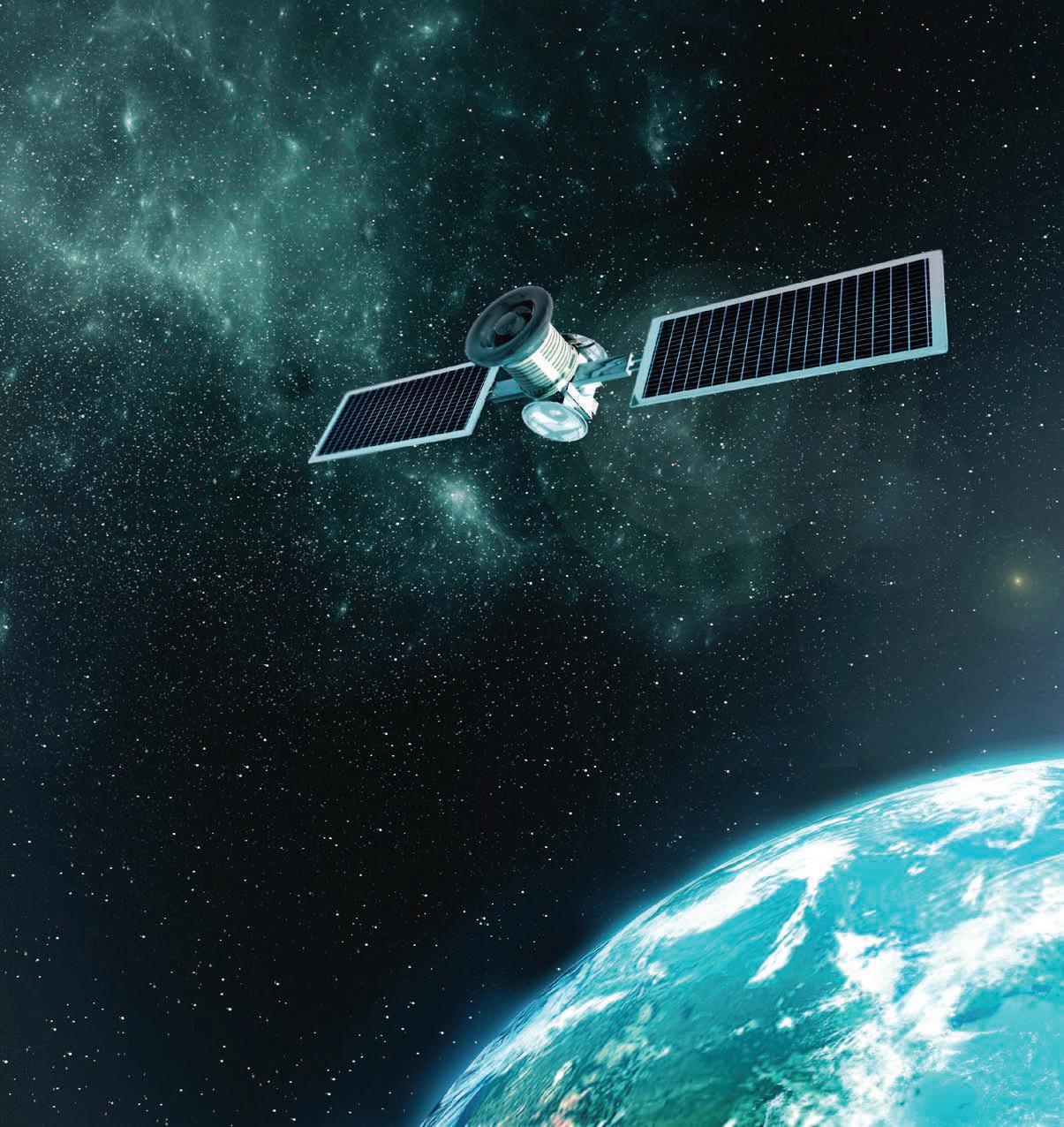
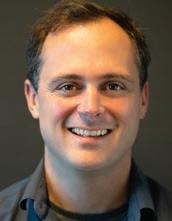
ADI’s AD9213S is the industry-leading, commercial space qualified 10.25 GSPS RF analog-to-digital converter that helps you thrive, not just survive in space. Trust ADI to get your high speed signal processing applications to market faster.
Whether your mission involves—air, space, ground or subsea—WINSYSTEMS’ rugged highly reliable embedded computer systems are designed to support your critical mission needs.
Need help in choosing the right solution? Let WINSYSTEMS assist you in selecting the optimal embedded computing solution that aligns with your application requirements, leaving you more time to focus on system features and delivery.
WINSYSTEMS’ Made-in-USA products deliver the quality, security and performance you need to embed success in every product.
Tiny Pico-ITX SBC: ITX-P-C444
Low power industrial-rated SBC with NXP® i.MX 8M series processor, on-board discrete TPM 2.0 hardware security, dual Ethernet, industrial connectors and I/O
COM Express Solutions: Type 10 Mini and Type 6 Compact Modules
Rugged highly reliable COM modules with Intel ® Atom ® E3900 and i3, i5, i7 processors with on-board discrete TPM 2.0 hardware security
PC/104 -Plus SBC: PPM-C407
Low power industrial-rated SBC with Intel ® Atom ® E3800 series processor, Gigabit Ethernet
Editor’s Perspective
7 Military satellites and space tech
Technology Update
8 AI-enhanced magnetic navigation for military planes gets capabilities trial
By Lisa Daigle10 The sky isn’t the limit for MOSA: New ways of doing business in space
By Dawn ZoldiDefense Tech Wire
14 By
Dan TaylorGuest Blog
43 As wiper threats rise, governments need better coordination, planning, and data-sharing
By Jim Richberg, FortinetChoice Products
44 By Military Embedded Systems Staff
SPECIAL REPORT: Military satellite communications
16 Scalable assurance: A new approach to sourcing rad-hard electronics for space
20 Optical communication: The next defense & aerospace satellite frontier
By Nancy Friedrich, KeysightMIL TECH TRENDS: Enabling artificial intelligence in military systems
24 How ChatGPT can help modernize defense & aerospace
By Ian Ferguson, Lynx Software Technologies
28 Diminutive AI-powered drones outwit threats
By Seth Spiller,Orion Technology Group
INDUSTRY SPOTLIGHT: Rad-hard electronics design trends
32 For New Space satellites, radiation tolerance means new design hurdle
By
SalahBen Doua and Ken Coffman, Vicor
36 Unlock high-performance rad-hard FPGAs
By Juan Garcia,
Renesas Electronics40 Rad-hard by design methodology in today’s military and space markets
By
JensVerbeeck, Magics Technologies
ON THE COVER:
In the arena of rad-hard electronics destined for use in space, there has been in the past few years a brighter spotlight on the rise of commercial off-the-shelf (COTS) components and open standards. While these developments continue to hold sway, the industry is witnessing an expanded array of demands from military customers. The call now: components that can withstand harsh radiation environments plus a broader spectrum of reliable solutions that can be scaled to fit the mission at hand. As the market diversifies, so does the need for a range of reliability, packaging, test levels, and radiation-performance options.
https://www.linkedin.com/groups/1864255/
@military_cots
PAGE ADVERTISER/AD TITLE
13 AirBorn –
Mini-modular hybrid interconnects
2 Analog Devices, Inc. –
Trusted on Earth. Qualified for space.
5 Behlman Electronics, Inc. –
When it comes to VPX, one company has the most flavors
9 GMS – X9 Spider.
The world’s most powerful fullfeatured wearable computer
27 Interconnect Systems –
Smaller, tougher, custom modules
48
Mercury Systems, Inc. –
Protect one of your most critical resources: Time
37 Phoenix International –
Phalanx II: The ultimate NAS
35 PICO Electronics Inc –
DC-DC converters, transformers & inductors
31 SeaLevel Systems, Inc. –
What’s better than our sync serial hardware? Our software.
19 State of the Art, Inc. –
Reliability the only option
3 WinSystems –
Embed mission success
IEEE Nuclear & Space Radiation Effects Conference (NSREC)
July 24-28, 2023
Kansas City, MO
https://www.nsrec.com/
DSEI 2023
September 12-15, 2023
London, England
https://www.dsei.co.uk/
AUSA 2023
October 9-11, 2023
Washington, DC
https://meetings.ausa.org/Annual/index.cfm
Aerospace Tech Week Americas
November 14-14, 2023
Atlanta, GA
https://www.aerospacetechweek.com/ americas/
GROUP EDITORIAL DIRECTOR John McHale john.mchale@opensysmedia.com
ASSISTANT MANAGING EDITOR Lisa Daigle lisa.daigle@opensysmedia.com
TECHNOLOGY EDITOR – WASHINGTON BUREAU Dan Taylor dan.taylor@opensysmedia.com
CREATIVE DIRECTOR Stephanie Sweet stephanie.sweet@opensysmedia.com
WEB DEVELOPER Paul Nelson paul.nelson@opensysmedia.com
EMAIL MARKETING SPECIALIST Drew Kaufman drew.kaufman@opensysmedia.com
WEBCAST MANAGER Marvin Augustyn marvin.augustyn@opensysmedia.com
VITA EDITORIAL DIRECTOR Jerry Gipper jerry.gipper@opensysmedia.com
SALES/MARKETING
DIRECTOR OF SALES Tom Varcie tom.varcie@opensysmedia.com (734) 748-9660
DIRECTOR OF MARKETING Eric Henry eric.henry@opensysmedia.com
OPERATIONS & AUDIENCE DEVELOPMENT (541) 760-5361
STRATEGIC ACCOUNT MANAGER Rebecca Barker rebecca.barker@opensysmedia.com (281) 724-8021
STRATEGIC ACCOUNT MANAGER Bill Barron bill.barron@opensysmedia.com (516) 376-9838
STRATEGIC ACCOUNT MANAGER Kathleen Wackowski kathleen.wackowski@opensysmedia.com (978) 888-7367
SOUTHERN CAL REGIONAL SALES MANAGER Len Pettek len.pettek@opensysmedia.com (805) 231-9582
DIRECTOR OF SALES ENABLEMENT Barbara Quinlan barbara.quinlan@opensysmedia.com AND PRODUCT MARKETING (480) 236-8818
INSIDE SALES Amy Russell amy.russell@opensysmedia.com
STRATEGIC ACCOUNT MANAGER Lesley Harmoning lesley.harmoning@opensysmedia.com
EUROPEAN ACCOUNT MANAGER Jill Thibert jill.thibert@opensysmedia.com
TAIWAN SALES ACCOUNT MANAGER Patty Wu patty.wu@opensysmedia.com
CHINA SALES ACCOUNT MANAGER Judy Wang judywang2000@vip.126.com
WWW.OPENSYSMEDIA.COM
PRESIDENT Patrick Hopper patrick.hopper@opensysmedia.com
EXECUTIVE VICE PRESIDENT John McHale john.mchale@opensysmedia.com
EXECUTIVE VICE PRESIDENT AND ECD BRAND DIRECTOR Rich Nass rich.nass@opensysmedia.com
ECD EDITOR-IN-CHIEF Brandon Lewis brandon.lewis@opensysmedia.com
TECHNOLOGY EDITOR Curt Schwaderer curt.schwaderer@opensysmedia.com
ASSOCIATE EDITOR Tiera Oliver tiera.oliver@opensysmedia.com
ASSOCIATE EDITOR Taryn Engmark taryn.engmark@opensysmedia.com
PRODUCTION EDITOR Chad Cox chad.cox@opensysmedia.com
CREATIVE PROJECTS Chris Rassiccia chris.rassiccia@opensysmedia.com
GRAPHIC DESIGNER Kaitlyn Bellerson kaitlyn.bellerson@opensysmedia.com
FINANCIAL ASSISTANT Emily Verhoeks emily.verhoeks@opensysmedia.com
SUBSCRIPTION MANAGER subscriptions@opensysmedia.com
CORPORATE OFFICE
1505 N. Hayden Rd. #105 • Scottsdale, AZ 85257 • Tel: (480) 967-5581
REPRINTS
WRIGHT’S MEDIA REPRINT COORDINATOR Kathy Richey clientsuccess@wrightsmedia.com (281) 419-5725
The military satellite and overall space market looks robust both today and for the foreseeable future, as funding for classified and unclassified military space platforms continues to increase to combat the ongoing threats in that domain.
“It’s pretty well-known whoever owns space will own the future,” Frontgrade Technologies president and chief executive officer Mike Elias told me in a podcast (listen to it at https://tinyurl.com/5d29f5wf). The U.S. government has been investing a lot into big, exquisite programs for large and small satellites with a lot of capability, he noted.
That investment bodes well for radiation-hardened (rad-hard) component designers like Frontgrade, which was recently spun out as a standalone company by Veritas Capital after the private-equity firm acquired it from CAES.
These platforms will require very fast onboard processing capability and very fast memories, Elias said. “To combat the complex threats the Department of Defense (DoD) requires things to happen very, very quickly. They want the satellites to capture something and be able to process it and send it down to the warfighter, or to whoever needs to act on it.”
That data must be filtered at the satellite level before it’s sent to the ground to speed up decision-making, a move that requires much more computing, much more memory, and more efficient power-source systems, Elias explained.
With the increased funding also comes increased pressure to develop and deploy capability more quickly into the space domain. More rapid development of technology for space means leveraging more commercial components and unique radiation-hardening strategies, as our Technology Editor Dan Taylor details in his feature on page 16.
The DoD acquisition process, never known to be speedy, can move even more glacially where space applications are concerned. However, there are efforts being made within the DoD and in industry to speed up tech development for faster deployments, as Dawn Zoldi, (Colonel USAF, Retired) details in her piece on page 10 covering Lockheed Martin’s rapid development efforts, including its IGNITE internal rapid technologydevelopment program.
IGNITE is developing multiple internally funded on-orbit pathfinders including Lockheed Martin’s LM 400 technology demonstrator – the first satellite off the company’s new LM 400 production line – and the Tactical Satellite, known as the “TacSat.” Zoldi reports that both space-bound craft have
benefited from the company’s investment in three key areas: digital engineering to mature the platforms from a digital perspective, optimized production lines, and the use of flexible MOSA [modular open systems approach] strategies.
Market forecasters are echoing what we’re hearing from industry on military space applications. Analysts at Forecast International, in their report titled “2023-2032: A Glimpse into the Military Satellite Market” expect that over the next 10 years “approximately 850 military satellites to be produced, having a total value of about $53.95 billion,” according to a release from the firm about the report.
For the U.S. market, Forecast International analysts predict that “production of military satellites will be largely unaffected by this development for many years, leading to stable production forecasts. Factors driving production, such as the need to replace in-orbit assets and to counter growing threats to space assets, will remain the same.
“Production in China and Russia will drive the military satellite market during the middle of Forecast International’s current forecasting period of 2023-2032, although Russian efforts might be hampered by the war in Ukraine,” their analysis states. “The need for replacement of Western satellites by the start of the next decade should drive production through the 2020s.”
These upgrades will happen more quickly as they leverage more commercial technology.
Looking at “the satellite industry and the space industry over the past 10 years, the progress of technology has moved at a very rapid pace,” Elias told me. DoD leaders are willing to take a little bit more risk than they typically used to when it comes to leveraging commercial technology, he noted.
For decades, technology developed for space always lagged generations behind that used by the average consumer. I remember writing a story 20 years ago about how the space shuttle was upgrading to Power PC technology from the 1980sera computer system installed in the spacecraft’s cockpit.
“The acceleration of that technology is what’s going to be the future,” Elias predicted. Technology for space may only be “one generation behind instead of five generations behind what the technology is today.”
For more on space-related content, please see our coverage starting on page 10.
A team of researchers at the Air Force Research Labs (AFRL) and the Massachusetts Institute of Technology (MIT) reported a successful demonstration of the capabilities of an aircraft outfitted with artificial intelligence (AI)-enhanced magnetic navigation, known as MagNav.
U.S. Air Force materials describe MagNav as a system that enables aircraft to determine their position using Earth’s geomagnetic field as a reference. The system, installed in an AgilePod pod-based modular sensor package, uses a magnetometer to read Earth’s local magnetic field and determine the aircraft’s location. The system is regarded as a potential replacement for satellite-based GPS, which although widely used, is a feared single point of failure for those military personnel depending on it for navigation.
Earth’s geomagnetic field is an invisible field generated by the planet’s core and crust. The field is well-known and mappable by detecting local variations in direction and intensity, often caused by things like iron deposits and even human-made objects. Unlike satellites, the geomagnetic field can’t be shot down and is ever-present. The Air Force gave early, non-AI-assisted MagNav a test in 2017, when an aircraft equipped with a magnetometer continuously determined its location within 13 meters over an hour-long flight.
The Air Force/MIT team conducted the demo – during the May 2023 Exercise Golden Phoenix out of Travis Air Force Base (central California) – using a C-17A Globemaster III aircraft leveraging AI and machine learning (ML) capabilities from the Air Force-MIT AI Accelerator (AIA). The AIA is a joint project between universities, small businesses, traditional defense, and nontraditional commercial ventures working with the U.S. Air Force and Space Force that seeks to develop and ensure the ethical use of AI
technologies for the U.S. and society as a whole. (Figure 1.)
The joint Air Force and MIT announcement states that during the trial, the MagNav team powered up the AIA’s calibration and positioning neural network, which was trained during flight in just a matter of minutes on a regular laptop computer. The AIA’s neural network architecture removed magnetic noise generated by the aircraft to derive position by comparison to a known magnetic map.
The Air Force/MIT team reported mixed but encouraging results: The system tested on the C-17 was accurate to around one kilometer (0.62 miles), a notable downgrade from the more accurate GPS, which can navigate a craft to within a few feet of its destination. The MagNav team pointed out a positive side in its report, though, noting that for a majority of missions, navigation to within a single kilometer is sufficient before traditional tools like visual navigation or landmark recognition kick in and are available to guide the aircraft to its final destination.
Perhaps the most promising advantage of magnetic navigation is the fact that it is virtually impossible to jam or spoof, especially from any significant distance. “Every pilot fears single points of failure,” explained Maj. Kyle McAlpin, AIA MagNav liaison, in the Air Force’s MagNav test announcement. “Our strategy documents lament the DoD’s overreliance on GPS, a single point of failure in our ability to navigate precisely.” McAlpin pointed out that every flight demands what he called “unassailable positioning and navigation.
“We can achieve that by augmenting GPS with alternatives like celestial navigation, signals of opportunity, visual navigation, and magnetic navigation,”
he asserted, saying that the MagNav demonstration was an important step toward making that a reality.
Also, unlike GPS, the MagNav system relies solely on the equipment carried on the aircraft and not on external equipment like satellites or communication equipment, both of which can be targeted or jammed.
Additional benefits of a future, more mature MagNav system would include the fact that the earth’s crustal magnetic field remains fairly stable over time, plus they can also be found in the world’s oceans, which cover two-thirds of the earth’s surface; this feature could make MagNav even more beneficial to the military, which operates at sea at least as often as over land.
The gains seen in the AI-MagNav trial, according to the Air Force announcement, are potentially the first critical step toward implementing the system across such military platforms as aircraft, submarines, uncrewed aerial systems (UASs), and hypersonic glide vehicles.
Personnel from the Air Force Research Laboratory Sensors Directorate, Air Force Institute of Technology Autonomy and Navigation Center, MIT, and MIT Lincoln Laboratory supported the AI-outfitted demonstration.
The U.S. Department of Defense (DoD) is changing the way it does business. Recognizing the need for speed and agility to rapidly onboard ever-evolving commercial technologies to benefit the warfighter, it has promoted a modular open systems approach (MOSA) and, in significant respects, reframed its business model.
The DoD’s new focus on industry collaboration and outreach has spawned an entire ecosystem of innovation units focused on transitioning commercial technology at scale (e.g., Defense Innovation Unit (DIU), AFWERX, SOFWERX, NavalX, Army xTechSearch). Defense industry players have started to pivot as well. One of these is Lockheed Martin, which has reorganized its team internally, opened up innovation cells, and embraced MOSA in several of its space projects. At the 38th Space Symposium (held in mid-April 2023), the Lockheed Martin team told attendees how it views space as anything other than business as usual.
Similar to the U.S. Department of Defense (DoD) and its innovation hubs, Lockheed Martin instituted a national network of innovation centers, with the aim of enabling industry, government, and academia to develop technology together. At its OneLM Innovation Center at the Catalyst Campus for Technology & Innovation in Colorado Springs, Colorado, for example, the company hosts industry days, community days, pitch days, expos, internship programs and an AFRL-sponsored Sherpa Program that guides campus cohort companies through an accelerator process and facilitates connections with potential end users from the DoD and other agencies.
The company also created a $400 million Lockheed Martin Ventures Fund to strategically invest in companies developing cutting-edge technologies in mutually beneficial core businesses and new markets. Emphasis areas include artificial intelligence (AI) and autonomy, sensor technologies, quantum computing, augmented reality, space, and more. Portfolio companies gain access to the company’s engineering talent, technologies, and research and development (R&D) as well as access to the company’s international business relationships and supply chain. One such beneficiary is Skydio, which is a DIU Blue UAS-certified autonomous uncrewed aircraft system (UAS) manufacturer.
On the workforce side, Lockheed Martin recently created IGNITE, an internal rapid tech-development team, with three primary missions: exploratory R&D, innovation with acceleration, and product innovation on both the hardware and software sides.
“The goal of IGNITE is to move with speed and urgency … to show that its space tech is not only viable, but to also create it at scale,” says Dan Tenney, the company’s vice president of strategy and business development.
IGNITE – which ramped up to more than 1,000 employees – has in its sights the development of multiple internally funded on-orbit pathfinders. Among these are the LM 400 technology demonstrator (Figure 1) – the first satellite off the company’s new LM 400 production line – and the Tactical Satellite, known as the “TacSat.”
Both of these satellites have benefited from the company’s investment in three key areas: digital engineering to mature the platforms from a digital perspective, optimized production lines, and the use of flexible MOSA strategies. The leaders of both programs – belonging to the company’s modernized family of digital-enabled satellite designs have leveraged and emphasized commonalities across missions to
produce the sats faster and at a lower cost to the customer.
The LM 400, a scalable, modular midsize satellite bus, is one example of Lockheed Martin’s modernized family of digital-enabled satellite designs. The satellite was the company’s first satellite that “was truly born digital … seamlessly from laptop to hardware,” according to Paul Pelley, the company’s Global Security senior director. He refers to these digital origins as “‘digital DNA.”
“Digital DNA allows us to flex the design in ways not before possible,” Pelley explains. “It lets us do more of the work in the factory left of launch. We can lean into calibrating the bus and integrated space vehicle, before launch.”
These digital touchpoints help to mitigate schedule and safety risks, which ultimately save time and money for end users. One of the many features of digital design is the ability to rapidly reconfigure in the virtual world, instead of performing costly rebuilds in the physical world that just get scrapped.
Using digital models, the Lockheed Martin team designed the LM 400 to conduct a variety of Joint All-Domain Command and Control (JADC2)-relevant missions, including remote sensing images, radar persistence, and surveillance. The LM 400 can carry out these missions across all orbit regimes: low Earth orbit (LEO), medium Earth orbit (MEO), and geosynchronous orbit (GEO).
The farther away from the Earth, the harsher the radioactive environment. Using digital models, Lockheed Martin has been able to infuse both higher resilience and power into the LM 400 bus, which ended up having four times the power of similar platforms, which enables higher-power payloads.
The team also built in launch flexibility: Users can launch the LM 400 in six-plus stack configurations, either solo or as part of a rideshare.
In the LM 400 design, the company kept MOSA top of mind, exploring widely accepted standards, such as Common
Payload Interface Specifications and NASA’s Common Instrument Interface electronics standard. (Figure 2.)
“By taking this open standards approach we’re able to provide even greater flexibility for our customers. As mission needs change or enhanced capabilities become available in the future, this versatility allows us to easily adapt and integrate new technology into the LM 400,” Pelley adds.
The company also used its own software-defined tech, integrating its HiveStar intelligent mission orchestration system into this bus. With HiveStar, if one satellite experiences an anomaly, another satellite can pick up the mission function autonomously. Also used was SmartSat: Similar to the iOS on an iPhone, SmartSat can push
updates and corrections to the satellite after they’ve been worked on from the ground.
The LM 400 will flow across a stateof-the-art production line located in Lockheed Martin’s 266,000-square-foot Gateway Center satellite manufacturing facility in Colorado. The bus for the first satellite just successfully completed Electromagnetic Interference/Electromagnetic Compatibility testing, ensuring that its bus components’ signals will not interfere with critical payloads during operations.
“This successful testing of LM 400 helps prove the satellite’s design integrity and operational capabilities,” says Malik Musawwir, Lockheed Martin Space’s satellite center of excellence vice president. “This is a significant accomplishment for this new satellite and the space vehicles that will follow from our advanced digital LM 400 production line.”
The next step is thermal vacuum (TVAC) testing, meant to ensure that platforms will work under the extreme thermal stress across all orbital environments. The first batch of three buses will be used in Raytheon’s missile-tracking system supporting the planned U.S. Space Force (USSF) Missile Track Custody in MEO. The USSF has set 2026 as the target date for this capability.
A bit closer to Earth, and with a shorter time horizon, Lockheed Martin plans to launch its Tactical Satellite (TacSat) during 2023. It provides advanced, reconfigurable connectivity, sensing, and processing designed to interoperate with tactical systems in Joint All Domain Operations (JADO) activities and military demonstrations of cross-domain F2T2EA [Find, Fix, Track, Target, Engage, Assess] kill-webs. It will be the first Lockheed Martin platform to provide tactical LEO-based 5G network communications to users on multiple platforms for space-enabled warfare. (Figure 3.)
John Schierling, director for Lockheed Martin’s Tactical Space Programs, states that “The TacSat began with the end in
mind for the tactical warfighter. We made it to address the growing demand of warfighters to prosecute maneuvering threats at long ranges in contested and denied environments on compressed timelines.”
The Lockheed Martin team designed the TacSat with MOSA as one of its two core prerequisites. “It had to be interoperable with the existing weapons systems our forces already have,” Shierling asserts. The other requirement was that those forces had to both own and operate it.
The company designed the TacSat in the digital world and did so in a cross-disciplinary effort. This started by funding the project at corporate level, as opposed to Lockheed Martin Space level. Schierling describes how he matched the expressed customer needs with the tech located across multiple Lockheed Martin departments: “We did what Ford does with the Crown Vic [automobile] for law enforcement. They took some things off of this thing and put some things onto that thing to create a Police Interceptor. That’s how we approached the TacSat design.”
For example, the TacSat leverages a proven infrared (IR) sensing payload, previously developed by Lockheed Martin for another domain. In the TacSat, this IR payload will be used with advanced multiphenomenology mission processing to demonstrate indications, warning, tracking, and targeting of threats.
This low-SWaP [size, weight, and power] satellite – weighing in at about 300 kg or 660 pounds, about the size of a small dorm fridge, and consuming under 300 W of power – also performs onboard processing and uses a new antenna to enable the upload and demo of a wide range of tactical waveforms and datalinks.
“This about being ‘ahead of ready’,” Schierling explains. “We can’t operate in a networked environment and remain a stovepiped platform-centric model. Satellites are just another node in a larger JADO network. Space is the interstitial tissue between platforms and effectors.”
Lockheed Martin seeks to demonstrate the TacSat in upcoming exercises to definitively prove how JADO can be enhanced from space and also to demonstrate how new ways of conducting space business will unlock unprecedented value for the warfighter. MES
SInergy interconnects are both in and out-of-the-box solutions — available in vertical and right-angle board-mount, and LVDS, High Speed, and RF signal cables. Customers can configure SInergy in 1-5 bays manufactured to suit their unique needs. Exemplifying Mil-Grade performance, SInergy is tested to stringent MIL-DTL-83513 requirements.
Ideal for Mil-Aero, Space, and Industrial applications where rugged durability and high-performance are a must. SInergy’s dependable 4 points-of-contact and 50 micro inches of gold will handle the roughest conditions on Earth or in space.
Six Bell 407GXi aircraft to be
Bell Textron Canada announced the sale of six Bell 407GXi helicopters to the government of Argentina in a government-to-government contract that was brokered between the Argentina’s defense ministry and the Canadian Commercial Corporation. The Argentinean air force and army will use the Bell 407GXi helicopters for search-and-rescue missions. All six helicopters will be equipped in a utility configuration designed to optimize rescue missions in Argentina’s mountainous terrain, the company says. Bell Textron Canada has built more than 5,600 commercial helicopters and delivered more than 1,000 aircraft to Canadian customers, the company notes. The Bell 407GXi includes technology designed for enhanced situational awareness, reduced pilot workload, and the ability to operate in all weather conditions.
The U.S. State Department approved a potential Foreign Military Sale to the government of Ukraine involving the National Advanced Surface-to-Air Missile System (NASAMS) and associated equipment, according to a recent statement from the Defense Security Cooperation Agency (DSCA). DSCA has delivered the required certification to Congress, marking the next step in the sale process.
The government of Ukraine’s request includes one AN/MPQ-64F1 Sentinel radar, along with a fire distribution center (FDC), canister launchers, secure communications, GPS receivers, code loaders, cable sets, tool kits, test equipment, and support equipment. The sale aims to support U.S. foreign-policy goals and national-security objectives by improving the security of Ukraine, according to the DSCA statement. This purchase is intended to bolster Ukraine’s ability to defend against missile strikes and protect its critical national infrastructure.
Northrop Grumman completed a Preliminary Design Review (PDR) for the U.S. Space Force Space Systems Command’s Next Generation Overhead Persistent Infrared Polar (NGP) program, according to a company statement. This PDR milestone moves the firm closer to starting production of the national defense-focused early-warning missile system. The design review process helps validate the technical approach for the comprehensive integration of the Eagle-3 spacecraft with the infrared sensor and high-bandwidth communication payloads, which are elements that are currently under development, the Northrop Grumman statement says. The NGP program involves two satellites operating in highly elliptical orbits, designed to detect and track ballistic and hypersonic missiles. By having broad coverage over the polar region, the Space Force believes that these satellites will offer an increased likelihood of detecting potential missile launches, the statement adds.
The U.S. State Department approved a possible Foreign Military Sale to the government of Australia of surveillance towed-array sensor system expeditionary (SURTASS-E) mission systems for vessels of opportunity (VOO, which refers to local vessels that may be called upon to respond to a crisis) and related equipment for an estimated cost of $207 million.
According to information from the Defense Security Cooperation Agency (DSCA), the proposed sale will support the foreign-policy and national-security objectives of the U.S., because Australia acts as one of its most important allies in the Western Pacific. The strategic location of this political and economic power contributes in large part to ensuring peace and economic stability in the region, say DSCA officials, who termed the deal “vital to the U.S. national interest to assist such an ally in developing and maintaining a strong and ready self-defense capability.”
Ground-based surveillance radars to be supplied to U.K. by Elbit Systems
Elbit Systems UK won a contract from the U.K. Ministry of Defence (MOD) to provide a series of ground-based surveillance radar (GBSR) systems to the British armed forces, the company announced. The GBSR systems, manufactured and developed in the U.K. and Europe, aim to bolster front-line threat detection for a variety of end users.
Elbit Systems UK expects to deliver 90 GBSR systems throughout 2023 and 2024, with the potential for an additional 40 systems. The portable GBSR uses digital signal processing to detect, track, and classify targets moving on or near the ground, the company says. Enhancements to the radar include an optimized stabilization unit and open architecture software upgrades to facilitate integration with both existing and future MOD systems, the statement adds.
Athena AI – a Brisbane, Australia-based artificial intelligence (AI)-enabled soldier decision-support company – completed its first export sale in partnership with Tomahawk Robotics (Melbourne, Florida), according to an Athena statement. The sale leverages Tomahawk’s common controller for unmanned systems, augmented by Athena AI’s Mission Module, for use by the U.S. Department of Defense (DoD), according to Athena officials.
Athena AI is an AI partner in Tomahawk Robotics’ Kinesis command-and-control software ecosystem, which enables control of robotic platforms. Athena AI is developing products, it says, to support accelerated, ethically sound targeting cycles; autonomous geospatial analysis; AI-enabled mission planning; and autonomous tasking of uncrewed air and ground platform effects.
Uncrewed aerial systems (UASs) classified as MALE [medium-altitude, long-endurance] and HALE [high-altitude long-endurance] will continue their growth path into 2031, according to a new study by Market Forecast, “MALE and HALE UAVs – Market and Technology Forecast to 2031.”
Contributing to the ongoing popularity of the MALE and HALE UAS form factor, say the study authors, will be their technological superiority, which will lead to additional spending on UAS programs and procurement.
The successful deployment of these and similar UASs in contested war zones has revolutionized military operations in battle areas and thus increased the demand for these platforms, according to the study findings. In addition, the availability of affordable UASs has enabled smaller economies to use these UASs, thereby strengthening their autonomous air power without risking human assets.
The concept of “scalable assurance” is now at the forefront of the military space electronics industry, reshaping the way rad-hard designs are developed, acquired, and employed.
In the arena of rad-hard electronics destined for use in space, there has been in the past few years a brighter spotlight on the rise of commercial off-the-shelf (COTS) components and open standards. While these developments continue to hold sway, the industry is witnessing an expanded array of demands from military customers.
The call comes now not just for components that can withstand harsh radiation environments, but for a broader spectrum of reliable solutions that can be scaled to fit the mission at hand. As the market diversifies, so does the need for a range of reliability,
packaging, test levels, and radiationperformance options.
A new frontier in rad-hard electronics Scalable assurance, in the context of military and aerospace applications, refers to the ability of a system or a component to offer varying levels of
reliability and performance based on the mission requirements. This concept involves the use of a tiered system of products that range from simple COTS devices all the way up to parts that meet stringent military standards. This scalability is also reflected in the radiation tolerance of the devices, levels of which can vary from radiation-tolerant to radiation-hardened.
The scalability of these systems enables mission designers the flexibility to use components that are so-called fit for purpose, rather than having to overspecify components to meet the most demanding environments. This approach not only enables cost savings, but it also ensures that the devices perform optimally in the intended environment.
As military customers increasingly demand versatile solutions, the industry has responded with an approach that offers flexibility without compromising on quality or performance. Bob Vampola, vice president of the Microchip Technology (Chandler, Arizona) aerospace and defense business unit, says integrators are placing an increasing emphasis on tiered levels of radiation performance.
“Military customers are looking for levels of reliability from COTS devices, all the way to QML-V and QML-Y, as well as supporting radiation performance from radiationtolerant (RT) all the way up to radiation-hardened by design (RHBD),” Vampola says. [Editor’s note: The traditional QML-V certification for radiation-hardened parts means that they are compatible only with legacy assembly processes and does not account for new-generation assembly techniques; QML-Y enables space systems manufacturers to use the latest technologies – including things like flip-chip on ceramic – while ensuring verification for each device produced.]
Turning to commercial parts
Meeting these demands while maintaining reliability isn’t easy, but the commercial chip industry and COTS components have helped. Manufacturers are offering a range of products and seeking new sources to help supply them.
The future of rad-hard electronics depends on companies like his finding cost-effective solutions to meet the evolving requirements in the world of rad-hard and rad-tolerant tech, says Anton Quiroz, CEO of Apogee Semiconductor (Plano, Texas). Apogee has dipped into the commercial space for help in that area, Quiroz notes. (Figure 1.)
“We use commercial foundries ... that not only maintain the costs low, but we get very high yield,” he continues. “Our yield for our products is very high … usually greater than 90%.”
The need for commercial foundries is driven by the challenge of creating electronics that are both rad-hard and can withstand higher voltages, which are “two things that don’t go very well together,” Quiroz notes. “So we’ve seen a push toward the area of investing more in commercial, like on feature sizes and on processes that can withstand higher voltages and be rad-hard.”
Volume also plays a critical role, Quiroz points out. “For that, you do need to run quite a bit of volume because you need to look at tens of thousands of data for tens of thousands of devices. It’s so difficult to do something like that when you’re just building ten of something,” he says.
Adding to the complexity brought by military space requirements is the ongoing global semiconductor supplychain challenge. This has had ripple effects in various sectors, including military space applications. Fortunately, space applications have been less disrupted due to the inherent nature of the industry, Vampola says.
“Because military space applications require components which typically have longer lead times, customers are accustomed to ordering devices with plenty of buffers,” he explains, adding that the key lies in the precise management of inventory to cater to industry needs amid supply chain hurdles.
Even so, supply chain issues continue to plague the industry. Given the intricate nature of these electronics, waiting for chips means projects can be stalled for extended periods. This delay can result
in cost overruns, delayed deployments, and potential loss of competitive edge, making this issue a crucial concern for industry stakeholders.
As a result, some in the industry are taking matters into their own hands by creating new ways to streamline their operations. Joel Placito, chief engineer at Aethercomm (Carlsbad, California), says his company has figured out ways to adapt to the shortage and develop radiation-resistant technology despite the long lead time for chips. (Figure 2.)
“I would not say I’ve seen the shortening [of the supply chain] just yet,” Placito says. “When the supply-chain challenge [initially] hit us, it forced us to revamp the way we do business in engineering, in particular. We sat down and had to flowchart our entire process and find ways to involve the purchasing and planning department early in the process because it was going to destroy all our schedules.”
The company focused on the availability of components, which is “just as important as performance and anything else,” he adds. The company now designs and develops around parts that they know already exist and they have access to, and they even have a space-parts library to ensure easy access to those parts in response to the uncertain supply chain. This approach is yet another arm of the scalable assurance method.
Although the demand for rad-hard solutions to enable artificial intelligence (AI) in space systems – AI being “the” hot topic in nearly all other electronic and other realms – is not quite at fever pitch yet, the industry is in fact bracing for it.
Sponsored by Renesas
We’re all familiar with cloud-based natural language recognition, but what can designers do to enhance the usability of their products with voice if people are not connected?
In this webcast, get an overview of the Renesas ecosystem around voice user interface (UI) algorithms that run efficiently on their MCUs – with resources and cycles left over for designers’ applications on the same device. Attendees will also learn how the company’s acquisition of the RealityAI edge AI software development environment can improve newly designed products.
(This is an archived event.)
Watch this webcast: https://tinyurl.com/ynkh96v4
intended to deliver radiationhardened, space-qualified hardware at TRL-9 (the highest level of technical readiness) across low-Earth orbit (LEO), geosynchronous Earth orbit (GEO), and lunar missions to support global cross-domain warfighter connectivity.
there are a host of new opportunities in the rad-hard electronics industry, and companies are aligning themselves accordingly.
“The push for a lot of the constellation work really just aligns wonderfully with our product mix and our business model,” Placito says.
For Vampola, “everything is in play,” with space exploration extending to “space sciences, manned flight, interplanetary travel, cislunar, proliferated LEO, and lunar LEO.” (Figure 3.)
With a focus on scalable assurance – in whatever form that might be – the industry is gearing up to provide solutions that cater to diverse needs while maintaining reliability. While reliability in space is a challenging path fraught with technical and logistical hurdles, Placito believes that he and his peers are ready: “We’re excited to see how that’s going to develop and how we hopefully can continue to support that.” MES
Placito suggests that while they aren’t seeing much yet in the way of requirements on the AI front, “as those requests come in, we will be able to utilize and transfer again some of that background and technology over into – hopefully – some of these coming space applications.”
On the other hand, Microchip’s Vampola notes the critical need for onboard processing of data, and thinks that AI may be able to help in this area. Microchip is positioning itself to provide solutions to enable AI in all types of space systems, he says.
It is important for warfighters to have “the ability to download intelligence rather than raw data, as the download links do not have enough bandwidth to handle the larger data processing that occurs with newer high-resolution sensors,” Vampola explains.
From the resurgence of space exploration to the development of constellations,
NASA first selected our chip resistors for a mission to Jupiter and Saturn that launched in 1977 and is still operating in interstellar space. Our chip resistors continue to be selected for high reliability applications on Earth and in space. On Earth, our resistors are selected for mission critical applications in military weapon and defense systems. In space, our chips are found in weather and communications satellites and on missions to Mars, Jupiter, and Pluto.
Mission Critical?
Choose State of the Art resistors.
RESISTIVE PRODUCTS
Made in the USA.
Currently, the military relies on nonrobust satellite connections that lack sufficient bandwidth. The rollout of satellite networks in low earth orbit (LEO) eliminates these hurdles by lowering the barriers to entry. A new wave of LEO megaconstellations is emerging as a result, creating
a growing opportunity for optical communication as the need for bandwidth grows exponentially. To take advantage of the benefits of optical communication, the easiest path for the satellite industry is to leverage commercial off-the-shelf (COTS) components. However, the risks and costs within the space industry are unique: Before a COTS component goes into orbit, it requires comprehensive testing to ensure it can reliably perform in space. From an engineering perspective, overcoming the harsh environment of space is a significant and mission-critical challenge.
This graphic depicts satellites in low Earth orbit (LEO). The rollout of LEO satellite networks drastically lowers the barriers to space entry compared to previous satellite systems. The space race is accelerating the need to quickly deploy new technologies and systems, prompting a move toward optical photonics communication systems that leverage commercial off-the-shelf (COTS) components.The need to access space for navigation; communication; surveillance and reconnaissance; remote sensing; internet access; and space experimentation has never been greater and shows no sign of abating. Optical communication, which uses light to transmit information, provides the aerospace and defense industry with several advantages over traditional radio frequency (RF) communication. These benefits include increased bandwidth, higher frequencies, improved security, and the ability to transmit data without signal degradation.
Deploying optical links connects satellites and establishes a more resilient and secure network for flexible data transfer over longer distances. By leveraging optical links, the industry is
hoping to eliminate the bandwidth and latency issues associated with traditional satellite systems.
Today, entrepreneurs and enterprises are fueling explosive growth and innovation, creating a “gold rush” to space. Technology advancements are the catalysts for much of this development, while the increase in competition has upended the space economy, leading costs to fall substantially. The following trends have lowered the barriers to entry, fueling rapid growth:
› reduced hardware costs
› reusable launch rockets
› densification of electronic components
› more standardization when connecting satellites to rockets
As the space rush intensifies, organizations are striving to be first to market by turning to COTS components widely deployed in other sectors. The adoption of COTS components reduces costs and speeds deployment; as the industry becomes more commercialized, the need to quickly integrate proven technologies will only continue to grow.
Taking advantage of components and technologies that are commonly used in terrestrial fiber-optic communication is an obvious path to minimize development costs in optical communicatios while optimizing performance. The benefits of this approach include the proven functionality and durability of both components and technologies for communications. Because the components are standards-based, designers also can use components from different manufacturers. Other advantages to already-proven parts include industrialized production, which typically enables rapid assembly and shorter lead times, as well as specifications and performance characteristics based on the volume of components and systems manufactured.
New satellite systems launching in LEO integrate COTS components to speed data transfer. To understand the growing need for COTS components, look at how satellite communications systems have changed over the last two decades. Figure 1 displays the entire communications chain of a simplified satellite communications system. The bent-pipe transceiver performs the following unique functions:
› receives the transmitted signal;
› filters and downconverts the signal to a lower RF frequency;
› amplifies and retransmits the same signal down to a receiver station.
The signal goes through amplification, filtering, and demodulation to pass the data into the network. However, these simplified systems have some limitations, including spotty connectivity. These performance issues pose challenges across industries, but especially in the defense sector. Military personnel must be able to access missioncritical insights on the battlefront or in remote locations at all times.
Figure 2 illustrates a more modern LEO satellite with internal-processing capabilities that enable it to change its function over time. In addition to the simple bent-pipe transceiver, modern systems can perform data processing within the satellite for data channelization, processing and even passing intelligence into the space network for optimized data transfer.
Embedded capabilities are key to the success of networked mega-constellations. The addition of intersatellite links (ISL) enable the implementation of traffic routing in the satellite network. With the majority of satellite launches now comprising constellations or groups of satellites, these systems will be in constant contact with their counterparts on the ground. Many will also communicate with one another directly. The systems’ increasing sophistication signals a more mature era for ISLs and helps make a case for optical communication in space.
Figure 3 shows an optoelectronic satellite communications system. In the model, the laser becomes a localized reference for the ground-station telescope. The laser gets
fed to an electro-optic modulator, and the data gets transmitted and encoded into the optical signal. The signal is then passed through the telescope optics to the satellite, which links its telescope and local references to an optoelectronic receiver. The satellite efficiently converts the data from optical to digital to enable processing before it converts the data back to optical and routing it in the relevant direction.
By using light to transmit data, optical communication offers specific advantages including achieving higher data rates. Occupied spectrum and interference with other systems are often communication hurdles when working with RF or microwave frequencies. These concerns are alleviated with the localized laser source and A-to-B communication associated with optical photonics. The benefit is that organizations can encode over wider bandwidths and obtain higher data rates.
Using a localized laser source is crucial to achieving the next frontier in satellite communications, as demand has recently begun to outpace the bandwidth provided by RF. The average transmission is currently tens or even hundreds of gigabits per second, and this is only expected to grow in tandem with new aerospace and defense innovations. In addition, optical communications are expected to play a critical role in supporting new Earth-observation technologies like synthetic aperture radar (SAR), a technology that enables organizations to send raw data to terrestrial applications in a highly secure manner.
Optical communication also supports increased security: SAR is just one of the use cases underscoring the importance of security. Optical communication’s tight, precise laser beam is much narrower than RF, meaning that the pointto-point communication of data is more secure. The laser beam protects optical links more effectively from eavesdropping, is more resistant to jamming and spoofing, and is better suited for confidential and military communication.
Figure 3 | In this example of an optoelectronic satellite communication system, the laser becomes a localized reference for the ground-station telescope. (Image courtesy of Keysight.)In addition, a laser’s physical makeup means that optical communication reduces energy dispersion in space. Unlike with RF, there is no need for a phased-array antenna for beam steering. Instead, optical communication uses fast-steering mirrors along with gimbals to ensure precision pointing with less energy dispersion.
As industries adopt optical communication, optical inter-satellite links (OISLs) will combine with intelligent routing to form a secure, robust network that rivals the terrestrial internet in speed and efficiency. Data remains in orbit, and artificial intelligence (AI) algorithms determine the most efficient ground station on Earth to deliver the information to the end user.
However, the industry must deploy new technologies and systems to realize this future. The most efficient and proven way to accomplish this is by using COTS components to accelerate deployment while keeping costs down. The use of COTS comes with one significant caveat, however. These technologies were not designed for space: a harsh environment replete with extreme temperature fluctuations, radiation exposure, and debris. Manufacturers need to ensure their COTS components can withstand these and other space-specific conditions without negatively affecting performance or reliability.
One of the best ways to determine whether COTS components can survive space conditions is by replicating space conditions on Earth and testing performance in the lab. Organizations can create simulated versions of systems and subsystems to ensure their COTS components can withstand any conditions in space. These emulations, known as digital twins, can significantly accelerate the development and decision-making processes. Digital twins enable designers to identify and address issues in the design phase rather than after the component is incorporated into a space system. Digital twins can also expose mission risks that might not be evident via traditional testing solutions and may also enable insight into how various COTS components will perform together in space conditions.
By bringing space into the lab and creating digital twins, COTS manufacturers can make better models, validate designs as they build the hardware, reduce the likelihood of failure in orbital demonstrations, and – ultimately – accelerate deployment. Looking at satellites specifically, power and the dynamic channel are two areas of operation that have a bearing on validating COTS optoelectronic systems. A satellite has no power source in space, and it must be self-sufficient once in orbit. Leakages or drains that exceed the satellite’s ability to generate and store power are tantamount to equipment failure. To test for power weaknesses in the lab, solar-array simulation can be performed to provide realistic power to the systems and subsystems, combined with power-analysis hardware that monitors the power distribution and current draw in an emulated environment.
For dynamic-channel emulation, it is critical to examine how Doppler, absorption, and scattering occur over time between communicating terminals – and also how these change based on orbits. For example, these factors may not be very important with ISLs as relative movement between satellites in the same orbit has minimal impact. However, linking with satellites in other orbits and to ground stations can impose higher Doppler and fading; users must test for variances to ensure they will not impede communications after launch.
It is also essential to test for launch vibration and forces, radiation damage, outgassing, space debris, and the components’ ability to withstand temperature extremes. Assessing performance across these areas and collecting the corresponding measurement data throughout simulation can give COTS manufacturers a solid foundation for in-orbit demonstration and – assuming all goes well – launch. A receiver optical sub-assembly (ROSA) may be used to convert an optical signal to an electrical one. A typical goal when testing this application is determining the wavelength-dependent responsivity of the photodiode and its polarization-dependent loss.
The space gold rush is creating an array of possibilities, and organizations are racing to deploy new technologies and systems. Optical communication is just one path to transforming satellite systems. Using light to transmit data in space brings significant benefits, including higher data rates, improved security, and lower energy dispersion. As organizations build large satellite constellations, they are increasingly turning to COTS components to reduce costs and speed up deployment.
For manufacturers looking to harness COTS, the ability to evaluate if those components are space-ready is the pathway to participating in the boom. As the space industry becomes increasingly commercialized, the need to quickly integrate proven technologies that perform reliably in space will continue to grow. By emulating and evaluating on the ground, it can be determined whether COTS components are ready for the rigors of space. MES
Nancy Friedrich is an aerospace and defense industry solutions marketer at Keysight, specializing in areas ranging from space/ satellite to EMSO, 5G, and military comms. Previously, Nancy was editor-in-chief of Microwaves & RF magazine.
Keysight
https://www.keysight.com/us/en/home.html
Digital twins enable designers to identify and address issues in the design phase rather than after the component is incorporated into a space system.
Enabling artificial intelligence in military systems
ChatGPT – an artificial intelligence (AI) chatbot – has emerged as a potential game-changer in the adoption of AI across all industries. For aerospace and defense, it can assist in increasing automation and data-driven analysis from the supply chain to aftermarket services.
In today’s rapidly evolving digital landscape, artificial intelligence (AI) is changing the way businesses in all industries operate. Aerospace and defense (A&D) is no different: AI solutions can increase operational efficiency, enhance safety, and amplify human teams.
Chatbots, AI-enhanced computer programs that simulate real-time conversation with humans, have emerged as powerful tools that have already been adopted in a wide array of industries, from ecommerce to healthcare to hospitality. Chatbots leverage natural language processing (NLP) and machine learning (ML) algorithms to understand user input and provide relevant responses.
ChatGPT, arguably the best-known chatbot today, is a versatile tool for many industries looking to automate tasks and enhance productivity. Developed by AI
As artificial intelligence (AI) advances, language models like ChatGPT will revolutionize defense acquisitions and contracting by automating repetitive and time-consuming tasks. Image courtesy U.S. Army/Lt. Col. Robert Solano.research lab OpenAI, ChatGPT is a large language model based on deep-learning technology and trained on vast amounts of data, infusing it with the ability to understand the context of a conversation or other interaction. Its core API is available for developers interested in incorporating this functionality into their own proprietary systems.
But is there anything radically new with ChatGPT? Not really, it could be argued. It does use a model (known as “transformer”) at its core, which means it analyzes information at the sentence level as opposed to individual words. But the main differentiators is the massive data set of information it pulls from and the scale of the user base (which will, in turn, create even larger data sets of information).
When thinking about AI applications for the A&D industry, automated robots and autonomous aircraft are more likely to come to mind than chatbots. However, where robots and self-navigating jets might still be years in development, chatbots are assisting engineers and others in the industry today.
This industry, like others including the medical and automotive sectors, requires strong certification. At the center of this requirement is proving that system requirements match completely to the implementation’s behavior. The nature of AI/ML models like the one at the core of ChatGPT is that it is impossible to reverse-engineer the process to determine why the output was produced a particular way. Overcoming this barrier will be the key to how broadly the technology can be adopted into the mission-critical systems that keep humans safe and secure. So, it is unrealistic to expect an immediate removal of a human pilot (either in the plane itself or flying the craft from a remote position) anytime soon.
The consulting firm McKinsey found that more than half of A&D companies it surveyed on the subject have at least started to use AI, deploying it across R&D (71%), supply chain (65%),
manufacturing (61%), support functions (57%), and aftermarket services (51%). Let’s dive deeper into how a chatbot like ChatGPT could be applied in these areas.
1. Research & development: In the design and planning process, chatbots can streamline a number of functions, including concept ideation, material selection, and design validation. For example, engineers could use ChatGPT to generate new ideas for airplane features by providing it with the design specifications or performance goals, which the chatbot would then use to provide information on existing features or new ideas to incorporate into the design.
2. Supply chain: Chatbots can streamline a manufacturer’s supply chain by providing real-time updates on inventory levels and production or delivery schedules. This information can then be shared with suppliers, other manufacturers, and distributors, enabling them to make informed decisions and avoid delays. Chatbots can also use predictive analytics to forecast supply and demand, enabling customers to maintain the correct inventory levels and optimize production schedules. This use of AI can help avoid running out of a specific part (or sitting on overstock), reduce costs, and improve efficiency.
3. Manufacturing: On the factory floor, ChatGPT can help detect defective components by analyzing data from various sources such as sensors, visual inspections, or testing data. It can alert quality-control personnel to any potential issues early in production to avoid costly errors down the line. Additionally, the technology can assist in identifying the root cause of defects by analyzing data and identifying patterns, helping quality-control teams understand what’s behind the defect and decide on appropriate corrective action.
4. Support functions: Streamlining administrative-support functions such as HR [human resources] and finance is an ongoing process for any business, and A&D companies are starting to take a page from other industries and examine digital ways to increase efficiency. ChatGPT can be used by management to analyze employee-retention trends to identify those at greatest risk of leaving, by procurement to automate parts of the bidding and proposal process, or by finance to analyze workflow for better cost management.
5. Aftermarket services: The McKinsey report found that the services groups remain laggards in terms of their adoption of AI. Even so, ChatGPT and other AI-enhanced platforms can be used to analyze data to better understand which services lead to greater customer lifetime value, to streamline customer support, or to develop and manage a blockchain to create transparency across the entire product life cycle, from supply chain to service records. (Figure 1.)
Military Embedded Systems focuses on embedded electronics – hardware and software – for military applications through technical coverage of all parts of the design process. The website, Resource Guide, e-mags, newsletters, podcasts, webcasts, and print editions provide insight on embedded tools and strategies including technology insertion, obsolescence management, standards adoption, and many other military-specific technical subjects.
Coverage areas include the latest innovative products, technology, and market trends driving military embedded applications such as radar, electronic warfare, unmanned systems, cybersecurity, AI and machine learning, avionics, and more. Each issue is full of the information readers need to stay connected to the pulse of embedded technology in the military and aerospace industries.
militaryembedded.com
It is important to note that ChatGPT is just at the start of its journey. The nature of these systems is that as the model gets refined or trained, the answers will continue to improve. That being said, let’s think more broadly about what might be possible.
We are also seeing ChatGPT create software applications. Could some version of this tool help convert legacy code into newer, better-supported languages? The nature of our industry is that code is expected to run for decades. People move on or retire. Could there be an opportunity to help move Ada code into a language like Rust?
Aircraft and unmanned aerial systems contain hundreds, if not thousands, of sensors, cameras, radar, and other systems that generate vast amounts of data that can be used to detect potential threats. These threats can range from a potential system failure to a network vulnerability. Systems can learn what “normal” looks like and then identify unusual data patterns and abnormal behaviors that might indicate a security breach or threat.
To accomplish this task, the technology could be trained to monitor network traffic to detect potential cyberattacks. Unusual network activity, such as spikes in traffic or unexpected patterns in data transmission, could indicate a looming threat, and ChatGPT could be trained to alert a human security engineer to investigate further.
In addition to monitoring an organization’s network and data traffic, ChatGPT can also be used to scan external sources of information, take note of global cybersecurity incidents and patterns, and identify potential sources of threats, such as suspicious IP addresses. This data can then be fed back into a predictive-analytics algorithm to recognize potential vulnerability to emerging threats.
One of the gates determining how broadly AI and ML will be deployed in systems is how/if certification processes adapt. We have seen proposals in Europe that provide guidelines as to how to
embrace AI and ML as a powerful assistant to humans. This process will evolve, and we will see a time when AI and ML are at the core of mission systems in the air. Unlike the automotive market, which has struggled to achieve its vision of fully autonomous travel, it is arguably easier in the air, primarily because there are fewer unpredictable items (like humans stepping off the curb) to worry about. For this to become a reality, however, the technology will need to evolve to a point where the AI/ML decisionmaking is bounded – both in time and in terms of impact on other systems – and the system can be proved to be fit for purpose.
By all accounts, the A&D industry remains in the early exploration stage of implementing AI. But the sector has always been one of innovation. While perhaps the industry isn’t at the forefront of adoption, A&D stands to reap significant value from ChatGPT and other forms of artificial intelligence. Industry reliance on paper-based systems, siloed operational platforms, and other traditionally manual processes will be challenging to change. However, new generations of engineers and approachable AI platforms like ChatGPT may smooth the runway toward more widespread adoption.
MES
Ian Ferguson is vice president of marketing at Lynx Software Technologies, responsible for all aspects of the outward-facing presence of the company to its customer, partner, press, and analyst communities. Ian also is responsible for nurturing Lynx’s partnership program to accelerate the company’s engagement in mission-critical systems. Readers can contact him at iferguson@lynx.com
Lynx Software Technologies • https://www.lynx.com/
Robotic vehicles play increasingly important roles on the tactical battlefield. In addition to larger unmanned aerial and ground vehicles (UxVs) carrying armaments for open warfare, smaller man-portable vehicles are now being used for intelligence, surveillance, and reconnaissance (ISR) missions. With the addition of the onboard artificial intelligence (AI) needed to overcome the many threats thrown their way – both physical and electromagnetic (EM) – human-machine teaming is rapidly becoming a reality.
Tactical unmanned aerial systems (UASs) must work in hostile environments and must work to detect and avoid threats while operating without GPS. Fortunately, advances in artificial intelligence (AI) hardware and software are
making possible smaller nano drones with greater intelligence. Compact AI technology and supporting software is providing the capabilities needed to enable nano UASs as well as larger UxVs [unmanned aerial and ground vehicles]
to overcome multiple threats on the battlefield from safe standoff distances. Systems are able to become smarter with each mission and to share these learned behaviors with one another, creating more efficient and capable platforms.
AI-enabled UxVs are useful for many nonmilitary applications as well, enabling first responders, police, and firefighting professionals to help others while reducing risk to themselves.
Out of necessity, nano UASs carry smaller payloads but cannot afford to sacrifice key capabilities in tactical situations. The ability to communicate processed sensor data on-device, for example real-time video object detection made possible by AI and machine learning (ML), cannot be compromised due to a smaller airframe. In intelligence, surveillance, and reconnaissance (ISR) applications, where intelligence provides an advantage, gaining as much edge AI capability as possible is a benefit and can mean life-or-death outcomes.
Even a smaller UAS, when enabled with a powerful AI central processing unit (CPU) capable of running advanced AI and ML algorithms, can act as an extremely sensitive measurement probe within hostile operating environments. Adequate AI and ML processing power enable simultaneous analysis of multiple video and sensor inputs, such as potentially hazardous chemical mists or classification of visible and thermal images of unknown objects, by comparing them to known references stored locally in computer memory.
As miniature UASs are being developed for clandestine ISR, electronic warfare (EW), and other tactical operations, common component use simplifies deployment while AI capabilities enable navigating in hostile operating environments; one example is a situation in which GPS satellite signals are jammed or unavailable. A nano-sized AI-enabled UAS can now operate reliably inside buildings as well as outdoors. Within a building, these small craft face the challenges of no GPS, unknown and unstructured environments, multiple threats, and complex communication due to reflections and jammers. The ability to carry a full sensor suite, including optical and thermal cameras and onboard machine vision (MV) software, can provide dependable unpiloted navigation through an unknown building while providing a real-time map for others to follow. Multiple sensors drawing upon the assistance of AI algorithms for navigation and direction, using techniques such as simultaneous localization and mapping (SLAM), is used by the UAS to build a map and localize itself in that map at the same time. SLAM algorithms enable the vehicle to map out unknown environments and carry out tasks such as path planning and obstacle avoidance.
For years, military UASs have been evolving towards smaller electric-powered systems that can be carried by a single soldier. In addition to fixed-wing aircraft operating from a landing strip, portable rotary-wing drones that can be folded have been developed for ease of transport. Modern vertical takeoff and landing (VTOL) aircraft – including rotary-wing aircraft, fixed-wing aircraft, and hybrid combinations of the two aircraft structures – do not require runways for takeoff or landing operations.
Tactical AI-enabled portable VTOLs and cargo-pocket-sized UASs are often referred to as nano drones and can now be considered for in-building as well as outdoor surveillance and full ISR missions. Payload electronics miniaturization enables nano UASs to perform specialized tactical sensing missions without detection. They can operate with almost imperceptible visual and audio signatures for secretive missions in buildings, confined areas, and outdoor urban environments. Depending upon the mission, the payload capabilities may match those of larger UASs used for similar missions, but from hardware a fraction of the size of the larger UASs. At present, smaller UASs and their ground-control systems (GCSs) are being designed to fit into cargo-pocket-sized cases that can be transported to a battlefield (or field of interest) on board-standard all-terrain tactical vehicles or by dismounted soldiers.
When flying a tactical payload into a battlespace or contested airspace of any kind, smaller drones must achieve functions essential to all tactical UASs, such as real-time communication of sensor data from the UAS to GCSs and authorized recipients. UxVs can now be controlled by compact GCSs such as a body-worn end user device or
as large as a multiuser command post system; in any case, the need for reliable, instantaneous information from the UxV is critical in all cases.
Operating conditions may vary, but most UxVs must communicate wireless video and data from onboard cameras and other sensors. Latency is critical to be effective in keeping UxV operators and troops out of danger. Operators remain at a safe standoff distance from a few meters to more than 10 km (6.2 miles). Nano UAS endurance is determined by the nature of the mission as well as AI loading and the number of onboard electronic devices drawing power from the rechargeable battery cells. Minimizing size, weight, and power (SWaP) of all onboard components is especially critical in small and nano UAS systems and imposes limits on the size, power consumption, and capabilities of the UAS’s payload components. As part of flight navigation, for example, collision avoidance can be achieved through AI-driven time of flight (TOF) cameras, light detection and ranging (lidar), and sonar systems. For counter-UAS purposes, image resolution for detection and recognition must be of sufficient quality to differentiate a troop’s drones from an adversary’s UASs.
With sufficient onboard computer processing power, the AI process of comparing images of detected objects to known references can take place on device. When desirable, AI enabled UASs can orchestrate and coordinate movement between themselves in drone swarms to achieve a result. The use of a modular open systems approach (MOSA) simplifies interoperability while common interfaces enable payloads to be utilized across a wide range of platforms.
Strong networking capabilities in hostile radio-frequency environments is critical to AI-enabled UxVs being able to coordinate with one another and share real-time video and data with troops that depend on it. Radio electronics miniaturization enables mobile ad hoc network (MANET) communication systems to be incorporated on nano UASs, expanding the “edge” of the network “cloud.” Software-defined radio (SDR) technology provides the radio communications flexibility to adapt to jammers
and changing communications environments. Coupled with advanced antenna technologies – including low-power, electronically steered and mesh antennas – multipleinput, multiple-output (MIMO) SDRs enable reliable control and monitoring of even large swarms of nano drones under hostile conditions.
Compact integration is one key to fitting required AI and ML processing power, servo controllers, sensors, and communication systems into the smaller spaces of shrinking UASs. The Artificial Intelligence Mission (AIM) system (AIMind) robotic control unit for UxVs including nano drones is the result of densely packed, vibration isolated components while paying close attention to the placement of active devices to minimize power consumption and thermal footprint but to also limit electromagnetic interference (EMI).
The AIM system is comprised of VOLT, a lightweight power “wedge,” GCS, a bodyworn end-user device like the Samsung Galaxy S20 TE; and the wearable AITN module, a tactical radio with the AIMind computer. UxVs equipped with the 89 g (approximately 3 ounce) AIMind robotics control unit (Figure 1) are controlled by GCS running the Android Tactical Assault Kit (ATAK) and the ATAK UAS Tool plugin. With the AIMind nano UAS, AIM weighs less than 2 pounds and can easily be carried with other gear. By utilizing native ATAK UAS control software, live-streaming video and data is available across the secure ATAK network.
AIMind UxVs with AI/ML technology and control software can be used to create indoor and outdoor UxV swarming missions. Payload sensor data is processed ondevice by AI/ML algorithms with or without GPS or connectivity to ground control. Modular payloads are designed to fit the smallest tactical drones and include scanning lidar, 12-channel chemical detection, ultrasonic sensors, and EO/IR [electro-optical/ infrared] cameras. The payload gains impressive control and analysis capabilities from the on-device robotic control unit. The software provides fully integrated autonomous UAS navigation using multiple sensors for AI-enhanced object detection and classification. Working with integrated SDR radios, enabled UxVs coordinate using MANET communications at data rates to 92 Mb/sec over distances from 2 km to 10 km (1.24 miles to 6.2 miles).
UxVs equipped with AI technology can perform precise mapping and guidance through threat-filled indoor environments even where GPS signals are not available and enable the application of visual-augmentation system software (VAS) to manage virtual- and augmented-reality (VR/AR) algorithms. Navigation without light and visible cameras is possible using 40 KHz ultrasonic pulses and TOF lidar to create a 3D point cloud that represents the flight environment. With extensive onboard signal processing, there is no need to send data back and forth between a remote computer controller and a
drone to achieve accurate object recognition; it can be executed onboard the UAS. With the signal-processing and AI capabilities handled by software, smaller UASs can provide reliable surveillance in both indoor and outdoor environments.
Such a modular approach to adding and integrating AI/ML computing to a UAS provides flexibility and adaptability as new, smarter UxVs are developed. A modular UxV design approach simplifies the task of creating mission-specific platforms for each mission.
For example, the AIMind control unit with as many as eight cameras, lidar, audio microphones, and chemical sensors, along with the onboard AI/ML processing power can be rapidly integrated onto any PX4 [open-source autopilot software] UxV frame, whether aerial, ground, or surface of submersible. For example, flight times of more than three hours are possible with this system on an electric VTOL by careful choice of efficient wing design, rechargeable battery size, and number of sensor and other modules.
Of course, even a small UAS with the densest, most advanced AI computing engine requires consistent communications links to command stations to share valuable ISR data collected. Whether for control of single drone or in swarms, the radio links must often overcome hostile adversaries and environmental conditions, even the movement of the troops and the command post as the UASs are collecting data. Miniature concealment antennas (such as those developed by Southwest Antennas, https:// www.southwestantennas.com/ ) when onboard UASs and integrated as part of body-worn communication equipment have proven capable of maintaining MANET communications even when faced with demanding electrical, mechanical, and environmental requirements (Figure 2).
Such dual-band antennas are needed for transmission and reception between drones in a swarm as well as from a lead drone to body-worn communications equipment. An antenna built for MIMO use with vertical polarization from 2.1 to
2.5 GHz and 4.4 to 5.0 GHz frequency bands is such a candidate since it measures just 1.9 by 0.75 by 0.15 inches but can operate effectively even when closely mounted to a soldier’s body. The antennas can also be used in a slant-left/slant-right configuration
distances from danger while collecting as much sensory data as possible for successful missions. MES
Seth Spiller is president and CEO at Orion Technology Group. He has extensive engineering and international business development leadership experience working with artificial intelligence (AI) and autonomous robotics platforms. He is the co-inventor and holder of the first AI patent in 1997, technology that has been leveraged worldwide and successfully integrated in
While semiconductor device selection is at the heart of developing radiationtolerant power systems for space applications, it is just one of many design strategies that can be deployed at the component and circuit levels. There are basic strategies to explore, plus the multiple benefits of soft switching in radiation-tolerant power systems.
When it comes to building satellite power electronic systems that operate reliably in space, engineers have a plethora of challenges to overcome. The absence of Earth’s magnetic field makes it difficult for satellites to deflect high-energy particles. Moreover, without the Earth’s protective atmospheric shield, space systems are also vulnerable to greater levels of wave and particle radiation that can trigger component malfunctions and even compromise the system itself. Another hurdle: heat dissipation, as convection cooling does not work in space, thereby limiting the removal of heat through conduction to a radiating surface.
Against this backdrop, so-called New Space applications have shown they are able to incorporate radiation-“tolerant” components as opposed to more robust radiation-“hardened” circuitry, for example, by lowering the bar for total ionizing dose (TID) exposure. However, radiation damage is cumulative, so the length of the mission is a factor that determines the intensity of radiation, as does its orbital position.
Mitigating the effects of particle radiation
Wave radiation includes rays and electromagnetic waves. Generally, wave radiation has properties similar to optics, including reflection, absorption, refraction, and diffusion. However, radiation wavelengths in space extend above
and below the visible light spectrum. Radiation below visibility includes microwaves and radio frequencies (RF), while radiation above visibility includes ultraviolet, X, and gamma rays. In Figure 1, note the wavelengths and associated energies, which are key parameters for measuring radiation exposure.
Wave and particle radiation are not really two separate things, but the effects on electronic systems are different. Individual particles have little mass, but can be accelerated to high velocities. They can also carry charges, which are generally positive when negative-charge electrons are stripped from atomic orbits.
We see physical damage from particle radiation, particularly to semiconductor crystal lattices; this damage is permanent and/or cumulative. We can see
temporary upsets where electrons are dragged into depletion regions and make a nonconducting region conduct. We also see malfunctions when positive ions replace doping atoms in a crystal matrix, which can make a semiconductor conduct when or where it shouldn’t, resulting in permanent damage to equipment.
An added factor in the vacuum of space is that the useful convection used for terrestrial cooling does not work. Conduction works for spreading thermal energy, but eventually, excess heat must be radiated into cold space. A complicating factor is that surfaces exposed to the sun will get very hot, on the order of 250 ˚F (120 ˚C), while shaded surfaces will be very cold, around -238 ˚F (-150 ˚C).
Even in today’s fast-paced New Space business environment, costs to launch and replace dead satellites are substantial, so care in design is important.
How is this done? There is no one answer; solutions for creating robust space electronic systems are multifaceted.
First, components are selected for radiation tolerance. Some state-of-the-art semiconductor process nodes have improved radiation performance. Bipolar semiconductors can be selected for their displacement damage ratings. Wide-bandgap (gallium nitride or GaN) FETs [field-effect transistors] with inherent radiation tolerance can be selected. Some parts, like certain epoxies and aluminum electrolytic capacitors that outgas in a vacuum, are simply inappropriate for use in space environments.
Physical redundancy is also important to ensure that, if one system fails, another can take over. In some designs, three systems operate in parallel: If one disagrees with the other two, then its output can be ignored. Sometimes there are four redundant systems and one spare is swapped in if a system fails. Even with these safeguards, radiation-tolerant design requirements restrict the selection of components. The addition of performance monitors, safety mechanisms, power disconnects, and reset circuitry must not exceed the efficiency, size, and weight requirements of the final solution.
Regardless of design strategies and power-supply topologies, space electronic systems must be analyzed, simulated, and tested for environmental and radiation performance.
Balancing design tradeoffs by choosing an appropriate power system architecture is important. Topology and switching modes like soft switching (versus hard-switched power converters) can make a system less sensitive to parasitic effects like ringing, which can increase voltage stress on switching components.
As one example of the importance of topology selection in a new space design, the switching mode affects all the key specifications of power-conversion implementations, including power density, efficiency, transient response, output ripple, electromagnetic interference (EMI) emissions, and cost.
Dominant switching-loss terms are attributable to the turn-on behavior of a power train’s high-side MOSFET [metal-oxide semiconductor field-effect transistor] via gatecharge requirements and drain-to-source capacitance. Switching losses increase with, and thus limit, switching frequency. Body-diode conduction losses detract further from power-conversion efficiency in hard-switched converters. Though GaN FETs do not have a physical body diode, they do have a reverse conduction mode clamping at several volts. This setup makes the GaN dead-time conduction period challenging to manage.
In a synchronous, hard-switched buck topology, the high-side FET turns on when it has the maximum voltage across it (Figure 2) and it conducts its maximum current during the turn-on portion of the operating cycle. Power losses in the high-side switch, therefore, are at a maximum during the off-to-on transition. The larger the input voltage, the higher the power loss, so converters in high voltage-ratio applications (for example, 28 V to 3.3 V) tend to deliver poorer efficiency than the same converters in circuits demanding lower conversion ratios (for example, 5 V to 2.5 V).
The alternative, soft switching, significantly reduces these switching losses. Soft-switching techniques require more complex control circuits because the switch timing must be coordinated with the switched waveform.
One example of soft switching is the zero-voltage switching (ZVS) technique, which improves conversion efficiency across a range of power topologies. As the name suggests, ZVS switches the high-side FET on when the voltage across the switch is at or near zero (Figure 3a). This breaks the link between power losses and the voltage conversion ratio during the high-side FET’s turn-on interval. Operation of a clamp switch with the ZVS technique enables the converter to store a small amount of energy in the output inductor when both highside and low-side switches are off. The converter uses this otherwise-wasted energy to discharge the high-side FET’s output capacitance and charge the synchronous FET’s output parasitic.
Taking the FET’s output capacitance out of the switch’s turn-on behavior desensitizes FET selection with regard to gatedrain capacitance (CGD) and enables designers to focus on on-state channel resistance instead of having to concentrate on traditional figures of merit such as the product of channel resistance and gate capacitance.
This method of driving the high-side FET during turn-on avoids exciting the switch’s parasitic inductance and capacitance, which tend to resonate and induces large voltage spikes and ringing in hard-switched topologies (Figure 3b). By eliminating the spikes and preventing the ringing (Figure 3a), ZVS removes another power-loss term and eliminates a source of EMI emission.
Eliminating the voltage spikes from the switching behavior also enable designers to select lower-voltage FETs with lower drain source on-resistance [RDS(on)], improving the efficiency.
Soft switching is extremely versatile. For example, Vicor uses soft-switching techniques in its radiation-tolerant power module solutions for powering high-performance communication ASICs [application-specific integrated circuits] (Figure 4) dedicated to MEO and LEO [medium-Earth orbit and low-Earth orbit] satellite applications. The system modules use ZVS buck-boost topology for the PRM and ZVS and ZCS sine amplitude converters (SACs) for both the BCM and the VTM.
The small size of the VTM enables placement as close as possible to the ASIC. Optimizing the power distribution network (PDN) is critical when dealing with the high currents of modern ASICs, FPGAs [field-programmable gate arrays], CPUs [central processing units], and GPUs [graphics processing units]. Vicor modules combine soft-switching solutions, rad-tolerant active components, and automotive-qualified passive components.
To mitigate the single-event function-interrupt (SEFI), all radiation-tolerant modules include completely redundant power trains operating in parallel. If one power train is upset due to a single event, its protection circuits force a power-off reset. During the reset interval, the redundant power train carries the full load and after the reset both power trains operate in parallel again.
The bottom line: radiation-tolerant electronic systems are hard to design. MES
Salah Ben Doua has 30 years of experience in the field of power design and has been supporting Vicor for more than 20 years, providing expertise and advice in the development of DC/DC and AC/DC power systems in a multitude of areas, including aerospace and defense, industrial, rail, lighting, and communications. Salah received a Ph.D. from the National Polytechnic Institute of Toulouse (France), specializing in power conversion. Author, publisher, and engineer Ken Coffman holds a BS degree in electrical engineering technology from Cogswell College North, is the co-author of six technology patents, and has many years of power-supply design experience. Located in the Phoenix, Arizona area, he is assigned to Vicor’s New Space Initiative. Readers can reach Ken at kcoffman@vicr.com and he is easy to find on LinkedIn.
Today’s high-performance chips demand increasingly precise voltage tolerances on shrinking voltage rails and shockingly rapid, high-current load changes. In the satellite and space arenas, designers must also contend with the effects of TID [total ionizing dose] and SEE [single-event effects] on the output voltage accuracy of their supplies. Before choosing a power solution, designers playing in these spaces must create a regulation accuracy budget.
In an ideal world, a switching power supply can be set to output the precise voltage required to power a load, such as an FPGA [field-programmable gate array]. Under ideal conditions, if the input voltage or load current changes
the output voltage will remain the same. Additionally, if the temperature changes, the output voltage will remain the same. This perfect power supply will even maintain a solid output voltage when exposed to radiation in space. In the real world, however, the output voltage of a real power supply will vary over all these conditions.
The output voltage errors can be categorized into a number of errors.
Rad-hard electronics design trendsDC errors
DC errors arise from the reference voltage (VREF) accuracy, feedback resistors accuracy, line and load regulation, and PCB parasitic resistances.
Examining Equation 1 below, the key to ensuring the accuracy of the initial output voltage is to have an accurate VREF and feedback resistors values (RTOP and RBOT). The accuracy of the VREF primarily depends on the controller design. A good controller design will also minimize the error amplifier’s offset voltage (VOS) and input bias currents (IBIAS) to maintain an accurate voltage.
Eq. 1:
In addition to these sources of DC error are the line and load regulation. The line regulation refers to the change in output voltage in response to a change in the input voltage, while load regulation refers to the change in output voltage in response to a change in the load current. To minimize line and load regulation errors, the error amplifier’s open-loop (AOL) gain should be as high as possible. The final load regulation will depend on minimizing PCB trace resistances to the load.
AC errors
AC errors in a power supply include line and load transient response and switching noise.
Similar to line and load regulation, the line and load transient responses are AC changes to the output voltage in response to AC changes in the input voltage and load current. Minimizing the line and load transient response can be achieved using an
error amplifier with high AOL gain, a fast control loop response, a good compensation network, and a good input/output decoupling capacitor network. The key to minimizing switching noise is using high-capacitance, low-ESR [equivalent series resistance] capacitors at a fastswitching frequency in the megahertz range, and an appropriate value inductor to minimize ripple current.
There are unique features for minimizing the load transient and switching noise that system designers can consider when selecting a switching controller for their power-supply design aimed at use in the high-radiation environment of space.
Temperature errors
Temperature errors include VREF drifting, feedback resistor divider drifting, and temperature drifts in the error amplifier, including bandwidth, gain, IBIAS, and VOS. These errors are caused by temperature changes in the IC [integrated circuit] die.
Supports AES-256 and FIPS140-2 encryption
Looking at Equation 1 again, it is apparent that if the VREF and feedback resistor values change with temperature the output voltage will change. Datasheets typically provide information on the change in VREF over temperature. Some companies will include the errors caused by the error amplifier’s IBIAS and VOS changing over temperature. Lastly, the resistor divider temperature drift can be determined by reviewing the resistor datasheet.
Total ionizing dose (TID) errors – the abundance of high-energy particles a part will be exposed to in space – include VREF drifting as well as the error amplifier’s bandwidth, gain, IBIAS, and VOS changes. These errors are caused by the cumulative effect of energetic particles depositing trapped, positive charges in the oxide and interface regions of the IC.
Datasheets for radiation-hardened parts will specify parameter limits over total radiation dose. Additionally, radiationhardened IC providers will typically perform radiation lot acceptance testing to ensure the performance of their ICs over TID radiation.
SEE errors include latch-up, burnout, functional interrupt, and transients on the output of an IC, which are called single-event transients (SETs). SEE errors are caused by a single heavy ion depositing energy in a sensitive area of the IC.
Radiation-hardened IC providers will publish reports detailing the performance of the IC in a heavy ion environment. The reports also detail how often the IC might experience a functional interrupt or a transient in a heavy ion environment and how those events affect the operation of the IC.
It’s important to figure out all the sources of error a power supply will experience, because most high-performance ICs such as FPGAs will have specific requirements and tolerances for each of its rails. As an example, let’s look at a controller for a
power supply that can supply a max DC load of 135 A, at load transients up to 35% of DC load with a 200 A/µs slew rate, while maintaining ±4% accuracy over temperature, radiation, life, etc.
To start: Budget for a DC error of ±1% and AC errors of ±2% as an assumption. Subtracting these from the worst-case accuracy leaves a tolerance of ±1% for shifts caused by temperature, SET, and TID errors. The initial voltage regulation budget analysis is shown in Figure 1.
Regarding the choice of a controller, the ISL73847SEH PWM (Figure 2) is designed is designed for low-voltage, high-current applications such as the core rail of highperformance FPGAs.
Its ±0.67% VREF tolerance across temperature, radiation, and life includes the error amplifier’s VOS. The line and load regulation for this part is a little less than ±0.25% over temperature. This overage can be attributed to the feedback resistor divider values and temperature drift; ±0.1% tolerance feedback resistors are recommended.
The ISL73847SEH supports a switching frequency range from 250 kHz up to 1.5 MHz. The higher switching frequency will help minimize the switching noise. To further reduce the switching noise while still addressing the high load current needs of the FPGA core rail, designers can turn to a multiphase configuration, which the ISL73847SEH can support. Using a 5 V input rail for a VOUT of 0.8V gives a duty cycle of 16%. Two phases brings a 15% reduction in ripple current, with 50% for a fourphase system and around 90% for a six-phase system. A four-phase system will also be able to provide 200 A DC max current, with ±0.25% of switching noise allotted to the budget.
Figure 2 | Shown: the layout of the ISL73847SEH controller. Figure 1 | An initial voltage regulation budget analysis is shown.On its evaluation board the ISL73847SEH has a peak-to-peak transient response of ~100mV for a full-load step of 900 A/µs. These numbers can be reduced by almost 50% by adding a resistor between the DROOP and VREF pins, a feature
called droop regulation. In addition, ±2% can be allotted to load transient response.
From a radiation perspective, the part is high-performing, with no SETs up to
The military and aerospace systems marketplace is always growing and developing, as countries and organizations around the world continue to challenge one another. Companies operating in the space must constantly innovate – and at the board level, planar magnetics are one of the best and most cost-effective ways to address high-frequency circuits with high power density.
In this white paper, learn how space-saving planar transformers and power magnetics for military and aerospace applications can help designers ensure reliable performance in harsh military environments, meeting thermal and vibration military specifications. Read
a linear energy transfer (LET) of 86MeV cm2/mg and little to no parametric shift over TID.
The final regulation budget analysis is shown in Figure 3 for the ISL73847SEH, using droop regulation and a four-phase configuration. Notice how savings in temperature, radiation, and life errors enabled an increase in the budget for AC errors by ±0.25% (transient and switching noise), with ±0.58% margin leftover. MES
Juan Garcia has been an applications engineer for Renesas Electronics for four years, working in the Radiation Hardened Space Products Group. His focus areas are DC-DC switching and linear regulators.
Renesas Electronics
https://www.renesas.com/us/en
works with industry leaders to develop and publish content that educates our readers.
The demand for high-performance electronic components for the space, defense, and nuclear markets in 5G/6G communication, real-time imaging, and nuclear instrumentation is hindered by the limited availability of radiation-hardened (rad-hard) components. Two solutions exist: The first is up-screening commercial off-the-shelf (COTS) components, with the second being the use of space-graded electronics. While the COTS approach is cheaper, it requires expensive risk management and may incur high costs throughout the product life cycle. Rad-hard components are preferred, but are limited to legacy components with high costs that typically do not match COTS performance. A radiation-hardened by design (RHBD) approach can meet performance, availability, and cost requirements.
The current demand in high-performance semiconductors for 5G/6G communication, real-time imaging in defense and space markets, and high radiation-tolerant electronics (>1 MGy or milligray, a unit of absorbed radiation equal to one thousandth of a gray, or 0.1 rad) for nuclear fusion and SMRs is hampered due to limited availability and performance restrictions. While there exist strong growth drivers in the arena, the availability of components is limited, creating obstacles for the industry.
To date, two main solutions exist to utilize electronic components in harsh (radiationfilled) environments:
1. Upscreening of commercial off-the-shelf (COTS) components and/or
2. Use of space-graded-radiation-hardened electronics.
Using COTS components has the benefit of leveraging the latest technology advancements for new applications, leading to cutting-edge communication and imaging solutions. COTS electronics have been utilized in radiation environments, such as
space and high-energy physics experiments, for low-dose applications where rad-hard solutions are unavailable. This usage affects the system’s reliability. Risk management is therefore mandatory and often follows the following procedure:
› Apply sufficient shielding to reduce the level of radiation exposure
› Electronics parts selection through up-screening
› Following strict radiation-hardnessassurance policies
› Extensive radiation testing and understanding the radiation effects on the COTS components
The whole procedure is costly, and sometimes even inadequate, especially for missioncritical systems where no risk can be tolerated. The threshold for total ionizing dose (TID)-induced failure of CMOS [complementary metal oxide semiconductors] COTS components varies widely. Dose rate effects must of course to be taken into account, because these can significantly change the failure threshold. Typically, most CMOS components can stand dose levels of the order of 5 to 10 krad (1 Gy = 100 rad). Very few of them fail below 3 krad, some can make it to 30-50 krad (0.3-0.5 kGy), and a small minority can survive up to 100 krad (1 kGy). The variability in radiation tolerance is also well-known for COTS components due to variations and imperfections in the chipmanufacturing process. Another element that electronics used in space must endure: high energetic particles, which typically cause single effects; up to certain levels, these events can be prevented by implementing redundancy. However, more severe events –like single-event latchup – can outright destroy components.
Semiconductor vendors produce their components in several manufacturing plants worldwide, and it’s a fact that parts manufactured in different production lines are very likely to have different radiation tolerance. Moreover, as long as the specifications in the datasheet are met, the manufacturer can introduce as many changes as it wishes in the product without ever notifying the customers. Those changes – which could range from changing a few steps in the technology all the way up to a redesign of the circuitry in an accompanying technology – are usually not documented and may change the radiation tolerance.
Another important aspect of rad-hard is the life cycle cost: Initial procurement costs of COTS parts without radiation hardness are less than space- or military-graded parts, but many other factors – for example, added testing, characterization, markings,
binning, etc. – will determine whether the cost of ownership over the life of the system is actually reduced.
Life cycle costs are also impacted by part obsolescence: As COTS suppliers constantly introduce new products, previously introduced devices can become obsolete even within the planned product life cycle, which can place a critical or strategic application at risk.
Bottom line: While use of COTS components is possible in some nonextreme cases or noncritical systems, extreme caution must be taken to limit the risks of system failure. Moreover, life cycle costs should be considered, as a price hike during the systems’ lifetime could exceed the cost of a rad-hard component.
Those who go with solution number two –space-graded rad-hard electronics – will find limitations on the availability of the components, electronic functions, and use of legacy components, which typically cannot match the performance of available state-of-the-art off-the-shelf components.
Sponsored by Elma and Samtec
The 3U OpenVPX form factor – integral to The Sensor Open Systems Architecture (SOSA) Technical Standard – enables interoperability and commonality in military systems for radar, electronic warfare, signals intelligence, and more. Less known is that a smaller form factor is also being included in the standard. Applications in which this is needed require even further reduced size, weight, and power (SWaP), adding more challenges for embedded computing designers.
In this webcast, industry experts will examine how the SOSA standard is leveraging small-form-factor standards such as VITA 90 (VNX+) to address these smaller footprint needs. (This is an archived event.)
Watch this webcast: https://tinyurl.com/2p863jsh
Radiation-hardened integrated circuits (ICs) are available from a range of players – including TI, Analog Devices, ST, Honeywell, etc. – but the ICs offered by the majority of these players are only qualified to radiation doses of <10 kGy and are typically created in legacy processes that cannot withstand harsh environments or with older design methodologies. At the same time, components are expensive and may not even meet the power requirements or electronic specifications of COTS components available on the market.
Radiation-hardened components used in space and military applications need to withstand severe radiation effects caused by energetic particles such as electrons, protons and heavy ions. The radiation environment in space is very different from the civil nuclear radiation environment, where total-ionizing-dose level is much lower (typically below 10 kGy), but particle energy level is much higher (> 100 MeV). Therefore, space-grade electronic components are also designed with a TID tolerance of typically not more than 10 kGy. Using space-grade components in nuclear applications where the accumulated radiation exceeds 100 kGy – and can reach up to 1 MGy and higher – will require designers to take a similar approach as those who use COTS components.
In the nuclear industry, space-grade electronics can only be used in these highdose radioactive environments with the addition of heavy shielding, which leads to bulky solutions, making the design and installation of complex sensing and motion-control systems an impossible task. Such a situation – large-footprint systems – is untenable in the space environment, where small is better.
What’s needed: components that can both withstand high radiation tolerance (>1 MGy) and meet the reliability requirements of space and military markets, while at the same reaching performance levels of COTS components.
Such an approach requires the use of more advanced technology nodes available at commercial foundries worldwide, which is possible by creating semiconductors that are created by
radiation-hardening by design (RHBD) methodologies (Figure 1) through characterization of technology nodes and sophisticated modeling of transistors.
Today’s MGy radiation-tolerant integrated chips created through RHBD methodologies enable complex remote handling, automation, and predictive maintenance applications in high-radiation nuclear environments such as nuclear fusion and new reactor technologies.
In the same way, the space industry needs to use a method that ensures electronics in the radiation environment of space are resistant to damage yet remain compact, miniaturized, and high-performance. These demands are driven by the major incentive of reducing the average cost of satellites to enable construction of mega constellations.
RHBD methodologies can enable the development of highly integrated system-onchip solutions by using advanced semiconductor processes used to develop current COTS components. This approach can reduce mass and power consumption, improve performance, and ensure reliability.
In other words, RHBD is a door-opener to applications requiring extremely high-dosetolerant ICs while at the same time meeting the demanding electronic and reliability performance guidelines of the military and space industries. MES
Jens Verbeeck is an electronics engineer with a diverse range of experience in both academia and industry. He holds a master’s degree in electronics and began his professional career at Intersoft Electronics as an electronic hardware engineer. Later, he pursued a Ph.D. in electrical engineering from KU Leuven (Belgium). His research focused on mixed-signal ICs that tolerate increased temperatures and megagray radiation. This work led him to co-found (with Dr. Ying Cao) Magics Technologies, which specializes in radiation-hardened semiconductors.
While many other types of threats still exist in the wild, the rise of wiperware – a class of malware that maliciously erases data on the infected device – has sparked special concern among security teams in the public and private sectors.
Fears around the proliferation of threats emerging from the war in Ukraine are becoming a reality, with FortiGuard Labs researchers identifying seven new major wiper variants targeting government, military, and private organizations in the first six months of 2022, almost as many as the total identified since the first iteration observed a decade ago. Fortinet’s analysis shows that adversaries use wiperware attacks for everything from financial gain and sabotage to destruction of evidence and cyberwar.
One prominent wiper example is called CaddyWiper, a variant used to erase data and partition information from drives on systems belonging to a number of Ukrainian organizations soon after the war began. HermeticWiper – a tool for triggering boot failures that IT security firm SentinelLabs found being used in similar attacks – is another recent variant.
The threat has also moved beyond Ukraine’s borders with the use of disk-wiping malware detected in 24 additional countries. AcidRain, a wiper used to target a Ukrainian satellite broadband service provider, also ended up being used in an attack that knocked nearly 6,000 wind turbines offline in Germany. And of course, NotPetya, which many believe was wiper malware thinly disguised as botched ransomware, was initially launched in Ukraine in 2017 but rapidly spread worldwide and caused an estimated $10 billion in damage.
Preparing integrated responses across international governments as well as within federal agencies will become increasingly important as these sophisticated and damaging attacks become more prevalent. Collaboration in planning, preparation, detection, and response for wiper attacks will be critical to fending off attacks that could bleed not just into neighboring countries but also across continents and critical infrastructure sectors.
This is already happening in some situations. Ukraine understands how important coordination with surrounding countries will be not only in its fight against Russia, but also against any malicious cyber actors looking to take advantage of an already tenuous situation. The country signed a memorandum of cooperation with Poland as a way to stave off incoming cyberattacks. The agreement aims to both strengthen the joint fight against cybercrimes and share experiences and detailed information about cyber incidents more quickly and effectively.
Mykhailo Fedorov, Ukraine’s vice prime minister and minister of digital transformation, put a finer point on the situation: “The first world cyberwar is ongoing. Therefore, joining efforts and exchanging practices is a logical step in this area. With Poland, we have not only a common physical border but also a joint problem in cyberspace, where we experience the same kind of attacks.”
In mid-August 2022, the U.S. and Mexico announced that a cyber issues working group had convened its first bilateral cyber dialogue. The meeting’s stated mission was to advance bilateral cooperation on cyber issues to create “an open, interoperable, secure and reliable Internet and a stable cyberspace.”
The agreement includes measures to enhance technical coordination in the event of cyber incidents involving national and shared critical information infrastructures, facilitate the exchange of cyber threat intelligence data, and continue bilateral training programs for state and federal agencies in both countries.
Measures like the two outlined above are a good start in coordinating efforts, but the private sector has a big role to play as well. Governments can’t see or stop all attacks on their own, nor can they innovate to meet newer, more effective attacks at the speed and in the way that private cybersecurity companies can.
On the flip side, the private sector benefits greatly from government resources, research, and threat intelligence.
The wiper threat is real and is surmountable only if governments and industry work together. Cooperation and coordination will be key, as it often is, when talking about the future of cybersecurity. Organizations from both the public and private sectors can harness their unique skill sets to assemble a team that addresses all facets of the evolving threat landscape.
Jim Richberg is the public-sector field chief information security officer for Fortinet. Prior to joining Fortinet in 2019, he served as the National Intelligence manager for cyber, the senior federal executive focused on cyber intelligence within the $80B+/100,000 employee U.S. intelligence community (IC). He led creation and implementation of cyber strategy for the 17 departments and agencies of the IC, set integrated priorities on cyber threat, and served as senior advisor to the Director of National Intelligence (DNI) on cyber issues.
CodeMettle’s Terminus 3.0 software is designed for tactical communications, particularly for establishing and managing large-scale tactical communication nodes in contested networks for the U.S. Department of Defense (DoD). Terminus 3.0 is designed to be platform- and protocol-agnostic, enabling multiple users to operate it simultaneously, regardless of technical skill level. The software is aimed at simplifying the setting up and management of multinetwork hybrid communications kits in order to reduce the number of tools necessary to establish secure communications promptly and reliably at the edge.
The new software is also aimed at enhancing user and role authentication protocols with the goal of promoting accountability while keeping up with the changing security landscape within the DoD. Terminus 3.0 – designed for zero-trust and Federal Information Processing Standard (FIPS) 140-3 frameworks – leverages CodeMettle’s Pulse, which is intended to optimize data flow for battlefield communications and command decisions. The first public demonstration of Terminus 3.0 took place at SOF Week 2023.
CodeMettle | https://www.codemettle.com/
The Microchip Technology VSC8574RT radiation-tolerant (RT) Ethernet PHYs device is intended to streamline the adoption of Ethernet in space applications in anticipation of the aerospace industry transitioning from traditional dedicated networks to more flexible Ethernet solutions. The VSC8574RT PHY is designed for both serial gigabit media independent interface (SGMII) and quad serial gigabit media independent interface (QSGMII), which is intended to reduce the total number of signal pins in the design and free up the host device. It is compatible with both copper and fiber interfaces and features a fiber interface to meet the demand for data rates exceeding 1 Gigabit in the space industry.
Equipped with a quad port, the VSC8574RT PHY supports 10, 100, and 1000BASE-T Ethernet connections to match different device requirements, and it also includes features such as Synchronous Ethernet (SyncE) and IEEE 1588v2 Precision Time Protocol (PTP) for precise network timing and synchronization. Its specifications are designed to make it more resilient for space applications, offering single-event latch-up immunity above 78 MeVcm²/mg and total ionizing dose (TID) testing up to 100 krad.
Microchip Technology | https://www.microchip.com/
Bohemia Interactive Simulations (BISim) introduced its military training and simulation software, VBS4 and VBS Blue IG, with these offerings giving users such features as configurable radio antennas that enable users to modify and attach antennas to any vehicle in the battlespace, as well as damage charring, which is a visual effect that appears on objects and vehicles upon munitions impact and are visible in thermal imaging sensors. Additionally, the software offerings include a weather unit with detailed control over weather parameters, such as volumetric clouds and fog color.
The software also includes particle effect upgrades that enhance bullet impact effects, fire, smoke, dust, weapon effects, and explosion sizes. The software introduces mission briefing, rendering, and enhancements for developers through software development kit tools. Additionally, graphical settings in VBS4 enable customization to suit various hardware systems and user scenarios.
Bohemia Interactive Simulations | https://www.bisimulations.com/
A light-based wireless broadband solution designed for modern warfare environments emerged from a collaboration between Intelligent Waves (IW) and Signify. IW integrated Signify’s Trulifi technology with IW’s GRAYPATH – described by IW as an attack surface management solution that addresses VPN vulnerabilities to secure classified communications – as a way to create a more secure, non-RF [radio frequency] method of communication. The product is aimed at enabling high-speed communications and more efficient transmission of photos, videos, and other information to commanders.
The product leverages LiFi technology, a two-way wireless connection enabled by light that is intended for reliable, high-speed data transmissions; implementing LiFi in an operational environment is regarded as better at eliminating signals from electromagnetic sources and therefore reducing the likelihood of a transmission being intercepted or disrupted by adversaries.
Intelligent Waves | https://intelligentwaves.com/
RFeye AirDefense from CRFS is a wide-area 3D geolocation system designed to passively identify and locate transmissions from aircraft without any electronic detection. The system’s listed capabilities include border monitoring, radar augmentation, target acquisition, and detection of spoofing attempts. RFeye AirDefense utilizes a 3D time difference of arrival (TDOA) approach to achieve precise geolocation of RF emissions from entities like enemy aircraft, while avoiding any electromagnetic signature that could betray its presence. The system is designed to detect and locate transmissions, enabling individual identification of airborne targets, which is useful in both testing and battlespace environments where visibility and anonymity are important.
The system can function as a standalone geolocation solution or supplement existing air monitoring systems such as radar, and it is equipped to passively detect hostile aircraft without alerting them of being geolocated. The system is also intended to augment missile defense systems by locating emitted RF signals until the aircraft is within range. Additionally, the system is said to be able to detect unauthorized drones by identifying the RF signature emitted by their navigation and video links.
CRFS | https://www.crfs.com/
The SPECTRAN V6 MIL spectrum analyzer is capable of operating from 10 MHz to 6 GHz and offers real-time bandwidth up to 245 MHz (I/Q) [in-phase and quadrature-phase]. The analyzer is capable of monitoring frequencies, detecting signals, and analyzing and recording both, and can also detect unknown and potentially illicit signal transmissions. The product uses a Windows laptop with pre-installed software that permits real-time display and analysis of signals; it is also designed to work in harsh conditions and is MIL-STD-810G- and IP65-certified for resisting shock, vibration, dust, and moisture. Specifications listed by the company show a dynamic range of -200 to 100 dBm, an input power of 23 dBm, and an operating temperature range from -20 °C to +60 °C.
The analyzer is also able to identify hidden microphones, bugs, and surveillance devices, and it can set an alarm that activates when conspicuous signals appear in certain frequency ranges such as those used by 2G, 3G, 4G, 5G, and WLAN technologies. The device can record signals even when the display is closed and will emit a warning tone if a preset trigger has been activated, making it potentially useful for surveillance countermeasures, interference management, and signal monitoring.
Aaronia AG | https://aaronia.de/
Each issue, the editorial staff of Military Embedded Systems will highlight a different charitable organization that benefits the military, veterans, and their families. We are honored to cover the technology that protects those who protect us every day.
This issue we are highlighting Combined Arms, a Houston-based nonprofit organization launched in 2015 that helps military veterans and their families across the U.S. by providing centralized access to its network of over 900 social services and resources connected with its member organizations.
Combined Arms, according to information from the organization, works at creating a new model for veteran services, one focusing on collective impact, by creating pathways for them to access resources based on exactly what they’ve asked for, leaving the outdated model of “services in silos” behind. The organization believes that no single outlet can provide all the programming or resources necessary to be successful, so its mission is to focus on easing veterans’ access to those services.
The founders identified what they termed the “four pillars” of connecting veterans to services they need once out of the military: a single point of entry, a technology platform the veterans can initiate from anywhere in the world; efficient technology, using easily accessed online and mobile platforms; engagement, by which the organization reaches nationwide with geotargeted content and campaigns; and engaging the veteran community to empower veterans to serve as community leaders.
In addition to assisting traditional U.S. veterans, Combined Arms also works to provide aid to other U.S. military allies to ensure they are receiving the support they need. One recent addition is the SIV [Special Immigrant Visa] program, through which veterans and volunteers work closely with local resettlement agencies to fill resource gaps, provide newly arrived SIVs and wartime allies with opportunities, and support these people with navigating aid resources in the U.S. For additional information, please visit https://www.combinedarms.us/.
Sponsored by Military Embedded Systems
Uncrewed aerial systems (UASs), uncrewed ground vehicles (UGVs), uncrewed surface vessels (USVs), and uncrewed undersea vehicles (UUVs) continue to be force multipliers for U.S. military operations. These platforms not only deliver lethality in wartime but also feed the U.S. Department of Defense’s (DoD’s) thirst for sensor data, collecting critical intelligence, surveillance, and reconnaissance (ISR) during peacetime. Performance and capability requirements flowing out of the DoD are driving innovation at the payload, sensor, avionics, and communications level.
Powered by Military Embedded Systems, the Uncrewed Systems Virtual Conference drives awareness and thought leadership around embedded electronics technology for autonomous systems from artificial intelligence (AI) to signal processing to avionics safety certification; it also examines strategies for applying a Modular Open Systems Approach (MOSA) in such designs. (This is an archived event.)
Watch the webcast: https://tinyurl.com/4zm75fj5
Watch more webcasts:
https://militaryembedded.com/webcasts/archive/
In large multichannel RF [radio frequency] systems such as phased arrays where channels combine coherently, one objective is to achieve array-level dynamic range improvements from the coherent combination of distributed receivers and transmitters. One of the system engineering challenges with this objective lies in accounting for noise terms that are both correlated and uncorrelated in the system.
This white paper lays out ways in which system engineers can predict phase noise in large multichannel systems and compares it with measurements on a 16-channel S-band demonstrator. Such an analytic approach is based on a small set of measurements that can be used to estimate correlated vs. uncorrelated noise contributions; based on only a few measurements, phase noise under a wide range of conditions can be predicted. Assumptions based on the 16-channel demonstrator are discussed, along with limitations about when the assumptions apply and when to add additional noise terms as system complexity grows.
Read the white paper: https://tinyurl.com/yeshdufm
Read more white papers: https://militaryembedded.com/whitepapers
The September 2023 Military Embedded Systems Resource Guide will focus on embedded hardware and software used in military applications: Our Special Report covers topics in the world of shipboard electronics, while the Mil Tech Trends section will report on the latest in test and measurement. We’ll shine our Industry Spotlight on the perennial issue of managing the military supply chain. Also in the mix: the incredibly relevant topic of open standards for embedded military systems.
The September 2023 Resource Guide – our biggest issue of the year –will also highlight such key electronics-buying categories as avionics, communications, cybersecurity, electronic warfare, embedded hardware/ software, obsolescence/EOL, radar, RTOS and tools, RF and microwave, and safety certification. Don’t miss this special issue.
militaryembedded.com
THE RESOURCE GUIDE PROVIDES INSIGHT ON EMBEDDED TOOLS AND STRATEGIES FOR MILITARY-SPECIFIC TECHNICAL SUBJECTS
Dramatically improve the speed and costs of hardware and software upgrades. Mercury’s fully configurable family of mission computers fit most aircraft and support converged applications for sustainable, high-speed, agile computing at the edge.
FEATURES
MOSA, SOSA and FACE open standard design
Latest Gen Intel/ARM processing
Deployed in safety certified DAL A applications
mrcy.com/missioncomputing