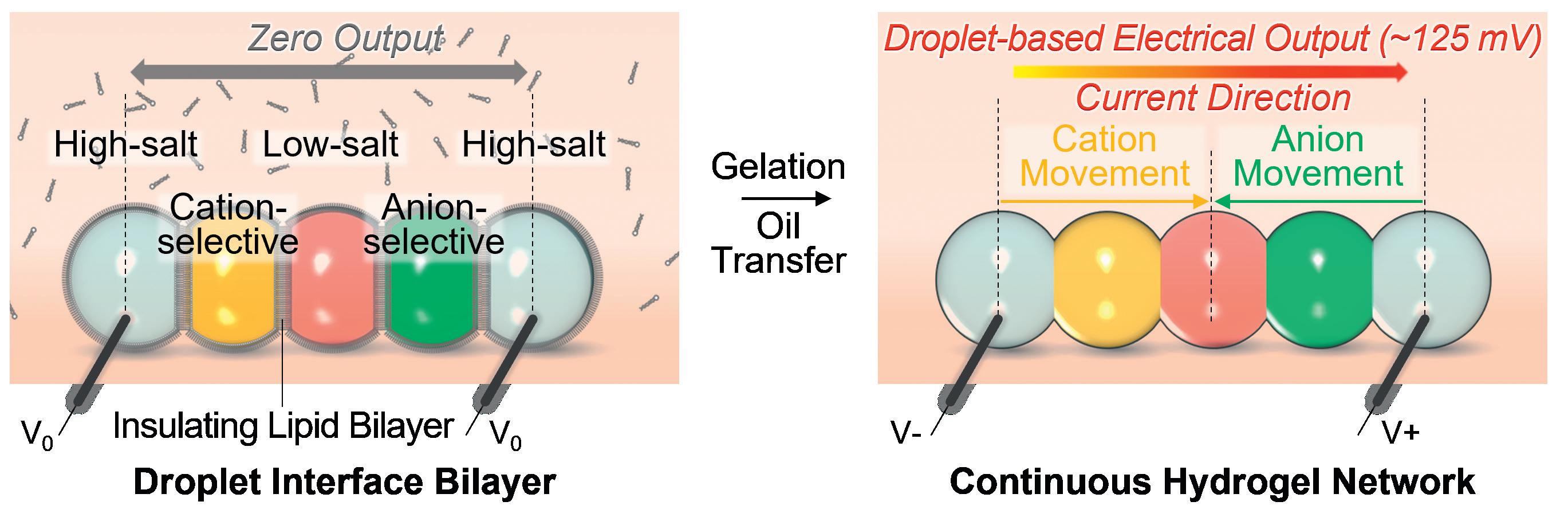
3D-Printed synthetic tissues that can be remotely controlled
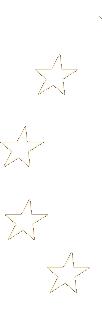
Many of the technologies currently used to provide power to bio-integrated devices rely on electronic components and circuits, so are bulky and rigid. We spoke to Professor Hagan Bayley, Dr Yujia Zhang and Dr Linna Zhou about their work in developing a miniaturised soft ionic power source and its wider potential in modulating the activities of cells and tissues.
Reliable sources of power are required for the operation of bio-integrated materials and devices, such as the tissue implants that are under development for the repair of damaged organs. Many of the technologies currently used to power these biomaterials have significant shortcomings, says Dr Yujia Zhang, a post-doctoral researcher in Professor Hagan Bayley’s group at the University of Oxford. “For example, lithium batteries are bulky and the materials used to construct them are not soft,” he explains. As part of his work in the EU-funded SYNTISU project, Dr Zhang is working to develop a new type of power source that overcomes these issues. “We are developing tiny, soft, chains of nanolitre (hundreds of micrometres in diameter) droplets comprising conductive hydrogels,” he says. “For example, our five-droplet system contains charge-selective polymers and salts at different concentrations. This allows the cations and anions – positively-charged and negatively-charged ions – to move through the conductive hydrogel in opposite directions, from the high-salt droplets at the two ends to the low-salt droplet in the middle, generating an ionic current and a power output.”
Droplet battery
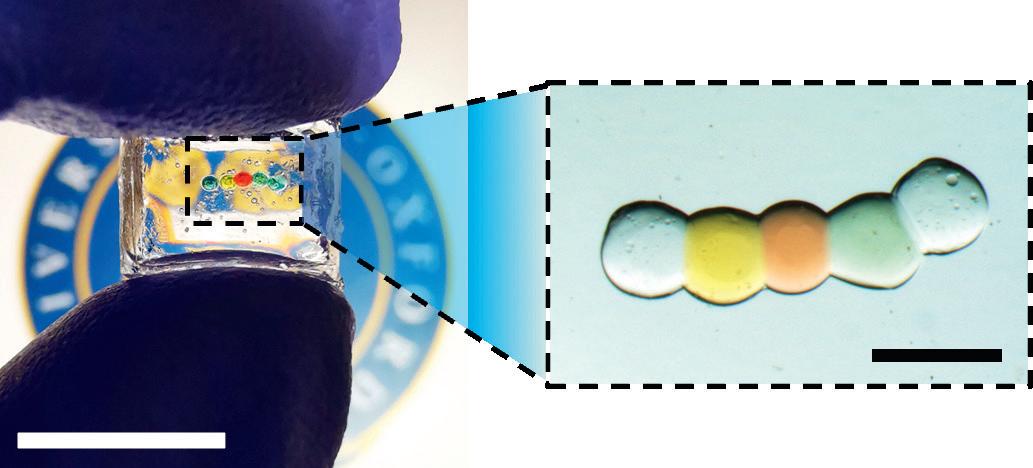
This research builds on earlier work in the Bayley group on the development of synthetic tissues, which require an external input to induce directional ion movements, enabling ionic communication between compartments, like cell-cell communications in natural tissue. In contrast to external light and wired-electrode inputs, the droplet battery provides an internal power source that meets this need for the first time. “These batteries are intended to move ions around; they are iontronic power sources,” notes Professor Bayley. Different approaches can be used to fabricate the battery. “In early work, we manually deposited each droplet to fabricate a single unit of five droplets, but we can also use 3D printing to achieve large-scale
fabrication,” outlines Dr Zhang. “For droplet chains containing hundreds of droplets, we use a mould to hold them in position. When a battery is fabricated, the droplets are in a pregel state and are separated by lipid bilayers. The battery is activated by gelation of the droplets and removal of the lipid bilayers to form a continuous hydrogel network.”
The ions can then flow through the network, and a variety of salts have been investigated in the project as a means of producing an internal energy gradient. Each salt combination produces a different output voltage, which in turn can be used to produce external ion movement in a
connected material or device. After detailed investigation, Dr Zhang decided to use calcium as the main cation in the droplet power source. “We are using calcium to create the energy gradient due to its high output performance,” he says. The battery is versatile however, so it’s not limited to a single type of salt. “We can use either organic or inorganic salts – as long as they are water soluble,” continues Dr Zhang.
A lot of attention in the project has been focused on maximising the output of the power source, by modifying the concentration gradient to achieve an optimal outcome. There is a trade-off here between the salt gradient and internal resistance. “If we increase the difference between the high-salt concentration and the low-salt concentration, we will have a higher output. But on the other hand, reducing the low-salt concentration to increase the salt gradient can also increase the internal resistance in the system, which will reduce the overall current,” explains Dr
listed in right column) and correct the rhythm of a mouse heart in unpublished work. The batteries — combined with living cells or with hybrid synthetic/living tissues — might eventually be key components in organ repair,” he notes. The droplet battery can also be adapted for different situations. “The size, shape and output of the droplet battery can be modified according to the needs of individual patients, reflecting the nature of an injury,” explains Dr Linna Zhou, an Investigator in the Department of Medicine at Oxford.
Modulating the activity of 3D brain tissues
A further application of these droplet power sources is in modulating the activity of 3D constructs comprising human neural cells that mimic aspects of brain activity. Dr Zhang says the power source has been tested in this area. “We have already shown that we can use the droplet power source to modulate neuronal activity, for example by stimulating intracellular calcium waves,” he points out.
“We are developing tiny, soft, chains of nanolitre (hundreds of micrometres in diameter) droplets comprising conductive hydrogels. Our five-droplet system contains charge-selective polymers and salts at different concentrations. This allows the cations and anions to move through the conductive hydrogel in opposite directions, from the high-salt droplets at the two ends to the low-salt droplet in the middle, forming an ionic current to power synthetic tissues or modulate the activity of living tissues.”
Zhang, who feels that a good balance has been found. Further effort is being devoted to controlling the battery output. “We want to be able to turn on the output when we want to, and we also want these batteries to last for a long time,” adds Professor Bayley.
In the study, the device could stably store its energy in the off-state for 36 hours. After being triggered to the on-state, the activated droplet power source produced a current which persisted for 30 to 120 minutes. The maximum output power of a unit made of of 5 50-nanolitre droplets was around 65 nanowatts.
At the present stage of development, the soft battery has sufficient output to be used as a power source for synthetic tissues. For example, it can induce the movement of charged molecules within a tissue. Professor Bayley says it also holds wider potential for applications with living tissues. “Indeed, these very tiny, microscale, soft batteries have been used to modulate neuronal activity as demonstrated in a published paper (Ref 1
The next step could be to commercialise this research and refine the ideas further outside an academic environment, while Dr Zhang is also keen to explore the wider possibilities of droplet-based iontronic networks. “Following this work, we have been developing other means to generate power, including lithium-ion batteries and enzymemediated biobatteries, both based on microscale, soft droplets,” he says. In addition to the potential of these droplet networks as energy sources, Dr Zhang envisages the use of hydrogel droplets as a platform for other applications. “We can put not only energy components into these droplets, but also integrate other functional components, such as stimulus-responsive nanoparticles, enabling light and magnetic responsiveness,” he continues. “We aim to develop dropletbased systems with a variety of functions in the future, including logic circuits, sensors, microrobots, and drug delivery pumps. Most importantly, these ion-based systems will be small, soft and biocompatible.” www.euresearcher.com
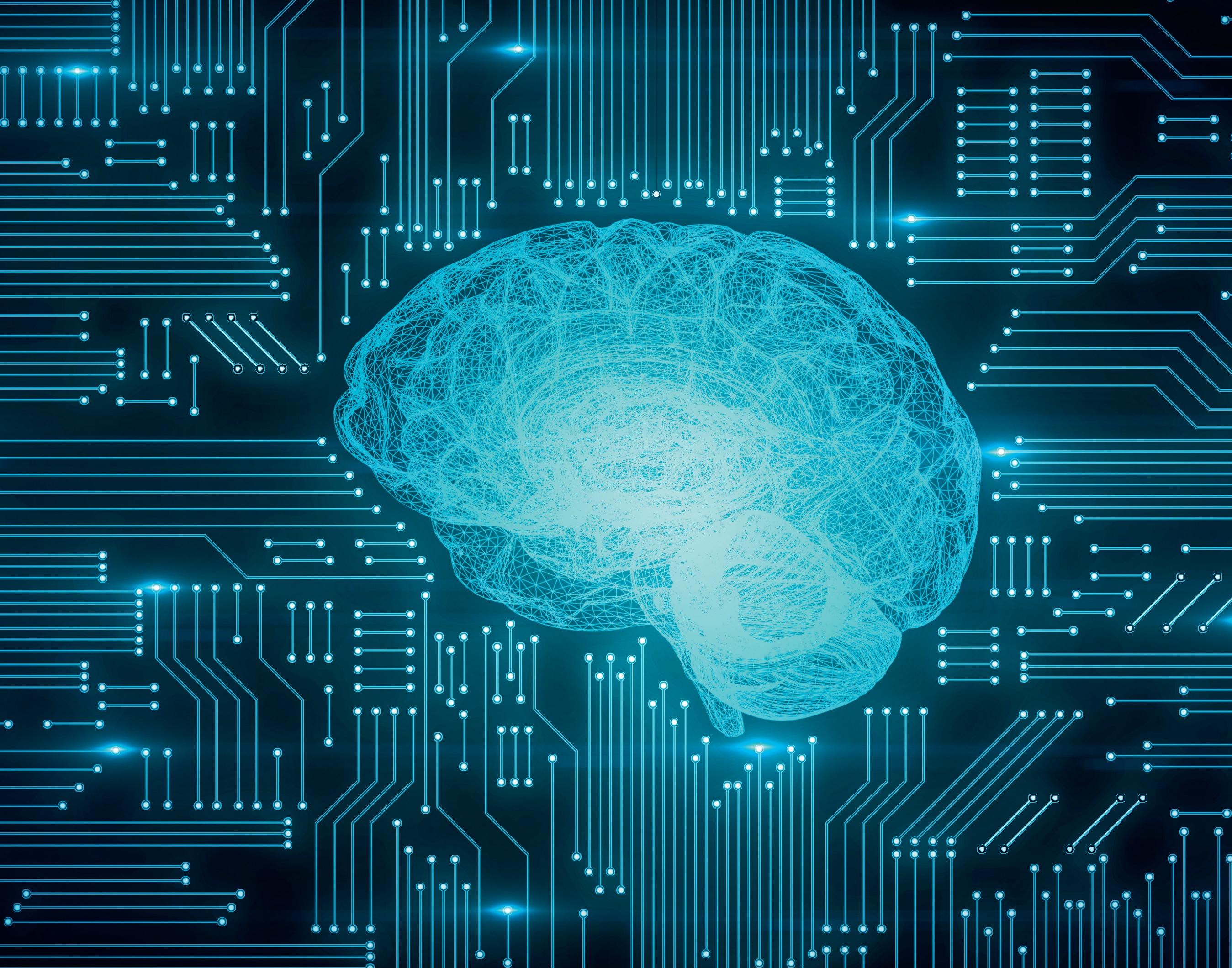
SYntiSu
3D-printed synthetic tissues will be remotely controlled to modulate cellular functions
Project Objectives
The majority of neurological injuries and neurodegenerative diseases are currently untreatable with devastating health consequences and substantial financial and societal burdens. Based on the technologies developed under our ERC Advanced Grant SYNTISU and a previous PoC grant BIOELECTRIC, the SYMPLANT project aims to produce synthetic neural implants (SNIs) as a potential new treatment for patients. The SNIs will contain both neurons and iontronic components, such as the batteries described here, in constructs that will replace damaged tissue.
Project Funding
This project has received funding from the European Union’s Horizon 2020 research and innovation programme under the Grant Agreement No. 101081795.
Contact Details

Project Coordinator, Professor Hagan Bayley FRS Professor of Chemical Biology University of Oxford 12 Mansfield Road, Oxford, OX1 3TA t: +44 01865285100
E: hagan.bayley@chem.ox.ac.uk
W: https://www.bayleygroup.co.uk/
1: Zhang, Y., Riexinger, J., Yang, X. et al. A microscale soft ionic power source modulates neuronal network activity. Nature 620, 1001–1006 (2023).
2: Jin, Yongcheng, et al. “Integration of 3D-printed cerebral cortical tissue into an ex vivo lesioned brain slice.” Nature Communications 14, 5986 (2023).
3: Zhou, Linna, et al. “Lipid-bilayer-supported 3D printing of human cerebral cortex cells reveals developmental interactions.” Advanced Materials 32, 2002183 (2020).
Professor Hagan Bayley
Dr Yujia Zhang
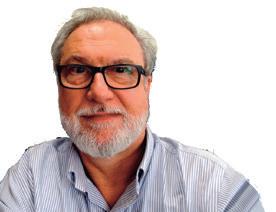
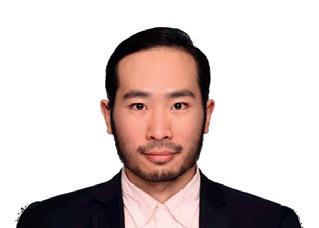
Dr Linna Zhou
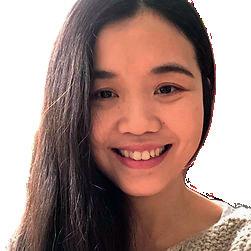
Hagan Bayley is the Professor of Chemical Biology at the University of Oxford. He was the 2009 Chemistry World Entrepreneur of the Year and was elected a fellow of the Royal Society in 2011. His lab has developed techniques for the fabrication of 3D tissues, both living and synthetic.
Dr Yujia Zhang is a postdoctoral research associate in the Bayley group at the University of Oxford. He received the Chinese Academy of Sciences Presidential Scholarship (Special Prize) in 2020 and was selected by the UK Parliamentary & Scientific Committee’s STEM for BRITAIN as a representative early-career scientist in 2023. His work focuses on the development of multifunctional iontronic synthetic tissues for biotic interfaces and soft implants.
Dr Linna Zhou is in the Nuffield Department of Medicine and the Department of Chemistry at the University of Oxford. She is an investigator and fellow of the Oxford Martin School. Her current research uses stem cell and bioengineering techniques to build 3D tissues.
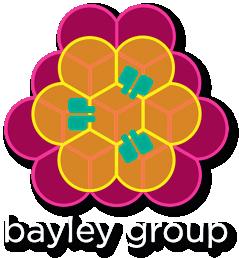
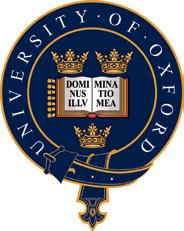