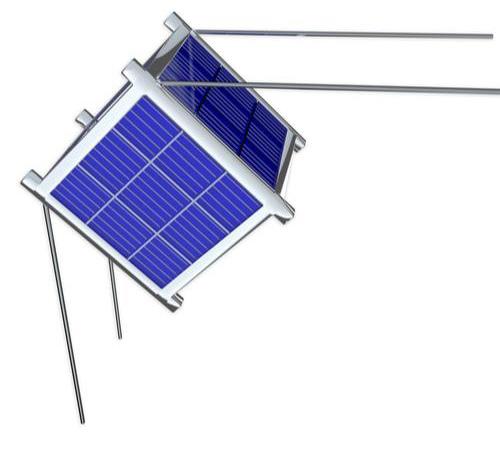
17 minute read
Breaking News
UNISA’s New Space Age Technologies to Boost the Power of Small Satellites The University of South Australia (UniSA) secured two out of six grants in the latest round of South Australian Defence Innovation Partnership Cooperative Research Grants.
Dr Kamil Zuber, a Research Fellow at UniSA’s Future Industries Institute (FII), said the development of freeform optics for small satellites will expand intelligence, surveillance and reconnaissance capacity and capability for space satellites. “The emerging technology of freeform optics, where mirrors can be designed and manufactured to take on complex shapes, allows us to produce large fields of view in smaller packages – which is a powerful adaptation for the new generation of small satellites that are in rapid development,” Dr Zuber said. Researchers will also collaborate with DST Group, Amaero, SMR and the University of Adelaide to prototype and validate durable coatings for freeform optical components used for small space satellites. “Achieving a stable, durable coating in the harsh low earth orbit environment that is impervious to radiation and atomic oxygen is one of the challenges that this project aims to address,” Dr Zuber said. In addition, another project is being led by FII Research Fellow, Associate Professor Craig Priest to address concerns in the space capabilities to develop satellite systems that can withstand and respond to adverse events. “With several hundred small satellites launched every year, space is becoming crowded and hostile,” Associate Professor Priest said. “Satellites need to be smaller, more agile, and more energy efficient, with on-board thrust mechanisms that also have minimal hardware. “Our research will be focussed on nanofluidic thrusters which can offer a solution to those challenges,” he concluded.
The development of freeform optics for small satellites will expand intelligence, surveillance and reconnaissance capacity and capability for space satellites. Image credit: UniSA. The burning of rice husks is a major contributor of pollution and landfill in India.
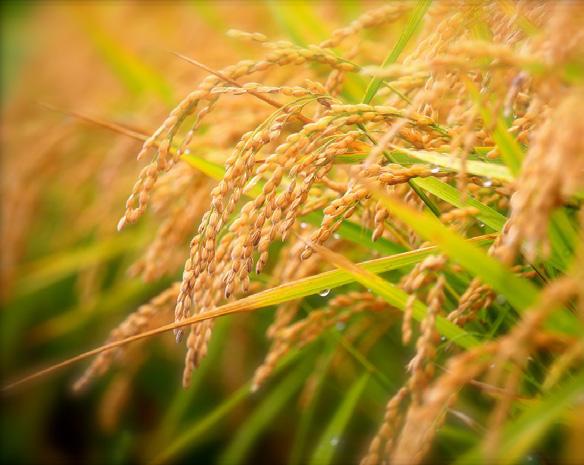
Thermal Energy Storage the Key to Reducing Agricultural Food Pollution
Thermal energy researcher at the University of South Australia (UniSA), Professor Frank Bruno has been awarded nearly $1 million by the Federal Government to find a solution to agricultural pollution in Australia and India. Professor Bruno, will lead a project with India’s biggest private university to develop a renewable energy-driven food processing and drying system to reduce pollution and landfill issues across both countries. The three-year $977,585 project is being funded by the Australia-India Strategic Research Fund – Australia’s largest fund dedicated to bilateral science collaboration. India is the largest global producer of food, while Australia is one of the world’s largest food exporters. Together, there is scope for greater collaboration between the two countries to help curve agricultural pollution. India also has nine of the top ten cities with the highest air pollution in the world, partially due to agricultural waste burnt by farmers in the field. Professor Bruno’s research will use technology that cuts air pollution and agricultural waste landfill, and also reduces food manufacturers’ costs. “Shifting towards this solution will undoubtedly result in significant amounts of biomass which can then be converted into high-value renewable biofuels,” Professor Bruno said. Professor Bruno’s research will focus on developing high temperature, electrically charged thermal energy storage (ECTES), which can provide heated air for drying, replacing fossil fuels. This research project will also build on existing research on high temperature ECTES being undertaken by Professor Bruno and his team at UniSA’s Mawson Lakes Campus, in addition to another project to improve the shelf life of milk.
BREAKING NEWS
Scientists Defy Nature to Make Insta-Bling at Room Temperature
An international research team has made diamonds in minutes at room temperature – a process that normally takes billions of years, huge amounts of pressure and super-hot temperatures. The team was led by RMIT University and the Australian National University (ANU), who worked with the University of Sydney and Oak Ridge National Laboratory in the United States. Together, they made two types of diamonds: the kind found on an engagement ring and another type of diamond called Lonsdaleite, which is found in nature at the site of meteorite impacts. Professor Dougal McCulloch from RMIT used advanced electron microscopy techniques to capture solid and intact slices from the experimental samples to create snapshots of how the two types of diamond formed. “Our pictures showed that the regular diamonds only form in the middle of these Lonsdaleite veins under this new method developed by our cross-institutional team.
“Seeing these little ‘rivers’ of Lonsdaleite and regular diamond for the first time was just amazing and really helps us understand how they might form,” Professor McCulloch explained. Lonsdaleite has a different crystal structure to regular diamonds, it is predicted to be 58 per cent harder. Professor Jodie Bradby from ANU described the research as a breakthrough. “Natural diamonds are usually formed over billions of years, about 150km deep in the Earth where there are high pressures and temperatures above 1,000 degrees Celsius,” she said. This new and unexpected discovery shows both Lonsdaleite and regular diamonds can form at normal room temperatures by applying high pressures – similar to 640 African elephants on the tip of a ballet shoe.
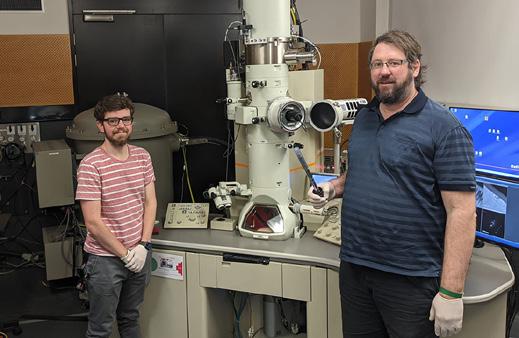
Abobe: PhD candidate Brenton Cook and Professor Dougal McCulloch in the RMIT Microscopy and Microanalysis Facility. Image credit: RMIT University. Left: RMIT researchers captured ‘rivers’ of Lonsdaleite and regular diamond. Image credit: RMIT University. Australia has an opportunity to create thousands of high-tech jobs in quantum technologies.
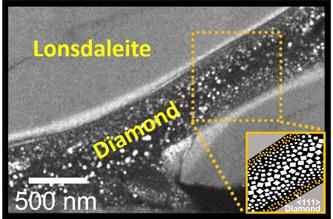
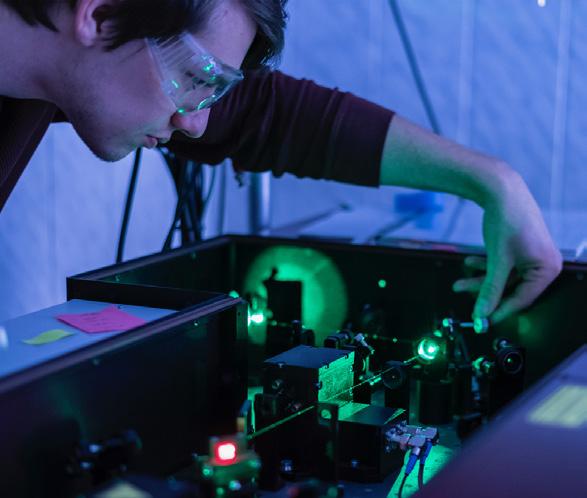
Sydney Poised to Be a Global Hub for Quantum Technology
The Sydney Quantum Academy was launched in December by UNSW and other partner universities. The Minister for Jobs, Investment, Tourism and Western Sydney, the Hon Stuart Ayres joined representatives from academia and industry to discuss plans to grow the city’s quantum economy, create new jobs and attract investment. The new venture is a partnership between four universities for quantum research: UNSW Sydney, Macquarie University, the University of Sydney and the University of Technology Sydney. It has been tasked with supercharging the sector’s growth. “The Academy will keep us at the forefront of quantum technology by developing the future employers, entrepreneurs and the workforce required to sustain the industry’s growth. “This includes investing in support networks for emerging technologies where we have credible expertise,” Minister Ayres said. Professor Peter Turner is the CEO of the Academy, who said the talent pipeline will be developed through education and training programs, industry partnerships and internships. “The potential for quantum is enormous, which is why we are seeing significant increases in effort and investment around the world. “Quantum technologies will fundamentally change areas like computation and sensing. They will help us to solve problems that we simply can’t solve with classical information technology,” he said. Quantum is an industry that is expected to catalyse a broader capability, which will be transformational for all industries, similar to the effect of the digital revolution. Professor Turner said the Academy is very fortunate to have global technology industry and government leaders involved. “It demonstrates the significance of what’s happening in the quantum space in Sydney,” he said.
BREAKING NEWS
Tiny Bubbles on Electrodes Key to Speeding up Chemical Processes
New Curtin University-led research has shown the formation of bubbles on electrodes – typically thought to be a hindrance – can be beneficial. The research found that deliberately added bubbles, or oil droplets, are able to accelerate processes like the removal of pollutants, like hydrocarbons, from contaminated water and the production of chlorine. Dr Simone Ciampi, from Curtin’s School of Molecular Life Sciences, explained that many industrial processes are electrochemical – meaning the desired chemical reaction to create an end product is assisted by the flow of electrical currents. “Electrodes assist chemists to achieve required electrochemical reactions, such as in the purification of alumina, and the technology used to produce chlorine for swimming pools,” Dr Ciampi said. Using fluorescence microscopy, electrochemistry and multi-scale modelling, the research team showed that in the vicinity of bubbles that stick to an electrode surface, valuable chemical reactions occur under conditions where normally such reactions would be considered impossible. Dr Yan Vogel is a co-researcher who is also from Curtin’s School of Molecular and Life Sciences. He said it was these reactions occurring in the corona of bubbles that led to the team’s interest, and warranted further exploration. “We revealed for the first time that the surrounding surface of an electrode bubble accumulates hydroxide anions, to surprisingly large concentrations. “This population of negatively charged ions surrounding bubbles is unbalanced by ions of the opposite sign, which was quite unexpected. Usually charged chemical species in solution are generally balanced, so this finding showed us more about the chemical reactivity of bubbles,” Dr Vogel said.
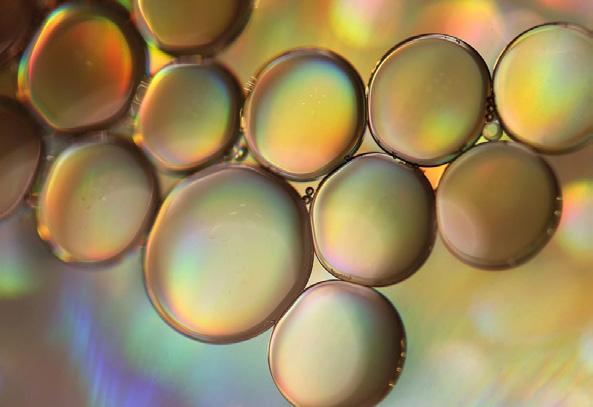
The formation of bubbles on electrodes can be beneficial, accelerating processes such as the removal of pollutants (like hydrocarbons) from contaminated water. Image credit: Curtin University. Lead researcher, Curtin University PhD candidate Liam Scarlett. Image credit: Curtin University.
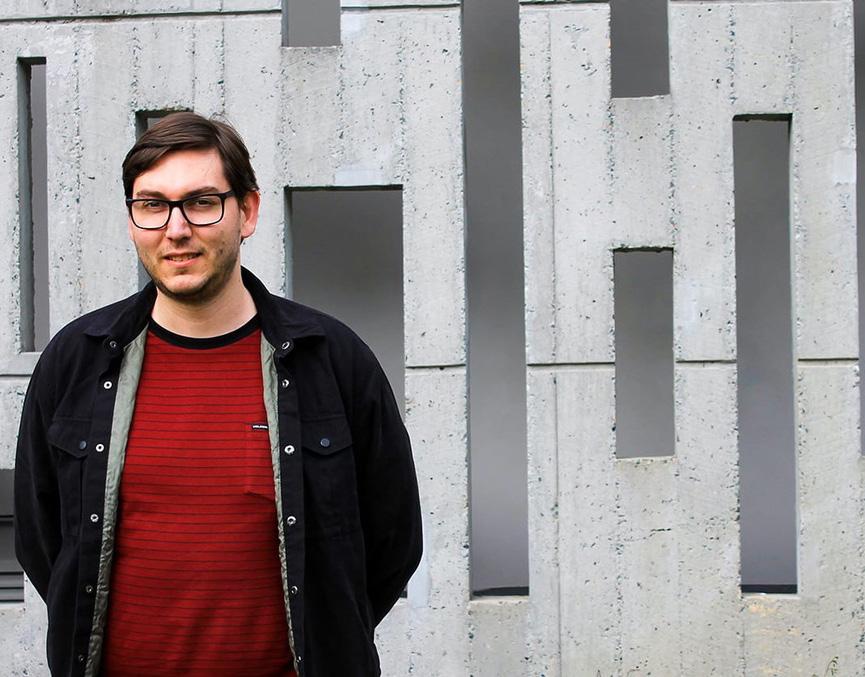
Curtin Collision Models Impact the Future of Energy
A new database of electron-molecule reactions, from Curtin University will make nuclear fusion power a reality. The database allows researchers to accurately model plasmas containing molecular hydrogen, and is supplying data to the International Thermonuclear Experimental Reactor (ITER) – one of the largest scientific projects in the world. Lead researcher and PhD candidate, Liam Scarlett from the Theoretical Physics Group at Curtin’s School of Electrical Engineering, Computing and Mathematical Sciences said his calculations will play a crucial role in the development of fusion technology. “Fusion is the nuclear reaction which occurs when atoms collide and fuse together, releasing huge amounts of energy. “This process is what powers the Sun, and recreating it on Earth requires detailed knowledge of the different types of collisions which take place in the fusion plasma – that’s where my research comes in,” Mr Scarlett said. The study was published in the Atomic Data and Nuclear Data Tables journal, in a collaboration with researchers at Los Alamos National Laboratory and the National Institute of Standards and Technology in the United States. “Until now the available data was incomplete, however our molecular collision modelling has produced an accurate and comprehensive database of more than 60,000 electronmolecule reaction probabilities which, for the first time, has allowed a team in Germany to create an accurate model for molecular hydrogen in the ITER plasma,” Mr Scarlett said. The research project was funded by the United States Air Force Office of Scientific Research as part of an international research endeavour to harness fusion power as a future energy source.
BREAKING NEWS
Meet 3DREDI: The Latest in 3D Bioprinting Innovation
The team from TRICEP, at the University of Wollongong have launched a new 3D bioprinting system on the global stage. The 3D bioprinting system, also known as 3DREDI, is designed to equip users with the essential hardware and skills to embark on projects in the rapidly emerging bioprinting industry. The system was designed and manufactured in Wollongong, and the online launch attracted interest from India, Indonesia, Finland, Dubai, and the United States. TRICEP Director, Distinguished Professor Gordon Wallace said the realisation of the 3DREDI system is an exciting advancement in establishing a new, innovative and sustainable 3D bioprinting industry. “Our team is at the forefront of building new approaches to 3D printing, and the success of this project draws on the significant developments we have achieved in this space in recent years and our focus on building our local capabilities in this area,” Professor Wallace said. 3DREDI features an intuitive bioprinting platform, and performs as a research and education tool. The system is also complete with interactive printing and characterisation tutorials to allow educators and students to familiarise themselves with the capabilities of multi-material bioprinting. Users also receive example cases to get started on their own research. “The 3DREDI system is an exciting advance in 3D bioprinting, and the intuitive and flexible platform has been developed with the input of world-leading clinicians. “3DREDI aims to educate the next generation of biofabricators by equipping them with the skills and tools to tackle big medical challenges, as well as serving as a biomaterials research tool,” Professor Wallace explained.
Below: The team from TRICEP has launched its new 3D bioprinting system on the global stage. Image credit: University of Wollongong. Co-author Dr Dan Sando preparing materials for study at UNSW. Image credit: FLEET.
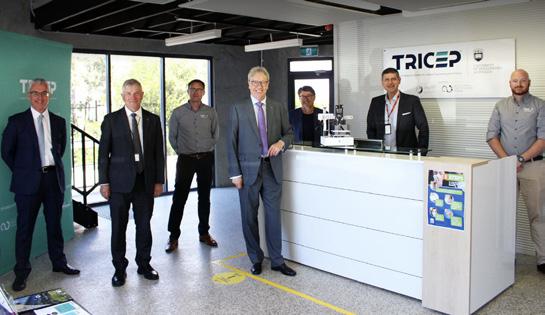
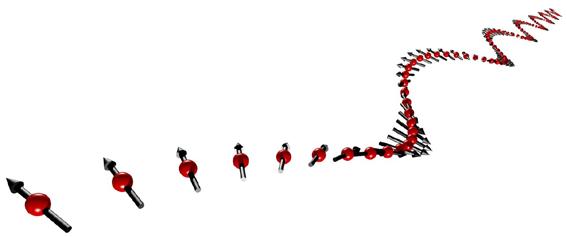
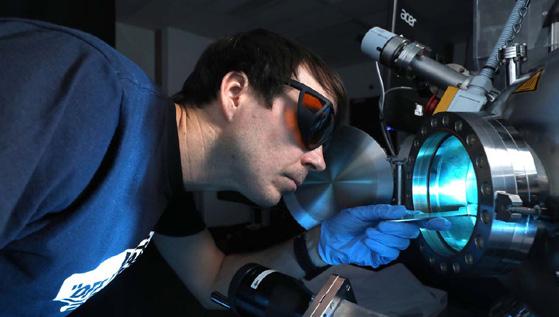
Reviewing Multiferroics for Future, Low-Energy Data Storage
A new study from UNSW has comprehensively reviewed the magnetic structure of the multiferroic material bismuth ferrite (BiFeO3 – BFO). The study reviewed the magnetic structure of bismuth ferrite, focusing on when it is grown as a thin single crystal layer on a substrate. The review advances FLEET’s search for low-energy electronics, bringing together current knowledge on the magnetic order in BFO films, and giving researchers a platform to further develop this material in low-energy magnetoelectric memories. BFO displays both magnetic and electronic ordering at room temperature, which allows for low-energy switching in data storage devices. An example is a magnetic material that displays magnetic order, which is typically made up of lots of neatly ordered, tiny magnets. In a ferroelectric material, some atoms are positively charged, while others are negatively charged, and the method of arranging these atoms gives a specific order to the charge in the material. The coupling between magnetic and ferroelectric order in a multiferroic material opens the way for applications in energyefficient electronics, like non-volatile memory devices. Dr Stuart Burns co-wrote the review, who said new researchers to the field of multiferroics will benefit from their work. “We structured the review as a build-your-own-experiment starter pack: readers will be taken through the chronology of BFO, a selection of techniques to utilise and various interesting ways to modify the physics at play. “With these pieces in place, experimentalists will know what to expect, and can focus on engineering new low-energy devices and memory architectures,” he said.
Spin (magnetic order) in the multi-ferroic material bismuth-ferrite ‘cycles’ through the crystal, offering potential application in emerging electronics fields such as magnonics. Image credit: FLEET.
BREAKING NEWS
Evaluating the Impact of Nanomaterials on Health and the Environment
ANSTO Human Health has embarked on a multidisciplinary approach to understanding the impacts of wide range use of nanomaterials in products, including foods and food packaging. Nanomaterials in the whole lifecycle of materials – in particular, in terms of human health and environmental impact – is an area of concern researchers. The ANSTO project team are continuing to investigate a common food additive, E171 titanium dioxide, which is used as a colouring agent in everyday foods. Dr Paul Callaghan leads the work, which brings toxicology, materials science, radiochemistry and ion beam analysis together to explore the food additives, where some will naturally contain nano-sized particles. The ‘nano’ size means they have a significantly higher surface area for interaction with biological molecules, which may lead to a different fate for the nanomaterials in the body compared to its bulk form. This could present opportunities, like more targeted delivery of drugs, but in some cases, it could be detrimental. Titanium is present within the body in trace amounts, including natural titanium from food ingredients and, potentially, from titanium dioxide macroparticles and nanomaterials, such as in food colouring. “Titanium dioxide is highly insoluble and very poorly absorbed in the gut. “To link exposure and potentially beneficial or detrimental impacts, we need much better sensitivity in assessing where dietary additives go in the body, and how long they stay there,” said Dr Callaghan. In designing their study, the group selected an exotic radioisotope, vanadium-48 (48V), with the appropriate halflife and appropriate emission to radiolabel the titanium for bioimaging and quantification studies.
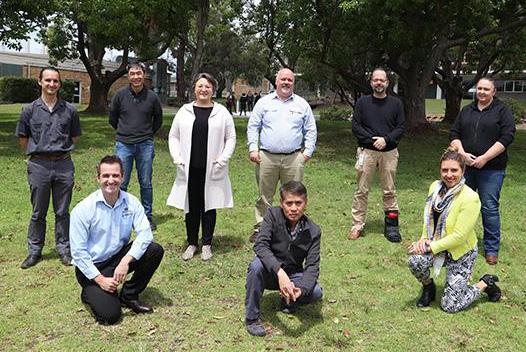
Back row (L to R): Attila Stopic, Herni Wong, Inna Karatchevtseva, Me, Frederic Sierro, Charmaine Day. Front row (L to R): Grant Griffiths, Vu Nguyen, Katie Sizeland. Image credit: ANSTO. A colour map illustrates the inherent colours of 466 types of carbon nanotubes with unique (n,m) designations based their chiral angle and diameter. Image credit: Kauppinen Group and Aalto University.
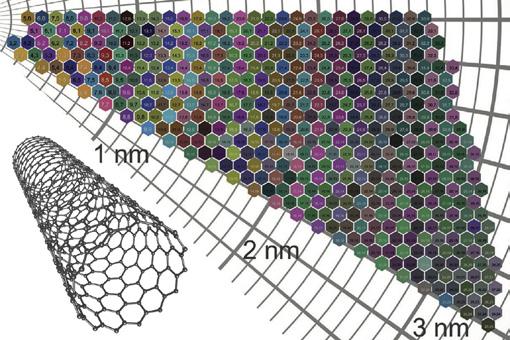
Sheets of Carbon Nanotubes Come In a Rainbow of Colours
Nanomaterials researchers in Finland, the United States and China have created a colour atlas for 466 unique varieties of single-walled carbon nanotubes. The nanotube colour atlas research was conducted by researchers from Aalto University in Finland, Rice University and Peking University in China. The corresponding author of the study, and Aalto physicist Esko Kauppinen explained the benefits of the research. “Carbon, which we see as black, can appear transparent or take on any colour of the rainbow. “The sheet appears black if light is completely absorbed by carbon nanotubes in the sheet. If less than about half of the light is absorbed in the nanotubes, the sheet looks transparent,” he explained. Carbon nanotubes are long, hollow carbon molecules, similar in shape to a garden hose but with sides around one atom thick and diameters about 50,000 times smaller than a human hair. The outer walls of nanotubes are made of rolled graphen, and the wrapping angle of the graphene can vary, much like the angle of a roll of gift wrap paper. If the gift wrap is rolled carefully, at zero angle, the ends of the paper will align with each side of the gift wrap tube. If the paper is wound carelessly, at an angle, the paper will overhang on one end of the tube. “When the atomic structure of the nanotubes causes only certain colours of light, or wavelengths, to be absorbed, the wavelengths that are not absorbed are reflected as visible colours,” Mr Kauppinen said.
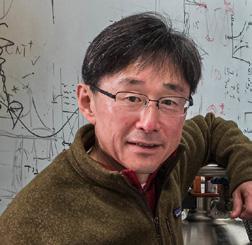
Junichiro Kono. Image credit: Jeff Fitlow and Rice University. Esko Kauppinen. Image credit: Aalto University.
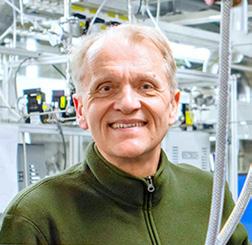
BREAKING NEWS
‘Magic’ Angle Graphene and the Creation of Unexpected Topological Quantum States
A new finding, which was recently published in Nature journal, holds the potential to revolutionise electrical engineering, materials science and especially computer science. Physicists have discovered that under certain conditions, interacting electrons can create topological quantum states. “The last decade has seen quite a lot of excitement about new topological quantum states of electrons,” said Professor Ali Yazdani from Princeton University. “Most of what we have uncovered in the last decade has been focused on how electrons get these topological properties, without thinking about them interacting with one another,” he explained. Professor Yazdani and his team used a material known as magicangle twisted bilayer graphene, which allowed them to explore how interacting electrons can give rise to surprising phases of matter. “This was a wonderful detour that came out of nowhere,” said Kevin Nuckolls, who is the lead author of the research paper. “It was totally unexpected, and something we noticed that was going to be important.” The researchers generated extremely low temperatures and created a slight magnetic field. By directing a scanning tunnelling microscope’s conductive metal tip on the surface of the magic-angle twisted graphene, they were able to detect the energy levels of the electrons. They found that the magic-angle graphene changed how electrons moved on the graphene sheet. “It creates a condition which forces the electrons to be at the same energy,” said Professor Yazdani. They discovered that the interaction between electrons creates topological insulators, which unique devices are serving as insulators in their interiors. This restricts electrons on the inside, and therefore does not conduct electricity.

A Princeton-led team of physicists have discovered that, under certain conditions, interacting electrons can create what are called ‘topological quantum states’. This diagram shows a scanning tunnelling microscope imaging the magic-angle twisted bilayer graphene. Image credit: Kevin Nuckolls.
This diagram depicts the different insulating states of the magic-angle graphene, each characterised by an integer called its ‘Chern number’, which distinguishes between different topological phases. Image credit: Kevin Nuckolls.
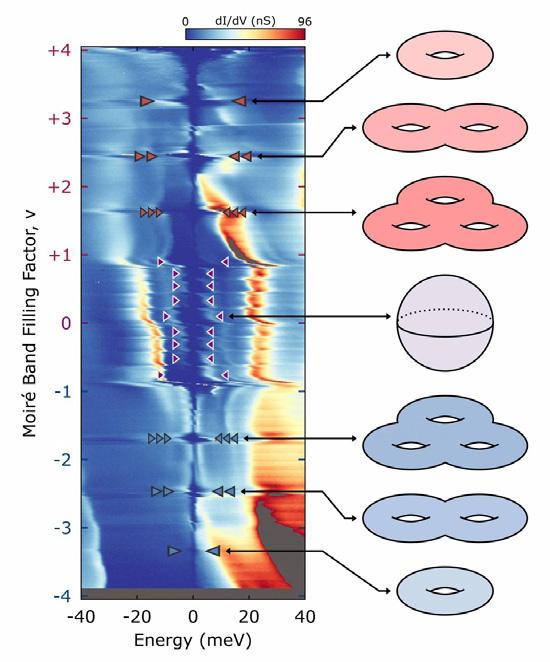