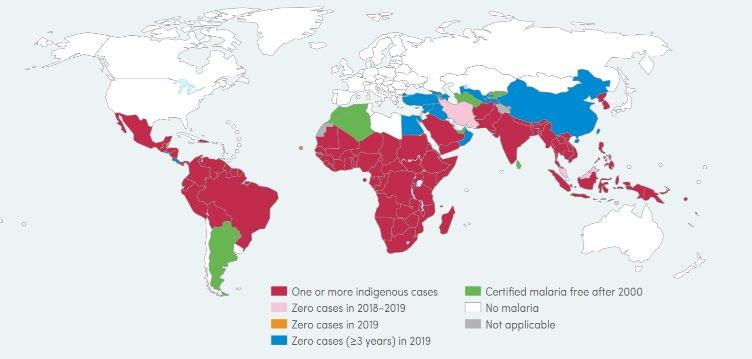
16 minute read
Synthesis of Pyrimethamine
Jess Samuelson
Barker College
Pyrimethamine, introduced in 1952, is an essential medicine due to its anti-malarial, lifesaving properties. However, this essential medicine is now harder to buy due to Turing Pharmaceuticals price increase of the essential medicine, raising the price by 5000% overnight, raising concerns surrounding the ethics of big pharma companies such as Turing. In 2016, Sydney Grammar School was able to successfully synthesise pyrimethamine, cheaply, accessibly and within a high school laboratory, bringing to light the unethical price for pyrimethamine. This report will outline Sydney Grammar’s method to successfully replicate and produce a comparable yield to Sydney Grammar to prove the reliability, accessibility, and the inexpensive nature of their method. The compound itself was analysed at the University of Sydney using nuclear magnetic resonance spectroscopy (NMR) to determine the purity and structure of the compound made. After completing the first two parts of the Sydney Grammar method, a greater yield and similar purity was found to that of Sydney Grammar, further emphasising the reliability of their method.
Literature Review
Malaria is a protozoan parasitic disease transmitted by the female Anopheles mosquito and is found in tropical and sub-tropical regions of the world (Bloland & WHO, 2001) (Figure 1). According to the World Health Organisation (WHO), infection rate is going down, from 238 million new infections in 2000 and 736 000 deaths (WHO, 2020) to 229 million new infections and 409 000 deaths in 2019 (WHO, 2020). There are five different strains of malaria, Plasmodium falciparum, P. vivax, P. ovale, P. malariae and P. knowlesi, with P.falciparum being responsible for 90% of infections (Ashley and Phyo, 2018).
Figure 1: Global map of the geographical distribution of Malaria (Source: WHO, 2020, pg19)
According to the WHO malaria website, symptoms will appear in a non-immune individual 10-15 days after being bitten by an infected mosquito (WHO Global, 2021). Early symptoms include fever, headache, and chills and may be difficult to recognise as malaria (WHO Global, 2021). If not treated over a span of 24 hours, infection can progress to severe illness, with an infected individual experiencing nausea, vomiting and diarrhea (CDCP, 2020) and can be fatal, potentially leading to multi-organ failure (WHO Global, 2021). Children with severe malaria usually develop severe anaemia, respiratory distress, or cerebral malaria (WHO Global, 2021).
Pyrimethamine
Pyrimethamine, (Figure 2), is an antimalarial drug and 2,4-daminopyrimide derivative. 2,4-diamniopyrimides were found to be powerful antagonists of pteroylglutamic acid, further suggesting that pyrimethamine had anti-malarial activity (Falco et al., 1951). It was first synthesised by Gertrude Elion in 1952 and came into medical use in 1953, sold under the name of Daraprim. WHO declared pyrimethamine as an essential medicine (satisfies the priority health care need of the population determined by WHO) as it was an effective drug for treating P.falciparum strains of malaria. It is commonly used in combinations with other compounds, such as sulfoxine, when treating malaria and is combined with sulfoxine and amodiaquine for chemoprevention (WHO, 2019). The drug has also proved effective when treating pregnant women and children with malaria and for treating other diseases such as Toxoplasmosis, caused by a parasite in undercooked, contaminated meat.
However, in the late 20th century, new strains of P.falciparum were found to have developed in Southeast Asia and Africa, leading to an increase in child mortality (Ashley and Phyo, 2018). In 1990, WHO stopped recommending the triple-drug combination of mefloquine-sulfadioxine-pyrimethamine as resistance to the combination was proven (Oaks et al., 1991).
Issues with accessibility
Accessibility to pyrimethamine is extremely important, owing to its natural anti-malarial properties and its use in treating fatal illnesses and diseases. In 2015, Turing pharmaceuticals acquired market rights for Pyrimethamine (Daraprim) from CorePharma and raised prices by 5000% in the United States overnight (Pollard, 2015). To put this into perspective, Daraprim originally cost $13.50 per tablet, which was raised to $750 per tablet (USYD, 2016). For comparison, in Australia, Daraprim costs between 1-2 dollars per tablet. Martin Shkreli, founder of Turing claimed, ‘This isn’t the greedy drug company trying to gouge patients, it is us trying to stay in business.” (Pollard, 2015). Unfortunately, this is not an isolated issue, with drugs used to treat cancer, hepatitis C and high cholesterol also having unfair prices to begin with, while drugs such as cycloserine, used to treat tuberculosis, had a $343.33 price rise per pill. (Pollard, 2015).
Even in 2021, Daraprim remains at $750 USD per tablet, affecting those in developing nations, who are more likely to be at risk of developing these illnesses and may be unable to afford the unnecessarily expensive price. As a result of the high price, research around the development of analogues has been stunted, although they could have the potential to be highly effective against fatal diseases.
Breaking Good
Breaking Good is a project led by Associate Professor Alice Motion at the University of Sydney. The project provides an opportunity for high school students to contribute to drug development to shed light on ethical issues such as raising the price of Daraprim.
In 2016, students at Sydney Grammar School were able to cheaply synthesise the compound in a three-part method, using accessible materials that are safe to use by high school students. They were able to successfully produce 3.7g worth of Daraprim in a high school lab (USYD, 2016). This summates to approximately $111000 which is equivalent to 148 tablets of Daraprim.
Sydney Grammar School is the only school that has been attempted the synthesis of Daraprim. My project will aim to test the reliability of the Sydney Grammar Method by repeating the three-part method in the Barker College school laboratory, measuring the yield and purity of the compound after each step. This will allow us to compare yields to Sydney Grammar and compare purity, meaning we can analyse the reliability of their method effectively.
Reliability within a School Laboratory
It is harder to achieve a successful synthesis within a school laboratory due to the limited technology at our disposal and the difficulty in obtaining chemicals.
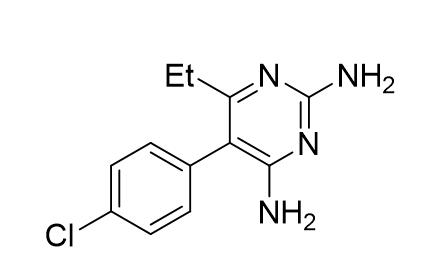
Figure 2: Chemical structure of pyrimethamine.
However, based off Sydney Grammar’s successful synthesis, it can be expected that with the same equipment at our disposal that a similar yield and purity can be gained within a Barker laboratory.
Planned Synthesis
The planned three-step synthesis is the exact procedure Sydney Grammar carried out, testing the reliability by replicating the method within a different school laboratory (Figure 3).
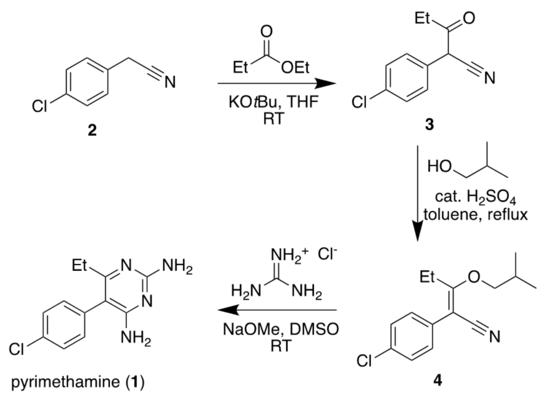
Figure 3: Synthetic pathway of pyrimethamine. Figure 4: Chemical structure of 2-(4-chlorophenyl)-3oxopentantrile.
Figure 5: Chemical structure of 2-(4-chlorophenyl)-3-(2methylpropoxy)-pent-2-enetrile.
Scientific research question
How reliable is the Sydney Grammar School Method to synthesise pyrimethamine?
Scientific hypothesis
That pyrimethamine can be synthesised with a comparable yield at Barker College following the Sydney Grammar School method.
Methodology
Part 1
4-chlorophenylacetonitrile (10g, 0.066mol, 1 equiv.), ethyl propionate (6.87g, 0.067mol, 1.05 equiv.) and potassium tert-butoxide (16.31g, 0.15mol, 2.3 equiv.) were combined in Tetrahydrofuran (THF) (100mL) with stirring. The colourless solution turned red, and the temperature of the solution rose rapidly. The reaction flask was covered in aluminium foil to retain heat. All potassium tert-butoxide dissolved after an hour, leaving a homogeneous solution.
The reaction mixture was worked up by pouring it into 250mL of 1M Hydrochloric acid (HCl) solution and dichloromethane (100mL) was added. An emulsion was noticed, so a saturated solution of brine was added to further separate the layers. However, a precipitate formed, so the precipitate was filtered out of the organic layer and was separated from the aqueous phase. The organic layer was washed with brine (100mL) and dried over anhydrous sodium sulfate, after which the solvent was removed in vacuo to yield 2-(4-chlorophenyl)-3oxopentanentrile (BBG-2020-10, 11.23g, 0.054mol, 81.98%) as a red oil (Figure 4).
Part 2
2-(4-chlorophenyl)-3-oxopentanenitrile (BBG-2020-10, 11.23g, 0.0541mol, 1 equiv.) was dissolved in a mixture of toluene (100ml) and 2-methylpropan-1-ol (12mL). Concentrated H2SO4 (2mL) was added, and the mixture was refluxed for 4 hours using a Dean Stark apparatus. A thin-layer chromatography (TLC) (dichloromethane) of the reaction mixture indicated that some starting material was still present. The main products were two close spots corresponding to the E and Z isomers of the enol ether. The reaction mixture was worked up by pouring onto a solution of saturated sodium hydrogen carbonate and dried over anhydrous sodium sulfate (150mL). The organic layer was separated and dried over anhydrous sodium sulfate. Dichloromethane (100mL) was added to the organic layer.
Triethylamine(10mL) and chromatography silica gel (50g) was added to the reaction mixture to convert the unreacted starting material to its very polar triethylammonium enolate salt. TLC indicated that the polar baseline material had disappeared from solution and absorbed on to the silica. The reaction mixture was filtered to remove the silica gel and washed with 1M HCl (50 mL) and deionised water (50mL) to remove all traces of triethylamine. The solvent was dried over anhydrous sodium sulfate and the solvent was removed in vacuo to yield 2-(4-chlorophenyl)-3-(2methylpropoxy)-pent-2-enenitrile as an orange oil (10.85g, 0.044mol, 80.61%) (Figure 5).
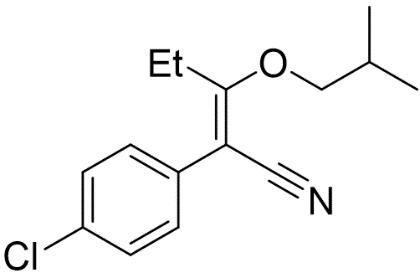
Step 1: Synthesis of 2-(4-chlorophenyl)-3-oxopentanentrile.
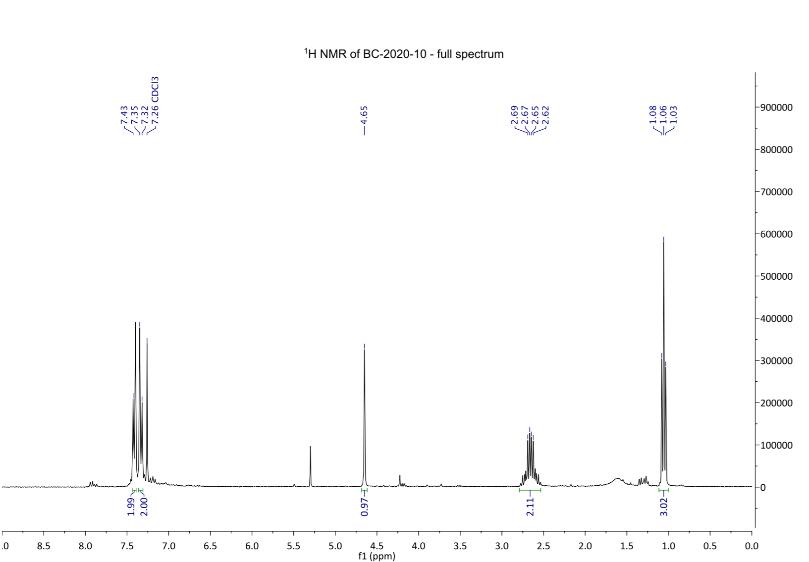
Figure 6: 1H NMR spectra after step 1. 1H NMR (300 MHz, chloroform-d): δ 1.06 (t, J = 7.2 Hz, 3H), 2.66 (m, 2H, H4), 4.65 (s, 1 H, H3), 7.33 (d, J = 8.4 Hz, 2H, H1), 7.41 (d, J= 8.2 Hz, 2H, H2).
Figure 7: TLC after step 1. Figure 9: TLC after substitution reaction
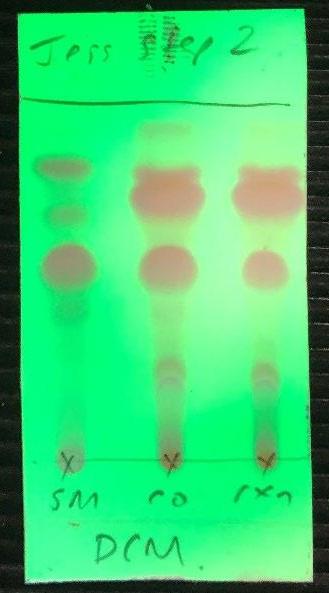
Step 2: Synthesis of 2-(4-chlorophenyl)-3-(2-methylpropoxy)-pent-2-enenitrile
Figure 2: TLC after step 1.
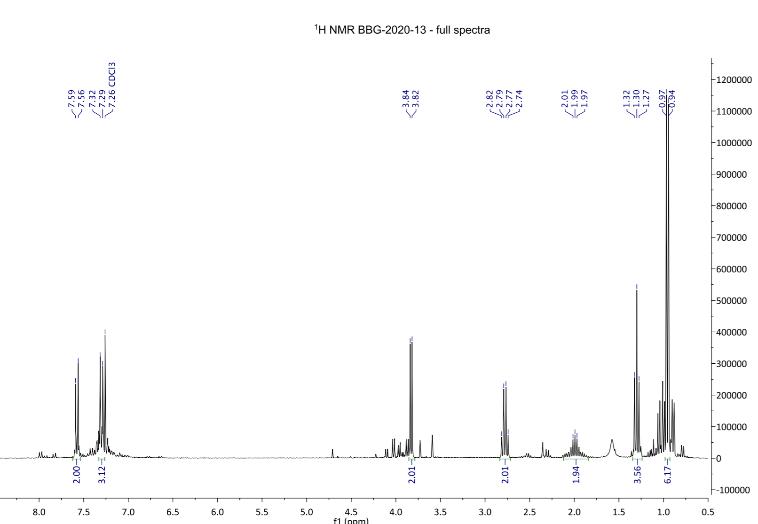
Figure 8: 1H NMR spectra after step 2.
1H NMR (300 MHz, chloroform-d): δ 0.96 (d, J = 76.7 Hz, 6H, H3), 1.30 (t, J = 7.6 Hz, 3H), 2.12-1.84 (m, 2H), 2.78 (q, J = 7.5 Hz, 2H, H4), 3.83 (d, J = 6.4 Hz, 2H), 7.30 (d, J = 8.8 Hz, 2H, H2), 7.58 (d, J = 8.8 Hz, 2H, H1).
Discussion
Step 1: Synthesis of 2-(4-chlorophenyl)-3oxopentanentrile.
O
Cl
2
N Et OEt
KOtBu, THF RT, 82% Cl Et
3
O
N
Figure 10: Reaction of 4-chlorophenylacetonitrile (2) to form 2-(4-chloroophenyl)-3-oxopentanenitrile (3)
The first step of the synthesis was performed to form Compound 3 from a condensation reaction between Compound 2 and ethyl propionate. The potassium tertbutoxide added is a strong base, allowing deprotonation of the CH2 group within Compound 3. The reaction between Compound 3 and ethyl propionate removed the ethanol from the compound. The yield produced for this step was 82%, which is slightly smaller than Sydney Grammar’s yield of 90% (SGS, 2016). This may be the result of the precipitate that formed during the work-up phase, and while in the process of removing the precipitate, some of the compound may have been lost. However, this result is still positive, as it is within the same range of Sydney Grammar, taking the complications into account.
The polar baseline material that appeared on the TLC (Figure 7) matched the expected increased polarity of compound 3 compared to compound 2. The 1H NMR spectrum revealed the high purity of the substance. The doublet at 7.33 ppm was assigned to the aromatic H1 protons and the doublet at 7.41 ppm was assigned the aromatic H2 protons. This was the expected result, as hydrogen atoms on a benzene ring tend to appear between 7ppm to 8ppm due to the deshielding effect of the benzene ring. The singlet signal at 4.65ppm was assigned to the H3 hydrogen adjacent to the carbonyl
and nitril groups. The singlet is further upfield than the two aromatic doublets as the nitrile group and carbonoxygen double bond withdraws electrons, causing the Hydrogen atom to become deshielded in comparison to the upfield signals. The signal at 2.66ppm from the CH2 of the ethyl group, which was expected to be a quartet, but may have been a multiplet due to impurities or restricted rotation at this part of the molecule. The triplet signal at 1.06 ppm indicated that attached to the ethyl group CH2 was a CH3 group (Figure 6). Finally, there is an impurity to be noted between 5.5 and 5.0 ppm, which is most likely residue from the THF solvent used. There is comparable yield between Sydney Grammar’s and Barker College’s compound 3, Sydney Grammar obtained a 90% yield and Barker college obtaining an 82% yield. The NMR shows a mostly pure substance which is comparable to Sydney Grammar. All peaks align from both spectrums, presenting that compound three was formed (Figure 11), meaning that the hydrogens of compound 3 are in similar environments to that of Sydney Grammar. This further emphasises the reliability of Sydney Grammar’s method, as we were able to produce similar results with no significant differences.
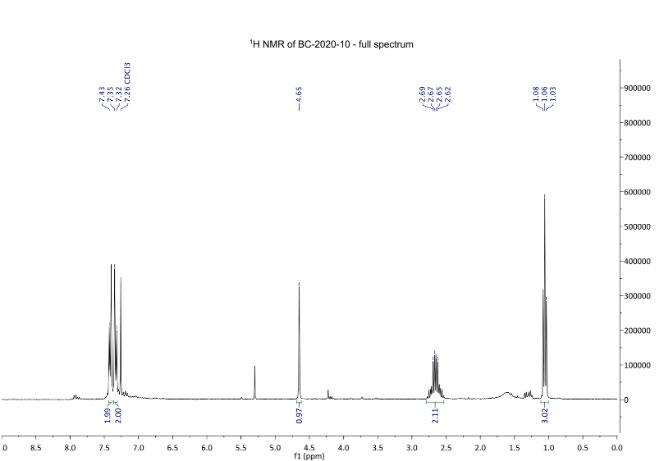
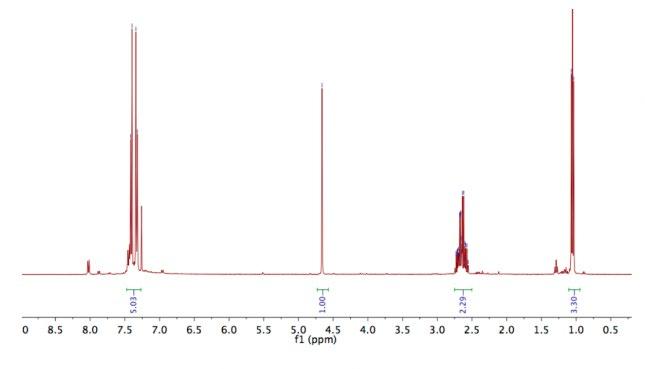
Figure 11: Comparison between Barker College (Top) and Sydney Grammar School (Bottom) 1H NMR after step 1. (After: SGS, 2016)
Cl Et
3
O
N
HO cat. H2SO4 toluene, reflux 81% Cl Et
4
O
N
Figure 12: 2-(4-chloroophenyl)-3-oxopentanenitrile (3) to form 2-(4-chlorophenyl)-3-(2-methylpropoxy)-pent-2enenitrile (4).
The second step of the synthesis was performed to form Compound 4 under reflux, where compound 3 underwent a substitution reaction. A Dean Stark apparatus (Figure 13) removed water, shifting the equilibrium action to the right. TLC indicated that not all starting material had been removed (Figure 9). Sydney Grammar had undergone a similar outcome, so this was to be expected. To remove this residual starting material, triethylamine was added and reacted with the starting material to form triethylammonium salt. Silica gel was added to remove the salt from the reaction mixture. The NMR revealed that some starting material remained (Figure 8). The yield produced for this step was 81%, which is significantly greater than Sydney Grammar’s yield of 60% (SGS, 2016). This may be caused by the amount of starting material remaining after the Dean Stark Apparatus.
The 1H NMR spectrum revealed to be less pure than compound 3. The H1 and H2 signals were assigned two doublets at 7.58 ppm and 7.30 ppm and was assigned to the aromatic H1 and H2. Similar to compound 3, this was the expected result, as these signals come from the benzene ring which tend to appear between 7ppm to 8ppm due to the deshielding effect of the benzene ring. The doublet signal at 3.83 ppm was assigned to the
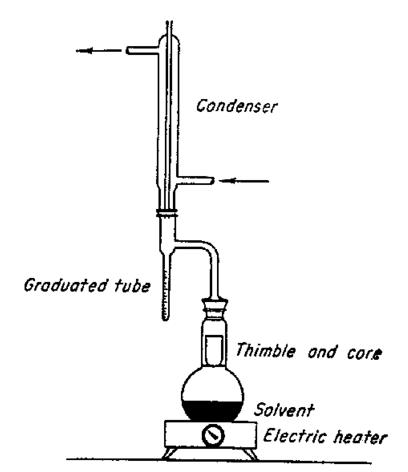
Figure 13: Dean Stark Apparatus Source: (Torsaeter and Abtahi, 2003).
hydrogens adjacent to the carbonyl and CH3 group (H3). The signal is further upfield than the two doublets as the carbon-oxygen double bond withdraws electrons, causing the Hydrogen atom to become deshielded in comparison to the upfield signals. The signal at 2.78 ppm from the hydrogens assigned is a quartet, and it indicates that it is adjacent to the same carbonyl group, meaning Hydrogen atoms become deshielded. The multiplet signal between 2.12 and 1.84 ppm indicates that the Hydrogen is adjacent to a CH3 group. The signal at 1.30 ppm is a triplet and is adjacent to a CH2 group. The doublet at 0.96 ppm is the CH3 group of the isopropyl compound (Figure 8).
There is a significant difference in yields between Sydney Grammar’s and Barker College’s compound 3, Sydney Grammar obtained a 60% yield and Barker College obtaining an 81% yield. Unfortunately, the NMR for Sydney Grammar’s step two synthesis could not be found, so the purities of the two substances could not be compared.
Future Research
Unfortunately, due to other commitments and time constraints, the third part of the synthesis was unable to be completed. If further research is to be commenced, step three would need to be successfully synthesised to further solidify Sydney Grammar’s method as reliable. The method is straightforward due to the accessible materials. Comparing yields and NMR would give a more accurate result of the reliability and potential improvements. Proving this method as reliable is important, as it will be able to shed light on the unethical practice of Turing Pharmaceuticals, while also putting a more reliable, cheaper, and more accessible method into place.
Conclusion
My research project explored the reliability of Sydney Grammar School’s method of synthesizing pyrimethamine. I synthesised pyrimethamine as a threepart process and observed the yield and purity of the compound. Data was collected by NMR from Sydney University to test the purity of each compound and the mass was of each compound was used to calculate the yield after each part. Data analysis involved interpreting the data from the NMR to determine the chemical structure of the compounds. The results of my data showed a comparable yield and purity to that if Sydney Grammar, leading me to accept my hypothesis that pyrimethamine can be synthesised with a comparable yield at Barker College following the Sydney Grammar School method. The first two steps of Sydney Grammar’s method of producing pyrimethamine proved successful in testing reliability, with both steps produced the desired compound for the synthesis. The yields produced by Barker College were either comparable or greater than that of Sydney Grammar, and the 1H NMR of step one revealed a similar purity level in both compounds. This provides evidence that the Sydney Grammar method is reliable, as high yields and purity were able to be obtained in two school laboratories, both produced by high school students, further emphasisng the unneeded expenses and inaccessibility of the drug.
Acknowledgements
I wish to thank Dr Katie Terret for helping me throughout all aspects of my report and my experiment. I would like to thank Kymberley Scroggie for analysing my compounds and providing me with NMR.
References
Ashley, E. and Phyo, A.P. (2018). Drugs in Development for Malaria. pp.861–871.
Bloland, P. (2001). Drug resistance in malaria, pp.1–10, 12–19.
Centres for Disease Control and Prevention (2020). CDC Malaria - FAQs. [online] www.cdc.gov. Available at: https://www.cdc.gov/malaria/about/faqs.html#:~:text=Sympt oms%20of%20malaria%20include%20fever [Accessed 9 Jun. 2021].
Falco,E.A., Goodwin, L.G., Hitchings, G.H.,Rollo, I.M and Russell, P.B. (1951). 2:3-Diaminopyrimidines-A new series of antimalarials. British Journal of Pharmacology and Chemotherapy, [online] 6(2), pp.185–200. Available at:https://bpspubs.onlinelibrary.wiley.com/doi/abs/10.1111/j. 1476-5381.1951.tb00634.x [Accessed 9 Jun. 2021].
Oaks, S., Mitchell, V., Pearson, G. and Carpenter, C. (1991). Malaria: Obstacles and Opportunities. 1st ed. Pollack, A. (2015). Drug Goes From $13.50 a Tablet to $750, Overnight. The New York Times. [online] 20 Sep. Available at: https://www.nytimes.com/2015/09/21/business/a-hugeovernight-increase-in-a-drugs-price-raises-protests.html [Accessed 9 Jun. 2021].
Sydney Grammar School (2016). Consult Terrett. [online] Our Experiment. Available at: https://malaria.ourexperiment.org/daraprim_synthesis/15412/ Third_synthesis_of_24chlorophenyl3oxopentanenitrile_SGS _104.html [Accessed 13 Jun. 2021].
The University of Sydney (2016). Students make $750 drug cheaply with Open Source Malaria team. [online] The University of Sydney. Available at: https://www.sydney.edu.au/news-
opinion/news/2016/11/30/students-make--750-drug-cheaplywith-open-source-malaria-team-.html [Accessed 9 Jun. 2021].
Torsaeter, O. and Abtahi, M. (2003). Experimental Reservoir Engineering Laboratory. Norwegian University of Science and Technology.
World Health Organisation (2019). World Health Organization Model List of Essential Medicines. [online] pp.23–25. Available at: https://apps.who.int/iris/bitstream/handle/10665/325771/WH O-MVP-EMP-IAU-2019.06-eng.pdf [Accessed 9 Jun. 2021]. World Health Organisation (2020). World Malaria Report 2020.
World Health Organisation (2021). Malaria. [online] www.who.int. Available at: https://www.who.int/healthtopics/malaria#tab=tab_2 [Accessed 9 Jun. 2021].