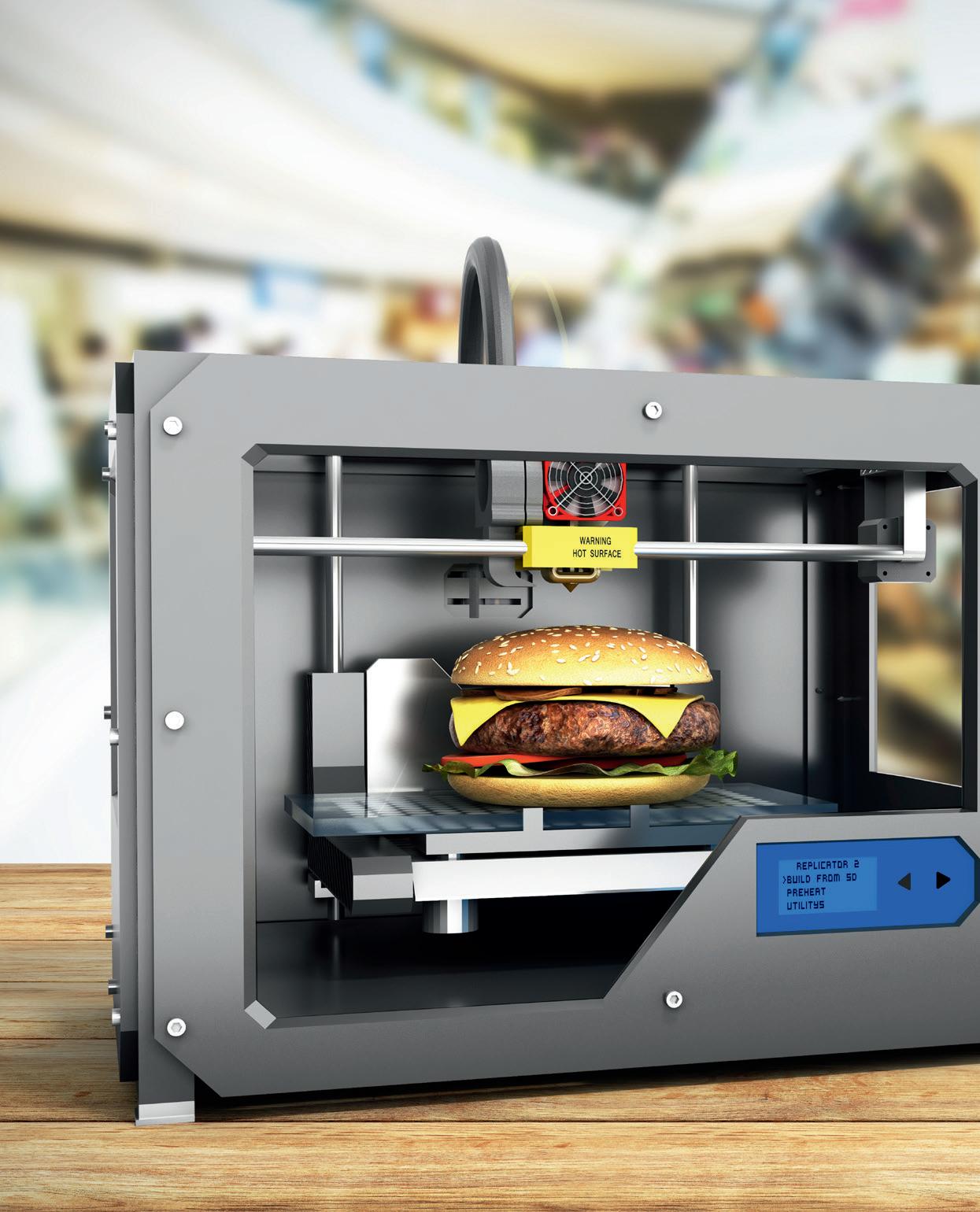
26 minute read
3D printing: Will we 3D print the bread of the future?
from f2m Automation Book
by landmagd
© nosorogua – stock.adobe.com
Will we 3D print the bread of the future?
There are numerous challenges using a 3D printer to produce bread, from adapting the recipe to reproducing its texture and pore distribution. The aim is to have the resulting product taste and smell like bread. To achieve this, the 3D printing process offers the advantage of its ability to react flexibly to all requirements by individually adapting the different process steps. Here are the technical requirements and the current state of research in the field.
+The manufacturing process of 3D printing has already established itself in various industries over the last few years. In the food sector, however, the application is still in Research and Development. The innovation potential in the food industry is considered to be high and the number of scientific publications has increased disproportionately over the last few years, from 19 in 2010 to 129 in 2015 and 821 in 2020 (sciencedirekt.com) (R. Derossi et al., 2021). In this context, 3D printing technology combines mechanical manufacturing with digital processing, which enables the rapid and conflict-free customization of products. The created objects are transmitted to the 3D printer using appropriate software, which then builds them layer by layer. This allows both the shape and the interior of the printed object to be customized. For example, new geometries, unusual textures and customized nutrient contents can be made possible, thus also reducing food waste (Godoi et al., 2016).
The challenges of producing bread with a 3D printer are quite different. First, the recipe of the dough must be adapted so that it can be printed. The viscoelastic properties of the dough play a decisive role here. Then the different textures of the bread (crumb and crust) must be reproduced in a product-typical manner by means of suitable product design and post-processing. Attention must be paid to the different pore distributions as well as to the different texture impressions between elastic (especially in the crumb) and firm (especially in the crust). Crucial for this is above all a heating step that can be integrated into the 3D printing process in order to stabilize the printing mass after printing and also to improve its digestibility. Finally, it is of course also important that the bread from the 3D printer tastes and smells like real bread. In conventional bread, the aroma is primarily formed by the fermentation of yeast and/or sourdough as well as the formation of roast aromas during baking (Pico et al., 2015). As the use of yeast and other leavening agents in a pressurized process is currently not possible, as this would change the properties of the pressurized mass over time, the aroma must be added elsewhere or created through post-processing.
A decisive advantage of the 3D printing process is, as already mentioned above, the ability to react flexibly to all requirements by individually adapting the different process steps. It is important to understand the individual process steps of the 3D printer in order to be able to make the desired settings. If each distinct area of food 3D
Figure 1: Schematic representation of the process steps in 3D printing printing is mastered, new and innovative products can be created that revolutionize the food industry.
Printing process
Among the various printing processes used in additive manufacturing, Fused Deposition Modeling (FDM) has so far been best established in the food sector. In this process, a printing compound is extruded through a nozzle and deposited onto the previous layer by the movement of the print head (Crump, 1992). In this process, the printing material is stored in a reservoir and conveyed through the nozzle by a feed mechanism. The process, originally developed for plastics, metals and ceramics, is ideally suited for printing foodstuffs. The most important prerequisite for the printing material is that it can be extruded. The 3D printing process can also be divided into five steps (see Figure 1), all of which must be controlled and managed to achieve the desired end product. It should be noted that each of these steps is influenced by the others and also influences them itself. A change at the beginning results in a correction in all other steps.
The 3D printing process begins with the production of the printing materials, the pre-processing. The individual recipe components are mixed with an agitator or kneader and placed in a storage container, usually a cartridge or syringe, which is then connected to the 3D printer. In this process step, it is important that the rheological properties of the printing materials are adjusted. When selecting the food ingredients and also, above all, when dosing the liquid, care must be taken to ensure that they meet the rheological requirements of the printing process. Once the printing compound has been prepared and the cartridge connected to the 3D printer, the actual printing process can begin. This section is divided into the extrusion and the deposition of the printing material. Extrusion here simply refers to the transport of the printing material through the nozzle opening. In the extruder of the 3D printer, the printing material is neither mixed nor textured yet. In the extrusion process, it is crucial which forces act and thus the flow properties of the printing materials are changed.
The volume flow rate as well as the nozzle diameters are the most important factors (Fanli Yang et al., 2019). Once the printing material has passed the nozzle tip, it is deposited onto the printing plate or onto the already printed layers (deposition). During this process, the print head follows the paths previously created on the
Printing process
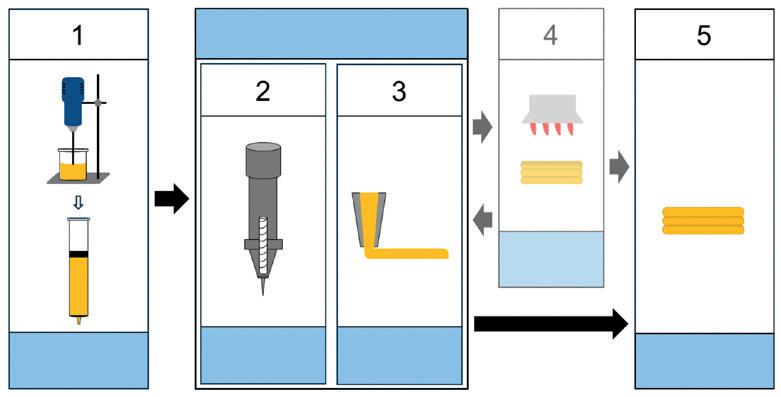
Pre-processing Extrusion Deposition
Postprocessing
End product
computer that result in the desired object shape and infill structure. For an exact print image, it is important that the print material bonds with the previous layer. For a good bond, the properties of the printing materials are crucial (Fahmy et al., 2020a; Li et al., 2017). To increase the stability of the printed objects, another processing step can be added after printing. Post-processing can be used to stabilize during the printing process as well as to finalize the printed objects. It usually involves thermal post-processing such as freezing, baking or even freeze-drying (He et al., 2019). The arrows in Figure 1 indicate that stabilization can also occur during the 3D printing process, e.g., after each printed layer. This increases the possibility of influencing the texture of the printed object. At the end of the printing process, there is the finished printed object, which has all the digital properties, which were set at the beginning by means of digital models on the computer and suitable material selection. The deviations from these specifications determine the success of the food 3D printing. Apart from the individual process steps, there are further possibilities of influencing the printing process by changing the printer settings. Alternatively, the accuracy (resolution) of the print can be improved by selecting the nozzle diameter and the layer height. A reduction of both para-meters usually not only leads to an improvement of the printing accuracy, but also to an increase of the printing time. However, this can be counteracted by adjusting the printing speed. For a good printing image it is necessary to find the optimal settings.
The FDM printing process with its individual process steps is also suitable for the production of bread. The combination of extrusion and postprocessing can enable complex printed objects with unique textures. However, the scientific literature so far has mainly printed pastries such as biscuits and cookies (Kim et al., 2019; Fan Yang et al., 2018) or doughs with a high-fat content (A. Derossi, Caporizzi, Paolillo, et al., 2020). Thus, the challenge for future research is to develop bread dough suitable for the 3D printing process. Several prerequisites, which will be discussed in the next section, need to be considered.
Material conditions
In order to be able to print foodstuffs, certain rheological requirements must be met. Firstly, they must be extrudable. This means that, on the one hand, the consistency of the printing compound must be soft enough to print through a nozzle. On the other hand, it must also be firm enough to retain its shape after printing to minimize deformation (Q. Liu et al., 2020). To meet these conflicting properties, the rheological behavior of the printing materials must be precisely adjusted to the printing process.
The extrudability of the material enables the shear-thinning property of many food products. As described in the last section, the printing ink is pressed through the die during the printing process, which subjects it to strong forces (Fanli Yang et al., 2019). However, these forces cause the viscosity of the shear-thinning materials to decrease, making them printable. The reason for this is that the internal structure of the printing materials changes greatly and the individual particles or polymers can slide past each other more easily. This partial destruction is an advantage during extrusion, but weakens the stability of the printing material after it has been deposited on the printing platform or on the previous layer. The internal structure must therefore recover as quickly as possible after leaving the nozzle tip so that the viscosity increases again and provides the necessary stability. This rheological behavior is called thixotropy and describes the decrease in viscosity over time due to external influences and the return to the initial viscosity only after the stress has ended. In addition, a complete regeneration of the internal structure should take place if possible in order to enable the best possible stability of the printed objects. This can be controlled by the viscosity and the yield point of the printing materials. To prevent the print objects from spreading, these two parameters must be high enough (Paxton et al., 2017).
In order to be able to print bread or bread-like products in the 3D printer, the bread dough must be simplified. Due to the various ingredients and processing steps during production, the
Figure 2: 3D printing of a starch-protein mixture of wheat starch and soy protein isolate. a) Printing of a cube (edge length: 1.5 cm), b) Water leakage from the extruder due to phase separation of the printing mass during the printing process conventional dough is subject to change over time. The yeast in particular changes the dough properties enormously by expanding the gas pores during proofing (Alpers et al., 2021). These temporal changes are unsuitable for 3D printing, as the dough properties are specifically adjusted to the printing process at the beginning. Therefore, 3D printing requires the omission of ingredients such as yeast, which are essential for traditional bread production. The main components of bread dough are water and flour. By combining these two raw materials in the right proportions, a printable dough can already be produced (Fahmy et al., 2020a; Severini et al., 2016). The addition of water in those doughs made from wheat flour varies from 50g to 80g H2O/100g of flour. In this regard, Fahmy et al. demonstrated that different water contents of the doughs have a strong influence on the printing accuracy. The higher the water content, the more unstable the printed geometries. Furthermore, it was found that the properties of the gluten network strongly influence the 3D printing process. On the one hand, gluten serves as a stabilizing element due to its network formation, which provides an improved printed image especially at a high water content. On the other hand, the elastic behavior of the network causes the dough mass to be ‘pulled along’ by the movements of the print head, especially at a low water content, which decisively deteriorates the printing accuracy (Fahmy et al., 2020a). In order to circumvent this complex relationship, it seems sensible to use gluten-free doughs for 3D printing.
In order to maintain the dough-like properties and nutritional value of printing material, other proteins can be used to replace the gluten in wheat flour. In the study by Fahmy et al. mentioned earlier, egg white protein was used for this purpose. The results showed similar good stability of the printed objects compared to the standard wheat dough (Fahmy et al., 2020a). Another possible recipe for 3D printing is composed of wheat starch, soy protein isolate (SPI) and water. In this case, the ratio between starch and protein is kept at the same ratio as for a wheat flour, as in the study by Fahmy et al. However, due to the high water absorption capacity of SPI, the amount of water must be increased to a 110g H2O/100g starch-protein mixture. That this printing mass can be printed well can be seen in Figure 2 a). A cube with almost vertical sidewalls, which corresponds to a good stability after extrusion, can be realized without any problems. However, during printing with this formulation, it has been shown that water partly leaks from the extruder (see Figure 2 b)). This means that starch and SPI are not able to bind or hold the water completely during extrusion depending on the water amount in the recipe. Due to the increasing pressure, phase separation
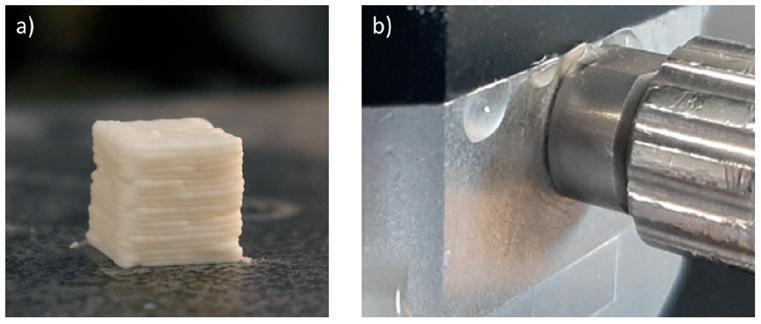
occurs. This could prove to be a problem in the course of printing, especially afterwards, as the properties of the printing material may change as a result of the leaking water. In addition, the nozzle becomes blocked, which means that further printing is no longer possible. One possibility to prevent this is the use of hydrocolloids. The waterbinding or gel-forming properties of these additives prevent water from escaping during the printing process. When selecting hydrocolloids, care must be taken to ensure that they also meet the rheological properties of the printing process described above. This has already been confirmed in the literature with the hydrocolloids xanthan gum, carrageenan, agar and gelatine (GholamipourShirazi et al., 2019).
By adjusting the recipe using additives and varying the water content, a dough-like mass based on starch and protein can be printed. This is the first step towards bread from the 3D printer. In addition to a suitable printing mass, it is also necessary to simulate the typical bread structures of the crust and crumb using a 3D printer. The resulting requirements are described in the next section.
Structure and infill pattern of the printed objects
The internal structure of a bread resembles a sponge with a gas phase (pores) and a solid phase (gelatinized starch and denatured protein). A typical pore structure for a wheat bread with smaller and larger cavities can be seen in Figure 3 a). This pore distribution is specific for different bread types and thus also determines the sensory character of a bread type. With a 3D printer, these internal structures (also infill patterns) can be customized and standardized. As mentioned above, the object to be printed is created digitally, e.g. using Computer-Aided Design (CAD) programs. This technique enables the creation of completely new shapes and infill structures that are impossible with a conventional manufacturing process. Thus, 3D printing offers an opportunity for the entire food industry to develop new innovative products. Furthermore, it offers new possibilities for research to examine the structure of the crumb in more detail. For example, the thickness of the lamellae between two pores can be varied or the behavior of a single pore with a defined shape within a starch-protein matrix can be investigated.
For the creation of an infill pattern, the programs with which one can control a 3D printing process (slicer software) already offer some pre-settings. Different patterns can be selected and the density of the infill structure can be set (see Figure3 b)). Using conventional materials for 3D printing (e.g. plastics), these infill patterns serve on the one hand to give the material the necessary stability and on the other hand to save as much material as possible. There are already first studies that show the influence of different infill patterns on the texture of printed food objects. Liu et al. used a formulation of potato flakes, wheat starch, soybean oil and water to print objects with different infill patterns (similar to Figure 3 b)). As expected, the strength of the objects post-treated by microwave vacuum drying increases with increasing infill density. At the same time, a difference in strength between infill patterns was also found (Z. Liu & Zhang, 2021). Severini et al. also found similar results with a mixture of wheat flour and water (Severini et al., 2016). Derossi et al. took aim at creating targeted textures using 3D printing by varying the number of voids. Again, by varying the ratio between void and dough, different texture impressions can be created (A. Derossi, Caporizzi, Paolillo, et al., 2020).
With the infill patterns, which have been quite simple so far, it is already possible to influence the texture of the printed objects and to evoke different sensory impressions. However, the patterns still have little in common with the pore structure of a loaf of bread. As can be seen in Figure 3 a), the pores in a bread crumb are round to oval. In addition, there are individual cavities that are completely enclosed by dough (closed pores) and, more frequently, others that are connected to other cavities (open pores). To create a bread crumb with a 3D printer, these closed and open pore structures must be created inside the printed object. That this is possible
Figure 3: a) Crumb structure of a whole wheat bread, b) Infill settings by the slicer software on a 3D printer
Figure 4: Print of closed pores inside a dough matrix of wheat starch, soy protein isolate and water
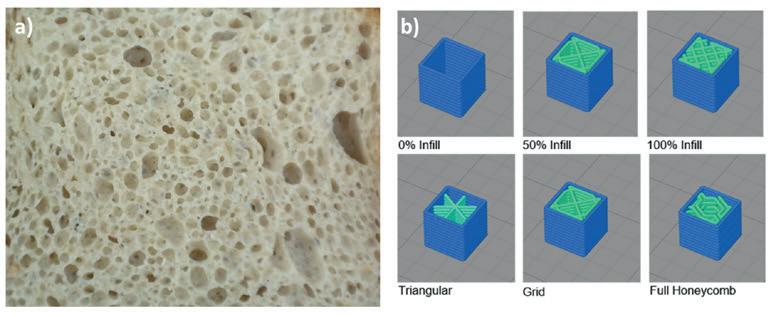
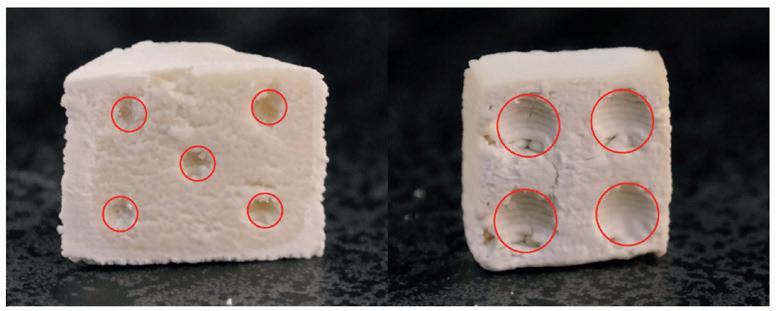
with a 3D printer can be seen in Figure 4. In these experiments, a dough matrix of wheat starch, SPI and water was printed and closed pores of different sizes and in different positions were placed inside the printed object. As Fahmy et al. could show, the texture of the printed objects can thus also be specifically influenced and desired strengths of the printed objects can be produced. The position of the closed pores inside does not play a major role. The decisive factor is the ratio between void and dough, the so-called porosity of the printed objects. The greater the porosity, i.e. the more or larger the voids inside, the softer the final product will be (Fahmy et al., 2020b). This shows that crumblike structures are possible with a 3D printer. A next study investigates more complex pore arrangements with open and closed pores to study the influence of the pore distribution and their arrangement on the texture of the printed objects. There are still limiting factors that are mainly defined by the properties of the 3D printer. Thus, the lamella thickness between two pores can be minimally reduced to the size of the nozzle diameter. For the printed objects shown in Figure 4, a diameter of 0.84mm was used. However, the average pore wall diameter of wheat bread is about 0.2mm (Lassoued et al., 2007). Therefore, there is still a need for further optimization in order to reproduce the crumb structure of traditional breads with the 3D printer.
The current results show that the texture of the printed objects can be influenced by varying the infill pattern. Even typical bread structures such as closed pores can already be printed. However, in order to realistically reproduce a crumb structure on the 3D printer, the printing process must be further optimized. Ultimately, however, it has been shown that it is primarily the porosity of the printed objects that determines the strength of the end products. Texture, however, can be influenced not only by the design of the infill pattern, but also by the mechanical properties of the printing materials. By regulating the water content, which can be influenced by the postprocessing step, the strength of the printed objects can also be influenced (Fahmy et al., 2020b).
Post-processing
The texture of a bread is largely determined by the difference between the crumb and the crust. The dry and firm crust is clearly distinguished from the moist and elastic crumb. This characteristic profile must be reproduced with the 3D printing process. Post-processing is the decisive step in order to influence the texture of the printed object downstream and at the same time offers the possibility of breaking new ground in terms of texture design. This process step should primarily be used to stabilize the printed objects by inducing phase transitions of the biopolymers starch and protein and reducing the moisture of the printed objects. Furthermore, the sensory character of the printed objects can be changed by Malliard reactions. An important criterion is that the shape of the printed objects does not change as a result of the post-treatment.
In traditional bread making, the dough is baked at the end. Hereby the fermentation process is stopped, the starch is gelatinized, protein is denatured and different flavors are created through the Maillard reaction and caramelization. This makes the bread taste like what we are used to and makes it more digestible. Thus baking is an essential step and hence has been used by many scientists in the 3D printing of grain-based products. The most commonly used method, similar to traditional bread making, is baking in an oven. Temperatures of 150°C-190°C are used and baked for 10-18min (A. Derossi, Caporizzi, Oral, et al., 2020; A. Derossi, Caporizzi, Paolillo, et al., 2020; Kim et al., 2019; Pulatsu et al., 2020; Fan Yang et al., 2019). The studies show that printed objects made of dough containing fat and sugar (cookie dough) tend to collapse due to the heating step. The high temperatures melt the fats and sugars contained in the dough, thus changing the rheological properties. The dough becomes softer and collapses under its own weight (Kim et al., 2019; Pulatsu et al., 2020). However, by using thickening agents such as xanthan gum, this can be counteracted (Kim et al., 2019). Doughs containing only oil (similar to a pizza dough) were studied by Derossi et al. Again, a reduction in the volume of the printed objects after baking was observed. However, this could be attributed primarily to the loss of moisture during heating. It was found that the larger the surface area of the printed objects, due to varying infill patterns, the greater the loss (A. Derossi, Caporizzi, Paolillo, et al., 2020). An alternative method to baking was applied to a potato dough made from potato flakes, wheat flour, soybean oil, and water in the research by Liu et al. The printed objects were dried after the printing process using a microwave vacuum dryer at 70°C and -0.085 MPa. Here, the printed objects also became smaller due to heating, albeit much smaller (Z. Liu & Zhang, 2021).
Another major disadvantage of the described methods is that the printed object must be removed from the 3D printer. This decouples the post-processing step from the actual printing process and thus leads to an additional work step during which the printed object can also be damaged. As mentioned at the beginning, the post-processing step should be integrated into the printing process and should not only be applied after the printing has been completed. For example, the printed object can be treated after each printed layer and the texture can be affected differently throughout the printing process. One way to implement this is to use an IR heater that is mounted on the 3D printer's housing. The printed object then simply needs
to be moved under the emitter by controlling the printing plate. This can then be incorporated into the printing process as many times as required, allowing the printed object to be treated after each layer or even after multiple layers. In the investigations of Fahmy et al., an IR radiator was used which was moved at different speeds over the print object after each layer. By controlling the intensity of the heating, the moisture content of the printed objects could be varied and thus the texture could be influenced (Fahmy et al., 2020b).
The texture of the cereal-based printed objects can be influenced by post-treatment. Current research only focuses on the strength and hardness of the printed objects. The elasticity of a bread crumb has not yet been reproduced on a 3D printer. An important factor here is starch gelatinization and protein denaturation, which has also not been the focus of any of the investigations to date.
Summary and outlook
3D printing is about to revolutionize food production. Not only is the interest of the food industry in this new innovative technology increasing, but also research is intensively engaged in the development of new recipes and attempts to understand the printing process in detail. It has become clear that the knowledge gained from recipe development, structural design of the infill pattern and post-processing must be combined in order to achieve specific product properties.
By combining starch, protein and hydrocolloids, an edible ink can already be produced that is suitable for printing bread-like products. Similarly, it has been shown that by varying the infill pattern, the texture of the printed objects can be influenced. This can furthermore be extended with a post-processing step, whereby heat treatment can also be used to adjust the texture to the desired properties.
This development will be continued by constant new approaches and thus a bread from a 3D printer will become more and more realistic. An important step will be the extension of the printing mass by different flavors. A study by Fahmy and Amann et al. has already experimented with an inhomogeneous distribution of salt for taste in order to specifically influence sensory perception (Fahmy et al., 2021). Through these and similar studies, it will be possible in the future to integrate flavors into products to evoke specific taste sensations. There is no limit to the use of bread-like or product-untypical flavors.
Furthermore, the further development of 3D printers will make it possible in the future to create realistic pore distributions like those in a loaf of bread. With the already tested post-processing treatment, the firmness and elasticity of the crumb and crust of conventional breads will be made possible through the gelatinization of starch and the denaturation of proteins, thus laying the foundation for bread from the 3D printer. Research or new innovative approaches from the industry will show which bread imitations or new fancy products can still be realized with this technology. Through the further development of the initial findings and a combination of recipe development, innovative structure design and finalizing post-processing, it is only a matter of time before we can enjoy the first bread from the 3D printer. +++
Authors
Martin Heckl1*, Mario Jekle2*, Thomas Becker1
1Technical University of Munich, Chair of Brewing and Beverage Technology, Cereal Technology and Process Engineering Unit, Weihenstephaner Steig 20, 85354 Freising, Germany 2Department of Plant-based Foods, Institute of Food Science and Biotechnology, University of Hohenheim, Garbenstr. 25, 70599 Stuttgart, Germany.
*Corresponding authors: PD Dr. Mario Jekle, mario.jekle@uni-hohenheim.de Martin Heckl, martin.heckl@tum.de
Sources
Alpers, T., Tauscher, V., Steglich, T., Becker, T., & Jekle, M. (2021).
The self-enforcing starch-gluten system-strain-dependent effects of yeast metabolites on the polymeric matrix. Polymers, 23 (1), 1-15. https://doi.org/10.3390/polym13010030 Crump, S. S. (1992). Apparatus and method for creating threedimensional objects. In Google Patents. https://doi. org/10.2116/bunsekikagaku.28.3_195 Derossi, A., Caporizzi, R., Oral, M. O., & Severini, C. (2020).
Analyzing the effects of 3D printing process per se on the microstructure and mechanical properties of cereal food products. Innovative Food Science & Emerging Technologies, 102531. https://doi.org/10.1016/j.ifset.2020.102531 Derossi, A., Caporizzi, R., Paolillo, M., & Severini, C. (2020).
Programmable texture properties of cereal-based snack mediated by 3D printing technology. Journal of Food
Engineering, 289, 110160. https://doi.org/10.1016/j.jfoodeng.2020.110160 Derossi, R., Caporizzi, R., Paolillo, M., Oral, M. O., & Severini, C. (2021). Drawing the scientific landscape of 3D Food Printing; Maps and interpretation of the global information in the first 13 years of detailed experiments, from 2007 to 2020. Innovative Food Science & Emerging Technologies. Fahmy, A. R., Amann, L. S., Dunkel, A., Frank, O., Dawid, C., Hofmann, T., Becker, T., & Jekle, M. (2021). Sensory design in food 3D printing - Structuring , texture modulation , taste localization , and thermal stabilization. Innovative Food
Science and Emerging Technologies, 72, 102743. https:// doi.org/10.1016/j.ifset.2021.102743 Fahmy, A. R., Becker, T., & Jekle, M. (2020a). 3D printing and additive manufacturing of cereal-based materials: quality analysis of starch-based systems using a camera-based morphological approach. Innovative Food
Science & Emerging Technologies, 63, 102384. https://doi. org/10.1016/j.ifset.2020.102384 Fahmy, A. R., Becker, T., & Jekle, M. (2020b). Design and modulation of food textures using 3D printing of closed-cell foams in point lattice systems Hardness targeted design of textures in the elastic regime. 63, 85354. Gholamipour-Shirazi, A., Norton, I. T., & Mills, T. (2019). Designing hydrocolloid-based food-ink formulations for extrusion 3D printing. Food Hydrocolloids, 95 (December 2018), 161-167. https://doi.org/10.1016/j.foodhyd.2019.04.011 Godoi, F. C., Prakash, S., & Bhandari, B. R. (2016). 3D printing technologies applied for food design: status and prospects. Journal of Food Engineering, 179, 44-54. https://doi. org/https://doi.org/10.1016/j.jfoodeng.2016.01.025 He, C., Zhang, M., & Fang, Z. (2019). 3D printing of food: pretreatment and post-treatment of materials. Critical
Reviews in Food Science and Nutrition, 0(0), 1-14. https:// doi.org/10.1080/10408398.2019.1641065 Kim, H. W., Lee, I. J., Park, S. M., Lee, J. H., Nguyen, M. H., &
Park, H. J. (2019). Effect of hydrocolloid addition on dimensional stability in post-processing of 3D printable
cookie dough. Lwt, 101, 69-75. https://doi.org/10.1016/j. lwt.2018.11.019 Lassoued, N., Babin, P., Della Valle, G., Devaux, M. F., & Réguerre, A. L. (2007). Granulometry of bread crumb grain:
Contributions of 2D and 3D image analysis at different scale. Food Research International, 40(8), 1087-1097. https://doi.org/10.1016/j.foodres.2007.06.004 Li, H., Tan, Y. J., Leong, K. F., & Li, L. (2017). 3D bioprinting of highly thixotropic alginate/methylcellulose hydrogel with strong interface bonding. ACS Applied Materials and
Interfaces, 9(23), 20086-20097. https://doi.org/10.1021/ acsami.7b04216 Liu, Q., Zhang, N., Wei, W., Hu, X., Tan, Y., Yu, Y., Deng, Y., Bi,
C., Zhang, L., & Zhang, H. (2020). Assessing the dynamic extrusion-based 3D printing process for power-law fluid using numerical simulation. Journal of Food Engineering, 275. https://doi.org/10.1016/j.jfoodeng.2019.109861 Liu, Z., & Zhang, M. (2021). Texture properties of microwave post-processed 3D printed potato snack with different ingredients and infill structure. Future Foods, 3, 100017. https://doi.org/10.1016/j.fufo.2021.100017 Paxton, N., Smolan, W., Böck, T., Melchels, F., Groll, J., & Jungst,
T. (2017). Proposal to assess printability of bioinks for extrusion-based bioprinting and evaluation of rheological properties governing bioprintability. Biofabrication, 9 (4), 044107. https://doi.org/10.1088/1758-5090/aa8dd8 Pico, J., Bernal, J., & Gómez, M. (2015). Wheat bread aroma compounds in crumb and crust: A review. Food Research
International, 75, 200-215. https://doi.org/10.1016/j. foodres.2015.05.051 Pulatsu, E., Su, J. W., Lin, J., & Lin, M. (2020). Factors affecting 3D printing and post-processing capacity of cookie dough.
Innovative Food Science and Emerging Technologies, 61, 102316. https://doi.org/10.1016/j.ifset.2020.102316 Severini, C., Derossi, A., & Azzollini, D. (2016). Variables affecting the printability of foods: Preliminary tests on cerealbased products. Innovative Food Science & Emerging
Technologies 2, 38 (A), 281-291. Yang, Fan, Zhang, M., Fang, Z., & Liu, Y. (2019). Original article
Impact of processing parameters and post-treatment on the shape accuracy of 3D-printed baking dough. International Journal of Food Science and Technology, 54, 68-74. https://doi.org/10.1111/ijfs.13904 Yang, Fan, Zhang, M., Prakash, S., & Liu, Y. (2018). Physical properties of 3D printed baking dough as affected by different compositions. Innovative Food Science and
Emerging Technologies, November 2017. https://doi. org/10.1016/j.ifset.2018.01.001 Yang, Fanli, Guo, C., Zhang, M., Bhandari, B., & Liu, Y. (2019).
Improving 3D printing process of lemon juice gel based on fluid flow numerical simulation. Lwt, 102, 89-99. https:// doi.org/10.1016/j.lwt.2018.12.031