OF UNDERGRADUATE SCIENCE AND TECHNOLOGY
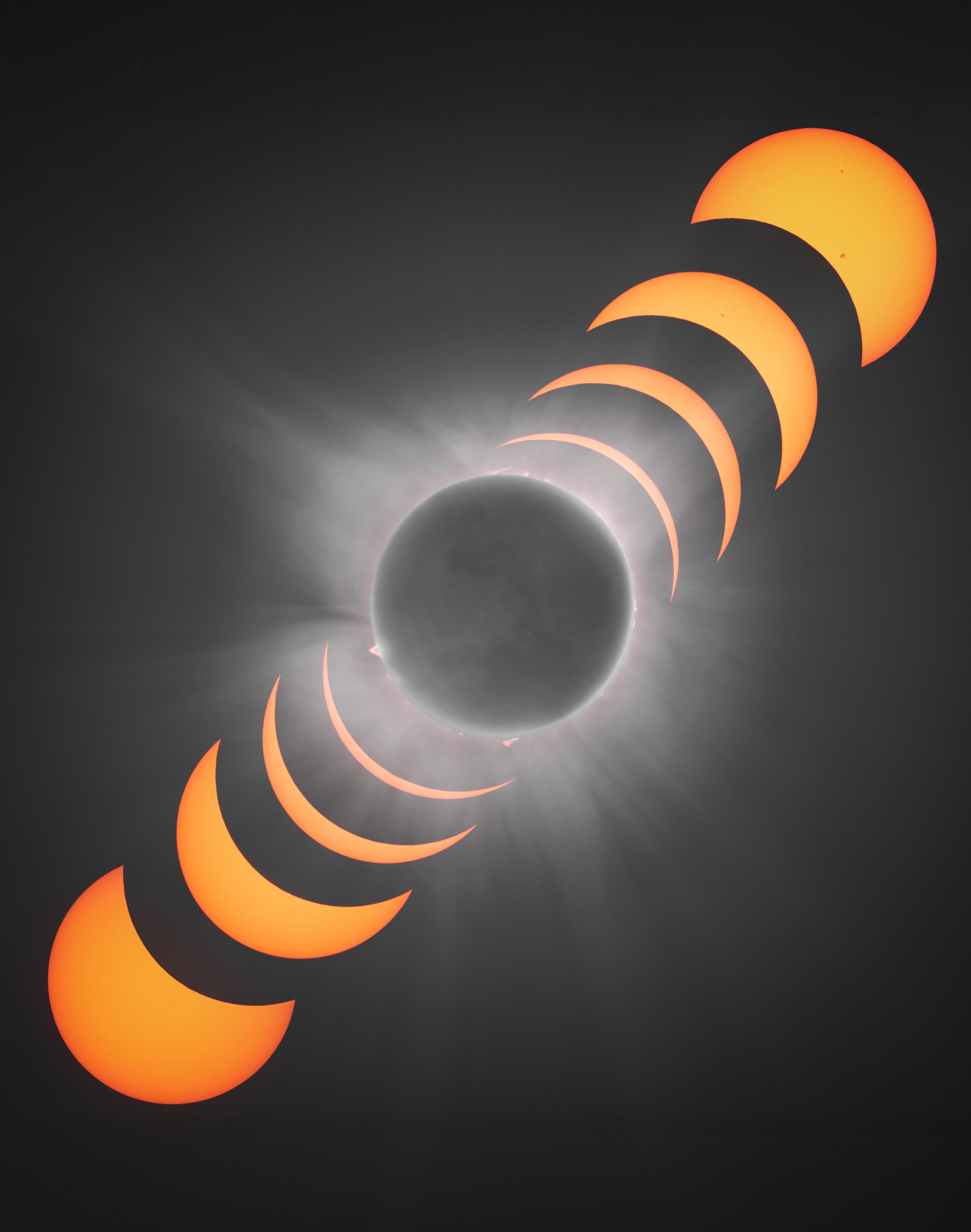
OF UNDERGRADUATE SCIENCE AND TECHNOLOGY
A COLLECTION OF PHOTOGRAPHS OF THE 2024 ECLIPSE PAGE 16
in Universal Flu Vaccine Development: The Multivariant mRNA vaccine PAGE 10 Sowing Roots of Conservation: UW Madison’s Environmentalist Heritage PAGE 12 Antibiotic Pollution: An Ecological Problem PAGE 22
Dear Readers,
It is with great pleasure that we unveil Volume IX, Issue II of the Journal of Undergraduate Science and Technology (JUST). Founded upon the common goal of supporting and amplifying undergraduate voices, our journal aims to showcase some of the current and upcoming research and advancements within the world of science. JUST is not simply a journal but a hub for undergraduates who want to share their scientific voice – whether through research, writing, editing, or design – our journal is a space for all. We have the unique ability to provide hands-on training to undergraduate students, giving them a chance to publish research in a peer-reviewed journal and expose members to the academic publication process. Such experiences are vital in setting students up for success, and we are profoundly grateful to be able to play a part in the larger effort of making scientific writing and research available to those beyond academia.
I would like to take a moment to recognize all the work done by our extraordinary team behind the publication. Our writers, editors, designers, marketing, finance, and web design teams worked diligently over the past semester to transform knowledge into the pages you now hold in your hands while balancing lives as students and individuals. A special thank you to our advisors Dr. Todd Newman and Dr. Joan Jorgenson for all their help over the last year, without which we would be unable to publish such high-quality pieces. Lastly, I would like to take a second to recognize the Associated Students of Madison and the College of Agriculture and Life Sciences for their unwavering support of undergraduate science journalism.
This issue will mark my sixth and final semester working amongst such talented individuals. Having started my undergraduate career as an inexperienced editor who didn’t know how to make a simple comment on an editorial to finishing up my degree as editor-in-chief, I am truly grateful for how much this journal has taught me. Not only does JUST foster academic confidence in its members, but I have found that I have learned a new way to communicate and inspire readers to learn more in the process. I have come to recognize that science literacy is not simply about how many complicated academic papers one can read but rather that you must have the inspiration to want to learn about a topic for the content to truly make sense. JUST aims to provide a platform for readers to do just that!
As you delve into the pieces that follow, remember that science is all around us from the water we drink to the stars above us. I hope that the following editorials, manuscripts, and photography inspire you to keep discovering the beauty of our world. Even if you haven’t always felt drawn to scientific subjects, I hope we can ignite your inner scientist and show you the wonder hidden in everyday life. Have fun on your adventure through our spring 2024 journal!
Sincerely,
who we are
The Journal of Undergraduate Science and Technology (JUST) is an interdisciplinary journal for the publication and dissemination of undergraduate research conducted at the University of Wisconsin-Madison. Encompassing all areas of research in science and technology, JUST aims to provide an openaccess platform for undergraduates to share their research with the university and the Madison community at large.
Submit your research below to be featured in an upcoming issue!
EDITOR-IN-CHIEF
Manasi Simhan
MANAGING EDITORs
Dima Hamdan
Trang Pham
Adina Shaikh
HEAD WRITERs
Amy Li
Natalie Martinson
DESIGN Director
Quinn Ruzicka
Head Web Developer
Shreyanshu Dekate
Director of Finance
Dev Chatterjee
design assistant
Olive Haller
Web assistant
Sophie Liu
Marketing Assistant
Alexa Dreyfus
CONTENT Editors
LeAnn Aschkar
Analise Coon
Carmela De Leon
Walid Lakdari
Ivy Raya
Mackenzie Twomey
STAFF WRITERS
Manal Aditi
Kaashvi Agarwwal
Zane Brinnington
Khadijah Dhoondia
Daniel Molina
Adrian Sieck
Vedaa Vandavas
Alex Wong
Short-Form Editors
Ayah Amer Dannah Altiti
Harvey Chen
short-Form Writers
Daniel Del Carpio
Sean Hugelmeyer
Mahathi Karthikeyan
Contributing Writers
Nedda Besharat
Mary Hegeman
Alex Walsh
Contributing
Researchers
Jessica Randall
Pragati Maheshwary
We would like to sincerely thank the College of Agriculture and Life Sciences [CALS], the Wisconsin Institute for Discovery, the Associated Students of Madison (ASM), and the Wisconsin Alumni Research Foundation for financially supporting the production of JUST’s Fall 2023 issue.
Thank you!
Manal
Five Decades Since the Last Moon Landing; How Does NASA’s Artemis Program Compare to Apollo 17?
Zane Brinnington
Kaashvi Agarwwal
After the irreparable damage caused by the COVID-19 pandemic, the demand for faster and more efficient technology has increased tenfold. As demonstrated in 2019, artificial intelligence has undoubtedly affected our world with varying degrees of success. Although artificial intelligence (AI) helped in the Pfizer vaccination trials, it failed to live up to its potential in numerous ways (Castellanos, 2021). AI was thought to be the solution to finding COVID-19 symptoms, with its ability to predict and analyze, which is believed to be a critical tool for efficiency during this race against time. However, it’s prophecy was left unfulfilled as the chaos during the pandemic became inescapable. With newer models developing daily, can AI be a significantly beneficial tool for a future pandemic? If we are to address biases in AI, collection of data, and increase international cooperation, we may find our key solution to saving ourselves.
History of AI during COVID-19
COVID-19 is the most recent pandemic the world has experienced, with devastating consequences. This pandemic had a mortality rate far lower than other contenders, such as the H1N1 influenza A virus or the Spanish flu. What made COVID-19 so potent was its rate of infection and its ability to form new variants faster than previous strains. Since COVID-19 is highly contagious, preventing its spread is of utmost importance. However, the consequences did not need to have been this bad. Within the United States, 52% to 92% of COVID-19 deaths could have been avoided with better management (Syrowatka et al., 2021). To combat the spread, public policy officials and politicians established masking requirements, travel restrictions, and the closing of businesses, workspaces, schools, etc. Most recommendations were made using expert testimony rather than real-time data in analysis; had there been access to data-driven models, it would have helped balance out the given response. Data-driven models have a greater capacity to store incoming pandemic and patient data compared to policymakers and experts, as well as the flexibility to adapt to a given dilemma due to their ability to analyze large datasets and give much-needed predictions regarding the future and solutions to help in the present (Syrowatka et al., 2021). Doing so would have ensured the economy could run smoothly and that social structures could have been left in place while maintaining citizens’ health and well-being, which is a delicate balance. Most of the AI models created during the pandemic were entirely
unusable. In a Highest Attainable Standard of Evidence (HASTE) comprehensive systematic review by Wynants et al., more than 100 COVID-19 prediction models were examined for diagnosis and prognosis, resulting in the proposed AI systems being highly liable to statistical bias. They also lacked meticulous reporting and portrayed an overly optimistic outlook. Given these findings, the authors have taken precautions against using any of the models in medical practice. Many of the AI models have since been repurposed for public health, from a tool for United States prisons to calculate repeated offences of inmates to the purpose of determining home confinements. The present racial biases within the correctional facility tool were on full display, as the AI models sentenced Black inmates at higher rates to longer stays within the prisons, risking COVID-19 exposure and, consequently, death (Leslie et al., 2021). Biased AI models certainly created significant risks for citizens during the pandemic.
Another significant issue during the pandemic was the disparities in data governance policies, such as data localization laws, lack of international consensus on data sharing in healthcare, and the prevention of the complete collection of data essential for running effective AI models for COVID-19. The differences in data governance policies significantly impeded cooperative efforts in pandemic management. Disparities between developed and developing nations in sharing healthcare data highlight concerns regarding fairness, credit features, and the fair allocation of benefits from data-sharing initiatives (Chakravorti, 2022). The historical and sociopolitical landscape is tailored to the countries’ data-sharing perspectives which, in turn, influences the acceptance of invasive measures like AI-aided exposure identification and contact tracing apps. While some countries, like South Korea, have embraced extensive data collection due to past experiences with outbreaks, other countries, like Germany, have rejected centralized approaches due to strong historical skepticism of state surveillance. There is also substantial variability in patient data between countries, as factors such as race, demographics, socioeconomic status, healthcare quality, and underlying health conditions vary from country to country, which limits extrapolating predictions.
Dangers of Biased AI Datasets
Artificial intelligence databases function as warehouses of
healthcare information, with information such as patient data and treatment plans. These databases are used to train the AI model to find patterns, detect symptoms, and create treatment plans, which means that poor-quality databases worsen patients’ quality of care because of intrinsic bias. The lack of oversight on AI databases has had devastating impacts on the reach of medicine and increased disparities in healthcare towards minorities. Health inequality comes in many forms, like inconsistent treatment due to the lack of access to healthcare. In the long run, this can result in disproportionate levels of immune vulnerability due to higher levels of chronic stress, overcrowding, and “weathering,” a condition of premature aging and decay from continuous stress (Leslie et al., 2021). The consequences of overlooking such algorithmic biases in datasets increase the pandemic’s effects on minorities. The body of evidence supporting these findings is swiftly expanding: COVID-19 mortality rates are over twice as high among residents of more deprived areas. Additionally, Black, Asian, and ethnic minority individuals in Britain face up to double the risk of death if infected compared to their white counterparts. Even after adjusting for age, the risk of death for Black men and women is more than four times that of white men and women (Leslie et al., 2021). Artificial intelligence is only as good as the data it is trained upon. With clear and present bias within healthcare and its professionals, change must be implemented in future AI models.
One must question how the bias of the data models seep into the healthcare that citizens receive. First, data taken from healthcare professionals can intrinsically be biased from the data samples and people’s preconceived biases. Vulnerable groups often lack access to healthcare and live in
areas where there is an inherent lack of resources. Compounding this issue are the discriminatory healthcare practices that are still in place, with biased stereotypes taken as fact by many healthcare professionals. One example of a prevalent bias is the physician rating of Black patients as less intelligent and less likely to complete cardiac rehabilitation. These stereotypes lead to Black patients having a lower likelihood than white patients to receive cardiac medication or undergo coronary artery bypass surgery, even when accounting for factors such as age, income, and other medical conditions. Stereotypes have also led to Black patients being less likely to be seen than white patients in emergency rooms (Smedley et al., 2003). With this bias inherent to the data that healthcare professionals input, it is crucial to consider the ramifications of the biased treatments of the future applications of AI models in pandemics.
These inequalities result in discriminatory data and sampling bias, leading to datasets that lack an accurate representation of the people. Without data representativeness, biases can lead to the divergent performance of the AI models in diagnosing and creating plans for medical problems. Biased data includes written notes, health records, training curriculums, clinical trials, etc., in which Natural Language Processing models can be biased from stereotypes, from racism to homophobia (Leslie et al., 2021). All of the biased data combine into problems for AI model diagnosis, as these models are then focused on identifying symptoms of pandemic diseases. The patterns will start applying bias in the solutions they provide to vulnerable groups, exacerbating the health inequalities that persist in our society today.
Health Inequality Effects Increasing From AI (Source: The British Medical Journal)
There is huge potential for AI, but first, we must look into the future of how it can be used once we address the bias and the issues with collaborative global health data usage. The utilization of AI in pandemic research and response holds immense promise for revolutionizing our approach to future global health crises. Recent advancements, such as the EVEscape tool developed by researchers at Harvard Medical School and the University of Oxford, showcase the potential of AI in predicting viral variants with remarkable accuracy (Chakravorti, 2022). This predictive capability not only aids in developing vaccines targeted towards specific variants but also facilitates the creation of tailored treatments for individuals based on the predicted variant they are likely to contract. Moreover, AI-driven insights enable more precise and transparent communication with the public regarding the risks associated with emerging variants, thereby alleviating anxiety and fostering trust in healthcare authorities.
“ we must look into the future... once we address the bias and the issues with collaborative global health data usage
”
Furthermore, AI tools like EVEscape empower public health researchers and policymakers to monitor viral mutations proactively in real-time, enabling swift responses to potential threats (Caruso, 2023). This tool can also be used to make predictions regarding new unknown variants of SARS-Cov-2 before they emerge. If EVEscape had been used at the beginning of the COVID-19 pandemic, it could have been used predict its most frequent mutations and worrying variants (Caruso, 2023). How its first model, EVE, was developed is key to understanding its success. EVE was first created as a generative model
that used evolutionary data across species on a large scale to determine protein functionality (Caruso, 2023). When the pandemic hit, EVE was rebuilt with biological and structural details of the virus that could be adapted to detect this specific virus. Although EVEscape was used to determine variants of SARS-Cov-2 and not to diagnose patients like other AI models discussed above, there are essential factors of EVEscape that made it successful. Firstly, using diverse datasets in its first model of EVE was essential for providing a comprehensive and more accurate analysis when used by AI models. Secondly, unlike other AI models adapted from sources that were as disconnected as prison tools, EVEscape was directly formed from EVE. It served a similar functionality that could translate directly to the new task it was given in this pandemic. These factors are key to resolving some of the essential factors that limited AI models used during the pandemic, providing hope for future AI models.
Despite the failures of AI in the past pandemic, there are valuable lessons to be learned, especially looking forward to the future of AI in pandemic preparedness and reaction. Several key points are for improving the assembly of comprehensive datasets, fostering diversity in data sources, and establishing more cohesive international rules for data sharing, which are crucial steps in improving the effectiveness of AI systems. By accelerating the development of vaccines and treatments, AI promotes greater acceptance of preventative measures such as vaccination for the overall picture of health campaigns. Embracing AI technologies in pandemic preparedness and response efforts is paramount in addressing the evolving challenges posed by future global health crises, offering a promising pathway towards more effective and timely interventions to safeguard public health globally. Once we start addressing these challenges, AI has the potential to play a pivotal role in creating a helpful address for the impact of future pandemics.
Works Cited
Caruso, C. (2023, October 11). An AI Tool That Can Help Forecast Viral Outbreaks | Harvard Medical School. Hms.harvard. edu; Harvard Medical School. https://hms.harvard.edu/news/ ai-tool-can-help-forecast-viral-outbreaks
Castellanos, S. (2021, April 1). How AI Played a Role in Pfizer’s Covid-19 Vaccine Rollout. Wall Street Journal. https://www. wsj.com/articles/how-ai-played-a-role-in-pfizers-covid-19vaccine-rollout-11617313126
Chakravorti, B. (2022, March 17). Why AI Failed to Live Up to Its Potential During the Pandemic. Harvard Business Review. https://hbr.org/2022/03/why-aifailed-to-live-up-to-its-potential-during-the-pandemic
Leslie, D., Mazumder, A., Peppin, A., Wolters, M. K., & Hagerty, A. (2021). Does “AI” stand for augmenting inequality in the era of covid-19 healthcare?. BMJ, 372(372), n304. https://doi.org/10.1136/bmj.n304
Smedley, B. D., Stith, A. Y., & Nelson, A. R. (2003). Unequal Treatment. National Academies Press.
https://doi.org/10.17226/12875
Syrowatka, A., Kuznetsova, M., Alsubai, A., Beckman, A. L., Bain, P. A., Craig, K. J. T., Hu, J., Jackson, G. P., Rhee, K., & Bates, D. W. (2021). Leveraging artificial intelligence for pandemic preparedness and response: a scoping review to identify key use cases. Npj Digital Medicine, 4(1), 1–14. https://doi.org/10.1038/s41746-021-00459-8
Watson, I., & Jeong, S. (2020, February 28). Coronavirus mobile apps are surging in popularity in South Korea. CNN; CNN. https://edition.cnn.com/2020/02/28/tech/korea-coronavirus-tracking-apps/index.html
Wynants, L., Van Calster, B., Bonten, M. M. J., Collins, G. S., Debray, T. P. A., De Vos, M., Haller, M. C., Heinze, G., Moons, K. G. M., Riley, R. D., Schuit, E., Smits, L. J. M., Snell, K. I. E., Steyerberg, E. W., Wallisch, C., & van Smeden, M. (2020). Prediction models for diagnosis and prognosis of covid-19 infection: systematic review and critical appraisal. BMJ, 369(8242), m1328. https://doi.org/10.1136/bmj. m1328
Humans took their first steps on the Moon on July 21, 1969 as the climax of the Apollo 11 mission, and have since returned five times. The last was the result of the Apollo 17 mission in December 1972, with mission commander Eugene A. Cernan taking a moment before his departure to gaze across the lunar surface and declare that they “shall return, with peace and hope for all mankind” (Lewis, 2024). In the 52 years since, nobody has again voyaged to the moon to fulfill his promise. In the next few years, NASA plans to change that.
Mankind’s peaceful and hopeful return to the lunar surface will be the result of NASA’s Artemis program. First announced in 2017, Artemis currently exists as a three-parted attempt to develop new technology capable of bringing humans back to the moon more efficiently than ever. Artemis I (the first part) took place in November of 2022 with an uncrewed flight test of the Orion spacecraft to the Moon’s orbit and back. Since this preliminary mission was successful, Artemis II is on track for September of 2025, where four astronauts will voyage to space on the Orion. Provided that this mission also goes according to plan, Artemis III will occur the following year, with the same astronauts using the Orion to conduct the first lunar landing of the 21st century. These astronauts are planned to continue the program well after the completion of Artemis III by making annual returns to its surface in the years that follow (NASA, n.d.). So, what exactly will the Artemis III mission look like? More specifically, what has changed in the five decades since our last visit to the moon?
There were three astronauts, each having different roles, who made the voyage to the moon in the Apollo 17 mission. The one responsible for piloting the command module to the Moon’s orbit and back to Earth was Ronald E. Evans, an engineer, navy aviator, and former member of the support crew on the Apollo 7 and 11 missions. Geologist (and future UW-Madison professor) Harrison H. Schmitt was the pilot of the lunar module from the moon’s orbit to its surface, and the first ever scientist-astronaut to walk on it. The mission commander was the aforementioned Cernan, who had been to space twice previously as a crew member of the Gemini IX and Apollo 10 missions. Cernan announced before boarding the Saturn V that the crew be-
lieved “very strongly that this flight belongs to [all Americans]” (Uri, 2021).
“
There were three astronauts, each having differnet roles, who made the voyage to the moon in the Apollo 17 mission.
”
While the qualifications and abilities of these three astronauts is of no question, they were, as all who have ever been to the moon, white men; for the Artemis mission, NASA is bringing Cernan’s sentiment closer to reality by making its crew of four more representative of all Americans. Mission specialist Christina Koch had been a flight engineer on the International Space Station for three expeditions and had spent 328 days in space, the longest of any female astronaut in history (NASA, 2023). She will be the first woman to ever walk on the Moon. The mission’s pilot, Victor J Glover, also had been an engineer on the ISS as well as a pilot on NASA’s Space X crew 1. An African-American man, Glover will be the first person of color to ever walk on the Moon (NASA, 2023). They will be joined by Canadian mission specialist Jeremy Hansen, a former mission control capcom, fighter pilot, and teacher of NASA’s Astronaut classes (Gov. of Canada, n.d.), as well as mission commander Reid Wiseman. Wiseman is a former naval aviator, engineer on the ISS, and chief of the Astronaut office (NASA, 2023). This crew is currently expected to be the participants of Artemis II, III, and the annual lunar missions that follow.
In order for the astronauts to be protected from the harsh and foreign conditions of space and the Moon, they need to don one of the most iconic symbols of spaceflight; the spacesuit. The spacesuit worn by Apollo 17 astronauts while traversing the Moon was called the Extravehicular Motility Unit (EMU) and was comprised of the Pressurized Suit Assembly (a.k.a. PSA, the suit itself) and the Portable Life Support System (a.k.a. PLSS, the suit’s “backpack”). The PSA was made up of three layers: An inner comfort layer, an Intermediate Pressure Garment assembly that controlled the atmosphere within the suit to maximize mobility, and the Thermal Micrometeoroid Garment (TMG) (ILC, 2013). The TMG consisted of several layers of Mylar and spacer fabric to reduce thermal conductivity (moon temperatures ranging from 121°C to -91°C between day and night) and deflect radiation, covered by a B-cloth layer providing fire resistance. The gloves were designed with chromel-R-chromium steel in the palms to protect against any lacerations, with silicone-infused fingertips to assist in grabbing samples and tools. The helmet kept the suit at a constant pressure, connected a feeding tube containing a “nutritive paste” to the astronaut’s mouths, and had an adjustable polycarbonate and gold-coated polysulfate visor to protect the eyes from the intense sun (ILC, 2013).
The PLSS kept the suit a constant pressure of 8 lb/in to ensure mobility as close to “the nude condition” as possible, as well as supplying 6 cubic ft/min of oxygen for up to 7 hours, eliminating CO2 and excess humidity, and providing an internal cooling system (Thomas, n.d.). The suit was also able to communicate with mission control via radio and record heart rate and certain conditions of the wearer (ILC, 2013). The lunar exploration spacesuit to be worn by Artemis astronauts is currently being designed jointly by Prada and Axiom and will be called the Axiom Extravehicular Mobility Unit (AxEMU). Because it is currently in the design process, with its critical design review planned for June 2024, little has been publicly released about the specific components that comprise it. However, Axiom has publicly promised that it will be designed to be able to fit 90% of the U.S. population, in accordance with the Artemis missions’ emphasis on diversity and representation. Furthermore, Axiom promises a wide range of motility, with a specific focus on bending and kneeling, which have been difficult in spacesuits of the past. Additionally, the suits are expected to be designed specifically for ease of use with Axiom tools such as hammers and grabbers on the Moon, shown in Figure 2 (Axiom Space, 2024). Inclusion of a Head-up Display (HUD) was conceptualized as an element on the suit’s wrist, giving the Astronauts the ability to easily see personal and suit data such as oxygen levels, heart rate, battery, and geographical positioning data, but this remains unconfirmed (NASA, 2022).
The Apollo suits were over a $100 million investment to produce, while the Artemis suits cost billions (NASA, 2022). Such investment reflects the necessity of getting the spacesuits working perfectly well before the beginning of the journey; their success or failure is one of the many factors a lunar voyage hinges on.
The Apollo 17 journey began with the launch of a Saturn V at the Kennedy Space Center on December 17, 1972. The model used for the launch of every Apollo mission, the Saturn V was a massive three-module rocket weighing 4,400,000 pounds and boasting five kerosine-liquid-oxygen F-1 engines that gave it a thrust of 7.6 million pounds (Lewis, 2021). The rocket reached speeds of 5,400 mph almost instantly after takeoff, taking under three minutes to travel 40 miles into the atmosphere, where it jettisoned the first module (3,000,000 pounds) and used five smaller engines to accelerate to 14,000 mph. At about 120 miles, the second module was jettisoned and the rocket propelled out of Earth’s gravity thanks to a single fuel engine bringing it to 25,000 mph. In space, the Command and Service Module (a.k.a. CSM, where the astronauts are housed during space travel) and the attached Challenger lunar module (the vessel taking the astronauts from lunar orbit to its surface) detached from the third Saturn module and began the three day journey to the moon’s orbit. Arriving, the astronauts relocated to the lunar module and made the descent to land in about two hours (Uri, 2022).
Once again, the 50 years between Apollo and Artemis have enabled great technological advancement, plain to see in the ex-
pected journey the Artemis III astronauts will take to get to the moon. Again launching from the Kennedy Space Center, the rocket responsible for sending the crew out of Earth’s atmosphere is called the Space Launch System (SLS). Weighing 5.74 million pounds, the SLS can reach a maximum of 8.8 million pounds of thrust thanks to its four hydrogen-liquid-oxygen powered RS-25 engines and two rocket boosters that will be jettisoned immediately after launch, both of which shown in Figure 2. The SLS is designed with the future in mind, with an “evolvable” base able to be modified for future missions to the Moon, Mars, Jupiter, and Saturn
After escaping the Earth’s gravity, the SLS’s cryogenic propulsion stage will “push” the Orion spacecraft at its peak on its lunar trajectory at about 25,000 mph (NASA, 2023). Reaching orbit after 2-3 days, the Orion will dock with a Space X-Produced lunar module named Starship that had been sent there independently, which Koch and Glover will enter and take to the surface (Mohon & Williams, 2023). Like the CSM, the Orion will remain in lunar orbit until the astronauts are prepared to return to Earth.
The Apollo 17 lunar module was set to land in the Tarais-Littrow region of the Moon’s equatorial highlands due to its diverse geographical features, ranging from some of its youngest volcanic areas to oldest rock samples, that promised a lot of discovery (Lewis, 2021). Cernan and Schmitt spent a total of 75 hours on the Moon, 22 of them actually traversing the lunar surface. With the aid of a rover, they spent their time collecting samples, taking photographs, and conducting tests across 19 miles of the surface. The tests they conducted were primarily focused on the Moon’s gravity, seismic activity, and atmospheric composition. Additionally, they deployed the Apollo Lunar Surface Experiments package that read head flow data and reported it back to scientists years after launch following their departure. By the end of their journey, the two astronauts had collected 243.65 pounds of rocks and soil to take back
to Earth for analysis, and that are still being used to this day (NASA, 2011). Some of the most significant discoveries from the mission are orange soil from moon lava and certain rocks revealing that the Moon once had a magnetic field (TPS, n.d.).
The Artemis astronauts will be landing far from the Apollo 17 site, being the first mission to explore the regions near the lunar south pole. This spot was chosen primarily due to the permanently shadowed areas of the large rock, which may provide the shade and cool temperatures needed to find and analyze lunar ice. The astronauts will remain on the moon’s surface for six total days, again using a rover to explore miles away from their landing site, collecting samples, taking photographs, and conducting tests (Mohon & Williams, 2023).
Data collection is really only one of two of NASA’s goals for the Artemis III mission, the second being unique to any lunar voyage in human history — exploring the lunar south pole for the optimal region to build a Moon base, somewhere on the lunar surface where astronauts could live for months at a time during future missions (Gregerson, 2024). Current concepts for this base camp feature a main cabin, permanent lunar rover, open-top lunar terrain vehicle (LTV), and “mobile home” unit for overnight exploration and camping. Access to ice, and therefore water, are why the south pole is the ideal location for this camp, making the Artemis team’s exploration crucial for the future of space travel (NASA, 2020).
Many of the changes made in Moon travel between Apollo 17 and Artemis are pretty clear reflections of how the country has changed over half a century. Obviously, there have been dramatic scientific and technological developments, but also many more. The increased demand for social equity and representation can be seen in the diversity of the Artemis crew, allowing so many more people to see themselves in the astronauts who walk in space for us. Furthermore, this mission isn’t a competition between countries to establish scientific
supremacy; Artemis is the culmination of work with several other countries, including a Canadian crew member, with an end goal of establishing a moon base that according to former NASA administrator Jim Bridenstine “[All countries] will use… to validate human safety protocols, technologies, and operational procedures before embarking on the ultimate human destination,” (Bridenstine, 2019)
Equally illuminating is what has stayed the same between the two missions; people working together to achieve impossible goals, a fervent passion for science, and an insatiable drive for discovery. Fifty years later and the only thing that has changed about that is that more people’s voices can be heard, and no longer shouting over each other. As for the “ultimate human destination” that Bridenstine referred to, take a look at the Artemis program logo (Figure 3).
The obvious parts of the logo are Earth at the bottom and the Moon at the top of the “A” for Artemis. Notice that the trail from Earth to the Moon is red and the tip of the “A” takes on the shape of an arrow, pointing into space past the Moon. This represents the ultimate human destination, and the road that Artemis leads to; Mars. While there is no doubt that the return to the lunar surface will be exciting, informative, and groundbreaking, it’s plain to see that, in more ways than one, the real purpose of Artemis is beyond the moon.
Works Cited
Axiom Space. “Axiom Suit.” Axiom Space, 2024, www.axiomspace.com/axiom-suit.
Bridenstine, Jim. “Artemis Is Our Future – Former NASA Administrator Jim Bridenstine.” Blogs.nasa.gov, 23 July 2019, blogs.nasa.gov/bridenstine/2019/07/23/Artemis-is-our-future/. Accessed 27 Mar. 2024.
Government of Canada. “Biography of Jeremy R. Hansen.” Www.asc-Csa.gc.ca, 13 May 2009, www.asc-csa.gc.ca/eng/astronauts/canadian/active/bio-jeremy-hansen.asp.
Gregersen, Erik. “Artemis | Definition, Name, Missions, & Facts | Britannica.” Www.britannica.com, 20 Mar. 2024, www.britannica.com/topic/Artemis-program. International Latex Corporation (ILC). A HISTORIC MECHANICAL ENGINEERING LANDMARK. 2013.
Lewis, Robert. “Apollo 17 | Overview & Facts | Britannica.” Www.britannica.com, 25 Mar. 2021, www.britannica.com/ event/Apollo-17.
Mohon, Lee. “Space Launch System - NASA.” NASA, 13 Dec. 2021, www.nasa.gov/directorates/esdmd/space-launch-system-ftdku/.
NASA. “Apollo 17: Mission Details - NASA.” NASA, 7 Apr. 2011, www.nasa.gov/missions/apollo/apollo-17-mission-details/.
“Artemis - NASA.” National Aeronautics and Space Administration- Artemis, www.nasa.gov/feature/Artemis/.
Artemis Generation Spacesuits Educator Guide ENGINEERING and LIFE SCIENCES next Gen STEM -Moon. Sept. 2022.
“Christina Koch - NASA.” NASA; Artemis; People, May 2023, www.nasa.gov/people/christina-koch/.
“Lunar Living: NASA’s Artemis Base Camp Concept – Artemis.” Blogs.nasa.gov, 28 Oct. 2020, blogs.nasa.gov/Artemis /2020/10/28/lunar-living-nasas- Artemis -base-camp-concept/.
“NASA Astronaut: G. Reid Wiseman - NASA.” NASA; Humans in Space, 2023, www.nasa.gov/humans-in-space/astronauts/g-reid-wiseman/.
“NASA Astronaut: Victor J. Glover - NASA.” NASA; Humans in Space, 2023, www.nasa.gov/humans-in-space/astronauts/victor-j-glover/.
“Space Launch System - NASA.” NASA, 2023, www.nasa.gov/ reference/space-launch-system/.
The Planetary Society (TPS). “Apollo 17.” The Planetary Society, www.planetary.org/space-missions/apollo-17. Accessed 27 Mar. 2024.
Thomas, Kenneth. The Apollo Portable Life Support System. Uri, John. “50 Years Ago: Apollo 17 Lights up the Night Sky on Its Way to the Moon - NASA.” NASA, 6 Dec. 2022, www.nasa. gov/history/50-years-ago-apollo-17-lights-up-the-night-skyon-its-way-to-the-moon/. Accessed 27 Mar. 2024.
“50 Years Ago: NASA Names Crew for Apollo 17, the Last Moon Landing Mission - NASA.” NASA, 12 Aug. 2021, www.nasa. gov/history/50-years-ago-nasa-names-crew-for-apollo-17the-last-moon-landing-mission/. Accessed 27 Mar. 2024.
Williams, Catherine, and Lee Mohon. “Artemis III: NASA’s First Human Mission to the Lunar South Pole - NASA.” NASA, 13 Jan. 2023, www.nasa.gov/missions/Artemis/Artemis-iii/.
For decades, the world has faced the evolving challenges of infectious diseases, from the Black Death in Medieval Europe to the Spanish flu in 1918 which took millions of lives after World War I. History is filled with grim examples of pandemics that have significantly influenced our societies, economies, and health systems. These echoes of past pandemics alongside the recent COVID-19 pandemic underscore the ongoing battle against infectious diseases. They highlight the significant impact these diseases can have on a global scale, particularly influenza, which remains a persistent threat to public health worldwide.
According to the World Health Organization, there are around a billion cases of seasonal influenzas annually, including 190,000 to 650,000 respiratory deaths (WHO, 2023). These statistics not only reflect the substantial burden influenza places on health systems but also highlight the urgent need for more effective prevention strategies, including the development of a universal flu vaccine. Currently, there are no platforms approved for human influenza virus vaccine implementation. However, existing approaches have not solved the obstacle of low influenza vaccination effectiveness. A potential solution to this problem is the development of a universal influenza vaccine that would provide effective immunity against all strains of the virus (Deviatkin et al., 2022).
In this editorial, we will dive into the scientific explanation of the multivariant mRNA that aims to targets multiple variants of a virus within a single vaccine and discuss the promise of this technology to usher in a new era of flu prevention – one that could potentially turn the tables on this pervasive threat by protecting a broad spectrum of flu viruses.
The Science Behind Multivariant mRNA Vaccine:
The influenza virus causes yearly outbreaks, often epidemics, and occasionally pandemics. These effects occur due to the high variability of the virus where the type A virus is subjected to both “antigenic shift,” which is a major change to a flu strain due to cross-species transfer, and “antigenic drift,” or a small change to a flu strain. In this regard, the development of vaccines against individual strains is untenable, leading to a desperate need to create “universal” vaccines (Deviatkin et al., 2022). According to the National Institute of Allergy and Infectious Diseases (NIAID),
the criteria for a universal vaccine are (1) at least 75% efficacy against symptomatic influenza virus infection, (2) protection against Group I and Group II influenza A viruses (influenza B virus would be a secondary target), (3) durable protection that lasts at least 1 year and preferably through multiple seasons, and (4) is suitable for all age groups. (Erbelding et al., 2018). The invention of multivariant mRNA vaccines bridges the gap in immunity by providing a broader range of immunity that incorporates antigens, which cause the body to make an immune response that includes toxins, chemicals, viruses, etc., from all 20 known subtypes of influenza A and B viruses. Such a pathogen and antibody interaction, described in Fig 1, indicates the importance of the development of a universal influenza vaccine that targets a limited set of antigens from conserved regions of viral proteins shared by many subtypes (Villanueva, 2023).
Figure 1: The human body needs to build specific antibodies against viral antigens made by a pathogen. Antibodies grab onto antigens and help defeat the pathogen. (Source: WHO, 2020)
The mRNA lipid nanoparticle vaccines use messenger RNA (mRNA) to instruct cells in the body to produce a protein that is part of the virus. This protein triggers an immune response, which prepares the immune system to recognize and fight the virus if the body is exposed to it in the future (Fig. 2). In the context of vaccines, scientists design mRNA sequences that encode the viral proteins recognized by the immune system. Once these mRNA sequences are introduced into the body through vaccination, they enter human cells and utilize their ribosomes to produce the viral proteins. mRNA vaccines can be divided into two types: conventional mRNA and self-amplifying mRNA (SAM) vaccines. Conventional mRNA vaccines take advantage of cellular machinery to translate the appropriate protein, whereas SAM vaccines have extra coding accessory proteins for self-replication (RNA-dependent RNA polymerase, capping enzymes, proteases) beside target RNA (Deviatkin et al., 2022).
However, the biggest challenge that scientists have faced is that the mRNA degraded quickly before it could “deliver” the
RNA transcript and be read into proteins in the cells. The solution to this problem came from advances in nanotechnology: the development of fatty droplets (lipid nanoparticles) that wrap around the mRNA like a protective bubble and allowing a better permeability of the negatively charged mRNA into the cell. Once inside the cell, the mRNA message can be translated into proteins, like the spike protein of SARS-CoV-2, and the immune system can then be primed to recognize the foreign protein (Chris Beyrer, 2021).
The Promise Of mRNA Technology:
Though many people first became aware of mRNA technology because of COVID-19 vaccines, it is not new to the scientific community. For decades, scientists have studied mRNA, looking for ways to unlock its potential to prevent and treat disease (Pfizer, n.d.). However, the success of mRNA vaccines made by Pfizer in the fight against COVID-19 has paved the way for their application in other areas, as the beauty of mRNA technology lies in its flexibility and speed of development which allows for quick adjustments in response to new strains. The potential to include multiple strains within a single vaccine could drastically improve the efficacy of seasonal flu vaccines and move us closer to the goal of a universal vaccine.
However, the widespread use of mRNA vaccines is hampered by the instability of mRNA. A potential solution to this problem is the introduction of covalently closed circular mRNAs which refer to the covalent joining of the 5’ and 3’ ends of the mRNA strand as a new platform for the delivery of antigen coding sequences to the human organism. Universal influenza vaccines based on this platform have not been developed before; therefore, there are potential pitfalls that are currently invisible, and the new platform should perhaps be treated with restrained optimism. Regardless, the mRNA-based technique could be a prospective strategy that shortens the time from identifying candidate influenza strains through the seasonal vaccine development and distribution process. Additionally, according to the proof-of-concept results of Chivukula et al., the mRNA strategy is also promising for broadly protective universal influenza vaccine creations as well (Deviatkin et al., 2022).
In conclusion, the development of a universal flu vaccine using mRNA technology represents a monumental stride forward in public health, with the potential to save countless lives and significantly reduce the global burden of influenza. However,
this innovation must be approached with a keen awareness of the ethical and equity issues it presents. As we navigate this promising frontier, it is our collective responsibility to ensure that these advancements are not only scientifically sound but also ethically grounded and equitably accessible. Achieving this balance will require concerted efforts from governments, healthcare organizations, communities, and individuals alike. Only by addressing these challenges head-on can we fully realize the potential of mRNA technology to transform our approach to influenza prevention and build a healthier, more equitable world for future generations.
“
Through many people first became aware of mRNA technology becuase of COVID-19 vaccines, it is not new to the scientific commuinty.
”
Works Cited
Beyrer, Chris. (2021, October 6). The long history of mRNA vaccines. Johns Hopkins Bloomberg School of Public Health. https://publichealth.jhu.edu/2021/the-long-history-of-mrna-vaccines Deviatkin, A. A., Simonov, R. A., Trutneva, K. A., Maznina, A. A., Khavina, E. M., & Volchkov, P. Y. (2022). Universal flu mRNA vaccine: Promises, prospects, and problems. Vaccines, 10(5), 709. https://doi. org/10.3390/vaccines10050709
Erbelding, E. J., Post, D. J., Stemmy, E. J., Roberts, P. C., Augustine, A. D., Ferguson, S., Paules, C. I., Graham, B. S., & Fauci, A. S. (2018). A universal influenza vaccine: The strategic plan for the National Institute of Allergy and Infectious Diseases. The Journal of Infectious Diseases, 218(3), 347-354. https://doi.org/10.1093/infdis/jiy103 WHO. (2020, December 8). How do vaccines work?, World Health Organization (WHO). https://www.who.int/news-room/feature-stories/ detail/how-do-vaccines-work WHO. (2023, January 12). Influenza (Seasonal)., World Health Organization (WHO). https://www.who.int/news-room/fact-sheets/detail/ influenza-(seasonal)
Tabak, Lawrence D.D.S., Ph.D. (2023, October 12). MRNA vaccine –NIH director’s blog. NIH Director’s Blog. https://directorsblog.nih.gov/ tag/mrna-vaccine/ Pfizer. (n.d.). MRNA technology: What it is and how it works. https:// www.pfizer.com/science/innovation/mrna-technology Villanueva, M. T. (2023). An mRNA universal vaccine for influenza. Nature Reviews Drug Discovery, 22(2), 98. https://doi.org/10.1038/ d41573-023-00013-z
Tracing the evolution of the environmental movement from campus to community
It is said that when greatness is nurtured by the fertile soils of academia, it gives birth to a legacy. Much like John Muir, the father of wildlife ecology and creator of the Yosemite and Grand Canyon National Parks, who found his intellectual grounding at the University of Wisconsin-Madison, many other environmental leaders also found their first footing on these beautiful campus grounds.
John muir
1860-1863
Isolated on his family farm in Marquette County, Wisconsin, John Muir was forced to run away from his father who believed that the only book a person needed was the Bible. Scraping together money from odd jobs, he found the means to enroll himself in a few science courses in his short time at the University of Wisconsin-Madison (1860-1863). While residing in the North Hall, the first campus building constructed in 1852, he developed and indulged in his love for mechanical inventions which soon opened doors for his global environmental contributions. As a freshman, he invented “a machine to make visible the growth of plants and the action of sunlight,
The University of Wisconsin-Madison is known nation-wide for its environmental programs and sustainability practices. No other university has its roots more deeply embedded in conservation and stewardship. The ideas and actions of Wisconsin pioneers such as John Muir, Charles Van Hise, Aldo Leopold, and Gaylord Nelson are an inspiration in addressing one of the greatest challenges of the 21st century: How to meet the needs of the present without compromising the ability of future generations to meet their own needs. Each of these figures carved out a significant place of conservation history. Let’s delve into their stories to learn more about their profound contributions.
*Timeline is not to scale
a very delicate contrivance, enclosed in glass.” (Holmes et al., 1999) However, after four years at the university he set on botanical and global expeditions to places such as the Gulf of Mexico. These journeys would last the rest of his life.
A leading figure of moral and aesthetic environmental conservation, Muir became known worldwide for his love for the wild and for identifying humanity’s role in it. His curiosity for nature took him all the way from California to Alaska and allowed him to come up with the theory of how ice shaped the valleys of Yosemite.. His unique observations about the land and novel ways of expressing the beauty of nature through poetry rekindled America’s love for wilderness. Muir’s articles “The Treasures of Yosemite” and “Features of a Proposed Yosemite National Park” became popular worldwide and months after their publication, he was able to successfully designate Yosemite as a national park with the help of President Roosevelt. Among his amazing resume, he also established the National Park Services in 1916 and founded the Sierra Club, a nonprofit organization promoting outdoor recreation and environmental advocacy. Muir’s conservation legacy lives on today, resonating with the values born here on our campus.
Image: John Muir (Source: UW Archives)
With a milestone essay on land ethic and conservation in 1949, Aldo Leopold was a pioneering figure of environmentalism, specifically during his time at the University of Wisconsin-Madison. With his graduate degree at the Yale School of Forestry made possible by Griffard Pinchot, another renowned environmental figure, Leopold’s experiences with wildlife hunting for the US Forest Service’s left him unsettled with the management approaches to environmental preservation. He believed that it was easier to maintain wilderness than to create it. In his era of ecological dominance, the term “wilderness” had a new meaning, representing nature as well as predators as a whole.
He joined the faculty of the University of Wisconsin-Madison in 1933 where he taught courses such as “Wildlife Techniques” and “Game Management.” He is best known as the author of the posthumously published A Sand County Almanac, some of which he drafted during his time at UW-Madison. An epochal, seminal, ageless work, the Almanac is considered to be the Bible for land managers, Koran for those who work the soil where literature overlaps ecology, and the urtext for the ecological restoration movement.
While growing up in southern Wisconsin, John Curtis spent his free time examining orchid seed physiology. This indeed spoke volumes about the bright future ahead of him, which included mapping Wisconsin vegetation as well as contributing to the establishment of the UW Arboretum, one of Wisconsin’s prized possessions and a beacon of conservation in the industrialized world. Gifted with problem-solving abilities, Curtis wanted to grow and hybridize orchids horticulturally, but knew that their reproductive physiology would have to be worked out carefully for each species for successful pollination and growth in specific environmental conditions. His doctoral work at the University of Wisconsin focused on the flower’s physiology and after his degree, he continued to work in the university as a plant pathologist. However, the time soon came for him to join the military in World War II and Curtis would soon travel to the Caribbean to work as a civilian research director. During his time there, he observed how invasive
Known famously as the “land ethic”, this concept proposed in the book focuses on the right to continued existence of all biotic things as well as on the notion of humans not being the controllers and conquerors of the environment, but rather just a plain citizen. His landmark works in environmentalism have shaped our understanding and love for our surroundings, and this is definitely not forgotten as we walk across the UW-Madison campus. His name continues to live on all over the United States, as we celebrate ‘Leopold’s Week’ every year, during the first week of March.
Image: Aldo Leopold at the Shack (Source: UW Archives)
species destroyed native plant species and made up his mind to return home and find ways to conserve Wisconsin’s natural plant species.
Upon his return to civil society, he rekindled his relationship with many renowned scholars at the University of Wisconsin-Madison and in the field of plant pathology across the country. Curtis, along with his colleagues and graduate students, dedicated years to conducting extensive field studies at sites where Wisconsin’s prairies once flourished. Their research revealed significant changes in natural communities since the state’s pioneer settlement era. These findings culminated in Curtis’s seminal work, “The Vegetation of Wisconsin: An Ordination of Plant Communities,” published in 1959. Often referred to as the “Bible of Wisconsin plant ecology,” the book synthesized their findings and provided invaluable insights into the state’s ecological landscape (Hathaway, 2004). The meticulously collected field data remains an essential resource today for the Plant Ecology Lab and researchers worldwide, serving as a crucial tool for assessing ecological change over time. His legacy continues to wander the grounds of campus, embodied by the Curtis Prairie – the oldest restored prairie in the world – home to hundreds of different plant species.
Image: John T. Curtis (Source: UW Archives)
Endearingly called the ‘Godmother of the Natural Landscaping Movement,’ Mary Lorraine Otto is a local and national environmental leader, famous for banning the use of DDT in Wisconsin (WCHF). A farmers daughter, Mary Otto was born on the outskirts of Madison, Wisconsin, and was aware of the impact that soil had on life and other supporting systems. She graduated with an art major from the University of Wisconsin-Madison in 1942. After marrying Owen Otto, son of UW-Madison professor Max Otto, who had a sister named Mary, she started calling herself Lorrie Otto. She lived in multiple places before settling down in the Village of Bayside, Wisconsin, located just a few miles away from Milwaukee. This decision of moving to Bayside proved to be a turning point in her life for many reasons. She achieved two major environmentalist achievements during her time there. First, was when she challenged weed laws in Wisconsin, triggered due to an incident involving the wildflower meadow in her front yard. After village officials mowed her wildflower meadow without warning, Otto used the incident to advocate against antiquated weed laws that promote uniform landscapes. She successfully lobbied for the protection of a 20-acre woodland, Fairy Chasm, which was under threat of development, eventually securing its preservation under Nature Conservancy protection.
Her second achievement and probably the most well-known one, was her activism toward banning DDT, a pesticide commonly used at that time in Wisconsin to control mosquitoes and the Dutch Elm Disease. Otto observed dead
birds and bats in her property, Fairy Chasm. She learnt from UW-Madison ornithologist Joseph Hickey that DDT was the probable cause. Her persistence in addressing this issue led to hearings on DDT in Wisconsin, where she organized scientists and witnesses to present evidence against its use. Ultimately, her efforts resulted in the banning of DDT in Wisconsin in 1970, followed by a nationwide ban two years later. In years that followed this hearing, Otto dedicated her personal and professional life to the cause that made her the most famous: promoting natural landscaping through art, podcasts and writing.
Image: Lorrie Otto in a native wildflower garden behind her home (Source: Wisconsin Historical Society, Lorrie Otto Papers)
Born in the small town of Clear Lake, Wisconsin, Gaylord Nelson always had a keen interest in politics and law. He received an L.L.B. degree from the University of Wisconsin-Madison and went on to become one of America’s most outspoken and keen environmental advocates. After earning his law degree in 1942 and serving in the Navy during World War II, Nelson climbed the Wisconsin political ladder. As a state senator and later governor, he consistently prioritized issues related to land protection, wildlife habitat, and environmental quality. However, Nelson’s most significant achievements came when he transitioned to the national stage as a U.S. Senator. He spearheaded the passage of several landmark environmental laws, including those protecting wilderness areas, establishing national trails and scenic rivers, and promoting environmental education. He also introduced legislation to improve fuel efficiency in cars, regulate strip mining, and ban harmful substances like phosphates in detergents and pesticides like DDT and other potent herbicides. He also went on to be the counselor of The Wilderness Society where he advocated protecting America’s national forests, national parks, and other public lands from harmful development.
While his work was already impressive amongst the environmental sphere, he is best known now as the founder of The Earth Day. Described as “one of the most remarkable happenings in the history of democracy,” his efforts on behalf of the
environment brought him widespread admiration and acclaim (Congressional Record Volume, 2005). On April 22, 1970, Nelson, alongside 20 million U.S. citizens, celebrated the first Earth Day with a teach-in to discuss conservation issues. That same year, the UW founded the Institute for Environmental Studies to support a similar mission: environmental education, research, and public outreach. In 2002, the institute added “Nelson’’ to its official name to honor Nelson’s legacy and channel his cause. This year — 52 years later — Earth Day and the Nelson Institute are still going strong. In fact, Earth Day is celebrated as Earth Week on the UW campus because there are too many events for a single day!
Image: Gaylord Nelson Speaking at a podium on Earth Day
(Source: Wisconsin Historical Society)
Born and brought up in India, M.S. Swaminathan’s commitment towards ensuring food security won him the title of the “Father of Green Revolution.” Growing up in an agricultural community, his interactions with farmers, farmlands, and the impact of climate and pests on crops played an instrumental role in developing his interests in agricultural and food technology. The principle turning point that set up his future work was when he witnessed the devastating impacts of the Bengal famine of 1943 during the Second World War and the shortages of rice throughout the entire Indian subcontinent. After such large-scale environmental dilemmas, he decided to dedicate his life towards ensuring that India would have sufficient access to food. After finishing his graduate studies in India, Swaminathan moved to the United States, where he accepted a post-doctoral research associateship at the University of Wisconsin’s Laboratory of Genetics, assisting in the setting up of the USDA potato research station. During his time at the University of Wisconsin, Swaminathan had the opportunity to work alongside esteemed faculty members, including Nobel laureate Joshua Lederberg, who was part of the laboratory’s faculty at that time.
Despite the tempting offer of a faculty position, Swaminathan made the decision to return to India to continue making a difference in his home country. The UW-Madison Discovery Building marks the birthplace of the Green Revolution, a globally recognized effort of M.S. Swaminathan
to transform his birth country from a food-deficient nation to a food sufficient one (Mueller, 2023). This plaque at the Discovery Building commemorates a significant partnership that began in an unexpected place. In 1953, Norman E. Borlaug and M.S. Swaminathan crossed paths at Rennebohm’s Drug Store, which once stood where the Discovery Building is now located, during a genetics workshop hosted by the College of Agriculture and Life Sciences (Mueller, 2023). Swaminathan extended an invitation to Borlaug to visit South Asia, igniting a collaboration that would later ignite the Green Revolution. The remarkable contributions of these scientists during a crucial period of food production and hunger alleviation has since been recognized worldwide.
Image: M.S. Swaminathan speaking when he received the 1987 World Food Prize (Source: World Food Prize)
Our lives are deeply entwined with the legacy of those who came before us. When I stroll through campus as a current UW-Madison student, I feel a sense of awe thinking about the various global environmental leaders who have walked the same path before me. As I wander through the Arboretum, I can almost envision Aldo Leopold, seated under a tree, penning his profound reflection of the land around him. And by the Lakeshore Path, I can almost imagine Gaylord Nelson passionately debating environmental law with his peers. In today’s world of rapid environmental changes and ecological destruction, these leaders stand as beacons of hope and action. Understanding the rich history of this university and its impactful alumni is a vital part of our journey towards engaging with global conservation issues.
Works Cited
Muir, John (1913). The Story of My Boyhood and Youth., Boston and New York, Houghton Mifflin Company, https://www.loc.gov/item/13005573/
Steven J. Holmes, Jasen, Patricia (1999). The Young John Muir: An Environmental Biography., Madison: University of Wisconsin Press., The American Historical Review, Volume 105, Issue 3, June 2000, Page 940, https://doi.org/10.1086/ahr/105.3.940
Muir, J. & Bade, W. F. (1916) A thousand-mile walk to the Gulf., Boston and New York, Houghton Mifflin Company, https://www.loc.gov/ item/16023580/ Leopold, Aldo, 1886-1948. (1949). A Sand County almanac, and Sketches here and there.
Where ethics meet earth. The Aldo Leopold Foundation. https://www.
aldoleopold.org/
The Aldo Leopold Archives. https://search.library.wisc.edu/digital/AAldoLeopold\ Wisconsin Conservation Hall of Fame. https://wchf.org/john-thomas-curtis/
University of Wisconsin Plant Ecology Laboratory. http://pel.botany. wisc.edu/history.html
Stanley A. Cain (1960). The Vegetation of Wisconsin: An Ordination of Plant Communities, Forest Science, Volume 6, Issue 2, June 1960, Pages 169–170 https://doi.org/10.1093/forestscience/6.2.169
Earth Day, Gaylord Nelson Earth Day. https://nelsonearthday.net
Christofferson, Bill (2004). The Earth Day Founder: Senator Gaylord Nelson, Madison, Wisconsin: University of Wisconsin-Press, https://uwpress. wisc.edu/books/4766.htm
Nelson, Gaylord 1916-2005 (1954-2006): Gaylord Nelson Papers, Wisconsin Historical Society, Division of Library, Archives and Museum Collection, http://digital.library.wisc.edu/1711.dl/wiarchives.uw-whsmss01020
Relative to the Death of Former Senator Gaylord Nelson, Congressional Record Volume 151, Number 92, The Congressional Record Online through the Government Publishing Office
Muller, Peyton (2023). Discovery Building Houses Plaque marking the Birthplace of Green Revolution
Nature India (2009). Borlaug’s unfinished crusade https://doi. org/10.1038/nindia.2009.304
Wisconsin Conservation Hall of Fame. https://wchf.org/lorrie-otto/ Otto, Lorrie (Mary Lorraine), 1919-2010 (1960-1996): Lorrie Otto Papers Wisconsin Historical Society, Division of Library, Archives and Museum Collections, http://dig
the cover:
Thank you to all of the photographers that submitted their work to JUST! All your beautiful photographs are greatly appreciated!
Agnes Moreno Fiona King Fiona King Fiona King Haley street Sam warfel on Sam warfelWe are all familiar with that last apple at the bottom of the bag, the one with a bruise or a cut that has something brown that just isn’t appealing, or the bag of spinach forgotten in the back of the refrigerator. It is estimated that between 30 and 40% of the food produced in the United States goes to waste; apart from consumer waste, there is also an incredible amount of food lost by producers, in transportation, or at supermarkets (USDA, 2024). While it may seem that wasted food is inevitable, there is a way for it to be sustainably exploited through composting.
Composting is the process of controlled decomposition and the natural breakdown of organic materials. In the current waste management system, almost all the organic waste goes to landfills where, under improper conditions, it breaks down into dangerous greenhouse gasses and perpetuates a cycle of wastefulness (Algahaiti et al, 2018). Composted, organic waste can be broken down into a soil additive that can fertilize soils, increase crop yields, and omit the production of aforementioned greenhouse gasses.
The core of the composting process is dependent on the microorganisms naturally occurring in soils. In nature, decomposition and natural composting is facilitated by insects and worms breaking down large organic materials. However, in industrial and home composting, such organisms are rarely present. Therefore, grinding and chopping the organic waste is necessary to achieve compostable finer sizes. Once these physical conditions are met, bacteria, fungi, and other microorganisms are activated.
Composting can be separated into three stages: mesophilic, thermophilic, and maturation. The first mesophilic stage could be imagined as softening organic matter and preparing it for the thermophilic stage. “Initial decomposition is carried out by mesophilic microorganisms, which rapidly break down the soluble, readily degradable compounds… the heat they produce causes the compost temperature to rapidly rise” (Trautmann and Olynciw, 1996). Mesophilic organisms are defined as those that thrive in moderate temperatures. As the heat makes the environment less survivable for these mesophilic microor-
ganisms, it makes it more ideal for another set of organisms known as thermophilic organisms.
These thermophilic organisms are responsible for a majority of the decomposition that occurs in compost. “During the thermophilic phase, high temperatures accelerate the breakdown of proteins, fats, and complex carbohydrates like cellulose and hemicellulose, the major structural molecules in plants” (Trautmann and Olynciw, 1996). As the microorganisms eventually run out of matter to decompose, the temperature of the compost decreases allowing for the resurgence of mesophilic organisms, which are responsible for leftover ‘curing’ of organic matter. The curing process is essential to tame any extreme elements left over in the compost material which could cause extreme pH values, high salt contents, or ratios of organic compounds (nitrogen and carbon) which would be unhealthy to plant life when compost is used as a fertilizer. Curing is a less defined process, some composters cure for around 3 months, while others cure for nearly 9 months. The final product of compost is considered ‘stable’ when no more feedstock (or raw organic material) is being decomposed.
Compost Microorganisms
Healthy composting requires a diverse microbial community. Bacteria are the most abundant and the most diverse in compost, providing most of the decomposition, heat generation, and enzymes to chemically break down organic matter. During the first phase of composting, mesophilic bacteria are dominant. At higher temperatures, once the environment becomes unsustainable for mesophilic bacteria, thermophilic bacteria take rise. These bacteria are found naturally occurring in geysers at Yellowstone National Park, and in deep sea thermal vents, and flourish under the conditions of composting (Trautmann and Olynciw, 1996).
Other bacteria are actinomycetes, the bacterial organisms that responsible for the musty smell of soil in compost. They are responsible for most complex organic matter decomposition like chitin, cellulose, and other proteins. Fungi in compost provides a similar service, breaking down tough debris so that bacteria can reach the finer organics. Fungi are extremely aerobic, indicating the extreme importance of oxygen in composting. Lastly, rotifers and protozoa are microscopic organisms that feed on organic matter but in drastically smaller quantities than other organisms mentioned. Protozoa are single-celled organisms that exist in compost heaps while rotifers feed on bacteria and fungi, helping to maintain a healthy balance within compost.
Compost may seem like an isolated, small-scale choice that individuals make, but when done on larger scales it can have incredible benefits and impacts on large scale farming. Through composting, land can be revitalized and made viable for farming, crops can be made to grow in more abundance, and waste is ultimately reduced. The byproducts of microorganism decomposition produce carbon and nitrogen rich molecules which are beneficial to plant growth and soil enrichment. In a
more micro context, home composting can be beneficial for personal use. Adding composted material to potted plants or gardens will have the same benefits on the plant as larger scale fertilizing.
Commercial composting is also a growing industry, while Dane County currently does not currently offer compost collection, by 2026, the Dane County Department of Waste and Renewables plans to open their Sustainability Campus which will be home to a compost facility (Dane County, 2022). The same department currently offers grants for community composting in an effort to incentivize the reduction of food waste and responsible organic waste management. Although this seems like a large-scale endeavor, composting may be as easy as an extra trash can and some mindful disposal habits, through which every Dane County resident can help defer the cycle of needless food waste, landfill proliferation, and greater greenhouse gas emissions.
Algahaithi, Mouna, et al. Food Waste and Recovery Guide for Madison and Dane County, City of Madison, Oct. 2018, fyi.extension.wisc.edu/danefoodsystem/files/2018/12/Food-Wasteand-Recovery-Guide-for-Madison-and-Dane-County-FINALfor-web.pdf.
Cooperband, Leslie. “The Art and Science of Composting.” Center for Integrated Agriculture Systems, University of Wisconsin Madison, 29 Mar. 2002, cias.wisc.edu/wp-content/uploads/sites/194/2008/07/artofcompost1.pdf.
“Dane County Launches $10,000 Organics Management Grant
This Earth Month.” County of Dane, Wisconsin, County of Dane, 20 Apr. 2022, https://www.countyofdane.com/PressDetail/11046. Accessed 20 Feb. 2024.
Draft National Strategy for Reducing Food Loss and Waste and Recycling Organics, Dec. 2023, www.usda.gov/sites/default/ files/documents/food-waste-strategy.pdf.
Trautmann, Nancy, and Elaina Olynciw. “Compost Microorganisms.” Cornell Composting -- Science and Engineering, Cornell Waste Managment Institute, 1996, compost.css.cornell.edu/microorg.html.
Many residents of Wisconsin are aware that there has been an effort to reintroduce wolves into the wild over the past few decades. This decision has had some controversy due to incidents like livestock predation, however, a study published in 2021 revealed that wolf presence has brought about a new effect: reduced deer-vehicle collisions. Interestingly, this reduction is not simply due to wolves lowering the overall deer population – instead, a large part of this effect is caused by wolves influencing the behavior of white-tailed deer.
Each year, approximately 1 million deer-vehicle collisions occur, with around $10 billion in economic losses (Raynor et al., 2021). It is quite a costly (and occasionally fatal) issue that has been worsening since the 1990s. The fact that wolves can help reduce such a prevalent issue is fascinating and suggests that the relationship between humans and wolves is more complicated than it may appear.
Due to unregulated hunting in the 1800s, settlers in Wisconsin hunted all the bison, elk, caribous, and moose. Alongside such megafauna, settlers left declining deer populations across the state, causing wolves to turn towards livestock as prey. Subsequently, a bill was passed by the state that created a bounty on wolf populations in the 1860s (Wisconsin DNR, 2023). This bounty, along with increasingly difficult living conditions, led to the eventual loss of all wolves in the area. By 1960, wolves were declared extirpated (i.e. completely removed by humans) from Wisconsin.
In some states, people reintroduced wolves by manually moving them to the desired region; in Wisconsin, the method of reintroduction was different. Moving on their own, wolves slowly infiltrated the state from Minnesota in the 1970s after
Source: Flickr, MrTinMD, 2021
receiving federal protection (Wisconsin DNR, 2023). Although most people agree that the return of wolves is a positive step forward, it is not without its consequences. Between 1985 and 2019, the state of Wisconsin paid $3.1 million in compensation for damages caused by wolves (Raynor et. al 2021). The ecological balance of wolf presence has come at the cost of some livestock and pets, making it somewhat controversial.
Deer have had a huge population boom in Wisconsin’s recent history, which unsurprisingly has led to an increase in deer-vehicle collisions since the 1990s (Raynor et al., 2021). When faced with the fact that wolf presence reduces deer-vehicle collisions, one would assume that it is because the wolves are eating the deer. If the wolves eat the deer, then there are fewer deer and thus decreased collisions. While that’s a fair assumption to make, it’s not completely true.
Controlling for deer abundance (i.e. adjusting the calculations in consideration of the number of deer killed by wolves) reveals that deer population changes do not have that great of an effect on collision reduction. Abundance-controlled models show that when wolves kill deer, it has a minimal effect on reducing collisions. Instead, most of the effect that wolves have is behavioral – the presence of wolves causes deer to alter their behavior in such a way that they get hit by cars less frequently. In fact, if the behavioral changes were removed, wolves would have to reduce deer abundance by 74% in order to reciprocate the same decreased collision effect (Raynor et. al 2021). Unfortunately, this also means that human hunting does not have much of an effect on the rate of collisions; although hunting affects the deer population, it does not affect deer behavior in the same way that wolves do. Because humans cannot cause these behavioral changes in deer, we would not be able to replicate the collision-reducing effect that wolves have.
How is it possible for wolves to influence deer behavior in a way that reduces car collisions? The working idea is that deer avoid roads since wolves are frequently found there; wolves are known to travel along roads and pipelines for efficiency (Raynor et al., 2021). When there are no wolves in the area, deer have no reason to be wary of these travel corridors, but when wolves are active, deer must stay alert and away from danger.
Generally, research has shown that white-tailed deer have a preferred tactic for safety from wolves – early detection. White-tailed deer gain the upper-hand by spotting wolves before the wolves find them, making it easier for deer to outrun them and escape (Ma, 2019). Other types of deer species don’t always have the same tactic, like the mule deer of the west coast. Mule deer avoid wolves by moving to higher elevations, as well as favoring rocky and brushy terrain (Ma, 2019). Incidentally, this means that they also stay away from roads. Regardless of the specific tactic for avoiding wolves, it seems that roads are not safe for deer. And when they stay away from the road, they stay safe from cars.
Conclusion
This phenomenon of behavioral change has proven to be an interesting example of something that has been hard to prove – that predators are crucial to maintaining ecological balance and that humans benefit from that balance. Ecologists and economists have both seen the potential for predators to benefit humans, but it has been hard to prove such until after predators were removed from an ecosystem (Raynor et al., 2021). Now, we can see very specific and significant benefits: the money saved by reduced collisions far exceeds the money spent on wolf incidents by a ratio of 63 to 1 (Raynor et al., 2021).
This economic difference helps support the case for protecting wolves beyond exclusively ecological reasons. Protecting and accommodating nature is not just a moral issue. As humans, we are a part of nature, and as time passes, we’ll continue to learn of ways in which we benefit from respecting nature. For now, though, hitting fewer deer with cars is a good start.
Works Cited
Ma, Michelle. “Return of the Wolves: How Deer Escape Tactics Help Save Their Lives.”
UW News, 27 Feb. 2019, www.washington.edu/ news/2019/02/27/return-of-the-wolves-how-deer-escape-tactics-help-save-their-lives/#:~:text=Overall%2C%20the%20 researchers%20found%20that,and%20toward%20brushy%2C%20rocky%20terrain.
Raynor, Jennifer L., et al. “Wolves Make Roadways Safer, Generating Large Economic Returns to Predator Conservation.” Proceedings of the National Academy of Sciences of the United States of America, vol. 118, no. 22, June 2021, pp. 1–10. EBSCOhost, https://doiorg. ezproxy.library.wisc.edu/10.1073/pnas.2023251118.
“Wolves in Wisconsin.” Wolves in Wisconsin | | Wisconsin DNR, dnr.wisconsin.gov/topic/WildlifeHabitat/wolf#:~:text=Unlike%20the%20famous%20wolf%20reintroductions,their%20 range%20into%20northern%20Wisconsin . Accessed 21 Feb. 2024.
Antibiotics, medications that inhibit or kill bacteria, are one of the most revolutionary discoveries of the 20th century (Davies & Davies, 2010). Not only have they significantly improved disease treatment and are attributed to saving the lives of humans and animals alike, but they have also transformed the agricultural industry.
However, the widespread production and use of antibiotics is not without consequence. In recent years, improper disposal of pharmaceuticals has led to antibiotic pollution with contamination primarily occurring in aquatic environments. After humans and animals consume antibiotics, pharmaceuticals enter the water cycle through their excrement (Serwecinska, 2020). About “10-90% of [antibiotics] ingested by humans and animals are metabolized (processed and utilized by the body) while the remainder remain active in feces (Serwecinska, 2020). From here, the processes by which human and animal excrement contribute to antibiotic pollution differ (Fig. 1). On one hand, human feces are flushed with water into sewage treatment plants, which often lack the technology to completely extract the antibiotics from the waste. When the treated wastewater is returned to the environment, the remaining antibiotics proceed to contaminate natural bodies of water. Animal excrement, on the other hand, contributes to antibiotic pollution more directly. Livestock manure is reclaimed for fertilization, allowing antibiotics to be transferred to the soil, groundwater, and surface water – thereby affecting both aquatic and terrestrial life (Zeng et al., 2022). Aside from human and animal contributions, wastewater produced by hospitals and pharmaceutical manufacturing plants also drives antibiotic pollution. Discharge of unused medications, pharmaceutical manufacturing byproducts, and antibiotic-containing bodily fluids, further amplify the environmental burden of antibiotic contamination.
Today, antibiotic pollution is considered an emerging issue, and the extent to which pharmaceuticals impact the environment is not yet fully understood. However, scientists have recently identified several consequences, such as the reduced effectiveness of antibiotics, disruption to biogeochemical processes, and biomagnification. Together, these implications demonstrate that antibiotic pollution must be considered a severe ecological problem that necessitates urgent action.
Antibiotic pollution must be considered a severe ecological problem because it leads to the development and spread of antibiotic-resistant bacteria, which reduces the effectiveness
of antibiotics and increases susceptibility to infectious diseases. When antibiotics are introduced to aquatic or soil environments, bacteria that are susceptible to antibiotics will be eliminated, while bacteria with adaptations (advantageous traits) to resist the pressures of antibiotics will be more likely to survive. Bacteria with antibiotic-resistant traits are therefore more likely to proliferate and spread antibiotic-resistant genes that code for this trait. As bacteria are exposed to various antibiotics at larger concentrations over time, they will continue to evolve to become resistant to antibiotics.
“
...several consequences, such as the reduced effectiveness of antibiotics, disruption to biogeochemical processes, and biomagnification.
”
Because antibiotics are polluting the environment at increasing concentrations, antibiotic resistance has increased at alarming rates; in fact, studies conducted in soil samples in the Netherlands found up to “15 times more genes-encoding resistance in 2008 when compared to soil samples from 1970” (Garcia et al., 2020). The increasing frequency of antibiotic resistance can result in dire implications for human and veterinary health (Kraemer et al., 2019). This is because antibiotic-resistant bacteria (ARB) can be acquired by animals and humans through ecological interactions – including exposure to antibiotic-polluted water, consuming food that has been contaminated by ARB, and the transfer of ARB between humans and animals (Larsson & Flach, 2022). Infections caused by ARB are incredibly difficult to treat due to the ineffectiveness of antibiotics on them. Furthermore, as ARB proliferate in animal and human hosts, they are further spread in the environment through animal and human excrement. The increased presence of ARB in the environment can therefore put more humans and animals at risk for infection, worsening the collective health outcomes of biota. In fact, it is predicted that “antibiotic resistance will kill 10 million people globally by 2050” (Zeng et al., 2022).
Overall, due to ecological interactions, pharmaceutical pollution has contributed to the rise of ARB and increased susceptibility to infection, thus demonstrating the severity of antibiotic pollution on human and animal health. Before ARB evolve to become even more resistant to antibiotics (perhaps leading to untreatable infection and immense population reduction), immediate action must be taken to address antibiotic pollution.
Antibiotic pollution should be addressed not only because it increases susceptibility to infectious diseases, but also because it alters bacterial community composition, disrupting biogeochemical processes that are essential for sustaining life on Earth.
For context, bacterial community structure is defined by the bacteria that make up a community and their corresponding interactions. Aquatic and soil ecosystems are colonized by incredibly diverse microbial communities, including bacteria of various species with varying tolerances to antibiotics. Therefore, when antibiotics pollute an ecosystem, they act as a disturbance that alters community diversity – killing off bacteria without antibiotic-resistance while causing limited harm to bacteria that do carry such genes. Additionally, it is important to note that diversity is defined both by species richness (the number of different kinds of species) and species evenness (the relative abundance of each species in proportion to the overall community population size). Thus, when disturbances are too frequent or too intense, such as when antibiotics pollute environments at high concentrations, community richness is reduced as some bacterial species are entirely depleted from a community.
Alterations to the community structure and the diversity of soil and aquatic environments are problematic because many of the bacteria in these ecosystems facilitate global biogeochem-
2: A diagram of role of bacteria in the nitrogen cycle (Source: Khan Academy, n.d.)
ical cycling (Kraemer et al., 2019). Biogeochemical cycling is the method by which essential chemical elements – including carbon, nitrogen, and sulfur – are transferred between living and nonliving beings (Fath, 2018). When antibiotics reduce community diversity in aquatic environments, this can lead to a loss of bacteria that are “responsible for carbon cycling and primary productivity” (Kraemer et al., 2019). Furthermore, studies have found that when antibiotics shift the composition of soil microbial communities, this often results in reduced bacterial activity in key steps of the nitrogen cycle – such as nitrification and denitrification (Fig. 2) (Kraemer et al., 2019). While these findings are scientific evidence of ecological harm, studies on biogeochemical cycles are often simulated in laboratory settings; opponents argue that, in reality, the concentration of antibiotic pollution in natural environments is too low to substantially impact biogeochemical cycling (Roose-Amsaleg & Laverman, 2016). However, it is necessary to consider that when antibiotics are present in the natural environment, they are often present as mixtures. Studies investigating the sulfur cycle have found that even when antibiotics are present at low concentrations and have no individual effect, but, when combined, antibiotics strongly inhibit sulfate reduction – an essential step of the sulfur cycle (Roose-Amsaleg & Laverman, 2016). Thus, even at low concentrations, the multitude of antibiotics that pollute natural environments work together to disrupt biogeochemical cycles.
Disruption of biogeochemical cycles has severe consequences. If cycles are disrupted, this may result in elements being less biologically available for plant and animal organisms, thereby inhibiting their growth and development (Mannion, 1998). For example, phosphorus and nitrogen are critical for soil fertility, and the reduction of these nutrients may reduce plant production (Hodges, 2010). Many organisms depend on the consumption of plants to obtain these elemental nutrients; the reduction of plant life or the nutrient availability in these plants therefore affects the health of organisms that consume them. Furthermore, biogeochemical cycles, such as the carbon cycle, regulate Earth’s climate. Disruptions to these cycles can lead to climatic changes that can alter plant growing seasons or
create environments that are unsuitable for organism survival. Through disrupting biogeochemical cycles and jeopardizing ecosystem stability, these detrimental outcomes demonstrate that the effects of antibiotic pollution are far-reaching and a serious ecological problem.
In addition to leading to the rise of ARB and disrupting biogeochemical processes, antibiotic pollution must also be considered a severe issue because the toxicity and bioaccumulation of antibiotics can lead to biomagnification. Bioaccumulation is the process by which pollutants gradually accumulate in an organism’s body, and there is currently evidence for antibiotic accumulation in both plants and aquatic organisms (Streit 1998). Studies have detected “antibiotic residues in crops fertilized by animal manure”, such as tomatoes, spinach, peppers, eggplants, and peanuts (Zhao et al., 2019). In addition, antibiotics have also been found in fish inhabiting natural environments, such as the Haihe River and Pearl River Delta in China (Gao et al., 2012). When antibiotics accumulate in plant and animal tissues, it leads to adverse organismal effects. In crops, antibiotics “inhibit root activity linked to nutrient absorption and biosynthesis” and cause an imbalance of “nutrients and hormones” (Zhao et al., 2019). Another study found that tetracycline, a class of antibiotics, inhibited zebrafish embryo development by prolonging hatching time, shortening body length, and manifesting in the loss of swim bladders (Zeng et al., 2022).
While the toxic effects on individual organisms make antibiotic pollution a problem worth addressing, bioaccumulation becomes a large-scale ecological issue when it results in biomagnification. Biomagnification is a phenomenon in which pollutants are transferred when predators consume prey, causing bioaccumulation to increase at each step of the food chain (Streit, 1998). It is important to recognize that due to limited research, antibiotic biomagnification is contested, with some scientists arguing that environmental concentrations of antibiotics are too low to bioaccumulate (Roose-Amsaleg & Laverman, 2016). A study investigating bioaccumulation in plankton communities found evidence of the antibiotic transfer between zooplankton and both their direct and indirect predators concluding that antibiotics “could undergo biomagnification along planktonic food webs” (Tang et al., 2020). Furthermore, some studies have also reported the health outcomes of biomagnification on humans. For example, due to the bioaccumulation of antibiotics in fish from Baiyangdian Lake, China, individuals who regularly consume these fish experience complications in child development and alterations to gut microbial communities, which are crucial for digestion and other bodily functions (Zahang et al., 2020; Zhao et al., 2019).
While more research is necessary to understand the extent of antibiotic biomagnification and its threat to higher-level organisms, the aforementioned evidence suggests that antibiotic pollution is toxic to organisms – with this toxicity compounding through biomagnification as organisms are found higher on the food chain. As the health of species is compromised by
biomagnification and their population sizes are reduced, this can alter community diversity and structure. If communities are unable to recover from the pressures of biomagnification, this can be detrimental to community stability (the ability of a community to maintain its structure over time), resulting in additional loss of species and ecosystem functions. Thus, this ecosystem impact further emphasizes the gravity of antibiotic pollution.
As the use of antibiotics has increased significantly for medical and agricultural purposes, debate persists about the consequences of antibiotic pollution and whether intervention is warranted. However, by contributing to the rise of ARB and reducing antibiotic efficacy, antibiotic pollution harms the health outcomes of humans and animals. Antibiotic pollution also disrupts biogeochemical cycles (which are essential for global processes) by altering soil and aquatic bacterial community structures. Furthermore, antibiotic pollution results in bioaccumulation, demonstrating a toxic effect on individual organisms – which compounds through the process of biomagnification. Taken together, these consequences demonstrate that the impact of antibiotic pollution is extensive, causing harm to organisms, communities, and populations and their corresponding interactions. Thus, antibiotic pollution must be deemed a severe ecological problem.
Given the urgency and recency of this issue, interdisciplinary action is required. Scientists and politicians must collectively develop strategies to reduce antibiotic pollution, especially before the consequences worsen over time. One such strategy includes reducing the concentration of antibiotics that enter the water cycle – this can be accomplished by establishing policies which regulate the over prescription of antibiotics and the use of antibiotics in animal feed (Zeng et al., 2022). Furthermore, failures in effective water treatment have also contributed to the presence of antibiotics in environmental settings because it has been found that only about 50% of antibiotics are removed during wastewater treatment (Deblonde et al., 2011). To address this limitation, scientists and engineers can contribute by developing improved and “economically feasible” water treatment technologies (Zeng et al., 2022).
Overall, it is only through recognizing the severity of antibiotic pollution that its consequences can be addressed proactively. By taking immediate action, we can prevent a future in which the harmful effects of antibiotics are fully realized, thereby conserving planetary health.
Source: Woods Hole Oceanographic Institution, 2018
Works Cited
Columbia Environmental Research Center. (2021). Basic overview of the nitrogen cycle. https://www.usgs.gov/media/images/basic-overview-nitrogen-cycle
DeVries, S. L., & Zhang, P. (2016). Antibiotics and the terrestrial nitrogen cycle: a review. Current Pollution Reports, 2, 51-67. Fath, B. D. (2018). Encyclopedia of ecology. Elsevier. Gao, L., Shi, Y., Li, W., Liu, J., & Cai, Y. (2012). Occurrence, distribution and bioaccumulation of antibiotics in the Haihe River in China. Journal of Environmental Monitoring, 14(4), 1247-1254.
García, J., García-Galán, M. J., Day, J. W., Boopathy, R., White, J. R., Wallace, S., & Hunter, R. G. (2020). A review of emerging organic contaminants (EOCs), antibiotic resistant bacteria (ARB), and antibiotic resistance genes (ARGs) in the environment: Increasing removal with wetlands and reducing environmental impacts. Bioresource technology, 307, 123228.
Hodges, S. C. (2010). Soil fertility basics. Soil science extension, North carolina state university, 22.
Hutchings, M. I., Truman, A. W., & Wilkinson, B. (2019). Antibiotics: past, present and future. Current opinion in microbiology, 51, 72-80.
Kraemer, S. A., Ramachandran, A., & Perron, G. G. (2019). Antibiotic pollution in the environment: from microbial ecology to public policy. Microorganisms, 7(6), 180.
Kumar, K., Gupta, S. C., Chander, Y., & Singh, A. K. (2005). Antibiotic use in agriculture and its impact on the terrestrial environment. Advances in agronomy, 87, 1-54.
Larsson, D. J., & Flach, C. F. (2022). Antibiotic resistance in the environment. Nature Reviews Microbiology, 20(5), 257-269.
Levy, S. B. (2005). Antibiotic resistance—the problem intensifies. Advanced drug delivery reviews, 57(10), 1446-1450.
Mannion, A. M. (1998). Global Environmental change: the
causes and consequences of disruption to biogeochemical cycles. Geographical Journal, 168-182.
Mountain Empire Community College. (n.d.). Lesson 17: Sulfur. https://water.mecc.edu/courses/Env211/lesson17.htm
Roose-Amsaleg, C., & Laverman, A. M. (2016). Do antibiotics have environmental side-effects? Impact of synthetic antibiotics on biogeochemical processes. Environmental Science and Pollution Research, 23, 4000-4012.
Streit, B. (1998). Bioaccumulation of contaminants in fish. Fish ecotoxicology, 353-387.
Qiao, M., Ying, G. G., Singer, A. C., & Zhu, Y. G. (2018). Review of antibiotic resistance in China and its environment. Environment international, 110, 160-172.
Tang, J., Wang, S., Tai, Y., Tam, N. F., Su, L., Shi, Y., & Zhang, X. (2020). Evaluation of factors influencing annual occurrence, bioaccumulation, and biomagnification of antibiotics in planktonic food webs of a large subtropical river in South China. Water research, 170, 115302.
Zeng, H., Li, J., Zhao, W., Xu, J., Xu, H., Li, D., & Zhang, J. (2022). The current status and prevention of antibiotic pollution in groundwater in China. International Journal of Environmental Research and Public Health, 19(18), 11256.
Zhang, L., Qin, S., Shen, L., Li, S., Cui, J., & Liu, Y. (2020). Bioaccumulation, trophic transfer, and human health risk of quinolones antibiotics in the benthic food web from a macrophyte-dominated shallow lake, North China. Science of the Total Environment, 712, 136557.
Zhao, F., Yang, L., Chen, L., Li, S., & Sun, L. (2019). Bioaccumulation of antibiotics in crops under long-term manure application: Occurrence, biomass response and human exposure. Chemosphere, 219, 882-895.
The Bermans J. Iskandar Lab within the Department of Neurological Surgery at the University of Wisconsin Hospital has conducted novel research in central nervous system regeneration since 1997. The principal investigator, Dr. Iskandar, is a pediatric neurosurgeon who cares for children with brain and spinal cord injuries and disorders. His inspiration for studying central nervous system regeneration came with the desire to directly impact patient symptom management and potential recovery. Dr. Iskandar sees a lot of patients with neural tube defects like spina bifida – a spinal cord defect. It has been well known since the early 1990s that folic acid supplementation in pregnant women can help reduce the risk of neural tube defects (CDC, 2023). Motivated by these results, which imply that folic acid plays an important role in central nervous system (CNS) development, Dr. Iskandar’s lab discovered that folic acid can also enhance the ability of the adult CNS to regenerate injured axons.
We, the undergraduate research assistants in the Iskandar Lab, focus on in vitro neuronal cell cultures that aim to uncover this relationship between folic acid supplementation and CNS regeneration after injury (Iskandar et al, 2004). Like most scientific experiments, this type of culture requires extreme attention to detail and sterility, because at any point during the two-week process, the cellular environment can be contaminated with debris or infectious agents. Unfortunately, it was the latter that affected the success of the Iskandar laboratory endeavors between the years of 2022 and 2023. The purpose of this article is to share the story of how we identified and addressed this contamination, in the hope that readers will have an understanding and appreciation of sterile technique, attention to detail, and problem-solving skills.
In a research laboratory, contamination can lead to loss of time and money that affects the productivity of scientists, and it can spread through the air to vulnerable cell populations and laboratory personnel via aerosol generation (Malik et al. 2023). Because contamination can stem from any step of the culture process, detecting and containing the source is challenging. The chemical contaminants of primary concern are often residual detergents and fixatives, while the living contaminants of concern include viruses, bacteria, and fungi (Nims & Price 2017).
We became concerned about the presence of a contaminant when, despite strict adherence to sterility protocols and established procedures, the cultured samples exhibited repressed axonal growth,
Figure 1: Rat Dorsal Root Ganglion Culture. This culture was contaminated, producing only a small number of live neurons with no axonal growth. The image depicts circular and transparent contaminants, possibly fungi, in the middle, with a few neurons with no growth towards the bottom.
absence of neuronal cells, accumulation of debris, and the appearance of a yellowish hue in the culture solution (Fig. 1). We realized that this problem required a comprehensive and systematic study of each step of the culture process to identify the source of contamination, which we later realized to be a considerable task.
We first inspected all of the solutions used during the failed cultures. If any of the compounds were expired, they may lose their potency and no longer be useful for cell cultures; additionally, if contamination is present it could also have infiltrated any test tube or bottle used during the cultures. Any expired and unlabeled bottles were thrown out along with unexpired but open containers due to the possibility of contamination. New products were purchased, and fresh solutions were made. Next it was important to decontaminate every surface, tool, and device that was utilized. This was when the major source of contamination was found.
It was almost six months after the first failed culture, when fungus was discovered growing in the metal vent of the biosafety cabinet. What was supposed to be the cleanest part of the lab had been the source of major contamination. It was a relief to have finally found the cause of the major setback in the lab’s research.
Fungal contamination is challenging to eliminate, as the fungal organisms develop a protective layer called an endospore. These coatings are resistant to a variety of environmental conditions allowing the cells to remain inactive for many years – even with
attempts at disinfection. Once these cells are in a favorable environment such as a media solution, a chemical mixture of nutrients with a particular pH range needed for cells to thrive in, these fungi can grow rapidly (Shi XX, 2019). The relief of finding the pollutant was short-lived. The efforts to decontaminate the lab were just getting started.
We called Environmental Safety and prepared for a deep-clean of the biosafety cabinet. Meanwhile, every other part of the lab was cleaned as well, including: the incubator, warm water bath, vacuum system, and biosafety cabinet. All pipettes, surgical tools, beakers, and test tube holders were autoclaved – a machine that sterilizes equipment with water vapor at a high temperature. After all of the equipment was sterilized, the old solutions were discarded and all new solutions were made or purchased: B27, FBS, RPMI, Triton X-100, collagenase, dispase, DNAse, laminin, poly-D-lysine, PBS, PBST, and PFA. After decontaminating the lab equipment and solutions, numerous trial cell cultures were conducted to ensure the fungal contamination had been eliminated.
It would have been risky to start another culture before ensuring that we successfully decontaminated the culture set up, because of its costly process in time and money. Thus, after completing the decontamination, a practice culture was conducted (Fig. 2). The cultures showed a tremendous cell density as well as axon growth as seen by the fluorescence in the images.
Once we were able to prove that the contamination has been eliminated, we took steps to minimize the chance of future contamination by updating the lab protocol. Scientific research is a lifelong learning process that continues to be improved and updated. Although resources and time may have been exhausted, these contaminated cultures have served as an invaluable learning experience that strengthened our understanding of wet lab techniques and the process of neuronal cell cultures. As students in the lab, we had the opportunity to experience the scientific method by problem-solving every step of the way to identify the source of contamination. We would like to thank Ligia Papale, Anas Abou Merhi, Roy Chebel, Joyce Kouiek, and our Principal Investigator, Dr. Bermans Iskandar, for their time and continuous support throughout this process.
Centers for Disease Control and Prevention. (2023). Folic Acid Recommendations. Centers for Disease Control and Prevention. www.cdc.gov/ncbddd/folicacid/recommendations.html#:~:text=A%20number%20of%20studies%20showed,chance%20of%20 neural%20tube%20defects.&text=CDC%20recommended%20 that%20women%20who,planning%20to%20become%20pregnant%20again
Iskandar, B. J., Nelson, A., Resnick, D., Skene, J. H., Gao, P., Johnson, C., Cook, T. D., & Hariharan, N. (2004). Folic acid supplementation enhances repair of the adult central nervous system. Annals of neurology, 56(2), 221–227. https://doi.org/10.1002/ana.20174 Malik, P., Mukherjee, S., Mukherjee, T.K. (2023). Microbial Contamination of Mammalian Cell Culture. Practical Approach to Mammalian Cell and Organ Culture. https://doi-org.ezproxy.library.wisc. edu/10.1007/978-981-19-1731-8_5-1
Nims, R.W., Price, P.J. (2017). Best practices for detecting and mitigating the risk of cell culture contaminants. In Vitro Cellular & Developmental Biology.-Animal 53, 872–879. https://doi-org.ezproxy. library.wisc.edu/10.1007/s11626-017-0203-9
Shi XX, Qiu HP, Wang JY, Zhang Z, Wang YL, Sun GC. A handy method to remove bacterial contamination from fungal cultures. PLoS One. 2019 Nov 6;14(11):e0224635. doi: 10.1371/journal. pone.0224635. Erratum in: PLoS One. 2020 Jan 21;15(1):e0228293. PMID: 31693673; PMCID: PMC6834272. https://www.ncbi.nlm. nih.gov/pmc/articles/PMC6834272/
Abstract: This study investigates microplastic abundance in Glover’s Reef Marine Reserve, addressing the pressing issue of plastic pollution in marine environments. Motivated by the escalating production and non-biodegradable nature of plastics, this research focuses on microplastic abundance in sediment, sea cucumber fecal matter, and surface seawater. The hypotheses anticipate finding microplastics in all samples, with sediment exhibiting the highest levels of microplastics due to density-dependent accumulation. Statistical analyses, including Kruskal-Wallis and Mann-Whitney U tests, revealed significant differences in microplastic abundance among the three treatments (p < 0.001) with sediment exhibiting the highest number of microplastics (median = 11.1 microplastics/mL; SE = ± 3.40). These findings illustrate the pervasive nature of microplastics even in remote, protected marine areas and provide insight into the implications of trophic-level bioaccumulation trends and potential risks to human consumers of contaminated marine organisms. The research highlights the broader impact of human-induced pollution on marine ecosystems, emphasizing the need for mitigation strategies. Study limitations include sample size constraints and the potential for variation in microplastic dispersion among remote reefs. Overall, this research underscores the urgency of addressing plastic pollution in even protected areas, emphasizing the collective responsibility to adopt sustainable practices and reduce environmental threats.
Abstract: Studying in groups is found to facilitate learning and academic success. This report investigates the effectiveness of a behavior modification plan that applies the principles of associative learning to increase the time spent studying with friends or in public settings. The study uses a single-subject design with baseline and behavior modification phases. Results show an overall increase in the target behavior. However, limitations to the plan include a lack of control for extraneous variables in the test environment and the need to address other factors that may influence studying habits. Suggestions for improving the training program for future behavior modifications are discussed alongside personal experience . Overall, this study suggests that instrumental conditioning using positive reinforcement can be effective in promoting academic success and increasing social interactions.
Contributing
Researchers: Jenna Barad, Grace Charlson, Lucy Kennedy Edited by Ivy RayaAbstract
This study investigates microplastic abundance in Glover’s Reef Marine Reserve, addressing the pressing issue of plastic pollution in marine environments. Motivated by the escalating production and non-biodegradable nature of plastics, this research focuses on microplastic abundance in sediment, sea cucumber fecal matter, and surface seawater. The hypotheses anticipate finding microplastics in all samples, with sediment exhibiting the highest levels of microplastics due to density-dependent accumulation. Statistical analyses, including Kruskal-Wallis and Mann-Whitney U tests, revealed significant differences in microplastic abundance among the three treatments (p < 0.001) with sediment exhibiting the highest number of microplastics (median = 11.1 microplastics/mL; SE = ± 3.40). These findings illustrate the pervasive nature of microplastics even in remote, protected marine areas and provide insight into the implications of trophic-level bioaccumulation trends and potential risks to human consumers of contaminated marine organisms. The research highlights the broader impact of human-induced pollution on marine ecosystems, emphasizing the need for mitigation strategies. Study limitations include sample size constraints and the potential for variation in microplastic dispersion among remote reefs. Overall, this research underscores the urgency of addressing plastic pollution in even protected areas, emphasizing the collective responsibility to adopt sustainable practices and reduce environmental threats.
Plastic pollution is a pressing environmental issue as humans contribute 4.80–12.7 million metric tons of plastic to the ocean annually, and the production of plastic is increasing (Li et al., 2021). Plastic serves many societal functions: it’s cheap, easily produced, and versatile. However, plastic is non-biodegradable, accumulates in ocean gyres, and breaks into microplastics—defined as pieces smaller than five millimeters (National Ocean Service, 2021). Microplastics accumulate in organisms, causing detrimental effects to their health by disrupting vital biological processes; these pose threats to organisms’ growth and cause toxicological effects and genetic damage (Li et al., 2021).
Extensive research shows a high abundance of microplastics in marine environments (Carbery et al., 2018). For instance, a study on a creek in Regina, Sasskatchewan, Canada revealed 73.5% of fish and 95.6% of water contained microplastics (Campbell et al., 2017). This issue is concerning for marine ecosystems but also raises risks for humans consuming these organisms. Studies show microplastics have negative effects on human health similar to those found in marine organisms that consume microplastics, including cell damage, oxidative stress, inflammation, and energy depletion (Carbery et al., 2018).
This research explored microplastic abundance in sediment, Holothuria mexicana (sea cucumber), and surface seawater in the remote Glover’s Reef atoll in Belize. In the remote reefs of Palau, Béraud et al. (2022) found 12.71 ± 8.92 microplastics per gram in sediment, which led us to expect a similar presence at Glover’s Reef. The study aimed to contribute to a better understanding of microplastic presence in remote reef environments and identify trophic accumulation relationships. Microplastics are cycled and distributed through oceans via ocean gyres and other forces (Carbery et al., 2018), which lead us to anticipate that microplastics would be found in all the treatments: sea cucumber fecal matter, sediment, and seawater. It was hypothesized that sediment would contain the highest levels of microplastics due to their density-dependent behavior which causes the densest microplastics to accumulate on the ocean floor (Khuyen et al., 2021). Finally, it was predicted that sea cucumbers and sediment would exhibit similar amounts of microplastics, as sea cucumbers are indiscriminate filter feeders and directly ingest sediment microplastics from the sea floor (Coc et al., 2021). While it was expected to find microplastics within the fecal matter that was tested, it was assumed that trace amounts of consumed microplastics would remain in their intestines, and therefore there would be more microplastics in their bodies than we count.
It was hypothesized that (a) microplastics will be present in all samples, (b) sea cucumbers and sediment will contain similar levels, and (c) sediment will contain more microplastics than the surface water. Our research findings have significant implications as they confirm even remote areas are not immune to the consequences of human plastic use. Understanding tro-
phic-level relationships of microplastic ingestion can provide insight into how bioaccumulation affects organisms, and the risks to humans who commonly consume them in many Asian and Middle East countries (Bordbar et al., 2011).
Methods
The study site was located within the Glover’s Reef Marine Reserve, approximately 45 km off the coast of Belize. The atoll spans 32 km in length, 12 km in width, and covers an area of 35,000 hectares (Wildlife Conservation Society, 2021). The research was conducted at Glover’s Reef Research Station on Middle Caye which has over 800 patch reefs with sandy bottoms, seagrass beds, and average water depths ranging from sea level to about 12 meters (ibid). The data collection was from July 22nd to 26th, 2023.
Eight sediment samples two meters from the shore were collected using a random number generator between 1 and 100 to calculate the number of paces taken around the island between each collection. A 20 mL vial was used to scoop up 10 mL of sediment per area sampled.
Eight sea cucumbers were collected alive, placed in a tank, and once they defecated, were released back into the ocean without harm. Two sea cucumbers were collected from each of four separate, patch reefs that were closest to the island dock. Every other sea cucumber seen at each reef was collected. They were transported back to land in mesh bags by snorkeling. Once on land, they were transferred into a saltwater tank until they naturally deposited enough fecal matter for collection. Subsequently, 10 mL of formed fecal matter was collected from each sea cucumber, and the sea cucumbers were then released back into the ocean.
Seven surface seawater samples were collected using an 80-micron plankton net. The net was towed for five minutes at a measured distance around the atoll, following a method adapted from Jeyasanta et al. 2020. Each sample was collected in a 150 mL container that accounted for a larger volume of surface seawater.
Organic matter was separated from all 10 mL samples using the hydrogen peroxide method, adapted from the Coastal and Marine Geology Program (Coastal and Marine Geology Program, 2002). The procedure for separation of organic matter and filtration of sediment and fecal matter samples was conducted as follows: 10 mL of sample was measured out into graduated cylinder and 25 mL of 3% hydrogen peroxide was added and stirred with a glass rod. After the bubbling stopped, another 25 mL of 3% hydrogen peroxide was added and restirred until the addition of hydrogen peroxide didn’t cause bubbling anymore, indicating that the matter was removed. The suspension was allowed to settle and then was siphoned or poured off the supernatant liquid. The sample was suspended in the graduated cylinder by swirling it around and simultaneously using a syringe to collect 10 mL of the sample and hydrogen peroxide mixture. A 0.8-micron piece of filter paper was then placed in the Millipore Filtration Unit, and the
10 mL mixture was poured into the filtration unit and pumped until it was dry (Coastal and Marine Geology Program, 2002). Using a similar procedure, the organic matter was separated and filtered out of the surface seawater samples and was conducted as follows: 150 mL water sample was suspended in the graduated cylinder by swirling it around and simultaneously used a syringe to collect 10 mL of the sample. A 0.8-micron filter paper was placed in the Millipore Filtration Unit and the 10 mL water sample was poured into the filtration unit. 12.5 mL of 3% hydrogen peroxide was added and stirred with a glass rod, and after the bubbling stopped. Another 12.5 mL of 3% hydrogen peroxide was added and restirred. When addition of hydrogen peroxide didn’t cause bubbling anymore, the matter was removed and allowed to settle. This was siphoned or poured off the supernatant liquid and the filtration unit was pumped until the sample was dry (Coastal and Marine Geology Program, 2002).
Microplastics in each sample were counted under a dissecting microscope by two to three observers. The counts obtained by each observer were averaged to determine the final microplastic number. Microplastics were distinguished from other particles according to the identification guide from Sartain et al. (2021).
As the sample sizes and methods varied for each treatment, the data was calculated to have the final units of microplastics per mL. The process for converting the measurements to microplastics per mL for the sediment and fecal matter was as follows:
The data for the surface seawater was calculated as follows:
In the equation, r = radius of plankton net in cm, ℓ = length of plankton net tow in cm, and x100 to account for differing filter sizes of the 80-micron plankton net versus the 0.8-micron filter paper used in the separation. Finally, the median number of microplastics per mL per category were compared using both a Kruskal-Wallis Rank Sum Test and a Mann-Whitney U Test.
The median number of microplastics per mL found in sediment was 11.1 ± 3.40 (n = 8). The variation in the sediment samples were relatively high compared to the other treatments due to an outlier on the upper end. For sea cucumber fecal matter, the median number of microplastics per mL was 6.0 ± 0.941 (n = 8) (Fig. 1). Finally, the median number of microplastics per mL found in surface seawater was 0.0009 ± 9.25E-05 (n = 7) (Figure 1). Due to limited time and resources, the sample sizes were only 8 for each treatment, therefore non-parametric statistical measures were used to analyze the data. A Kruskal-Wallis test was performed to compare
the number of microplastics per treatment (p < 0.001, df = 2). Finally, a Mann-Whitney U test was performed on the sea cucumber fecal matter and the surface seawater data (p < 0.001, df = 1).
Figure 1: The median number of microplastics per milliliter in samples of sediment (11.1; SE = ± 3.40), sea cucumber fecal matter (6.0; SE = ± 0.941), and surface seawater (0.0009; SE = ± 9.25E-05). A, b, and c are statistically distinct from each other.
Discussion
Microplastics were present in all samples of sediment, sea cucumber feces, and surface seawater collected in the Glover’s Reef Marine Reserve, a remote atoll 45km from the Belizean mainland. The amount of microplastics varied; sediment samples contained the highest microplastic abundance (Fig.1) which could be attributed to the microplastics’ density and tendency to sink and accumulate (Khuyen et al. 2021). Sea cucumbers likely ingested microplastics via filter feeding on sediment (Coc et al. 2021) and excrete them in their feces. Surface seawater showed the lowest microplastic content, as many microplastics sink, but those that are less dense than seawater remain cycling in the water column (Khuyen et al. 2021). Further research into the behaviors of microplastics of varying masses and densities may offer further insight into these accumulation patterns.
We recommend further investigation into the presence of microplastics in remote reefs, and how this may be related to ocean currents. The number of microplastics may be overrepresented compared to that of other remote reefs due to these ocean currents, which have been found to cause high rates of dispersion of microplastics far from their source (Martinez et al., 2009). We observed firsthand evidence of this phenomenon as we saw piles of plastic washed up on shore around the island, none of which were from its inhabitants.
The accumulation of microplastics poses evident harm to marine environments and organisms, although research on the long-term effects of microplastics on humans remains limited (Shemitz and Anastas, 2020). This research highlights trophic-level accumulation trends in sea cucumbers, offering insight into potential negative impacts on their habitats and the organisms that interact with them. As microplastics are
further integrated into marine ecosystems through trophic accumulation, potential harm to humans consuming marine organisms is of concern as well. Additionally, our findings underscore how even protected and remote areas are not immune to human-induced pollution, emphasizing the broader impact humans have on marine ecosystems and ultimately, on ourselves. Microplastics present hidden threats, necessitating further research such as studies focused on how the circulation of microplastics in the ocean results in differing levels of accumulation in places around the world. This could in turn present opportunities to target areas producing this plastic. To comprehend their true effects on marine environments and human health, further research is necessary to explore how microplastics behave in the human body and provide more information about the long-term effects of microplastic ingestion. Exploring solutions for plastic cleanup is also essential to address this issue, as human production of plastic continues to rise, and our oceans are filling up with floating islands of plastic like the Great Pacific Garbage Patch, which not only directly kills marine life but also indirectly harms them by being a source of microplastics (Lebreton et al., 2018).
This study highlights the widespread danger of microplastics that are found to be present in remote areas such as our study site off the coast of Belize. Our statistically significant results illustrate potential trophic-related accumulation patterns of microplastics in marine life which is worrisome as marine life is a source of food for many people. These bioaccumulated microplastics make their way into our bodies and cause significant health issues. To help combat this problem, a collective effort is crucial to stop the spread of plastics at the source. Simple actions like reducing individual plastic water bottle usage, spreading awareness of plastics’ detrimental effects, and supporting and voting for environmentally conscious candidates who call for and support federal water pollution control acts, clean water bills, and education outreach can make a significant difference. Every small change contributes to the greater cause, making it imperative that we act to protect our planet and its fragile ecosystems.
We would like to acknowledge Catherine Woodward, Ph.D., Joe Meisel, Ph.D., and Teal Guetschow, Ph.D. candidate, whose instruction and support were instrumental in making this research possible. We also extend our thanks to the staff at Glover’s Reef Marine Reserve for their hospitality and Adrian Martinez for his assistance in the field.
References
Béraud, E., Bednarz, V., Otto, I., Golbuu, Y., Ferrier-Pagés, C. (2022). Plastics are a new threat to Palau’s coral reefs. PLOS ONE, 17(7):1-16.
Bordbar, S., Anwar, F., & Saari, N. (2011). High-value components and bioactives from sea cucumbers for functional foods—a review. Marine Drugs, 9(10), 1761–1805.
Campbell, SH., Hall, BD., Williamson, PR. (2017). Microplastics in the gastrointestinal tracts of fish and the water from an urban prairie creek. FACETS, 2:395-409.
Carbery, M., O’Connor, W., Thavamani, P. (2018). Trophic transfer of microplastics and mixed contaminants in the marine food web and implications for human health. Environmental International, 115:400-409.
Coastal and Marine Geology Program. (2002). Removal of organic matter with hydrogen peroxide. Retrieved on 11/17/2022 from https://pubs.usgs.gov/of/2001/of01-041/htmldocs/methods/h2o2.htm.
Coc, C., Rogers, A., Barrientos, E., Sanchez, H. (2021). Micro and macro plastics analysis in the digestive tract of a sea cucumber (Holotcostalhuriidae, Holothuria floridana) of the Placencia Lagoon, Belize. Caribbean J. Of Science, 51:166-174. Jeyasanta, K.I., Patterson, J., Grimsditch, G., Edward, J.K.P. (2020). Occurrence and characteristics of microplastics in the coral reef, sea grass and near shore habitats of Rameswaram Island, India. Marine Pollution Bulletin, 160(2020):1-16.
Khuyen, V., Le, D., Fischer, A., Dornack, C. (2021). Comparison of microplastic pollution in beach sediment and seawater at UNESCO Can Gio Mangrove Biosphere Reserve. Global Challenges, 1(11).
Lebreton, L., Slat, B., Ferrari, F., Sainte-Rose, B., Aitken, J., Marthouse, R., Hajbane, S., Cunsolo, S., Schwarz, A., Levivier, A., Noble, K., Debeljak, P., Maral, H., Schoeneich-Argent, R., Brambini, R., & Reisser, J. (2018). Evidence that the Great Pacific Garbage Patch is rapidly accumulating plastic. Scientific Reports, 8(1).
Li, Y., Sun, Y., Li, J., Tang, R., Miu, Y., Ma, X. (2021). Research on the influence of microplastics on marine life. Earth and Environmental Science, 631(012006). Martinez, E., Maamaatuaiahutapu, K., Taillandier, V. (2009). Floating marine debris surface drift: Convergence and accumulation toward the South Pacific subtropical gyre. Marine Pollution Bulletin, 58:1347–1355. National Ocean Service. (2021). What are microplastics? Retrieved on 11/17/2022 from https://oceanservice.noaa.gov/ facts/microplastics.html.
Sartain, M., Wessel, C., Sparks, E. (2021). Microplastics sampling and processing guidebook. Retrieved on 07/19/2023 from http://extension.msstate.edu/publications/microplastics-sampling-and-processing-guidebook.
Shemitz, L., & Anastas, P. (2020). Yale experts explain microplastics. Retrieved 11/18/2022 from https://sustainability.yale. edu/explainers/yale-experts-explain-microplastics. Wildlife Conservation Society. (2021). Glover’s Reef Research Station. Retrieved on 11/17/2022 from https://gloversreef.wcs. org/About-Glovers-Reef.aspx.
Abstract
Studying in groups is found to facilitate learning and academic success. This report investigates the effectiveness of a behavior modification plan that applies the principles of associative learning to increase the time spent studying with friends or in public settings. The study uses a single-subject design with baseline and behavior modification phases. Results show an overall increase in the target behavior. However, limitations to the plan include a lack of control for extraneous variables in the test environment and the need to address other factors that may influence studying habits. Suggestions for improving the training program for future behavior modifications are discussed alongside personal experience . Overall, this study suggests that instrumental conditioning using positive reinforcement can be effective in promoting academic success and increasing social interactions.
Introduction
Associative learning is a type of learning where an individual develops a connection between two or more stimuli or between a behavior and its consequences. This process occurs when an individual learns to associate a specific behavior with a particular outcome. Applied behavior modification refers to the use of these principles of associative learning to modify or change an individual’s behavior to achieve a targeted goal or outcome.
Time spent studying with friends or in groups is an area where associative learning principles can be applied. Research shows that students who study in groups tend to perform better academically than those who study alone (Johnson & Johnson, 2014). This can be attributed to the concept of social facilitation, which is the tendency for individuals to perform better on tasks when in the presence of others (Zajonc, 1965). In addition to social facilitation, studies also show that collaborative learning can lead to the development of higher-order thinking skills such as critical thinking, problem-solving, and decision-making (Slavin, 2014). Such outcomes are due to the ability of group members to provide varying perspectives, insights, and experiences that may not be considered otherwise.
Taking from personal experience at the University of Wisconsin-Madison, studying amongst my peers did not take away from my educational quality, but rather allowed me to participate in stimulating and motivating conversations
that supported my performance throughout the school year. However, at the National University of Singapore, I was not able to construct a similar study environment for myself, as it took time to adapt to the new environment. At the start of the semester, having newly moved to Singapore, I spent most of my time in my room, only leaving my hall to attend classes every other day or two. When alone in my room, I used to get distracted by my phone or tempted to sleep instead of study; a possible reason being that my sleeping space was in close proximity to my study space. Such a lifestyle was not productive for my academic performance. Research also shows that studying in isolation could lead to procrastination and lack of motivation because, without the support and accountability of others, one may find it easier to postpone studying or engage in other distracting activities (Steel, 2007). Hence, in order to avoid such outcomes, I chose to focus on managing behavior associated with the amount of time spent outside of my room to improve my productivity. The amount of time spent studying outside my room – be it on campus, in common rooms or lounges of my hall, or elsewhere in the city – was logged and analyzed as the baseline (control) dataset of this report. However, after recording the baseline data, I noted that lecture/tutorial time did not necessarily involve input from me, nor did it involve interaction with other students or peers; additionally, my class schedule was set up by the university and could not be modified. Therefore, I chose to limit my target behavior to the time outside of lectures that I could control.
The control data (baseline time spent studying daily outside of my room) indicated that the availability of a study partner or conducive environments such as available space in libraries, or common lounge areas were the antecedents (the stimuli) that triggered the target behavior. The consequences of studying with friends were the successful completion of assignments which contributed to academic success, and positive social interaction through activities like playing games, watching movies, and/or outings. Therefore, the aim of the proposed behavioral modification plan was to increase the time spent studying with friends outside the room to promote academic success and enhanced social interaction.
The target behavior of studying with friends outside the room was recorded for three weeks while implementing the following Behavioral Modification Plan.
Step 1: Establishing a baseline
The first week was regarded as the baseline or control. The amount of time spent studying outside of the room every day for one week was recorded as a reference point for the target behavior. The baseline served as the starting point to determine the progress throughout the behavior modification plan. No specific procedures were implemented during this week other than the logging of time spent studying outside of the room.
Step 2: Determining reinforcement types
Positive reinforcement is found to be effective in increasing a desired behavior (Diedrich, 2010; Piazza et al. 2011). Hence, rewards (study breaks, extracurricular activities, snack breaks) were implemented when studying amongst peers was increased. Time spent studying in lectures or tutorials was not reinforced with intermittent breaks to control for compulsory learning as opposed to actively choosing to study.
Step 3: Establishing schedules of reinforcement
Continuous reinforcement schedules are considered more effective for developing a strong association between the behavior and the response because they provide consistent motivation to continue engaging in a behavior (Cherry, 2023). A continuous schedule of reinforcement was implemented in the first experimental week following the control week to establish a regular study session with peers every evening after meal time. The reward for consistent, focused studying was a break every evening in which I played board games in the lounge or a snack break for every 2 hours of studying with peers. For longer study sessions over the weekend (a total of more than 3 hours), I rewarded myself with a longer duration break in which I and my peers watched a movie or went for planned outings. Once a consistent behavior pattern was created within the first experimental week, a fixed ratio schedule was implemented in the second experimental week to help maintain the behavior consistently over time. I rewarded myself with extracurricular breaks or food breaks only on days when I studied with peers for 4 or more hours than the control week.
Step 4: Targeting motivation mechanisms
Intrinsic motivation was targeted through the reinforcement of positive triggers and emotions associated with enjoyment in the form of games played after every study session. Active reminders of personal satisfaction after successfully completing an assignment were introduced. Furthermore, study engagement alongside peers from my classes who were working on the same assignments created a sense of healthy competition and accountability to others.
Step 5: Implementing shaping
Shaping (a conditioning paradigm used to increase a targeted behavior through successive approximation-based reinforcement) was used to break down study tasks into smaller, more achievable time goals that gradually increase in duration when studying with friends or in public settings. The goal for the first experimental week was to study for 2 hours more
per day than the control week with friends or at a library. The goal for the second experimental week was to study at least 4 hours more than the control week with friends or in a public setting.
Step 6: Contiguity and contingency management
In experimental week 1, rewards in the form of games or snack breaks were provided after every 2 hours of continuous studying in the evenings. Hence, close contiguity was maintained through continuous reinforcement. This helped strengthen the association between the behavior and the reinforcer. Furthermore, the fixed-ratio schedule in experimental week 2 was implemented to ensure contingency as the target behavior was only reinforced for 4 or more hours of studying with friends, not less.
In order to prevent habituation and generalize the behavior across different settings, during experimental week 2, studying took place at different locations on campus including but not limited to libraries, student lounges, and the common study room of my hall. I also switched up the peers that I studied with from my hall, class group, and outside of class. The change in study environments and people ensured that the target behavior did not remain context-specific.
The baseline control week data indicated that the average time spent outside of the room was 3 hours per day, while the average time spent studying with friends was 1.57 hours per day. Results show a significant dip on day 1 (Fig. 1) where no social interaction was logged at all, as I spent the entire day in my room. The behavioral modification data is suggestive of a general trend toward an increase in time spent outside the room as demonstrated in the cumulative record of the behavior (Fig. 1). The variability of high and low peaks in Fig. 1 is likely due to the lecture/tutorial hours which varied based on the class and week (one class did not have tutorial during experimental week 1 of data collection). Since the behavior modification plan only included reinforcement of time spent studying with friends or in public settings and not lecture or tutorial, the cumulative record of the time spent studying with friends (Fig. 2) suggests the effectiveness of the plan in increasing the target behavior.
The goal for the first experimental week in which the behavioral modification plan was implemented was to increase the average time spent studying with friends by 2 hours per day. Fig. 3 suggests an overall increase of 1.57 hours spent outside the room between the control week and experimental week 1, and Fig. 4 suggests an overall increase of 1.86 hours studying with friends per day. This results in a new average of 4.57 hours spent outside the room per day and 3.43 hours spent studying with friends per day.
The goal for the second experimental week was to study with friends for a minimum of 4 or more hours per day relative to the control week . The average time spent outside the room in the second experimental week was 6.71 hours per day,
1: Cumulative graph of the time spent outside the room every day for 3 weeks
Figure 2: Cumulative graph of the time spent studying with friends (i.e. excluding lecture/tutorial time) every day for 3 weeks
while the average time spent studying with friends in the second experimental week was 5.29 hours per day. This indicated an overall increase of 3.71 hours spent outside the room per day and 3.72 hours spent studying with friends per day, both relative to the control. The results for experimental week 2 also suggest an increase of 2.14 hours spent outside the room per day and 1.86 hours spent studying with friends from the previous week (experimental week 1).
Discussion
The results suggested that the behavior modification plan was effective in increasing the time spent studying with friends or in public settings. There was an overall increase in the target behavior, as evidenced by the growing trend in the cumulative record graphs (Fig. 1-2). The goal of increasing the time spent studying with friends by 2 hours per day in the first experimental week was achieved, as indicated by the overall increase in Fig. 3 and Fig. 4. Furthermore, the goal of studying with friends for at least 4 or more hours a day compared to the control week in the second experimental week was also achieved, as evidenced by the increase in time spent outside the room and studying with friends in Fig. 3 and Fig. 4.
The outcomes of the behavior modification plan thus aligned with the principles of associative learning theory which posits that behaviors are learned through the association of antecedents and consequences. In this case, the plan targeted the antecedent of spending time in public settings or with friends and provided positive reinforcement in the form of different rewards. The use of positive reinforcement, which is a well-established strategy in behavior modification, evident-
3: Weekly comparison of the time spent outside the room
Figure 4: Weekly comparison of the time spent studying with friends (excluding the time spent in lecture/tutorial)
ly increased the frequency of the target behavior, particularly when the rewards were delivered consistently and immediately following the behavior (Fig. 4). Additionally, the effectiveness of shaping, or reinforcing successive approximations of the desired behavior, was evident in the results of the behavior modification plan. The approach allowed for small, achievable steps to be taken toward the ultimate goal of increasing the study time with friends outside the room, which may have contributed to the success of the plan.
However, the training program or plan used in this study had its limitations. Playing the double role of subject and experimenter limited me to use fixed-ratio reinforcement. I could not have made the rewards unpredictable as my own experimenter to implement a variable-ratio schedule, which could have resulted in a steadier pattern of responding and reduced reliance on rewards (Crossman et al., 1987, as cited by Domjan, 2014). This could possibly explain the sudden dip observed in experimental week 2 (Fig. 4), likely due to the predictable lack of reinforcement when the desired behavior was not exhibited. Moreover, the plan was designed for a specific individual and my unique set of antecedents, consequences, and motivations; it may not be effective or appropriate for other individuals with different antecedents or motivations. Extraneous variables such as study skills, studying habits, and exam scores could further influence the target behavior in other individuals’ cases. These external factors could also possibly contribute to the averages being slightly less than the set goals.
Future training programs could therefore be improved by including a control group if the study is being conducted
Figure Figurewith multiple participants to account for external factors and ensure that the results are valid and reliable, as self-reported data is usually subjected to bias. Furthermore, positive feedback has been found to increase the frequency of desired behaviors (Wofford & Goodwin, 1990). Even though I was unable to provide feedback to myself, future experimenter-facilitated studies could utilize feedback to better reinforce the target behavior. Nevertheless, building on the strengths of this study, it is clear that the implementation of a structured behavior modification plan can have a significant positive impact on studying habits. By clearly demonstrating the effectiveness of consistent rewards and setting achievable goals, this report provides a valuable framework for individuals looking to enhance their academic performance through disciplined and socially engaging study routines.
References
Cherry, K. (2023, March 13). How Schedules of Reinforcement Work. Verywellmind.com. Retrieved April 4, 2023, from https://www.verywellmind.com/what-is-a-schedule-of-reinforcement-2794864
Diedrich, J. L. (2010). Motivating Students Using Positive Reinforcement [Thesis]. In Published. https://soar.suny.edu/ handle/20.500.12648/5977
Domjan, M. P. (2014). The principles of learning and behavior. Cengage Learning.
Gable, R. A., Hendrickson, J. M., & Strain, P. S. (1978). Assessment, Modification, and Generalization of Social Interaction Among Severely Retarded, Multihandicapped Children. Education and Training of the Mentally Retarded, 13(3), 279–286. Johnson, D.W., Johnson, R.T. and Smith, K. (2014) Cooperative Learning: Improving University Instruction by Basing Practice on Validated Theory. In: Davidson, N., Major, C. and Michaelsen, L., Eds., Small-Group Learning in Higher Education: Cooperative, Collaborative, Problem-Based and Team-Based Learning. Journal on Excellence in College Teaching, 25(4). http://personal.cege.umn.edu/~smith/docs/Johnson-Johnson-Smith-Cooperative_Learning-JECT-Small_Group_Learning-draft.pdf
Piazza, C. C., Roane, H. S., & Karsten, A. (2011). Identifying and enhancing the effectiveness of positive reinforcement. Handbook of applied behavior analysis, 151-164. Slavin, R. E. (2014). Cooperative Learning and Academic Achievement: Why Does Groupwork Work?. [Aprendizaje cooperativo y rendimiento académico: ¿por qué funciona el trabajo en grupo?]. Anales de Psicología, 30(3), 785–791. https://doi.org/10.6018/analesps.30.3.201201
Steel P. (2007) The nature of procrastination: a meta-analytic and theoretical review of quintessential self-regulatory failure. Psychol Bull,133(1):65-94. doi: 10.1037/0033-2909.133.1.65. PMID: 17201571.
Wofford, J. C., & Goodwin, V. L. (1990). Effects of feedback on cognitive processing and choice of decision style. Journal of Applied Psychology, 75(6), 603–612. https://doi. org/10.1037/0021-9010.75.6.603
Zajonc, R. B. (1965). Social facilitation. Science, 149(Whole No. 3681), 269–274. https://doi.org/10.1126/science.149.3681.269
https://justjournal.club/
The Journal of Undergraduate Science and Technology (JUST) is an interdisciplinary journal for the publication and dissemination of undergraduate research conducted at the University of Wisconsin-Madison. Encompassing all areas of research in science and technology, JUST aims to provide an open-access platform for undergraduates to share their research with the university and the Madison community at large.
Submit your research below to be featured in an upcoming issue!