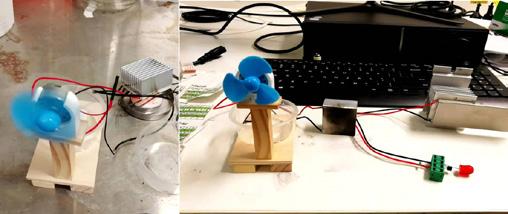
3 minute read
Game-Changer in Thermoelectric Materials: Decoupling Electronic and Thermal Transport
Source: Sally Wood
A new study at the University of Wollongong (UOW) has overcome a major challenge of thermoelectric materials, which can convert heat into electricity and vice versa, and improve conversion efficiency by more than 60 per cent.
Current and potential future applications range from low-maintenance, solid-state refrigeration to compact, zero-carbon power generation, which could include small, personal devices powered by the body’s own heat. “The decoupling of electronic (electronbased) and thermal (phonon-based) transport will be a game-changer in this industry,” said Professor Xiaolin Wang at the University of Wollongong, who lead the project. Bismuth telluride-based materials (Bi2Te3, Sb2Te3 and their alloys) are the most successful commercially-available thermoelectric materials. Current and future applications fall into two categories: converting electricity into heat, and vice versa. Converting electricity into heat is reliable, and involves low-maintenance solidstate refrigeration with no moving parts, no noise, and no vibration. Meanwhile, converting heat into electricity involves fossil-free power generation from a wide range of heat sources or powering microdevices ‘for free’, using ambient or body temperature. Heat harvesting takes advantage of the free, plentiful heat sources provided by body heat, automobiles, everyday living, and industrial process. But without the need for batteries or a power supply, thermoelectric materials could be used to power intelligent sensors in remote, inaccessible locations. An ongoing challenge of thermoelectric materials is the balance of electrical and thermal properties. In most cases, an improvement in a material’s electrical properties, like higher electrical conductivity, means a worsening of thermal properties. The three-year project at UOW’s Institute of Superconductivity and Electronic Materials discovered a way to decouple and simultaneously improve both thermal and electronic properties. The team added a small amount of amorphous nanoboron particles to bismuth telluridebased thermoelectric materials, using nanodefect engineering and structural design. Amorphous nano boron particles were introduced using the spark plasma sintering method. “This reduces the thermal conductivity of the material, and at the same time increases its electron transmission. “The secret of thermoelectric materials engineering is manipulating the phonon and electron transport,” explained Professor Wang. While electrons carry heat and conduct electricity, material engineering based on electron transport alone is prone to the perennial trade-off between thermal and electrical properties. In comparison, phonons only carry heat. Hence, blocking phonon transport reduces thermal conductivity induced by lattice vibrations, without affecting electronic properties. “The key to improving thermoelectric efficiency is to minimise the heat flow via phonon blocking, and maximise electron flow via (electron transmitting),” said lead author and PhD student Guangsai Yang. “This is the origin of the record-high thermoelectric efficiency in our materials.” The results include a record-high conversion efficiency of 11.3 per cent, which is 60 per cent better than commercially-available materials prepared by the zone melting method. It is also the most successful commerciallyavailable thermoelectric materials, as bismuth telluride-based materials are also typical topological insulators. In addition to support from the Australian Research Council Future Fellowship, Centre of Excellence and Linkage Infrastructure Equipment and Facilities programs, funding was also received from the China Scholarship Council and National Natural Science Foundation of China. Experimentation facilities included the UOW Electron Microscopy Centre, with technical support from the Australian Centre for Microscopy and Microanalysis at the University of Sydney. Professor Wang leads FLEET’s UOW node. He also leads the Enabling Technology team who are developing novel and atomically thin materials underpinning FLEET’s search for ultra-low energy electronics, managing synthesis and characterisation of novel 2D at UOW. FLEET is a collaboration of over a hundred researchers, seeking to develop ultra-low energy electronics to face the challenge of energy use in computation.
Thermoelectric material demonstration: powering a small fan, LED. Image credit: FLEET.
Left: Lead author, UOW PhD student Guangsai Yang. Image credit: FLEET. Right: Chief Investigator Professor Xiaolin Wang (University of Wollongong). Image credit: FLEET.
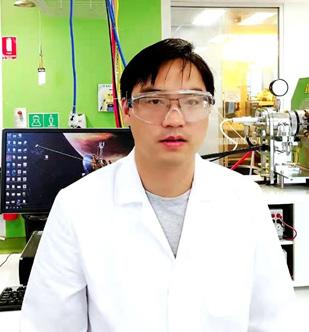
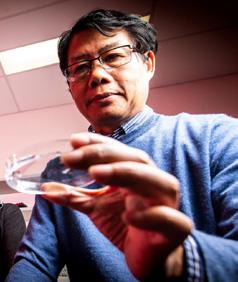