COOLER project gets under the surface of glaciers
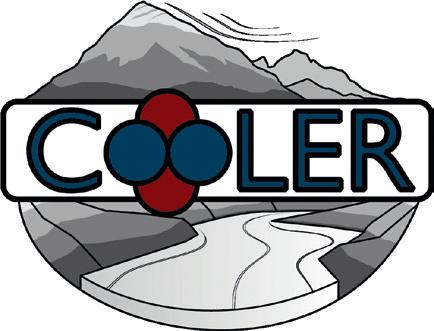
Parts of the earth became glaciated around 5-6 million years ago, and over the last 2-3 million years glaciers have developed more widely, dramatically changing landscapes. Researchers in the COOLER project are investigating the transformation of fluvial landscapes into glacial landscapes, as Professor Peter van der Beek explains.
The earth has been slowly cooling over the Cenozoic era, and parts of the planet have become glaciated over the last 5-6 million years. This cooling has been associated with the development of mountain ranges; the Himalaya for example emerged during the Cenozoic era and have very high erosion rates, which influences the climate. “If rocks erode rapidly they also weather, leading to chemical changes. Weathering consumes CO2 from the atmosphere and brings it into clay minerals, which may be transported to the ocean and deposited there. Rapid erosion of mountains also leads to burial of organic carbon, as trees and plants are stripped off the mountain sides by landslides, transported with the sediments to the sea and deposited before they decompose. It has been suggested that this geological transfer of CO2 from the atmosphere to the lithosphere is the main mechanism by which this very slow, longterm cooling over the Cenozoic era has taken place,” explains Peter van der Beek, Professor of Geology at the University of Potsdam. On the other hand, climate also has an influence on erosion and the way in which it occurs. “If it gets cold enough, we will start to get glacial erosion. How does that influence the landscape? Does it increase erosion rates? Does it change spatial erosion patterns?” asks Professor van der Beek.
Cooler project
These questions are at the core of Professor van der Beek’s work as the Principal Investigator of the ERC-backed Cooler project. In the project Professor van der Beek is analysing apatite minerals gathered mainly from the Alps and Norway, using a technique called thermochronology. “We use this technique to study the cooling of rocks as they are brought to the surface by erosion; since temperature increases deeper into the Earth’s crust (typically by about 20-30 ºC/km), erosional exhumation of rocks leads to cooling,” explains Professor van der Beek. Specifically, the project team is looking at isotopes of helium found within apatite minerals. “Helium-4 (4He) is produced in apatite by the radiogenic decay of uranium and thorium.
The helium-atom is quite small and it moves around in the mineral, so it tends to escape, but the speed at which it does that depends on the temperature,” says Professor van der Beek.
measure ratios than absolute values, so proton irradiation of the samples is being used to induce the homogenous production of helium-3 (3He) before step heating. “In the mass spectrometer we can measure the amount of radiogenic 4He with respect to the amount of induced 3He,” says Professor van der Beek.
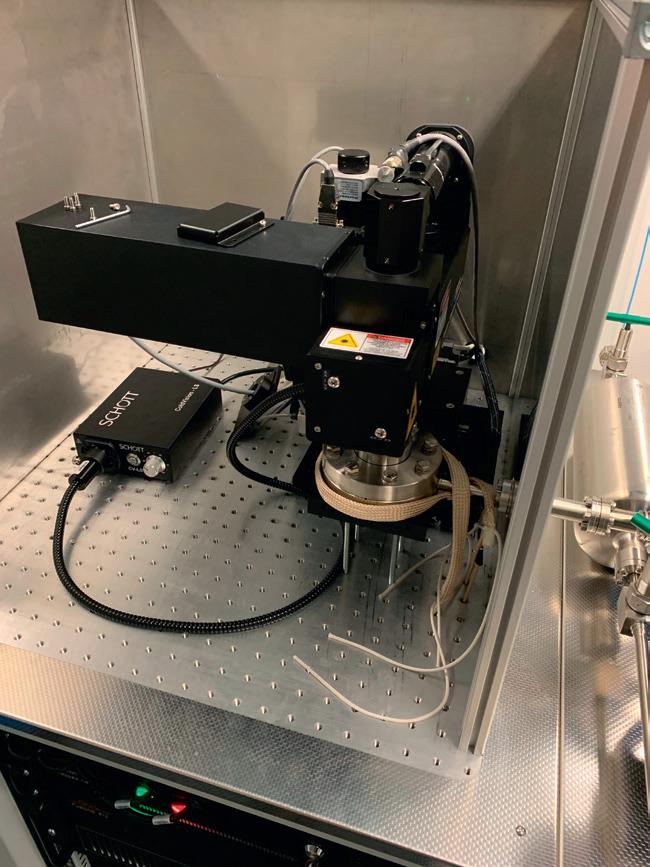
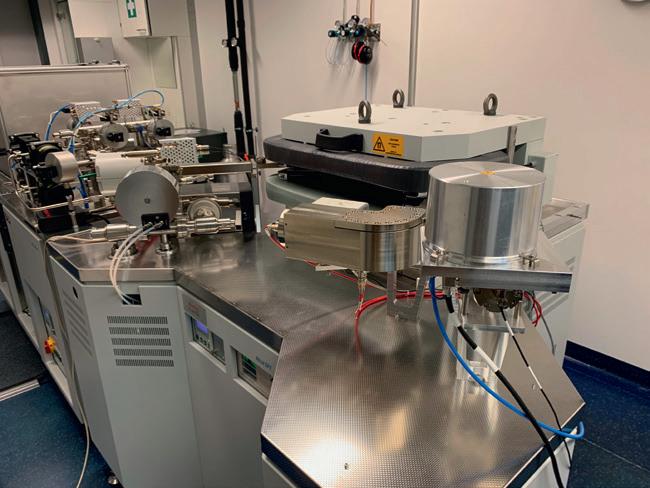
COOLER
Climatic Controls on Erosion Rates and Relief of Mountains Belts
Project Objectives
“Below what we call the closure temperature, this movement of helium in the mineral grain becomes so sluggish that it’s basically trapped – it quantitatively doesn’t escape any more. However, the closure temperature does not
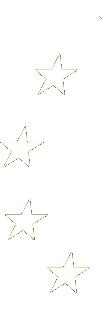
This will provide an insight into the distribution of 4He within the grain, as the 3He is induced homogenously, from which researchers can then learn more about how a grain cooled on its way to the Earth’s surface. For example, if it cooled very quickly, the helium would be expected to be homogenously distributed throughout the grain, whereas there would be a different distribution if it cooled more slowly. “The helium would still have time to diffuse partly out of the grain, and we would expect it to be depleted towards the rims. These are the kinds of things that we can see with this technique,” says Professor van der Beek. Samples are being collected at different
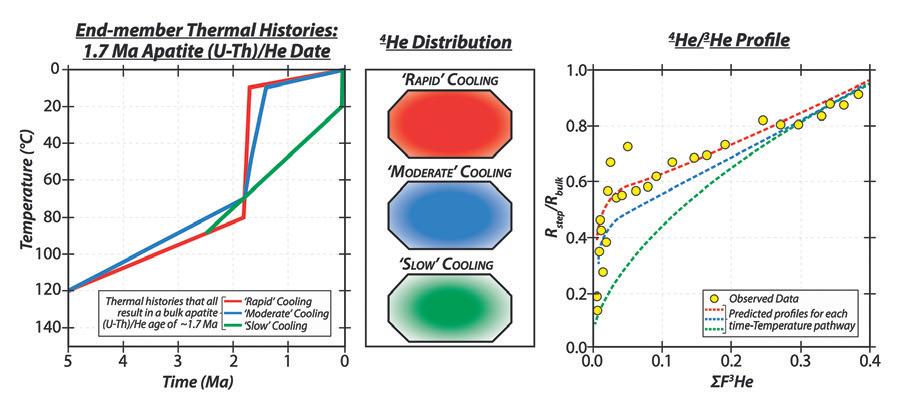
Weathering tends to transfer CO2 from the atmosphere to the lithosphere. It has been suggested that this is the main mechanism by which this very slow, long-term cooling over the Cenozoic era has taken place.
represent an ‘on-off’ switch in the diffusion of helium: it’s essentially a system where the speed at which these atoms move about is exponentially dependent on the temperature.”
The established method of analysing apatite grains helps researchers identify when the mineral crossed the closure temperature. Now Professor van der Beek is developing a new mass spectrometry tool designed to extract more information from a sample, which involves conducting step-heating experiments. “Instead of heating the whole grain all the way up in one go, we go in little steps. For example, we’ll go to 200ºC and stay there for a little while, then go higher in a step-by-step approach, which leads to the step-wise release of the helium,” he outlines. This approach helps to essentially map out where the helium is sitting in the grain. “The first helium that’s released will come from the outside of the grain. Then, as we continue this step heating, we’ll release more and more helium from the internal parts of the grain,” continues Professor van der Beek. It’s much easier on a mass spectrometer to
locations and elevations, from which Professor van der Beek hopes to build a fuller picture of how different landscapes developed. “We take samples along fairly steep profiles, and we also take samples from valley bottoms, to see maybe spatial gradients or spatial changes in erosion history along a single valley. Each sample will give you information at a specific point,” he outlines. “Then we combine these samples, looking to get a more regional overview.”
Numerical models
The plan in the project is to collect samples from the Pyrenees, Andes and Alps, as well as from Scandinavia, with researchers so far focusing on samples taken from Norway and the Alps. The best quality apatite minerals are generally found in granitic rocks, so these tend to be the focus of attention. “We collect samples in the field, crush the rocks, and separate the apatites,” Professor van der Beek outlines. Norway is a stable region where tectonic uplift ceased a long time ago, while Professor van der Beek says uplift rates in
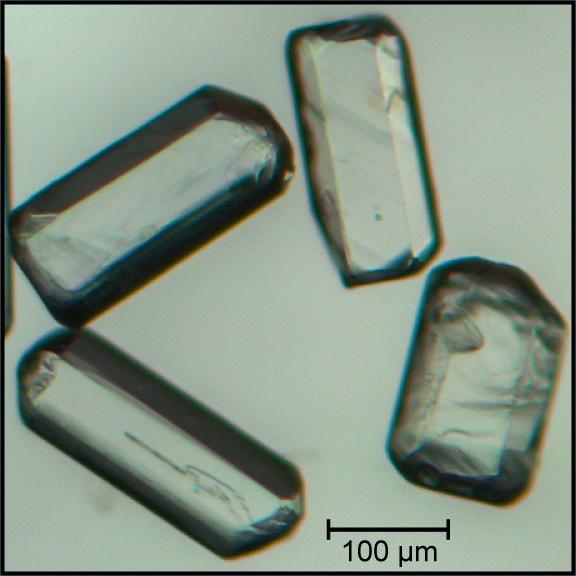
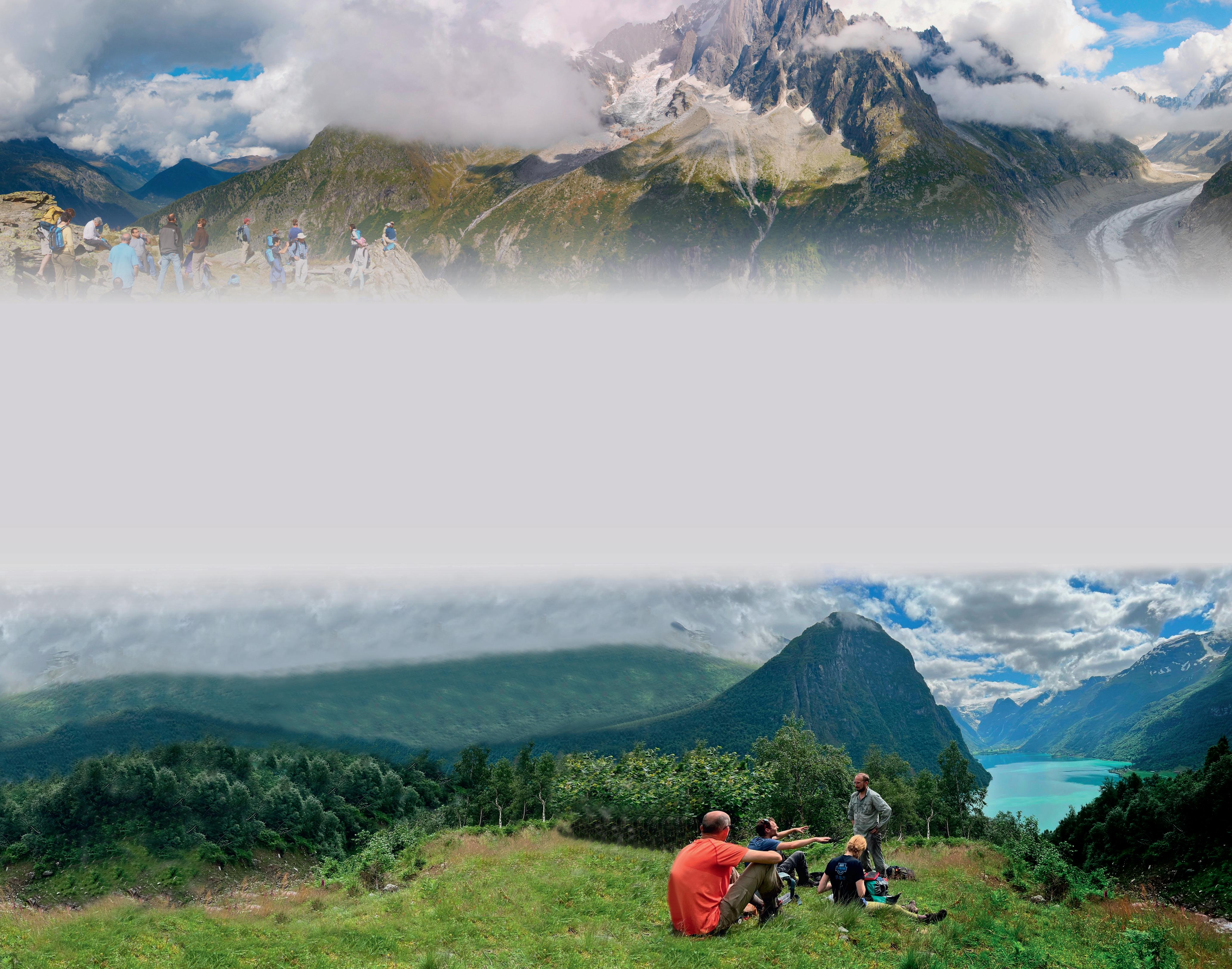
the Alps are variable. “They’re quite high in the Western Alps and lower in the Eastern Alps, so we can contrast them. The Pyrenees are somewhere between the Alps and Norway in terms of tectonic activity, so we can study a gradient in tectonic uplift rates and tectonic activity,” he says. The data gathered in the project will be integrated into two types of numerical model, one of which is designed to predict tectonic and topographic evolution from thermochronology data. “It’s a thermal model, in which a landscape evolves in a prescribed way. What will the thermal structure of the crust underneath that landscape be? If rocks go through that crust as they are exhumed towards the surface, what will their thermal history be?” continues Professor van der Beek. “From that we can then predict for instance the thermochronological age pattern of the rocks, or the spectra of 4He / 3He release.”
A second type of model being used in the project is a landscape evolution model. With this type of model, researchers prescribe glacial and fluvial erosion processes, let them act on the landscape, and then look at how that landscape evolves. “Let’s say we have a mountain range that starts glaciating because of a cooling climate. How is the topography of that mountain range going to change? What will be the effects on the rocks being exhumed towards the surface, and their thermal history, which we can record?” says Professor van der Beek. This research could ultimately lead to a deeper understanding of natural variability in the climate over longer timescales. “We are interested in how these couplings and feedbacks between tectonic processes and the atmosphere might influence the climate. If we can get a better handle on that, then we can look to gain a deeper understanding of natural variability,” continues Professor van der Beek. “However, the
pace of anthropogenic-induced climate change is currently orders of magnitude faster than that caused by natural processes.”
A lot of time has been devoted to setting up the protocol and the techniques, with Professor van der Beek and his colleagues starting to measure samples collected during fieldwork in the Alps and Norway, while work is also ongoing on the numerical models. The wider aim in this research is to build a deeper understanding of glacial erosion. “Does glacial erosion increase erosion rates over geological timescales? Or do we just see this locally and transiently, over relatively short timescales?” asks Professor van der Beek. “When were the Alps glaciated? When did that change from a fluvial to a glacial landscape happen? Did that happen during the very first glaciations or continuously over many glacial-interglacial cycles? These are the kinds of questions we would like to answer.”
The ERC-funded research project COOLER aims to quantify the feedbacks between tectonic processes in the lithosphere and climatic processes in the atmosphere. Advancing our understanding of these couplings requires the development of tools that record erosion rates and relief changes with higher spatial and temporal resolution than the current state-of-the-art, and integrating the newly obtained data into next-generation numerical models that link observed erosion-rate and relief histories to potential driving mechanisms.
Project Funding
This project has received funding from the European Union’s Horizon 2020 research and innovation programme under grant agreement No 834271.
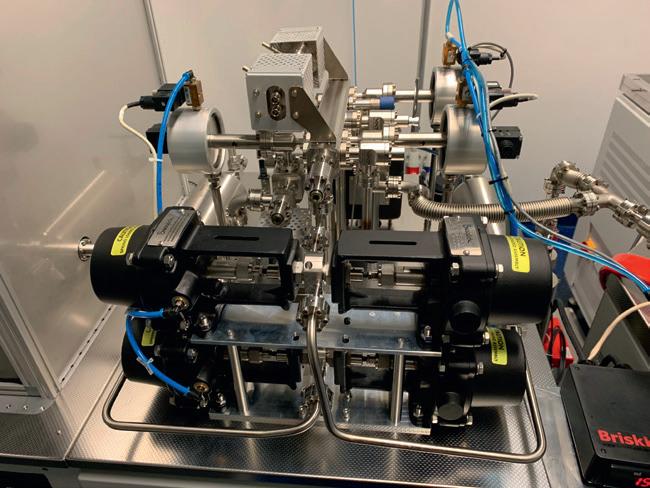
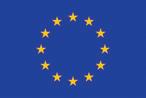

Project Partners
• Helmholtz Zentrum Berlin, Germany
Contact Details
Peter van der Beek
Professor of General Geology
Institut für Geowissenschaften
Universität Potsdam
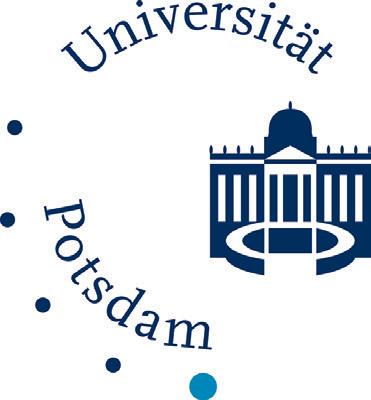
Karl-Liebknecht-Str. 24-25 14476 Potsdam-Golm
Germany
T: +49 331 977 5808
E: vanderbeek@uni-potsdam.de
W: http://erc-cooler.eu
Peter van der Beek is Professor of General Geology at the University of Potsdam, and has held research positions at institutions in Europe, America and Australia. He has been Chair of the Programme Committee of the European Geosciences Union.
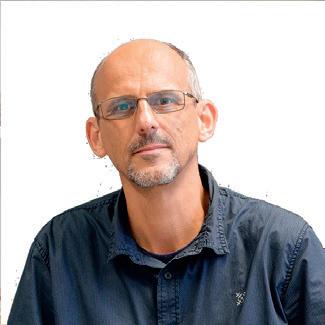