, ensuring that the benefits of biobased materials are maximized, from the
of
to the
Land and Sea through a Unified Production Network
In Phase 3, as demand for biobased materials rises, there is potential to further transition the workforce from extractive industries to biobased regenerative farming. Closed quarry sites could be repurposed for additional prototyping, while agroecological farming practices may expand throughout the region. This shift would not only enhance the availability of biobased materials but also contribute to a more sustainable and diversified local economy.
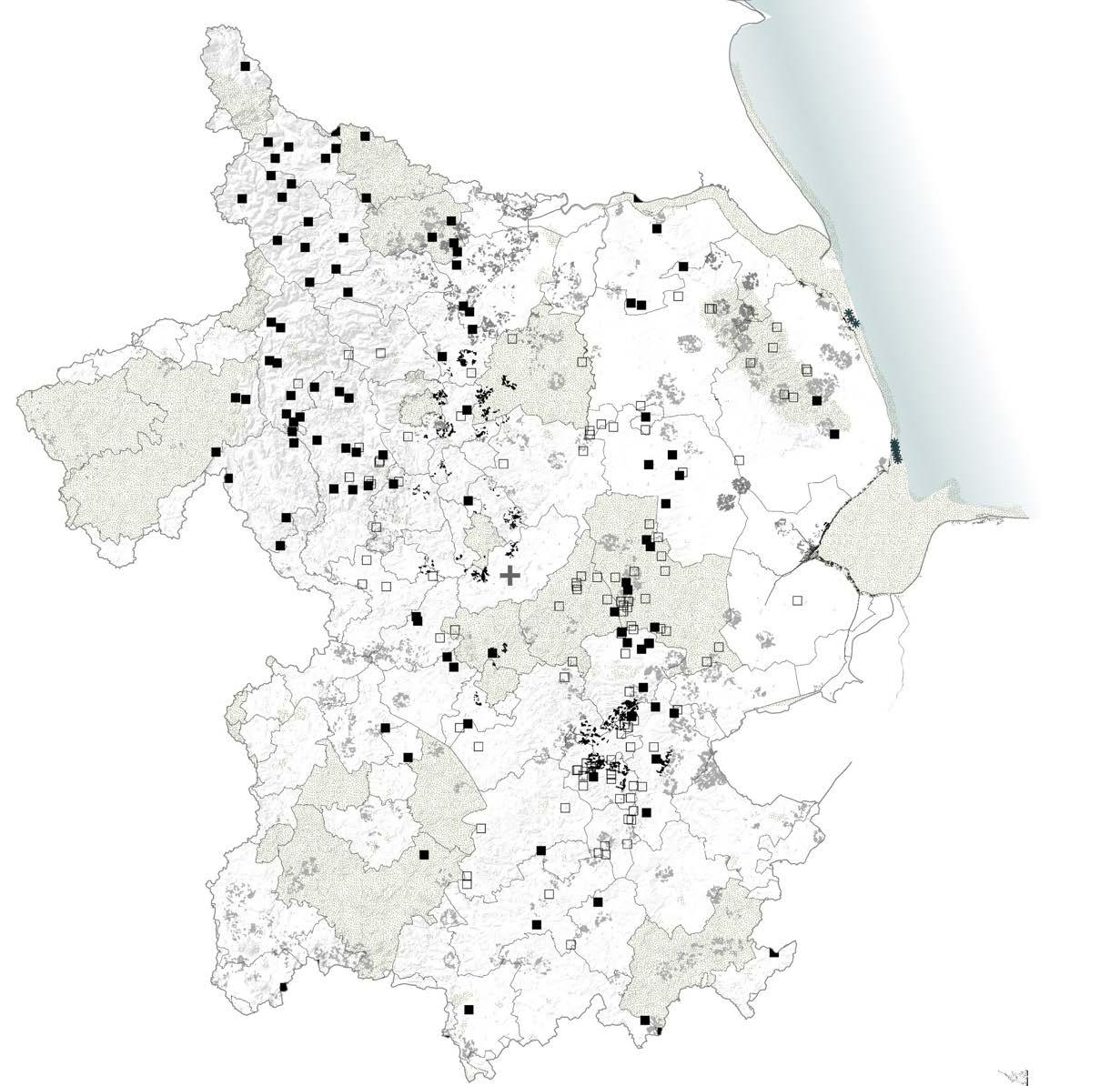



by Alejandra Iturrizaga Andrich
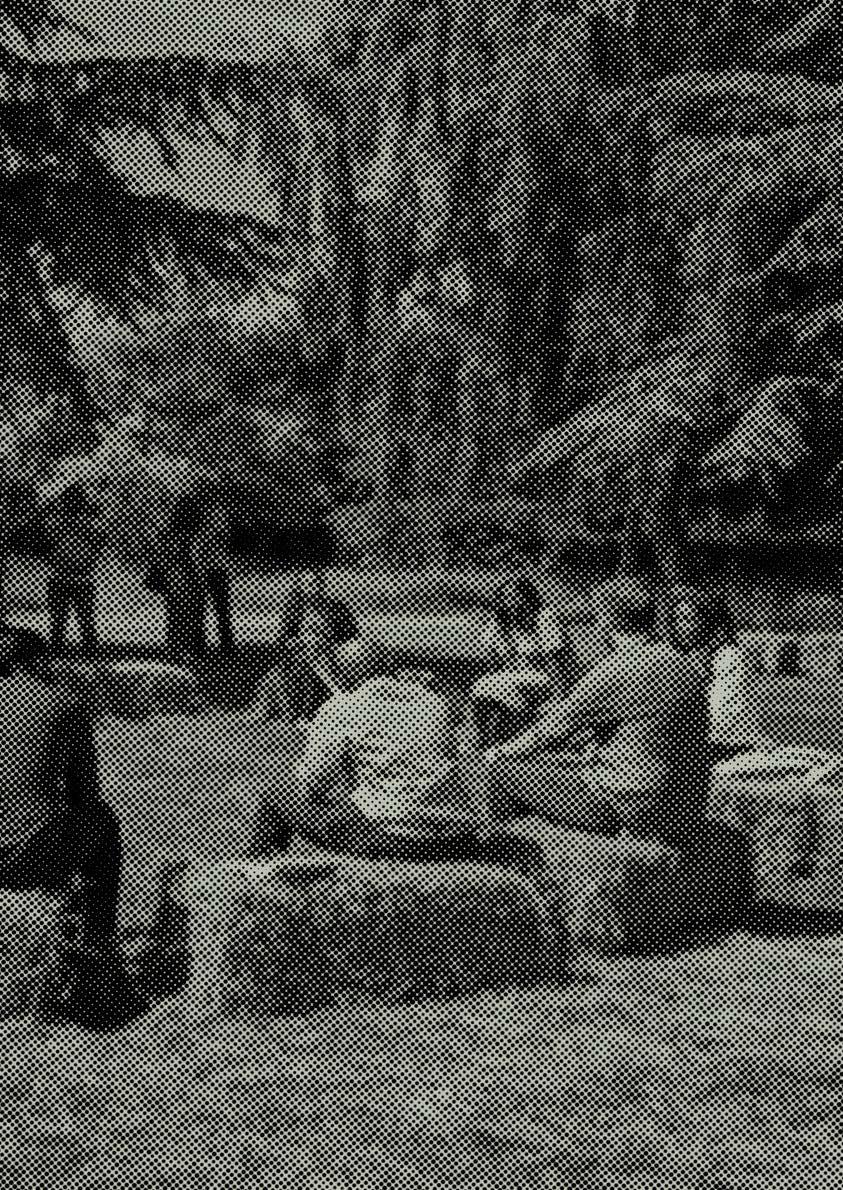
Summary
Designers play a pivotal role in advancing the use of biobased materials, shaping both the built environment and the natural landscapes that surround it. This thesis has demonstrated how thoughtful integration of biobased materials such as straw for insulation, seaweed for acoustic paneling, and shellfish-based renderings can transform construction practices and contribute to regional sustainability. Through detailed analysis, prototyping, and testing, we have explored how these materials can be effectively utilized in the BGS retrofit and broader regional applications in the East Midlands.
The construction industry faces an urgent need to reduce embodied carbon emissions, which account for a significant portion of a building’s total carbon footprint. As the UK works toward its Net Zero 2050 target, it’s essential to consider not only operational emissions but also the environmental impact of material extraction, processing, and disposal. Traditional building materials like cement and stone contribute to resource depletion and waste, while globalized supply chains add further complexities, including social and environmental issues abroad.
To address these challenges, the industry must prioritize the use of regenerative and low-carbon materials, and sustainable waste management practices. This holistic approach will be crucial in reducing the sector’s overall environmental impact and fostering more resilient communities.
The relationship between extraction landscapes and architectural design calls to an urgent need for a more integrated approach to material selection and environmental responsibility. Contemporary construction must evolve towards a post-waste economy where materials are designed for reuse, recycling, or composting. Biobased materials represent a key aspect of this transition, offering benefits such as carbon sequestration and soil fertility restoration, and aligning with both environmental and human needs. France’s proactive stance on bioconstruction, supported by regulatory frameworks and incentives, provides a valuable model for the UK, demonstrating how international collaboration can advance sustainable building practices. The proposed biobased materials viability framework is essential for navigating the complexities of material selection, including logistical, performance, and environmental factors. By adopting biobased materials and drawing lessons from successful international practices, the UK can improve its construction industry and contribute to a more sustainable future.
In reflecting on the just transition from extractive to biobased materials within the UK’s construction sector, it’s clear that this shift is not merely an environmental necessity but also a profound socio-economic transformation. Aligning with the principles of the 2015 Paris Agreement and subsequent COP24 declarations, the transition must holistically address the intertwined environmental, social, and economic challenges. Environmentally, this move towards biobased materials significantly reduces carbon emissions and environmental degradation, aligning with global sustainability targets. Socially, it emphasizes equity by fostering new opportunities for workers displaced by the decline of extractive industries, ensuring that vulnerable communities are not left behind. Economically, targeted investments in biobased materials can stimulate innovation, drive long-term growth, and create jobs, particularly in regions with high unemployment and the ones who would be most affected by the shift. This strategic multifaceted approach is essential for achieving climate goals.
The visit to the British Geological Survey (BGS) campus in Keyworth emphasized the critical need for retrofitting to meet contemporary standards of sustainability and functionality. The campus’s mix of older, historically significant buildings alongside newer structures presents both challenges and opportunities. The laboratories, which are pivotal to the BGS’s research mission, suffer from inadequate acoustics and thermal
conditions, directly impacting the efficiency and comfort of their workers. Addressing these issues is crucial for maintaining the BGS’s role as a leader in geological research. The analysis suggests two potential approaches: either retrofitting existing laboratories to improve their environmental performance or repurposing them for office use and constructing a new facility using biobased materials. Such steps would not only enhance the operational efficiency of the BGS but also align with broader sustainability goals, ensuring that the campus remains a cutting-edge hub for scientific inquiry.
The case study on Arup & Material Cultures highlights the transformative potential of biobased materials in construction, particularly through their environmental, economic, and regional benefits. However, it also highlights the challenges that remain in scaling up their use. Material Cultures’ work provides a solid foundation, illustrating how biobased materials can reduce carbon emissions, enhance biodiversity, and stimulate local economies through new supply chains. However, gaps exist in addressing the transition from traditional extractive industries and the need for a more holistic, regional landscape approach.
The Cultivating Commons project seeks to advance this by proposing a more integrated strategy, focusing on upskilling local workforces, implementing Public-Common Partnerships (PCPs), and establishing a regional bioeconomy that aligns with sustainable land management practices. By doing so, it aims to bridge the existing gaps, ensuring that the adoption of biobased materials is not only environmentally sustainable but also economically and socially equitable, providing a replicable model for future developments. PCPs provide a collaborative framework that ensures diverse stakeholder involvement, including public entities, local communities, and private partners. This collaborative approach is essential for balancing ecological, social, and economic benefits and preventing the concentration of advantages within a single entity. By engaging a broad spectrum of participants, PCPs promote equitable distribution of resources and opportunities, aligning with the goals of sustainability and regenerative design.
In adopting a bioregional approach, this study emphasizes the importance of aligning economic activities with the natural resources and ecological functions of the East Midlands. This approach not only fosters sustainability and resilience but also encourages a stronger sense of community identity and stewardship. However, the transition to a bioregional economy is not without its challenges, such as potential political resistance and logistical difficulties due to the misalignment with existing administrative boundaries. Despite these hurdles, the phased strategy outlined for the East Midlands focusing on biobased material production and leveraging local resources demonstrates the potential of this approach to drive regional development while addressing broader environmental and social challenges. This perspective highlights the need for comprehensive planning and cooperation across various sectors and scales to achieve sustainable outcomes.
The phased approach to the uptake of biobased materials in the East Midlands illustrated a strategic pathway from initial prototyping to broader regional implementation. Phase 1 focused on leveraging local resources and testing biobased materials, while Phase 2 projected a larger-scale integration, including potential synergies with offshore wind farms. This strategic expansion not only addresses material production but also considers the broader impact on regional landscapes and economies.
The prototyping of the British Geological Survey (BGS) campus and surrounding landscapes represents a pivotal step towards advancing sustainable construction and agricultural practices. The retrofit plan integrates biobased materials such as straw panels and shellfish-based facades, aiming to enhance the building’s functionality while fostering a harmonious connection
with the outdoor environment. The design emphasizes biodiversity through landscaping and water management solutions, which together address both aesthetic and ecological concerns. Additionally, the incorporation of straw, seaweed and shellfish materials not only improves the building’s insulation and acoustics but also supports local economies by utilizing regionally sourced resources. The establishment of prototyping sites for testing these materials and practices further facilitates the transition to biobased building. By engaging in hands-on experimentation and education, these sites aim to refine practices and empower workers and farmers in the East Midlands. Similarly, the Seaweed and Shellfish Farm Prototype offers a transformative approach to coastal resource management, enhancing both environmental restoration and economic resilience.
The concept of strengthening the corridor of production emerged as a strategy, emphasizing the need to connect land and sea through a network of ecological patches and corridors. This approach integrates recreational and ecological networks such as greenways, waterways, and traditional agricultural features to enhance regional biodiversity and connectivity. It underlines the importance of aligning material use with landscape and ecological considerations to foster a more cohesive and sustainable regional framework.
Reflecting on the introduction of this thesis, Cultivating Commons has addressed the urgent need for innovative solutions to climate change and sustainable development. The project shows the interconnectedness of production landscapes -both natural and built- and their potential to positively influence regional ecologies and local economies. By transitioning from extractive materials to biobased alternatives, Cultivating Commons aims to mitigate carbon emissions, enhance soil health, and support biodiversity while fostering community empowerment and economic diversification. Cultivating Commons envisions a regenerative economy where landscapes of production not only sustain people and the planet but also contribute to building a shared surplus of resources. The insights gained from discussions with experts have been instrumental in shaping our understanding and focus throughout this thesis. Their contributions have deepened our knowledge of biobased materials and highlighted key areas for development and application.
However, we recognize that challenges remain, particularly in the UK context, where issues such as insurance limitations currently hinder broader adoption of these innovative materials. Our research highlights the importance of scaling up and investing more in biobased materials. As illustrated in the Public Common Partnerships (PCP) model, addressing these constraints through increased investment and supportive policies can help mitigate existing barriers, making these materials accessible and encouraging more engagement. By overcoming these hurdles, we aim to foster an environment where biobased materials become standard practice, benefiting both the environment and the community.
As we strive to align with the UK’s target for Net Zero by 2050, it is crucial to understand that this goal extends beyond simply retrofitting or constructing new buildings. It demands a holistic vision that considers all aspects of the landscape impacted by these efforts. Designers are therefore called to integrate these principles into their practice, ensuring that every decision not only advances sustainability but also thoughtfully considers how their choices transform and shape the landscapes they design. The findings from this thesis provide a foundation for ongoing research and application, setting the stage for creating landscapes that offer benefits to both people and the environment.
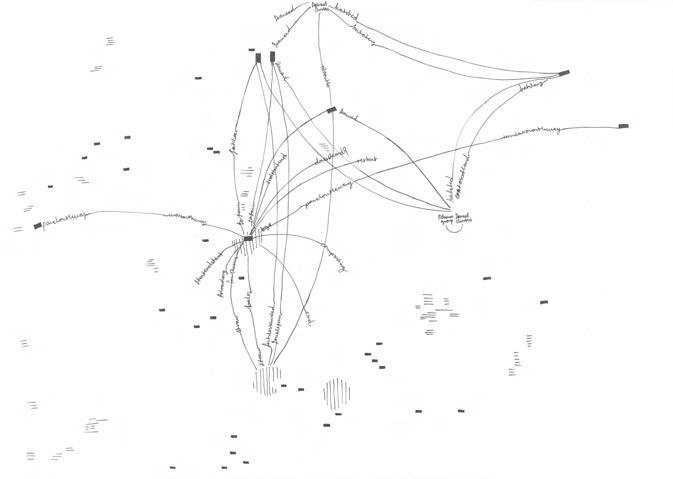
Conclusions
This thesis has been a transformative experience, shaping not only my understanding of biobased buildings but also deepening my appreciation for the complexities behind sustainable architecture. One of the key takeaways for me has been the realization that biobased construction does not need to remain confined to small-scale or niche projects. Too often, we associate biobased materials with either luxury eco-homes or low-cost housing initiatives. This view limits the extent to which biobased construction can make an impact. If we are really going to align with Net Zero goals we need to amplify the spectrum and make a concerted effort to scale up the production of biobased materials through PCP or community-owned regenerative farming practices, supported by quality labour and proper governmental grants. These materials should be implemented into cultural, governmental and institutional buildings, just as this thesis proposes for the BGS campus.
However, rather than demonizing brick and concrete, we should question the site to be intervened, ensuring that the solutions come from the site without alienating it, and responding directly to it. What I argue for is a more conscious selection process as designers. “Green” isn’t inherently better unless we can trace the entire lifecycle of a material. It’s crucial to ask, Where did this come from? How was it extracted? Who was involved, and were they treated fairly? Who owned the means of production? Often, in our rush to embrace sustainable trends, we overlook the labour practices tied to these materials, effectively externalizing the human cost from the product. This gap is something we need to close if we are to create truly sustainable systems.
We must centre local communities in the design, opening conversations about the place they will inhabit to make sure it responds and fosters local identity and community cohesion. There are challenges and open questions within the scope of this project, especially in predicting how local communities - such as fishermen or workers in extractive industries might react or engage with these biobased initiatives. The trails and corridors designed to connect the community to these changes are vital, but we must ask, Will they foster a sense of belonging and identity, or will they create resistance? This social engagement is as important as the material choices we make and could, in turn, shape stronger and more cohesive communities.
Incorporating a Public-Common Partnership (PCP) model, as proposed for the BGS retrofit, offers a more transparent and equitable way to manage material sourcing. It is not just about creating direct connections, but about establishing publicly and community-owned industries that drive the biobased transition. By empowering local communities to take ownership of the production and supply chains, we can foster a system that is ecologically, economically, and socially sustainable. And this, I believe, is the greatest challenge: achieving balance on all three fronts. Every step we take toward that balance is a sign that we are moving in the right direction.
Addressing the commons through the PCP model also has broader implications for resource management. By involving local communities in the stewardship of their landscapes, we can ensure that flora, fauna, and human needs are all considered in balance. This kind of holistic planning could benefit not just public institutions but also private companies willing to adopt these principles for their own projects.
Throughout this process, I’ve come to see the project as a vast canvas with a web of interconnected elements that goes beyond simple material choices. From harvesting to composting, every decision impacts the next, and this broader understanding of the supply chain has helped me reflect on how crucial it is to engage with the entire life cycle of a building. The story of each material, the people who work with it, and the environment that supports it all contribute to a more meaningful and sustainable design. Equally important is the role of policy not just as an incentive for change but as a tool for regulation. Without strong political frameworks that prioritize sustainability, we risk falling short in addressing the environmental, social, and economic dimensions of building. Policies are essential for ensuring that the biobased transition is not only possible but also equitable and scalable.
understand that real-world projects are often unpredictable, and many variables can shift the course of a plan. But this thesis serves as a theoretical spine for navigating those changes. In practice, things may evolve, but the core principles outlined here can help ensure that the decisions we make are grounded in a commitment to a truly sustainable future.
Growing up in Peru, I’ve had the privilege of working directly with artisans and local materials. This proximity to the source has always inspired my work. Artisanal craftsmanship is closely tied to the landscape and its ecosystems, and the materials used are often a reflection of what is available in the surroundings. Yet, also understand the challenge of tracking the supply chains of more industrial materials, like bricks and cement. This is often a blank box, and we rarely consider the working conditions of those who produce these materials. This disconnect has shaped my commitment to keep pursuing more traceable and ethical building practices.
APPENDIX
Invitation for Key-Informant interviews
Ethics Application - Approval notice
Engagement Overview
List of Figures
List of Illustrations
List of Maps
Bibliography
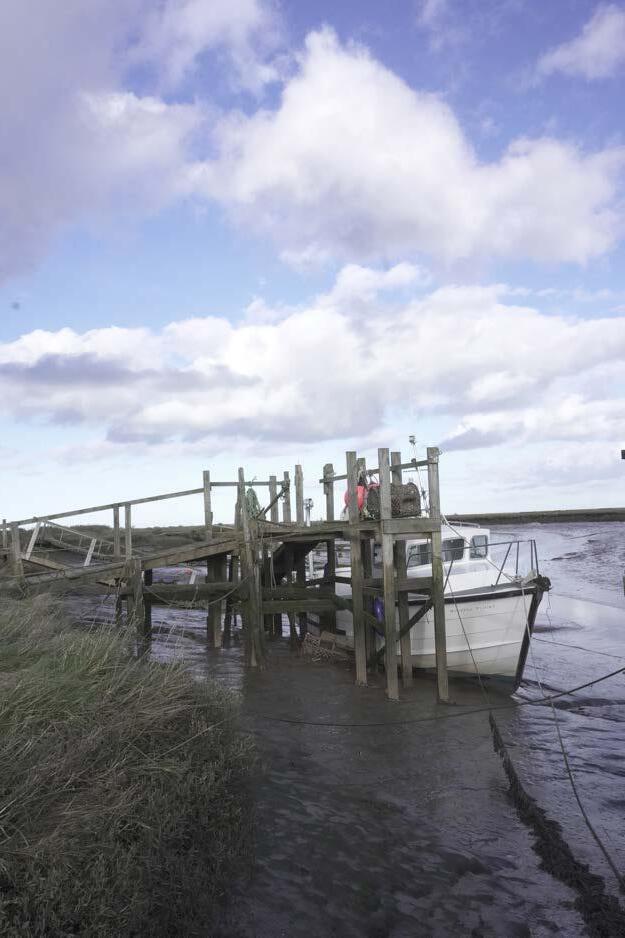
Invitation for Key-Informant interviews
Sample e-mail shared with experts to participate in an interview
Ethics Application - Approval notice
Formal ethics application submitted and approved by the AA ethics board
Norfolk Seaweed allie@norfolkseaweed.com
Hi Allie,
My name is Emily Bowerman, and I am a Landscape Architect currently studying at the AA School of Architecture in London in the Landscape Urbanism program. My colleagues Alejandra and Priyanka and I are undertaking thesis research exploring the potentials of using biobased materials in the construction industry – one of those materials being seaweed.
We were very excited to see your involvement in the Hethel Innovation project regarding seaweed farming in East Anglia. We are looking to learn more about seaweed production in the UK and would greatly appreciate your input on our work.
As we are in the initial stages of our study, there are lots of opportunities to shape and mould our work to reflect current industry needs. Therefore, we were wondering if there would be an opportunity to meet with yourself or one of your team members to share more about our research and learn more seaweed farming and the seaweed industry in the East Anglia region.
Please let us know if you would be willing to discuss with us. We would be happy to arrange a phone call, otherwise, we do intend to be in East Anglia/East Midlands region on February 23rd and could meet with you.
Please let us know if this is something you would be willing to accommodate.
Thanks kindly,
Emily
From: Mike Aling
Sent: Tuesday, February 20, 2024 3:40 PM
To: Priyanka Awatramani; Emily Bowerman; Alejandra Iturrizaga Andrich
Cc: Clara Oloriz
Subject: Ethics approval
Dear Priyanka, Emily and Alejandra,
I hope that you are keeping well. I am emailing to confirm receipt of your application for ethical approval for the project: Retrofitting of BGS using bio-based materials.
This application has been approved.
If you have not already done so, please make sure to read the participant checklist. For gaining signatures for consent, you can utilise the Participant Consent Form. Both of these forms can be found on the AA Intranet by going to the ‘Key Documents’ tab and clicking on the ‘Ethics…’ link under the heading ‘General Policies or Processes’.
Wishing you a successful project,
Kindest regards, Mike
Mike Aling
Deputy Head of Teaching and Learning Architectural Association School of Architecture 36 Bedford Square London WC1B 3ES mike.aling@aaschool.ac.uk www.aaschool.ac.uk
Ethics application completed by Emily Bowerman and Priyanka Awatramani
Emily Bowerman
Engagement Overview
NAME & ORGANISATION INTERVIEW STATUS NOTES
Allie Wharf, Nortfolk Seaweed, Norfolk UK In-Person interview & Site Visit February 2024 +Ongoing communications via e-mail. +Shared contacts for additional interviewees +Seaweed farming and East midlands coastal insights
Kai Heron, ABUNDANCE In-person interview +Identified areas for improving policy diagram for establishing PCP
Kathryn Larsen, Studio Kathryn Larsen Contacted via e-mail +Provided insights regarding Eelgrass vs. Kelp as a building material
BlueBlocks, Netherlands Unable to contact +Seaweed acoustic panels
Ella, Câr-y-Môr, Wales Declined interview & Site visit
Karishma Tuladhar, UCL Bio Integrative Design Graduate Virtual Interview +Microalgae research
Anika Riopel, Ecology Action Centre, Nova Scotia, Canada Unable to contact +Sustainable seaweed production in Nova Scotia
UK Hempcrete Unable to contact +Information regarding hempcrete products and cost
Ben and Loretta, Local Works Studio Declined interview +Architecture firm working with sustainable building materials
Bradley Nissen, UKGBC Virtual Interview +Sustainable building construction
make Architects, London Unable to contact +Straw panels for Insitutional projects
Johanna Gibbons, J&L Gibbons, London, UK Unable to contact +Sustainable resource management & landscape transformation in the UK
Material Cultures Attended in-person lecture at the Barbican February 2024 Unable to contact for an interview +Reinforced opportunities and gaps in their current research
Eco Cascade Unable to contact + Innovation start-up for Seaweed processing in Scotland
Sam Fenshawe, Blue Marine Virtual Interview + Seaweed farming insights
Anonymous Key Informant, Marine Research Organization Virtual Interview + Seaweed and farming insights
Cypren Edmunds, Straw Bale Building UK Virtual Interview + Straw bale building insights
Andrew Barkwith,BGS In-person Interview and BGS visit + BGS needs and campus tour
Ciaran Malik, AA In-person Interview + Construction details
Katya Briskina, EM Tech Alumni Virtual Interview + Shellfish as material
Nataly Nemkova, AA LU Alumni Virtual Interview + Shellfish as material
ill.02 Engagement Overview / Summary by Emily Bowerman and Alejandra Iturrizaga Andrich
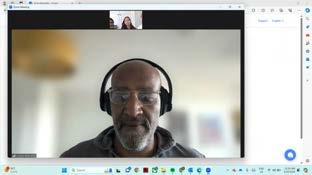
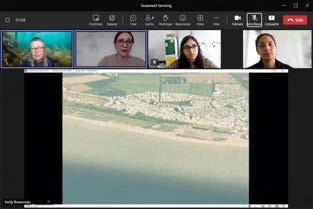
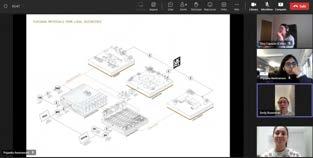
List of Figures
10, 152 fig.01
Source: Unknown at The Centre for Agroecology, Water and Resilience, Coventry University
16 fig.02 Aerial view of the Mary Ward College as it was offered for sale in 1975 / Source: BGS
36, 81 fig.03 Seaweed Harvest / Source: Atlantic Sea Farms
121 fig.04 Seaweed Acoustic Panels / Source: BlueBlocks, Netherlands
40 fig.05 Regenerative Ocean Farm / Source: Câr-Y-Môr, Wales
40 fig.06 Irish Moss Seaweed – Organic, Wild Harvested from Donegal Bay Source: unknown
169 fig.07 Seaweed Harvest / Source: Câr-Y-Môr, Wales
42, 169 fig.08 Straw harvesting / Source: © StockFood
169 fig.09 Straw Panel / Source: BlueBlocks, Netherlands
46 fig.10 Wheat Farm / Photo by Bryan Bian, 2019. Source: Pixels.com
46 fig.11 Straw Pile Photo by Dusan Petrovic
52 fig.12 Hemp Harvest / Source: East Yorkshire Hemp
169 fig.13 Hemp Panel / Source: Homeland Hempcrete
56, 169 fig.14 Urban Mycelium Farm Source: © Mike Botha, Vegout Fungi Ltd
169 fig.15 Mycelium Panel Source: Mykor
60, 169 fig.16 Clay Extraction Site / Source: Pixabay
169 fig.17 Adobe Brick Wall / Photo by Carlos Santa Maria, Source: Adobe Stock
64, 169 fig.18 Timber Forest / Source: Wikimedia Commons
169 fig.19 Wooden panel / Source: unknown
68 fig 20 Biobased materials, hempcrete, wood wool and timber in the interior of Flat House in Cambrigeshire / Source: Material Cultures
70 fig.21 Biobased materials, straw and timber in The Gateway Building, University of Nottingham’s Sutton Bonington Campus / Source: Make Architects
69 fig.22 Biobased material, Mycelium Foam Insulation in interior of a residence Source: Mykor
83 fig.23 Core Stores, British Geological Survey, Keyworth, March 08, 2024 / Photo: by Clara Oloriz
78 fig.24 British Geological Survey, Keyworth, March 08, 2024 / Photo: by Alejandra Iturrizaga Andrich
80 fig.25 NEIF Archaeological Radiocarbon Laboratory at BGS / Source: Geo-Biosciences Advanced E-Learning Academy
81 fig.26 Stone Wool Insulatio Panel / Source: Kooltherm Insulation
81 fig.27 Dunald Mill Limestone Quarry Nether Kellet, England / Source: Atlas Obscura
81 fig.28 Mineral Wool Acoustic Panel / Source: Prima acoustic Nimbus
81 fig.29 Slag Grinding Plant / Source: Guangxi Guigang Hengyao steel Co. Ltd
81, 122 fig.30 Straw Insulation Panel / Source: Ecococon.eu
81 fig.31 Wheat Farm / Source: unknown
87 fig.32 “7-day workshop” Source Createlondon Instagram
84 fig.33 Cover Page of Circular Biobased Construction in the North East and Yorkshire Repor / Source: Material Cultures and ARUP
86 fig.34 From the Report - Hemp Supply Chain Network Strategy (2036-38) / Source: Material Cultures and ARUP
88 fig.35 Adaptation of Kate Raworth’s Doughnut Economics model in The Doughnut for Urban Development - A Manual
142 fig.36 Site Visit: Visiting Allie Wharf from Norfolk Seaweed at Wells-next-the-sea, Norfolk on February 23, 2024 Photo: by Alejandra Iturrizaga Andrich
22 fig.37 Four Heavy Equipment Vehicles in a Quarry During the Day by McLendon, Shane / Source: Unsplash
26 fig.38 “Leicester’s High Street to Get New Look in Major Revamp.” Source: BBC News
35 fig.39 Zines, August 2024 / Photo: by Alejandra Iturrizaga Andrich
70 fig.40 “UK Wheat Crop Is the Largest since 2019. Image by Henry Arden Source: FeedNavigator
81 fig.41 Seaweed Acoustic Panels / Source: BlueBlocks, Netherlands
87 fig.42 “Rising from the Water: Designing in a Climate Emergency” Source: Createlondon Instagram
87 fig.43 “Material Matter[s] Workshop #1 - Straw CIVIC SQUARE Courtyard” / Source: Civic Square: Instagram
87 fig.44 “Material Matter[s] Workshop #1 - Straw CIVIC SQUARE Courtyard” / Source: Civic Square: Instagram
123 fig.45 1:10 model of refurbished construction detail / Photo: Alejandra Iturrizaga Andrich
124 fig.46 Alejandra building 1:10 model Photo: by Jose Bazo
125 fig.47 Alejandra making Shellfish rendering. Process Photo: by Jose Bazo
126 fig.48 Shellfish and lime rendering on straw / Photo: Alejandra Iturrizaga Andrich
127 fig.49 Shellfish rendering tests composition / Photos: by Alejandra Iturrizaga
139 fig.50 Economic Sector Review Report: Skegness Neighbourhood Plan/ Source: East Lindsey Distric Council
139 fig.51 “Skegness Gateway Development to Include 1,000 Homes and a Business Park.” Source: BBC News
146 fig.52 News / Source: Hethel Innovation
157 fig.53 Site Visit:
161 fig.55 Screenshot of Interview with Sam Fanshawe from Blue Marine Foundation
UK
161 fig.56 Screenshot of Interview with Anonymous Key Informant from Marine Research Organization
List of Illustrations
13 ill.01 Research Methodology
160 ill.02 Term 2 Engagement Overview
19 ill.03 3D of BGS Campus
30 ill.04 Biobased Material icons
33 ill.05 Viability Framework
37 ill.06 Seaweed Matrix
43 ill.07 Straw Matrix
48 ill.08 TImeline for Seaweed and Straw
53 ill.09 Hemp Matrix
57 ill.10 Mycelium Matrix
61 ill.11 Clay Matrix
65 ill.12 Timber Matrixd materials, hempcrete, wood wool and timber in
81 ill.13 BGS Retrofitting Conventional vs Biobased
89 ill.14 Supply Chain Diagram
93 ill.15 PCP Proposal
97 ill.16 East Midlands Bioregional boundaries
106 ill.17 Regional ecologies: bog/ fen/ peatland
107 ill.18 Regional ecologies: deciduous woodland
108 ill.19 Regional ecologies: cement plant
109 ill.20 Regional ecologies: sand and gravel quarry
110 ill.21 Regional ecologies: urban area
111 ill.22 Regional ecologies: cropland
112 ill.23 Regional ecologies: pastureland
113 ill.24 Regional ecologies: saltmarsh
114 ill.25 Regional ecologies: mudflat
115 ill.26 Regional ecologies: inter-tidal zone
117 ill.27 BGS current status
118 ill.28 BGS retrofit proposal in summer
120 ill.29 BGS retrofit proposal in winter
121 ill.30 BGS indoor lab and workspace retrofit proposal
122 ill.30 BGS retrofit proposal facade
128 ill.32 Cliffton town before agroecological farming
128 ill.33 Cliffton town after agroecological farming
130 ill.32 Agroecological farming section
132 ill.33 Agroecological farming
134 ill.34 Prototyping site section
137 ill.35 Prototyping site
138 ill.36 Community of Skegness before Seaweed farming and Rewilding
138 ill.37 Community of Skegness after Seaweed farming and rewilding
140 ill.38 Onshore activities and upskilling shellfish farmers
140 ill.39 Recreational floats
140 ill.40 Conceptual rewilding and seaweed farm section
154 ill.41 “Building connections”. Figure based on the supply network for BGS map by Alejandra Iturrizaga Andrich
map.01 Concentration of UKRI institutions
UK Research and Innovation (UKRI). “Nottinghamshire Map.” Accessed April 21, 2024. https:// www.ukri.org/.
UK Research and Innovation (UKRI). “Nottinghamshire Map.” Accessed April 21, 2024. https:// www.ukri.org/.
map.02 UKRI Institutions
UK Research and Innovation (UKRI). “Nottinghamshire Map.” Accessed April 21, 2024. https:// www.ukri.org/. Google Maps. “Building condition.” Google Maps. “Building condition.” Accessed April 21, 2024. https://www.google.com/maps/.
map.03 Geological Reserves and Stone Quarries
British Geological Survey. 2020. “Reserves & Quarries.” Directory of Mines and Quarries, 2020: 11th Edition. Keyworth, Nottingham, British Geological Survey. OR/20/036. © UKRI 2020. Accessed April 21, 2024. URL: www2.bgs.ac.uk/mineralsuk/download/dmq/Directory_ of_Mines_and_Quarries_2020.pdf.
map.04 Sand and Gravel Reserves and Industries
British Geological Survey. 2020. “Reserves & Quarries.” Directory of Mines and Quarries, 2020: 11th Edition. Keyworth, Nottingham, British Geological Survey. OR/20/036. © UKRI 2020. Accessed April 21, 2024. URL: www2.bgs.ac.uk/mineralsuk/download/dmq/Directory_ of_Mines_and_Quarries_2020.pdf.
McCarten, M., Bayaraa, M., Caldecott, B., Christiaen, C., Foster, P., Hickey, C., Kampmann, D., Layman, C., Rossi, C., Scott, K., Tang, K., Tkachenko, N., and Yoken, D. 2021. “Global Database of Cement Production Assets.” Spatial Finance Initiative, UK Centre for Greening Finance and Investment (CGFI).
McCarten, M., Bayaraa, M., Caldecott, B., Christiaen, C., Foster, P., Hickey, C., Kampmann, D., Layman, C., Rossi, C., Scott, K., Tang, K., Tkachenko, N., and Yoken, D. 2021. “Global Database of Iron and Steel Production Assets.” Spatial Finance Initiative, UK Centre for Greening Finance and Investment (CGFI).
map.05 Construction Minerals Global Imports Chatham House. 2021. “resourcetrade.earth.” Data. Accessed February 25, 2024. URL: https:// resourcetrade.earth/.
U.S. Geological Survey (USGS). “Major Deposit Database.” Accessed April 21, 2024. URL: https://mrdata.usgs.gov/major-deposits/.
map.06 Landfill vs Composting Sites
EMODnet Human Activities. “Algae Production.” October 9, 2018. Creation: Date identifies when the resource was brought into existence. Unique resource identifier: EMODnet_HA_ Algae_Producing_Industry_20220125. Available at: https://emodnet.ec.europa.eu/ geonetwork/emodnet/eng/catalog.search#/metadata/7b849214- e6ae- 4199-a3f0d4539f1f1289.
EMODnet Human Activities. “Aquaculture, Shellfish.” July 1, 2014. Unique resource identifier: EMODnet_HA_Aquaculture_Shellfish_20220225. Available at: https://emodnet. ec.europa.eu/geonetwork/srv/eng/catalog.search#/metadata/aa0d2b45-49c4-4b4286bb-8971a3c2d2cc.
EMODnet Human Activities. “Main Ports, Goods-Passengers-Vessels Traffic.” May 1, 2014. Creation: Date identifies when the resource was brought into existence. Unique resource identifier: EMODnet_HA_Main_Ports_Traffic_20231106. Available at: https://emodnet. ec.europa.eu/geonetwork/srv/eng/catalog.search#/metadata/379d0425-8924-4a41a088-1a002d2ea748.
map.07 Seaweed Industries and Opportunities
EMODnet Human Activities. “Aquaculture, Shellfish.” July 1, 2014. Unique resource identifier: EMODnet_HA_Aquaculture_Shellfish_20220225. Available at: https://emodnet.ec. europa.eu/geonetwork/srv/eng/catalog.search#/metadata/aa0d2b45-49c4-4b42-86bb8971a3c2d2cc.
EMODnet Human Activities. “Main Ports, Goods-Passengers-Vessels Traffic.” May 1, 2014. Creation: Date identifies when the resource was brought into existence. Unique resource identifier: EMODnet_HA_Main_Ports_Traffic_20231106. Available at: https://emodnet. ec.europa.eu/geonetwork/srv/eng/catalog.search#/metadata/379d0425-8924-4a41a088-1a002d2ea748.
EMODnet. “EMODnet Human Activities, Algae Production.” Accessed October 9, 2018. Unique resource identifier: EMODnet_HA_Algae_Producing_Industry_20220125. [URL: https://emodnet.ec.europa.eu/geonetwork/emodnet/eng/catalog.search#/ metadata/7b849214-e6ae-4199-a3f0-d4539f1f1289]
map. 8a and 8b Straw Producing Crop Yields
DEFRA. “Straw Producing Croplands.” Accessed April 21, 2024. URL: https://naturalenglanddefra.opendata.arcgis.com/datasets/provisional-agricultural-land-classification-alcengland/explore?location=52.504434%2C0.274396%2C6.53&showTable=true.
European Straw Building Association. “ESBA Certified Straw Bale Producers.” Accessed April 21, 2024. URL: https://strawbuilding.eu/map-of-straw-bale-producers/.
The British Hay and Straw Merchants Association. “BHSMA Straw Producers.” Accessed April 21, 2024. URL: https://hay-straw-merchants.co.uk/members.
map. 9 Straw Industries & Opportunities
DEFRA. “Straw Producing Croplands.” Accessed April 21, 2024. URL: https://naturalengland-
defra.opendata.arcgis.com/datasets/provisional-agricultural-land-classification-alcengland/explore?location=52.504434%2C0.274396%2C6.53&showTable=true.
European Straw Building Association. “ESBA Certified Straw Bale Producers.” Accessed April 21, 2024. URL: https://strawbuilding.eu/map-of-straw-bale-producers/. The British Hay and Straw Merchants Association. “BHSMA Straw Producers.” Accessed April 21, 2024. URL: https://hay-straw-merchants.co.uk/members.
map.10 Hemp Industries and Opportunities Hemp Club. “Hemp Farms.” Accessed April 21, 2024. URL: https://hempclubproject.com/map/ map.html. Hemp Club. “Hemp Product Producers and Distributors.” Accessed April 21, 2024. URL: https:// hempclubproject.com/map/map.html.
map.11 Mycelium Industries and Opportunities
Farming UK. “Mushroom Farms.” Accessed April 21, 2024. URL: https://www.farminguk.com/ agricultural-directory/category/mushroom-growers_642.html.
Iturrizaga, Alejandra. 2024. “List of Mycelium Producers in the UK.” Dataset. Self-published. Office for National Statistics. 2023. “Digital vector boundaries for Urban Areas in England and Wales as at 27 March 2001.” Dataset. Accessed June 9, 2023. URL: https://services1. arcgis.com/ESMARspQHYMw9BZ9/arcgis/rest/services/Urban_Areas_ December_2001_EW_BGE/FeatureServer.
map.12 Clay Industries and Opportunities
Mitchell, Clive, and Chloe Wrighton. 2022. “Mineral planning factsheet: brick clay.” British Geological Survey. Accessed April 21, 2024. URL: https://nora.nerc.ac.uk/id/ eprint/532490/1/Brick%20Clay%20Mineral%20Planning%20Factsheet.pdf.
map.13 Timber Industries and Opportunities
Forestry Commission. “Possibly Harvested Woodlands.” National Forest Inventory Woodland GB 2021. Accessed [date of access]. URL: https://data-forestry.opendata.arcgis.com/ datasets/5b91b7041f8b46e099f64aa6d2013e9d_0/about. Contains, or is based on, information supplied by the Forestry Commission. © Crown copyright and database right 2023 Ordnance Survey [100021242].
Forestry Commission. “Sustainably Managed Woodlands.” Woodland that is sustainably managed in England - 31 March 2023. Accessed April 21, 2024. URL: https://data forestry.opendata.arcgis.com/datasets/e1c1d7ead37d4feea303c1bf6f5a0369_0/about. Contains OS data © Crown copyright 2023.
Forestry Commission. “Timber Processing Sites GB.” Accessed April 21, 2024. URL: https:// data- fores try.opendata.arcgis.com/datasets/ e3950cc2d09f47a4ad60871797c83ea9_0/about. Contains Forestry Commission information licensed under the Open Government Licence v3.0. © Crown copyright 2016. map.14 UK Biobased Summary
Natural England. “Administrative Boundaries - Environment Agency and Natural England Public Face Areas.” Accessed April 21, 2024. URL: https://naturalengland- defra.opendata. arcgis.com/datasets/e1215f2ecb0e476dad3dc2d60944d259/explore.
UK Research and Innovation (UKRI). “Nottinghamshire Map.” Accessed April 21, 2024. https:// www.ukri.org/.
Maps 7 to 13, Cultivating Commons
map.15
UK Transition Opportunities
British Geological Survey. 2020. “Reserves & Quarries.” Directory of Mines and Quarries, 2020: 11th Edition. Keyworth, Nottingham, British Geological Survey. OR/20/036. © UKRI 2020. Accessed April 21, 2024. URL: www2.bgs.ac.uk/mineralsuk/download/dmq/Directory_ of_Mines_and_Quarries_2020.pdf.
British Geological Survey. 2020. “Reserves & Quarries.” Directory of Mines and Quarries, 2020: 11th Edition. Keyworth, Nottingham, British Geological Survey. OR/20/036. © UKRI 2020. Accessed April 21, 2024. URL: www2.bgs.ac.uk/mineralsuk/download/dmq/Directory_ of_Mines_and_Quarries_2020.pdf.
DEFRA. “Straw Producing Croplands.” Accessed April 21, 2024. https://naturalenglanddefra.opendata.arcgis.com/datasets/provisional-agricultural-land-classification-alcengland/explore?location=52.504434%2C0.274396%2C6.53&showTable=true
EMODnet. “EMODnet Human Activities, Algae Production.” Accessed October 9, 2018. Unique resource identifier: EMODnet_HA_Algae_Producing_Industry_20220125. [URL: https://emodnet.ec.europa.eu/geonetwork/emodnet/eng/catalog.search#/ metadata/7b849214-e6ae-4199-a3f0-d4539f1f1289]
EMODnet Human Activities. “Aquaculture, Shellfish.” July 1, 2014. Unique resource identifier: EMODnet_HA_Aquaculture_Shellfish_20220225. Available at: https://emodnet.ec. europa.eu/geonetwork/srv/eng/catalog.search#/metadata/aa0d2b45-49c4-4b42-86bb8971a3c2d2cc.
McCarten, M., Bayaraa, M., Caldecott, B., Christiaen, C., Foster, P., Hickey, C., Kampmann, D., Layman, C., Rossi, C., Scott, K., Tang, K., Tkachenko, N., and Yoken, D. 2021. “Global Database of Cement Production Assets.” Spatial Finance Initiative, UK Centre for Greening Finance and Investment (CGFI).
McCarten, M., Bayaraa, M., Caldecott, B., Christiaen, C., Foster, P., Hickey, C., Kampmann, D., Layman, C., Rossi, C., Scott, K., Tang, K., Tkachenko, N., and Yoken, D. 2021. “Global Database of Iron and Steel Production Assets.” Spatial Finance Initiative, UK Centre for Greening Finance and Investment (CGFI).
Society Digimap. “Semi-skilled and un-skilled manual occupations.” QS611UK (Approximated
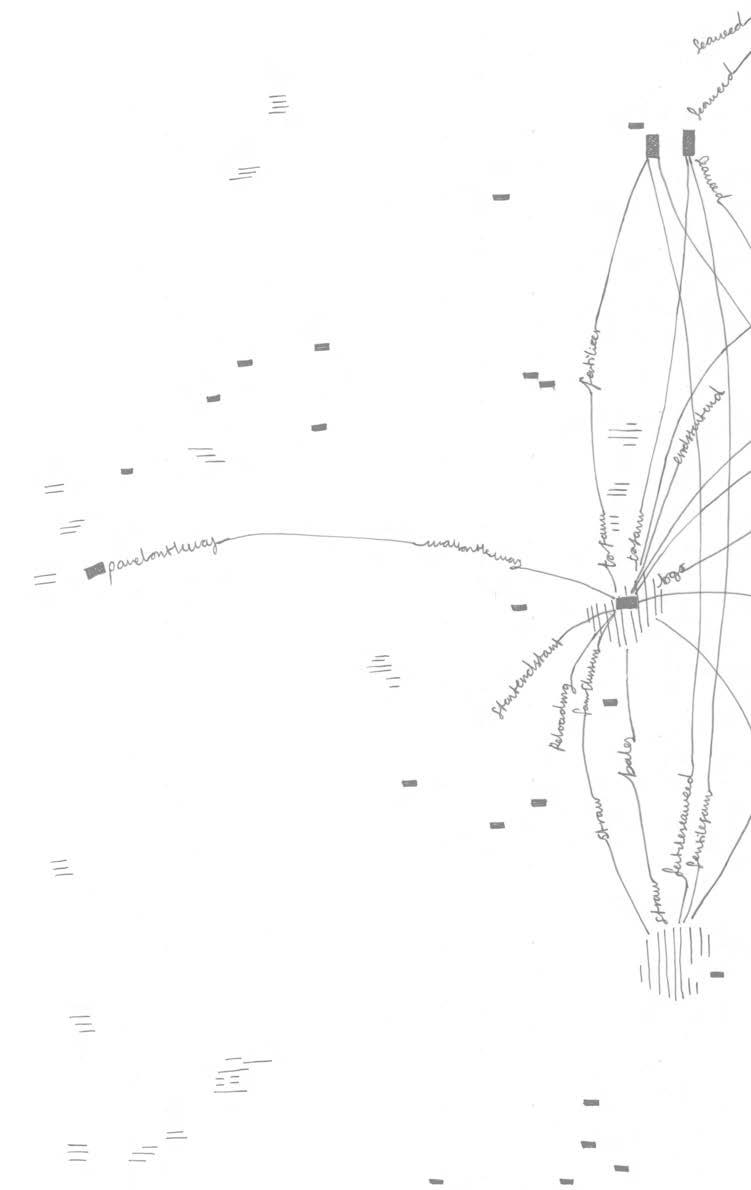
CULTIVATING COMMONS
CULTIVATING COMMONS
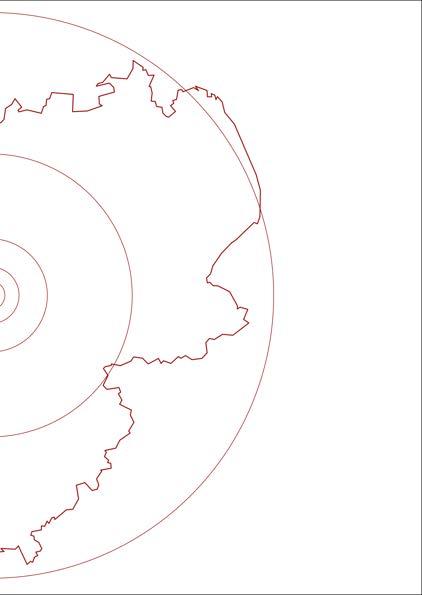
HANDBOOK BGS
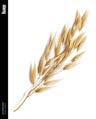
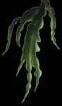
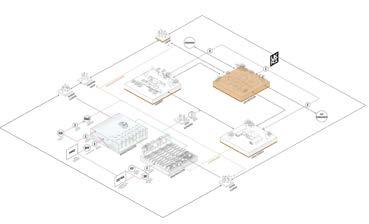
In 2019, the UK government set a target to achieve a 100% reduction in greenhouse gas emissions by 2050 (refer Climate Change Act 2008).1 This necessitates retrofitting public institutions such as the UKRI British Geological Survey (BGS). However, conventional retrofitting typically depends on carbon-intensive building materials, contributing to 40% of global emissions, according to the UK Green Building Council.2 Consequently, emissions from material extraction and production have adverse effects on local well-being and ecologies.
There is an urge to use locally produced biobased materials, offering low embodied energy and in some cases contribute to carbon sequestration or soil regeneration.
To raise awareness, support funding and ensure sustainable procurement practices to uptake biobased material use in retrofitting public institutions, a Public Common Partnership (PCP) is suggested.
Why biobased?
Environment
Biobased materials are renewable during its growth and it is compostable at the end of the building’s lifecycle. Using biobased materials for refurbishment and landscaping around BGS can significantly enhance the environmental and ecological quality of the area
Carbon storage
Biobased materials sequester carbon during their growth and often require less machinery and energy to produce, therefore reduce the carbon footprint.
Energy Efficiency
Most biobased materials have an excellent thermal quality providing comfort and performance to the building.
Health
Healthy environment for workers. Biobased materials have properties which enhance the indoor air quality.
Community Engagement
Using locally sourced biobased materials can engage the local community and boost local economies, fostering a sense of pride and ownership among BGS employees and people involved. This can also enhance the sense of belonging to the East Midlands landscape.
Biophilia
Biosourced materials can enhance the emotional connection between individuals and their living environment by promoting well-being. 1
Innovation and Research
BGS commitment to sustainability and innovation could attract parterships, collaborations and media attention.
Funding and Grants
Could attract funding from governmental organizations that promote green building practices or sustainable farming.
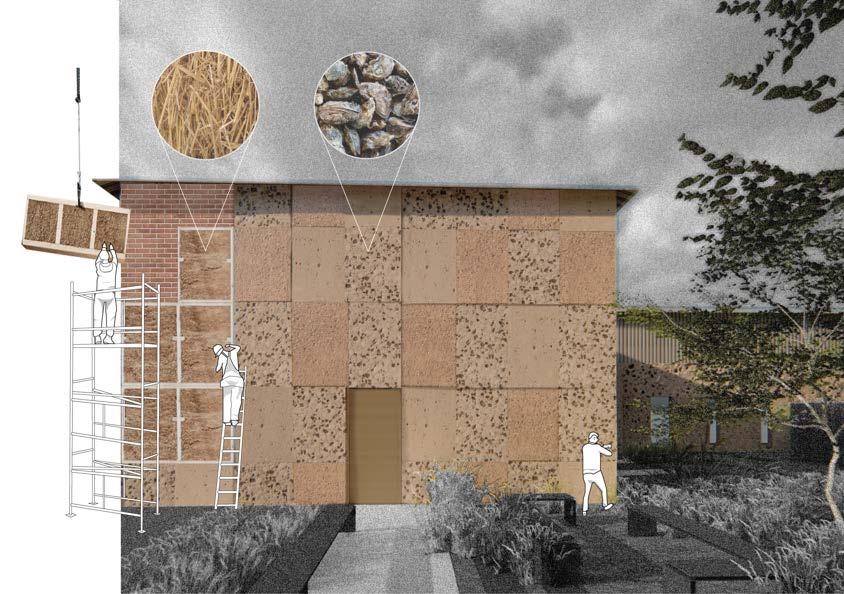
Alliance
Forming an PPP
Public Partnership
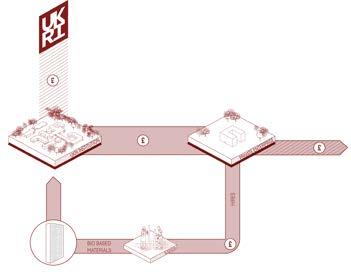
Business as Usual.
This partnership is a collaboration between a private enterprise and government. They often occur in large infrastructure projects that the private partner executes.
From the total investment amount, the private enterprise takes a portion as profit, and the remainder is invested in materials and skilled workers.
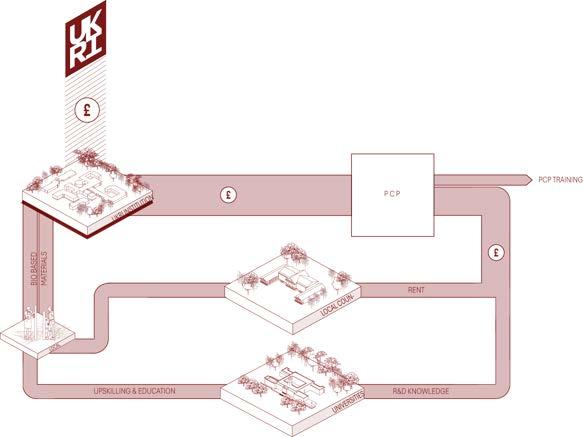
Why is it a
better option?
These partnerships involve collaboration between state authorities and community organizations, with contributions from relevant third parties such as trade unions and local experts.
Under the PCP model, the emphasis is not on profit maximization. Any surplus profits are reinvested into future projects or used to establish additional PCPs. This allows PCPs to focus on sustainable development practices. For instance, it can prioritize refurbishing buildings, reusing materials, and sourcing any needs locally.
Additionally, in this proposal, the PCP encourages the use of biobased materials and invests in research and development to foster innovation and ensure regulatory compliance. By prioritizing local procurement, this approach supports responsible and local sourcing, particularly for retrofitting projects. As a result, PCPs contribute to more sustainable and community-oriented development, strengthen the local economy, reduce transportation emissions, and build resilient supply chains, further enhancing the overall sustainability and community impact of their initiatives.
CURRENT CHALLENGES
1. Limited Awareness and Understanding of PCPs
Lack of awareness and understanding among stakeholders regarding the concept and potential benefits of Public Common Partnerships (PCPs) could impede successful implementation.
2. Securing Funding Sources
Relying only on public funding may pose challenges in ensuring financial resources for large-scale biobased retrofitting projects.
3. Overcoming Resistance to Change, Research and Verification
Resistance from stakeholders, including institutions and local communities, towards adopting biobased retrofitting methods and transitioning from traditional practices is prevalent. Additionally, limited material knowledge impacts the ability to obtain insurance coverage as builders need verified materials to comply with building regulations and insurance companies checks.1
4. Balancing Local Procurement and fair bidding process
Establishing a fair and transparent bidding process for the procurement of biobased materials without imposing restrictive distance radius requirements.
5. Technology and Innovation gaps
Limited technological advancements or innovations in the production of biobased materials may hinder scalability.
6. Limited Capacity for Large Projects
Local and regional businesses often face challenges related to their capacity to undertake large projects, including shortages in resources, inventory, and skilled manpower, restricting them from participating in bids for institutional scale projects.
1. Material Cultures, and Arup. “Circular Biobased Construction in the North East and Yorkshire,2021.” Material Cultures: 102. Accessed April 21, 2024. https://materialcultures.org/2021-circularbiobased-construction-in-the-north-east-and-yorkshire/.
HOW CAN BGS ADDRESS
THE CHALLLENGES?
Investment in Research & Development, Capacity Building and Biobased Production
The UKRI
- Allocate funds for biobased material research and development so materials comply with building regulations and insurance requirements.
- Establish partnerships with local councils, and research institutions to study biobased materials that addresses technological gaps.
- Ensure MMO and DEFRA farming funds, grants, and incentives are leveraged for regenerative raw material production.
Local Institutions
- Implement upskilling programs for the local workforce, facilitated by UKRI funding, to equip workers with the necessary skills for working with biobased materials and supporting the growth of the local talent pool.
LOCAL PROCUREMENT
Local procurement involves acquiring goods and services from suppliers within a specific geographic area, in this case in the East Midlands area.
Fair and Transparent Processes
Ensuring that procurement practices are open and fair to get good quality and competitive prices.
Bidding
Making it easier for local businesses to bid by breaking down larger contracts into smaller ones.
Supporting Local Economies
Buying locally helps boost local businesses and farmers, reducing transportation emissions and supporting the regional economy.
Creating Jobs
Local procurement can create job opportunities, contributing to economic stability in the community.
Economic and Social Value, Community Wealth Building
Ensuring that procurement spending benefits the local economy and community well-being. Financial stability for local workers can lead to increased spending in local shops and restaurants, further supporting the community.
Social and Environmental Impact
Considering the broader social and environmental benefits, like reducing emissions and fostering community health.
Local procurement not only fulfills immediate needs but also supports long-term community and economic health by promoting local businesses and sustainable practices.
Centre for Local Economic Strategies. “CLES - The National Organisation for Local Economies.” Accessed July, 2024. https://cles.org.uk/
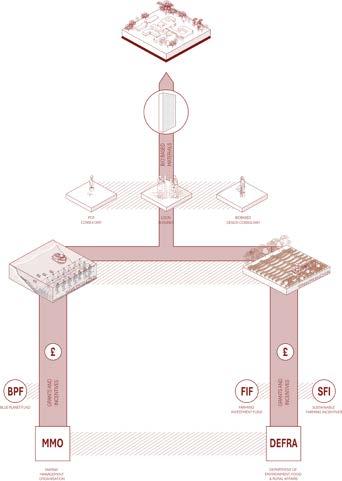
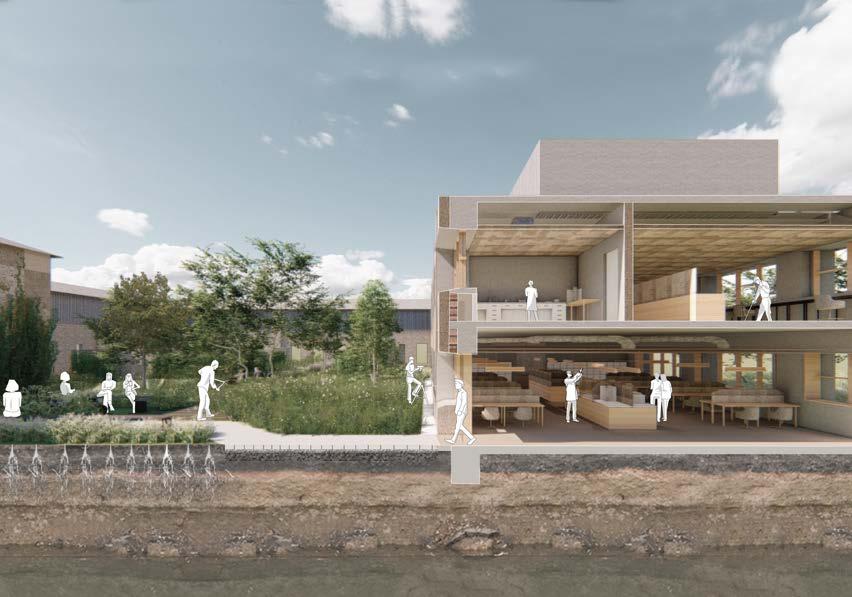
BIDDING ASPECTS
Referencing the French Guide to Biosourced Materials and Public Procurement
1. Identify the biobased materials
Identify the biobased materials that are suitable for the institutional building which needs retrofit by understanding where the material is sourced and the availability of it in the area.
2. Comply with regulations
Ensure compliance with regulations and standards for biobased materials in institutional buildings based on the technical and legal review. For example: Straw needs to be construction quality in order to comply with building regulations, but if used for insulation they do not need this requirement.
3. Energy efficiency
Prioritize energy-efficient biobased materials for improved building performance. These materials offer comfort in summer and winter as most of them are good insulators and regulate humidity which allows saving on heating and airconditioning during the operation phase.
4. Indoor air quality
Choose biosourced materials that contribute to good indoor air quality by selecting low VOC materials that promote a healthy and comfortable indoor environment for workers and visitors.
5. Durability and maintenance
Choose durable biobased materials which require minimal maintenance and evaluate the technical performance. It is important to make a maintenance plan from the beggining of the retrofit or new building to secure the correct performance of the building.
5. Cost
Evaluate the cost-effectiveness of biobased materials by conducting a cost-benefit analysis, considering both the initial investment and long-term savings in energy, maintenance, and operational expenses. Biobased materials usually have a reduced impact on the final cost of the project. For example, insulation can vary in a 1% of the final cost.
6. Collaborate with stakeholders
Engage with stakeholders, including building owners, facility managers, contractors, and suppliers, to promote collaboration and ensure a well coordinated approach when incorporating biobased materials in the retrofitting process.
7. Monitoring and evaluation
Implementing a monitoring and evaluation plan to assess the performance of biobased materials post-retrofitting. This allows to measure the effectiveness of the materials proposed.
5. Guide matériaux biosourcés et commande publique. April 2020, 16.
6. Guide matériaux biosourcés et commande publique. April 2020, 16.
7. Guide matériaux biosourcés et commande publique. April 2020, 19.
8. Guide matériaux biosourcés et commande publique. April 2020, 24.
BGS & East Midlands Authorities
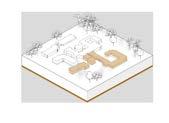
BGS 5 years
Full Refurbishment
Enhance Energy Issue performance.
Utilise existing space efficiently.
Improve building fabric: insulation and accoustics as priority
BGS 10 years
New construction
Build new storage space.
Phase 1
To mobilise the transition to biobased materials in the East Midlands, we are proposing a two-phase approach. Firstly, areas of high unemployment and unskilled workers could be engaged in prototyping and upskilling on lands designated as community allotments or government owned lands and brownfields.
Phase 2
With additional community uptake, we foresee farmers expanding construction grade straw on existing farms. More longterm, there also potential for expanding shellfish farms or establishing new seaweed farms as the industry expands in the UK. Croplands in conservation areas could require special consideration for regenerative farming.
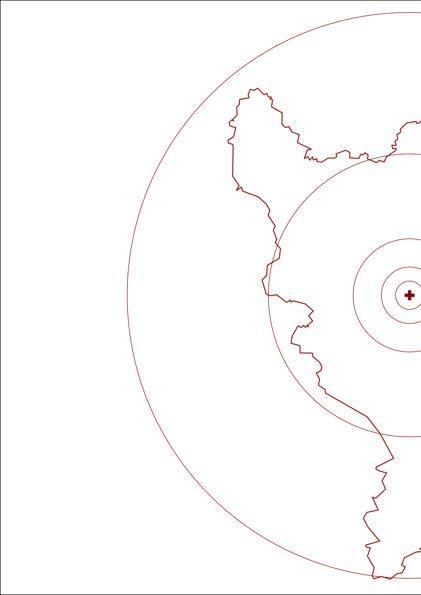
CULTIVATING COMMONS
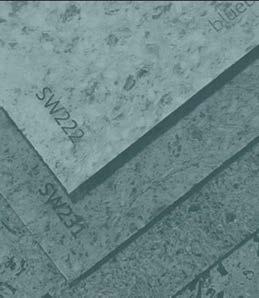
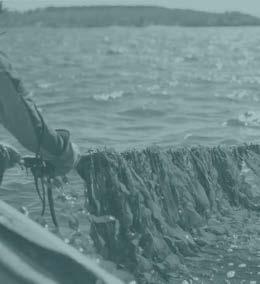
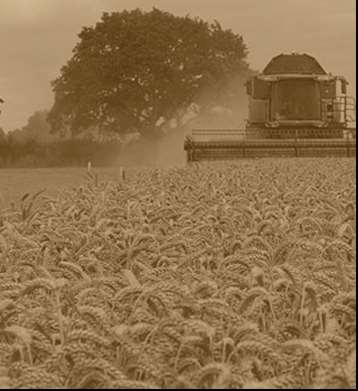
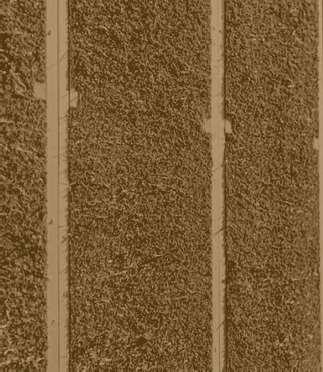
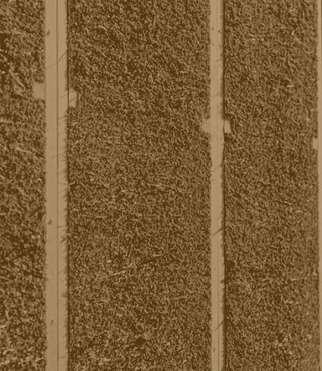
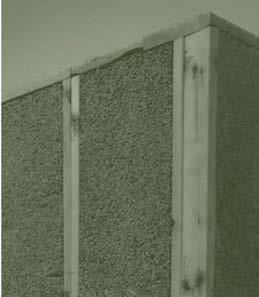

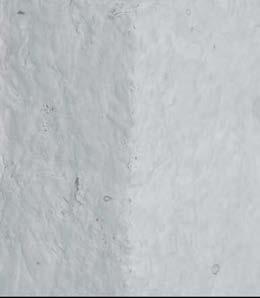
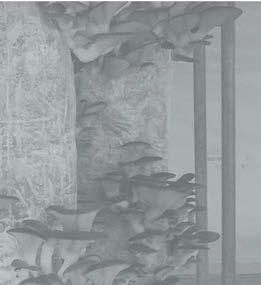
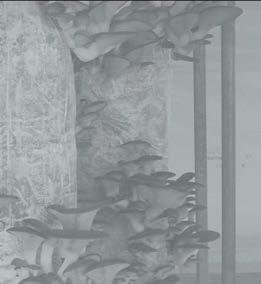
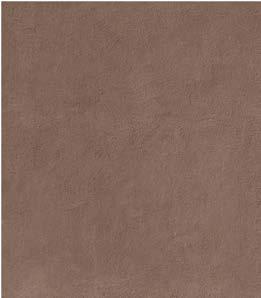
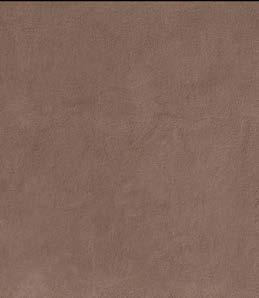
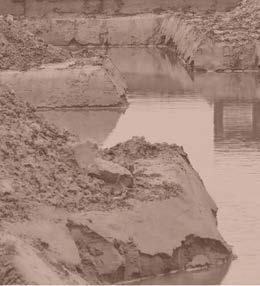
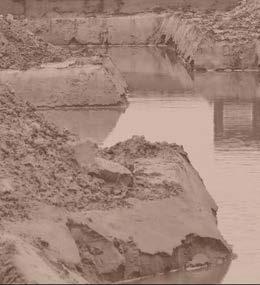
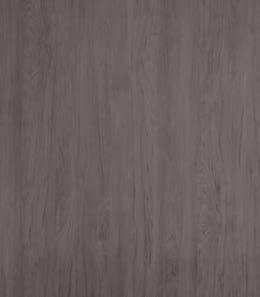
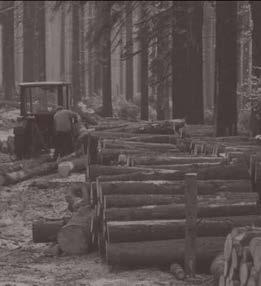