
12 minute read
Select the right battery energy storage
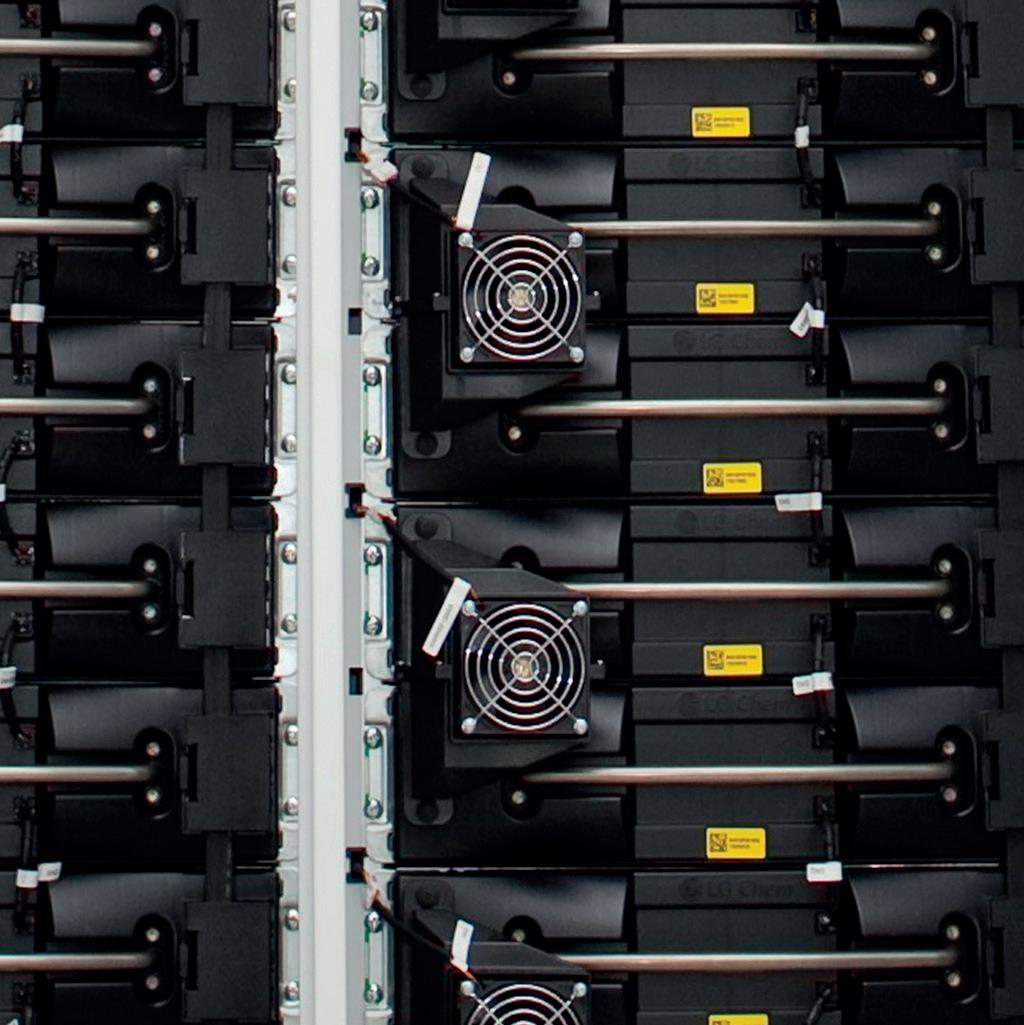
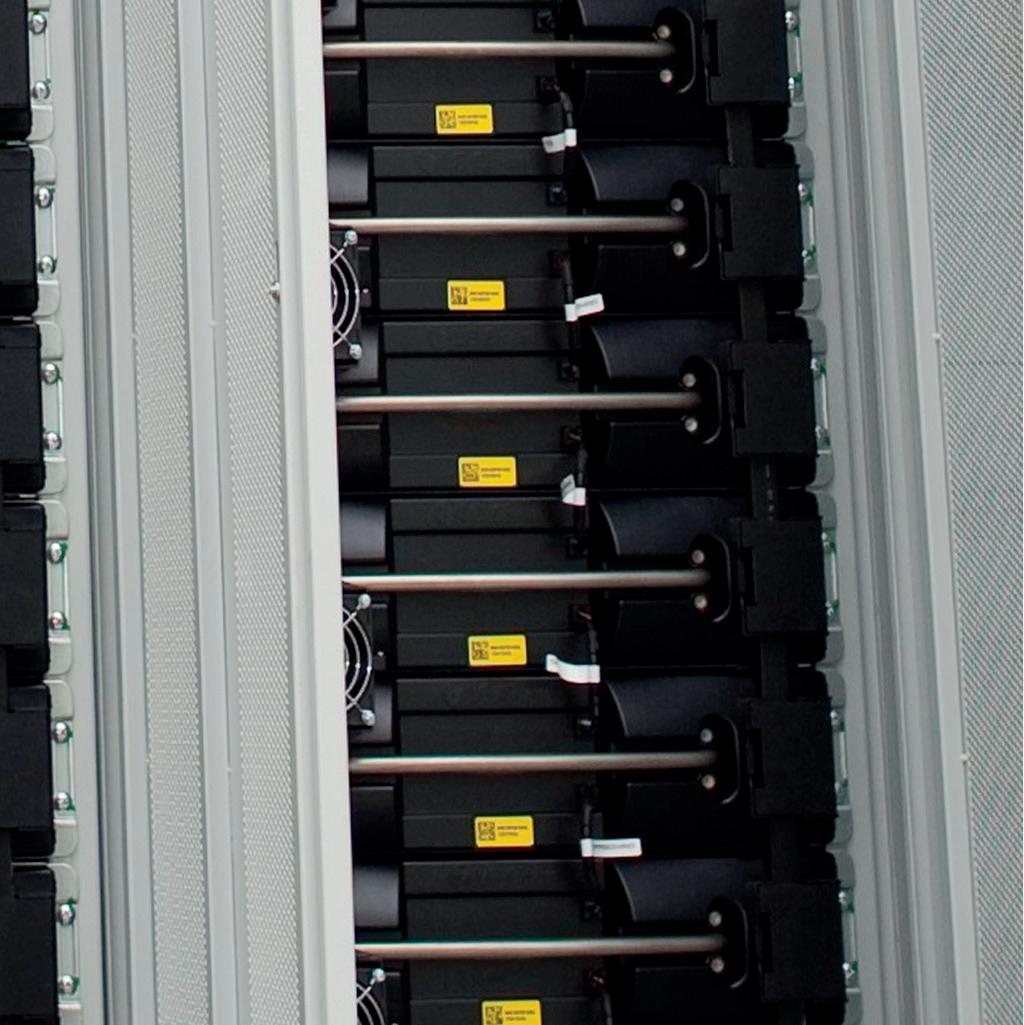
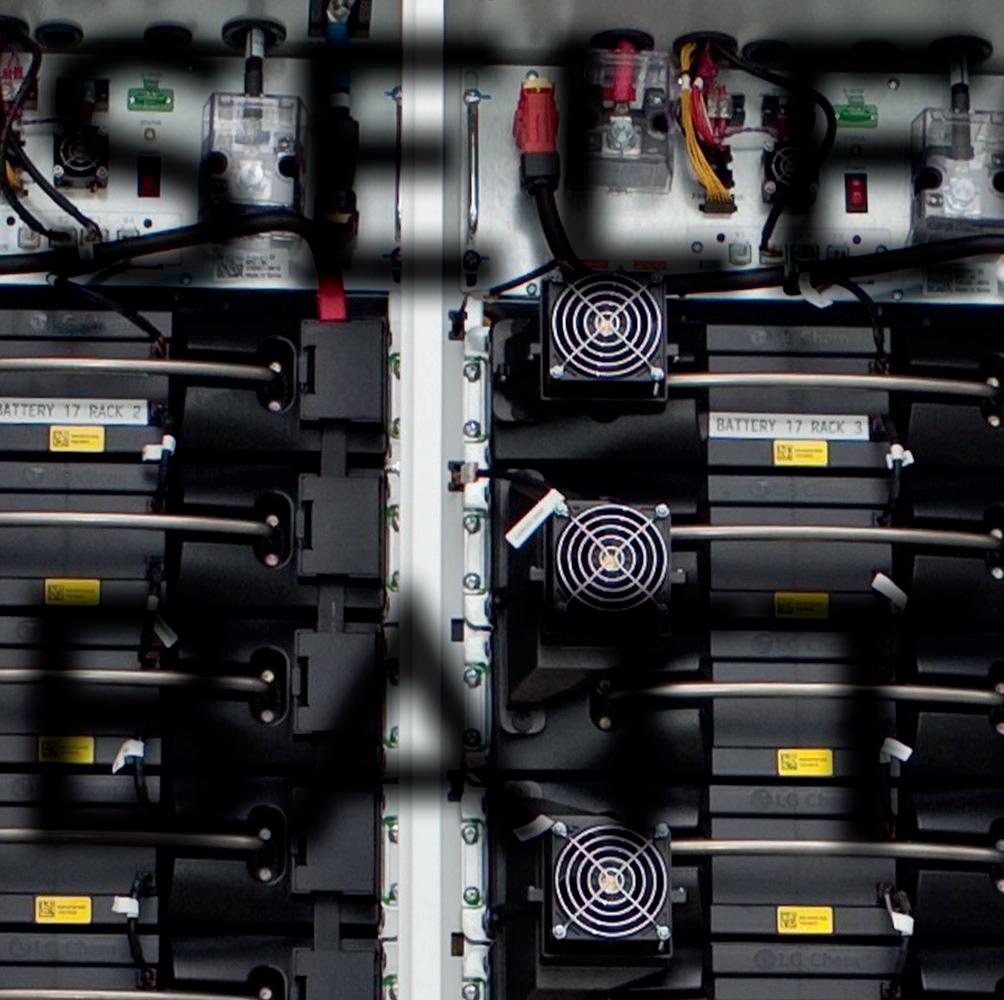
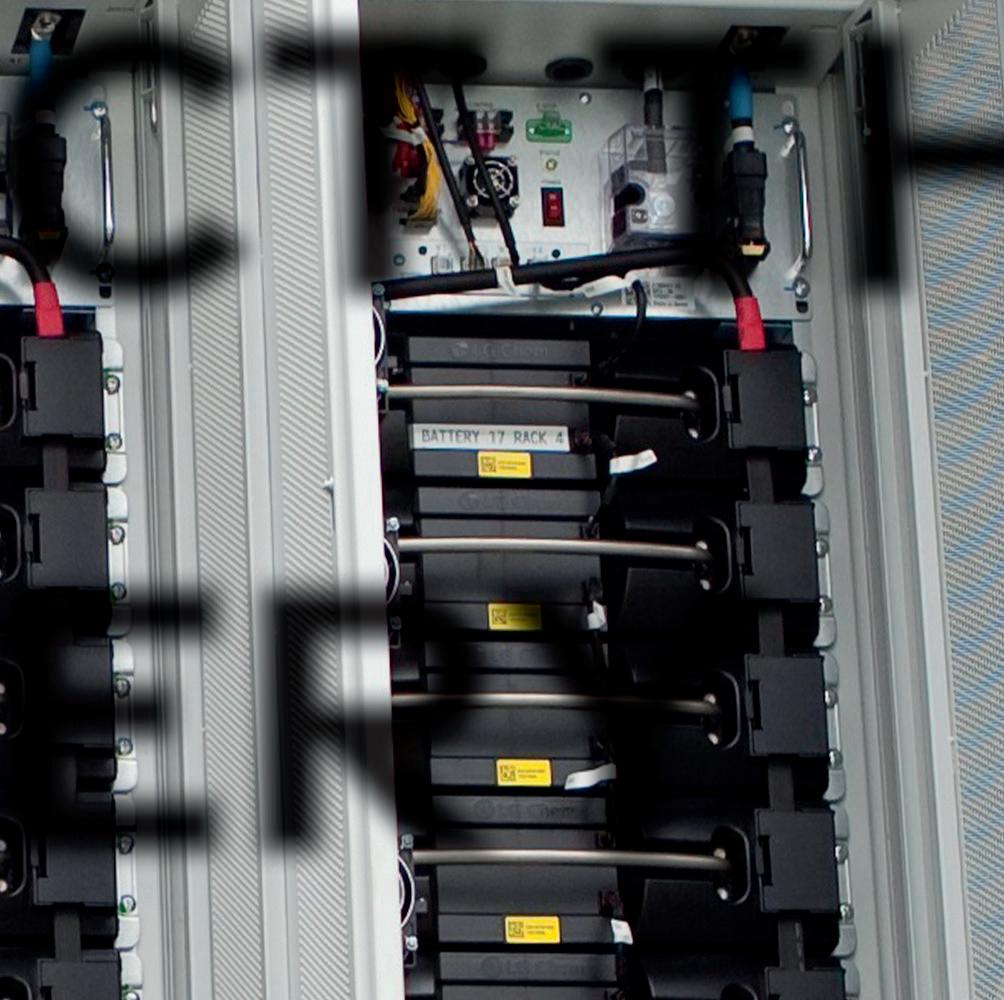
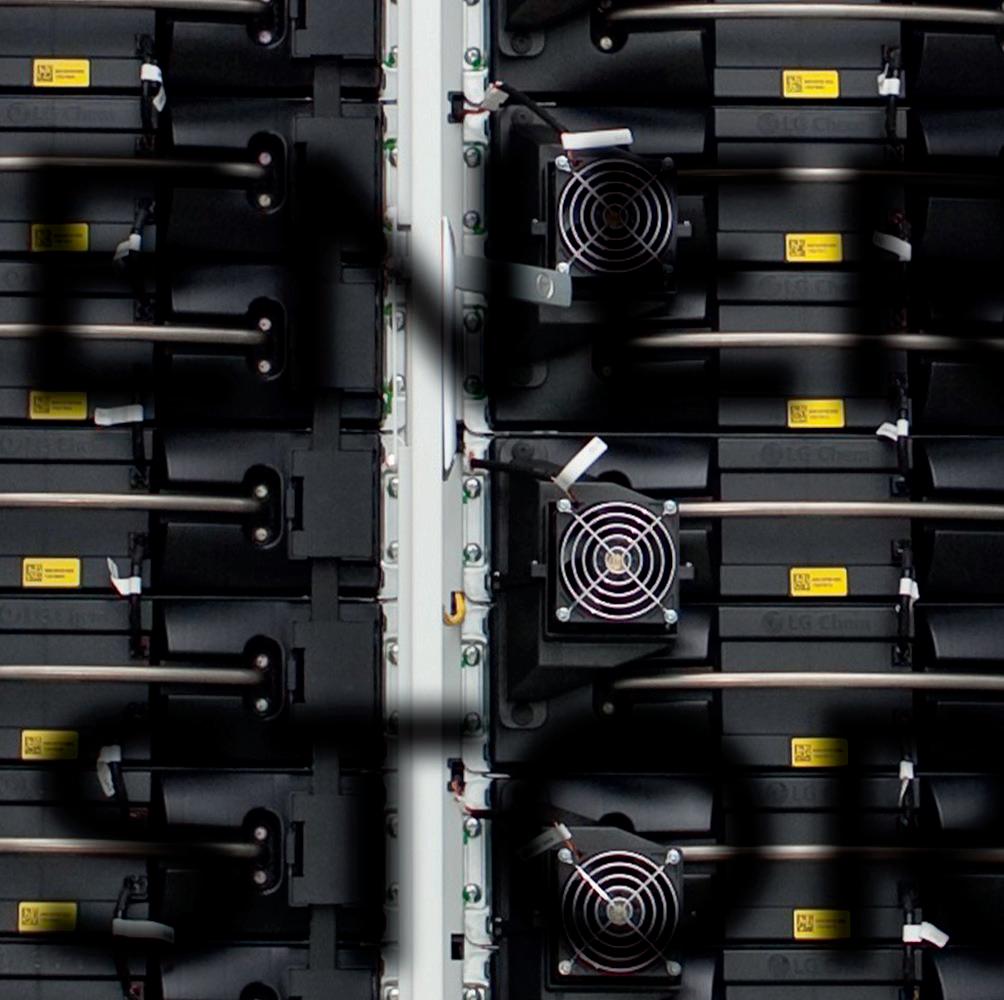
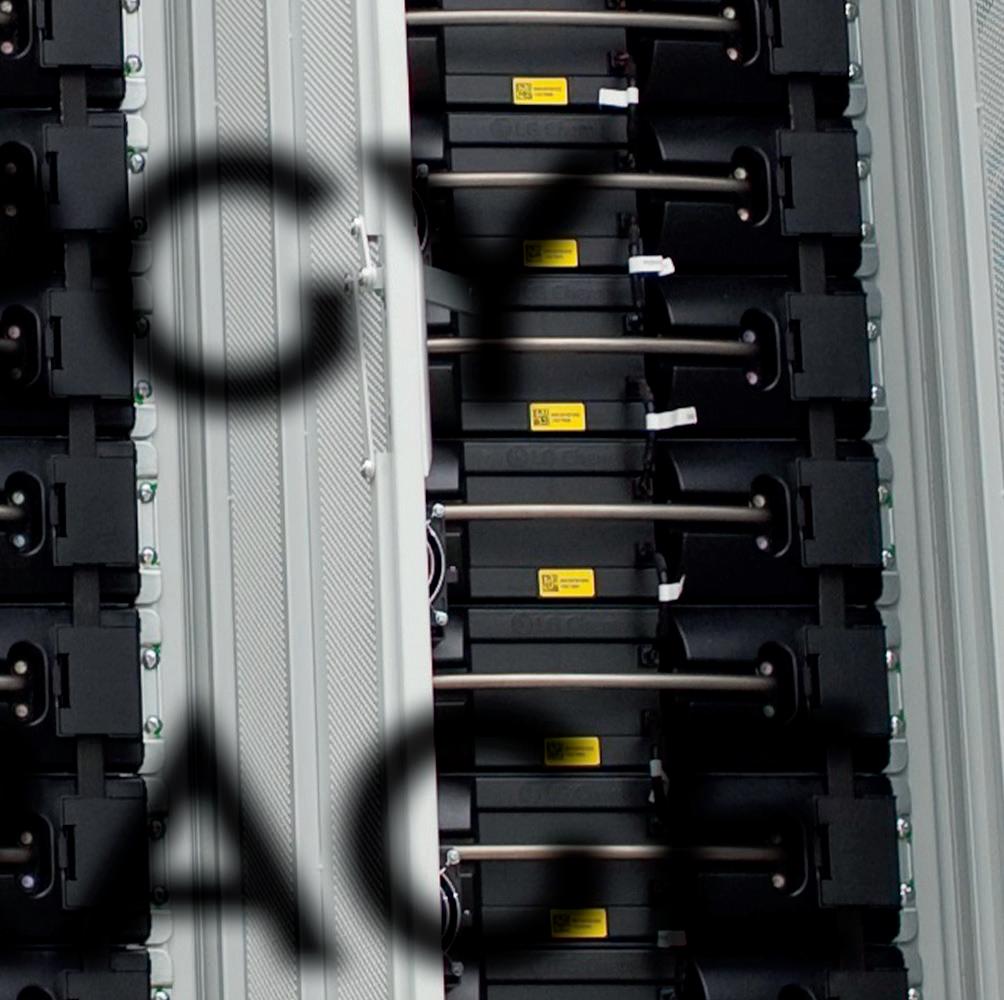

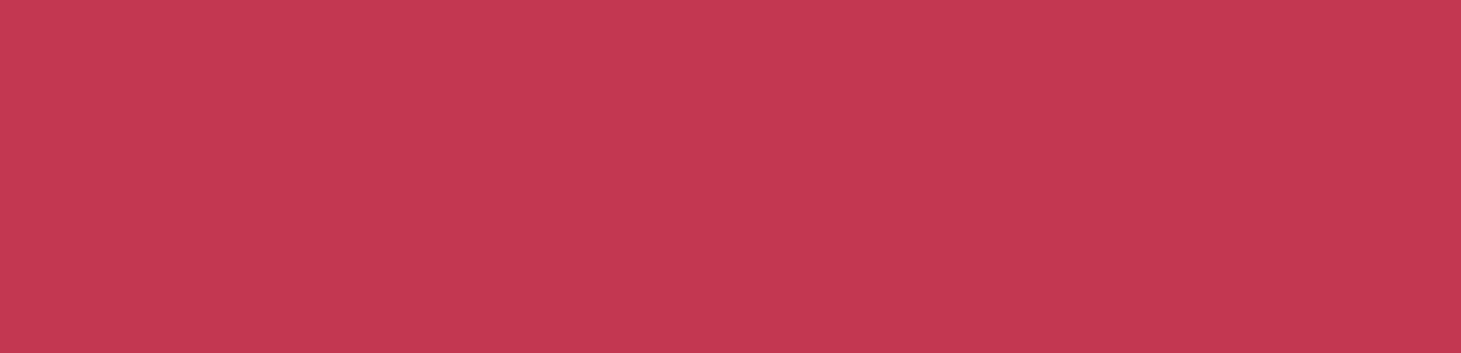
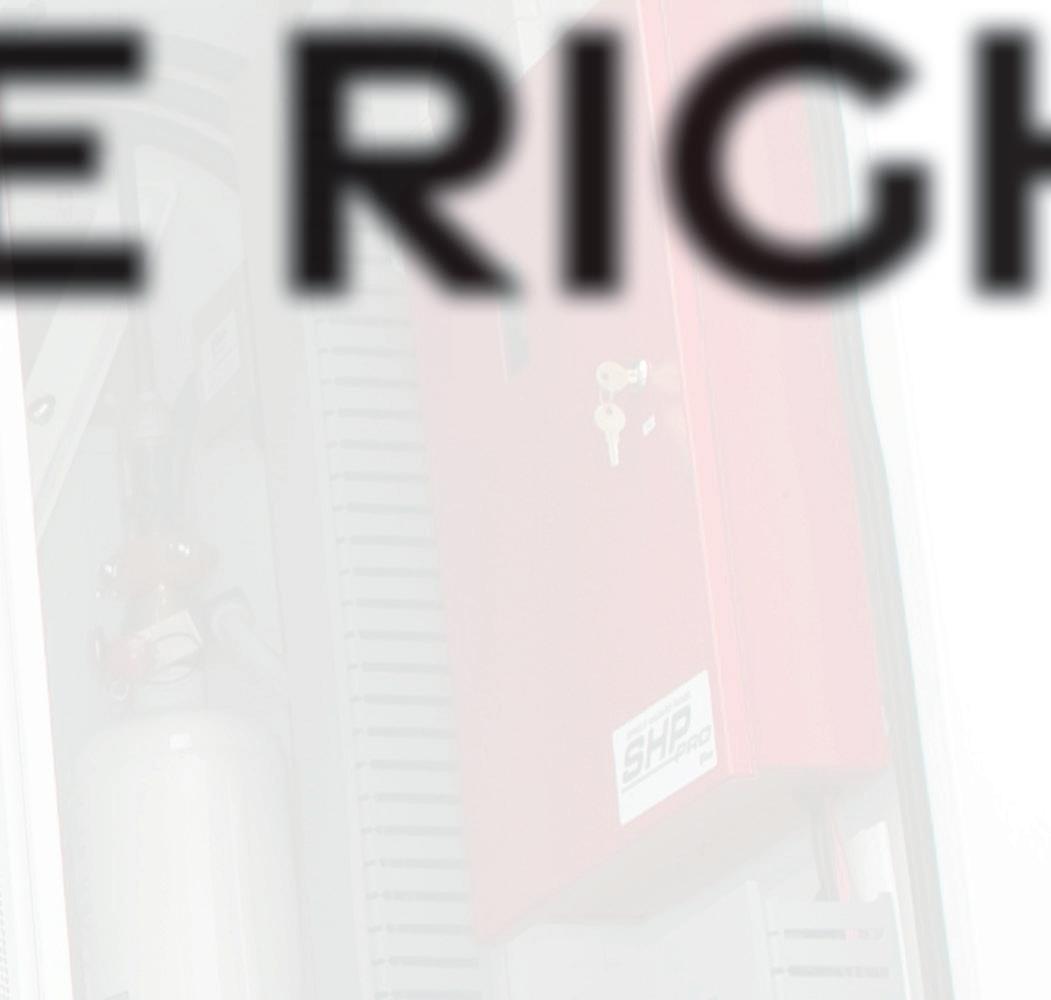
Dr. Pedro Ramirez, Centrica Business Solutions, UK, explains what s, UK, to consider when choosing an energy storage solution and how to to consider when choosing an energy storage solution and how to select the correct battery technology type to meet requirements. select the correct battery technology type to meet requirements.
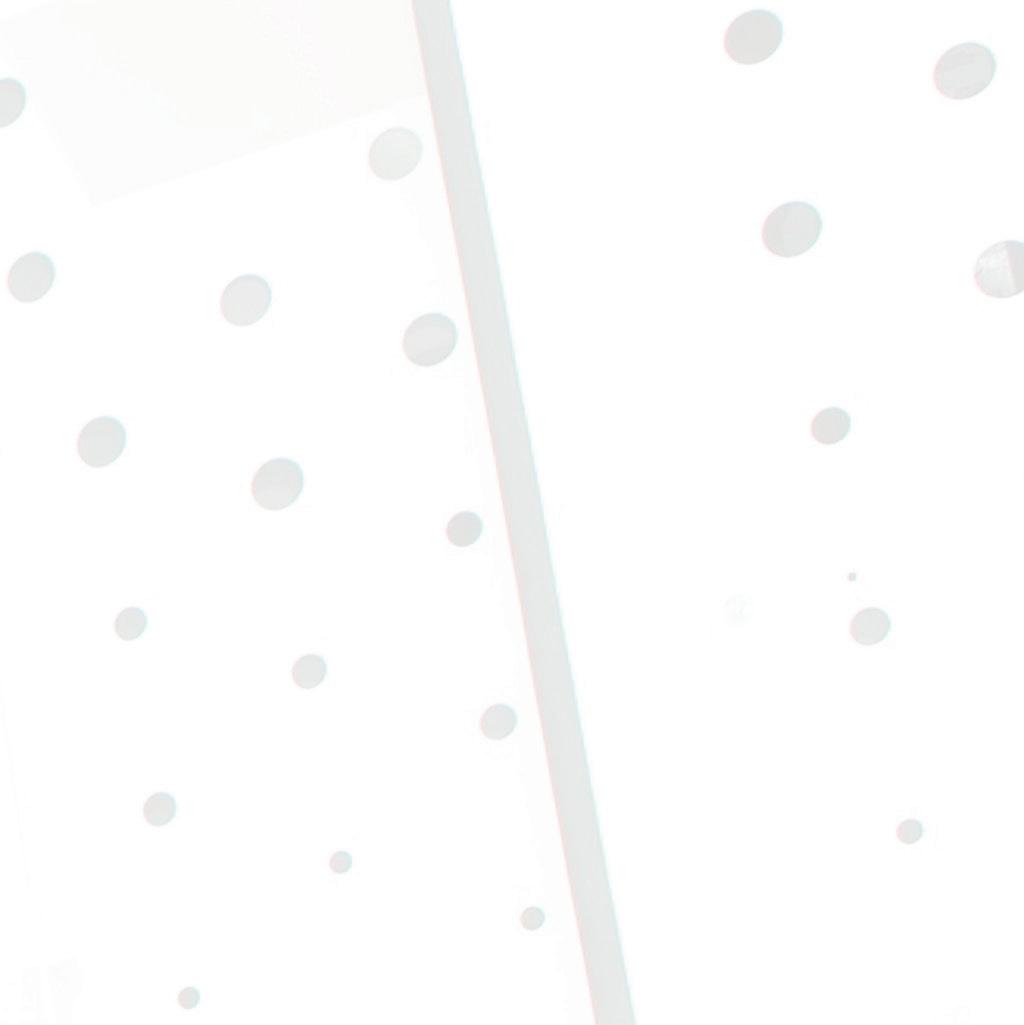
he development of lower-cost, longer-life, and more reliable battery technologies is opening up the opportunities of energy storage to an increasing number of organisations across multiple levels and energy sectors. Battery storage energy storage to an increasing number of organisations across multiple levels and energy sectors. Battery storage is particularly attractive to energy investors and developers looking to monetise batteries by placing them in energy is particularly attractive to energy investors and developers looking to monetise batteries by placing them in energy and grid support markets. and grid support markets.
Battery storage unlocks a range of economic, social, and environmental benefits, which is the reason why the Battery storage unlocks a range of economic, social, and environmental benefits, which is the reason why the technology is rapidly spreading and penetrating across multiple energy sectors. Flexibility and charging/discharging technology is rapidly spreading and penetrating across multiple energy sectors. Flexibility and charging/discharging capabilities uniquely enable this technology to provide and profit from grid support services and energy markets, and at the capabilities uniquely enable this technology to provide and profit from grid support services and energy markets, and at the same time contribute towards improving sustainability and decarbonisation. Three key benefits of battery storage are: same time contribute towards improving sustainability and decarbonisation. Three key benefits of battery storage are: F Provides fast, reliable, and zero-emissions power: Batteries can instantly be switched on/off, and can quickly change the power output as required, with no carbon emissions. This makes batteries a source of unique flexibility for providing grid power output as required, with no carbon emissions. This makes batteries a source of unique flexibility for providing grid support services and contributing to the cost-effective integration of renewables. support services and contributing to the cost-effective integration of renewables. F Maximises return on investment by participating in energy and grid support markets: Batteries’ flexibility can be monetised in a number of markets. Organisations can optimise their battery storage usage and generate new revenue monetised in a number of markets. Organisations can optimise their battery storage usage and generate new revenue streams via commercialising their battery storage in energy and grid support markets. The super-fast response time, streams via commercialising their battery storage in energy and grid support markets. The super-fast response time, high flexibility, and capability of generating and consuming electricity gives battery storage a competitive advantage high flexibility, and capability of generating and consuming electricity gives battery storage a competitive advantage over other solutions – such as flexible load, diesel generators, etc. On the other hand, the capability of storing energy over other solutions – such as flexible load, diesel generators, etc. On the other hand, the capability of storing energy allows batteries to charge at low-price periods and discharge when prices peak. allows batteries to charge at low-price periods and discharge when prices peak. F Improves sustainability: Battery storage increases the utilisation of intermittent generation. This not only contributes towards the decarbonisation of the grid but to the cost-effective integration and efficient utilisation of a larger share of towards the decarbonisation of the grid but to the cost-effective integration and efficient utilisation of a larger share of renewables onto the grid. renewables onto the grid.
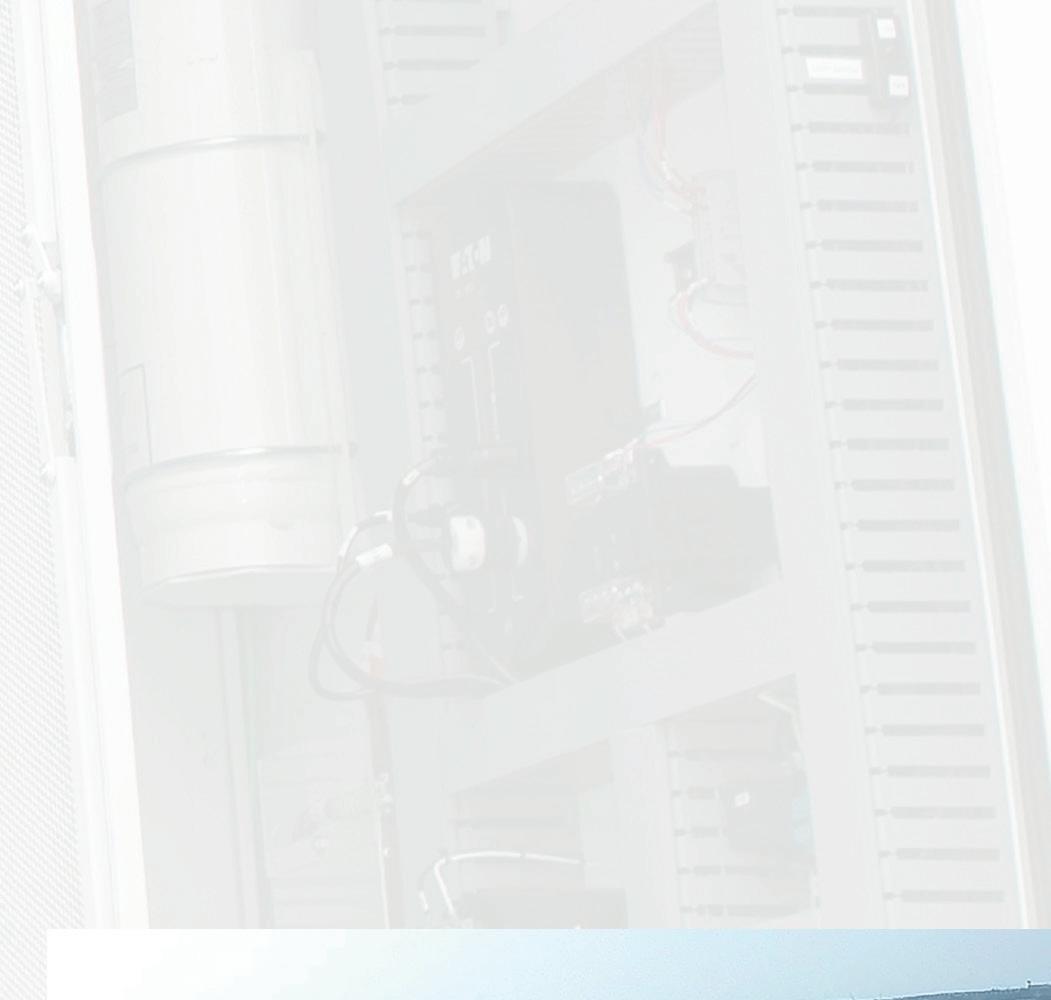
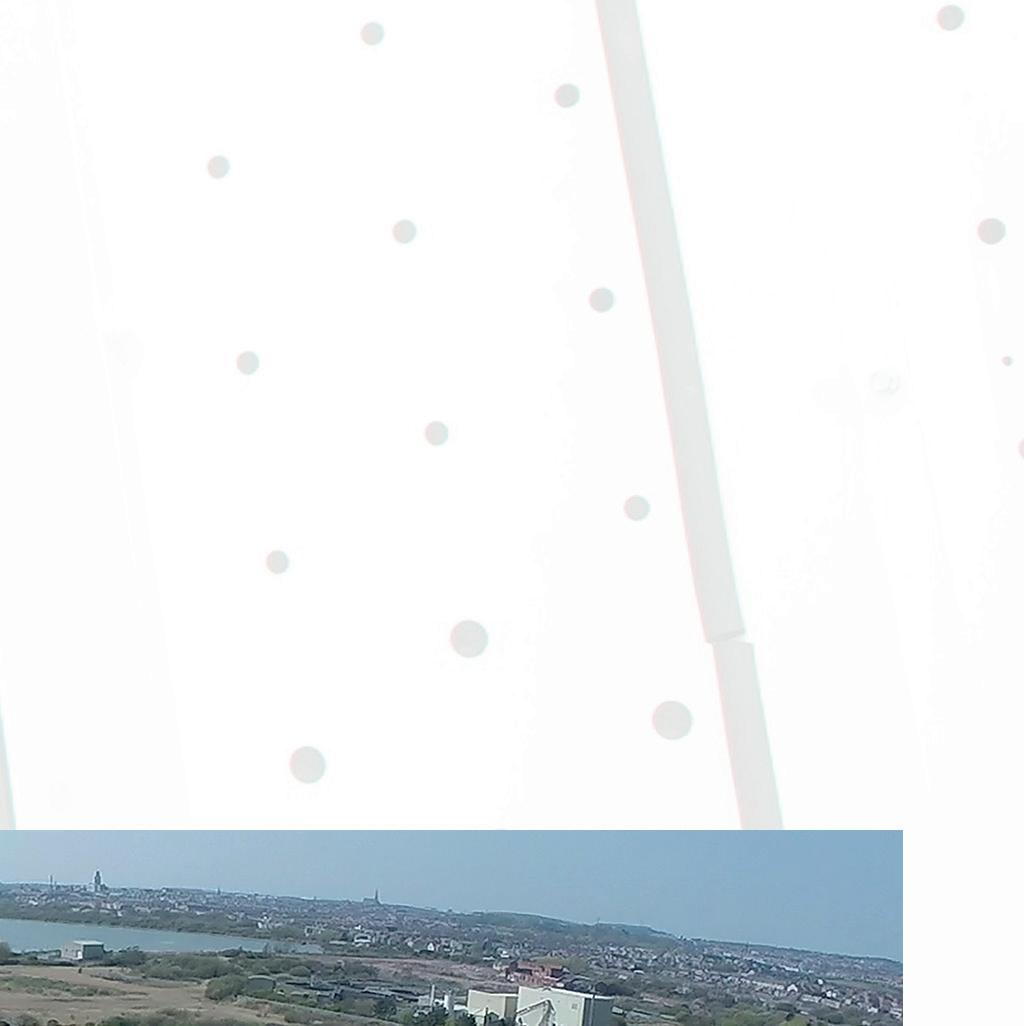
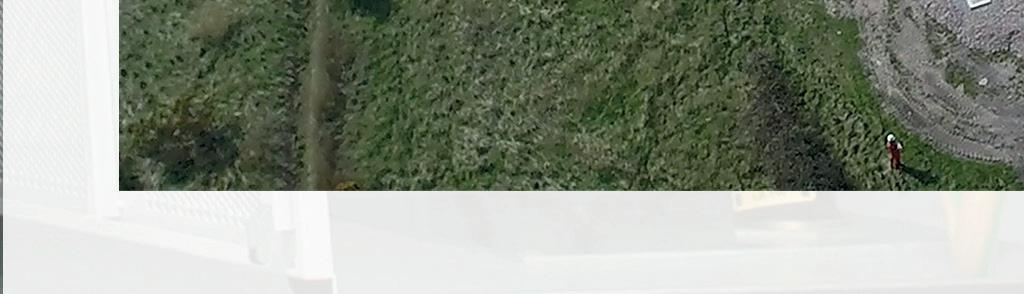
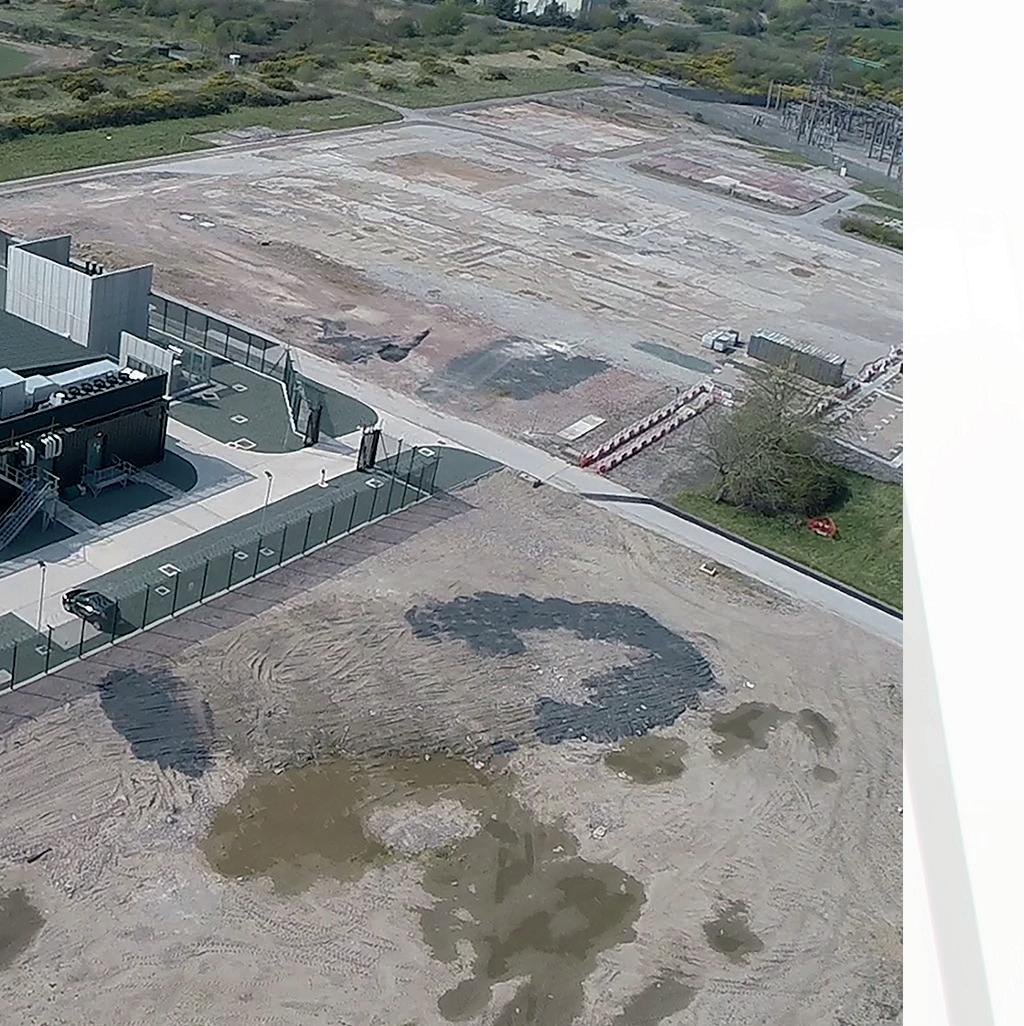
Figure 1. Aerial view of the Roosecote, UK, 49 MW/24.5 MWh lithium-ion BESS.
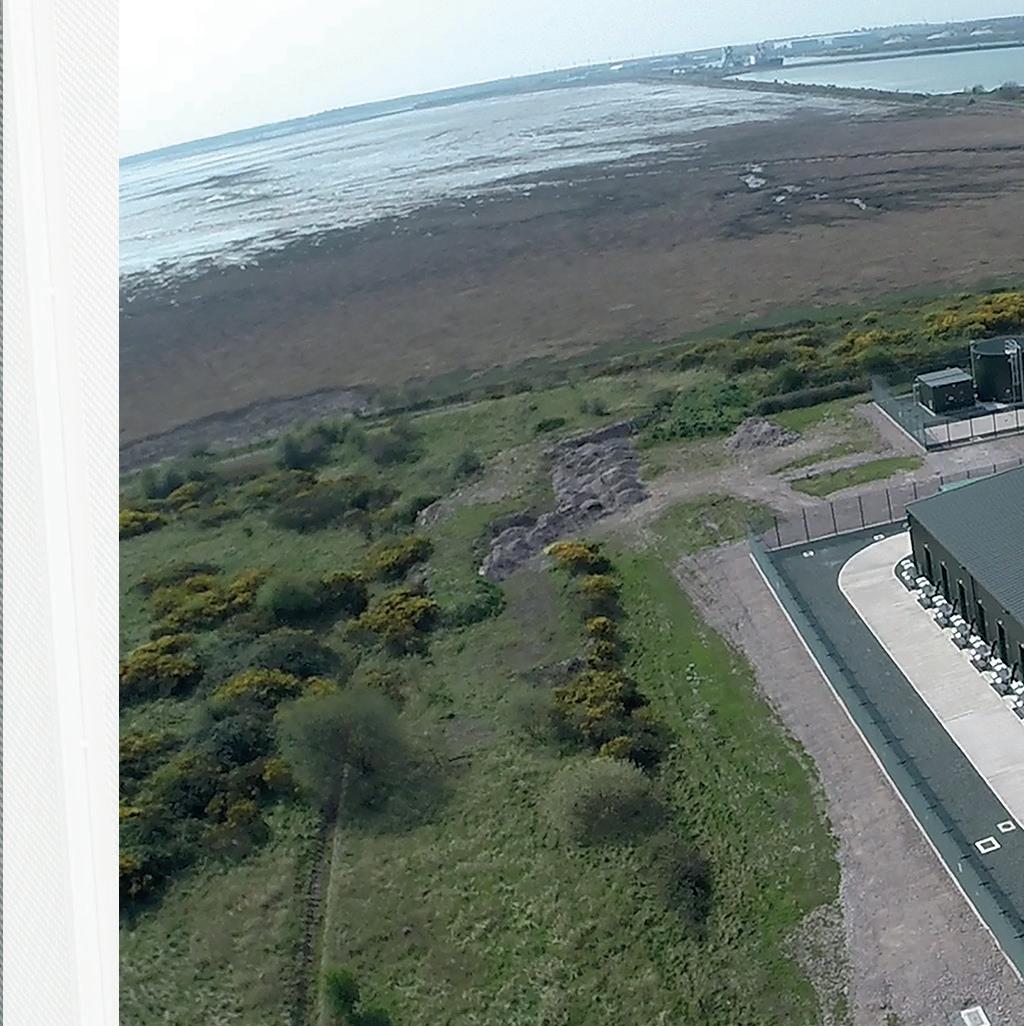
The basic components of a battery energy storage system
A battery energy storage system (BESS) is an electrochemical system capable of storing and discharging electricity by means of reversibly converting chemical energy into electrical energy. A BESS is composed of numerous functional building blocks, all of which are vital for the operation of the system. The basic four building blocks of any BESS are: F Energy storage system (ESS) – the physical energy storage device in which electric energy is stored in a chemical form. The physical energy storage device for lithiumion batteries (LIBs) is formed by hundreds of connected battery cells. In the case of redox flow batteries (RFBs), it is formed by the electrolyte and electrolyte tanks that store energy.
F Battery management system (BMS) – the low-level control and monitoring of the operational state of the physical energy storage device. The BMS ensures that the system at battery module/pack level performs within its operational limits and checks that the power that needs to be charged or discharged from the battery cells/ modules is within the operating range of the current system status. It also continuously monitors battery cells/ modules parameters (voltage, current, temperature) in order to estimate their state-of-health and state-ofcharge for cells balancing purposes.
F Power conditioning system (PCS) – the equipment that enables the battery system to interact (i.e. charge or discharge electric energy) with the grid.
F Energy management system (EMS) – the high-level control that integrates all parts of the BESS and that determines when and at what rate the storage system needs to be charged or discharged – or when it needs to be idle.
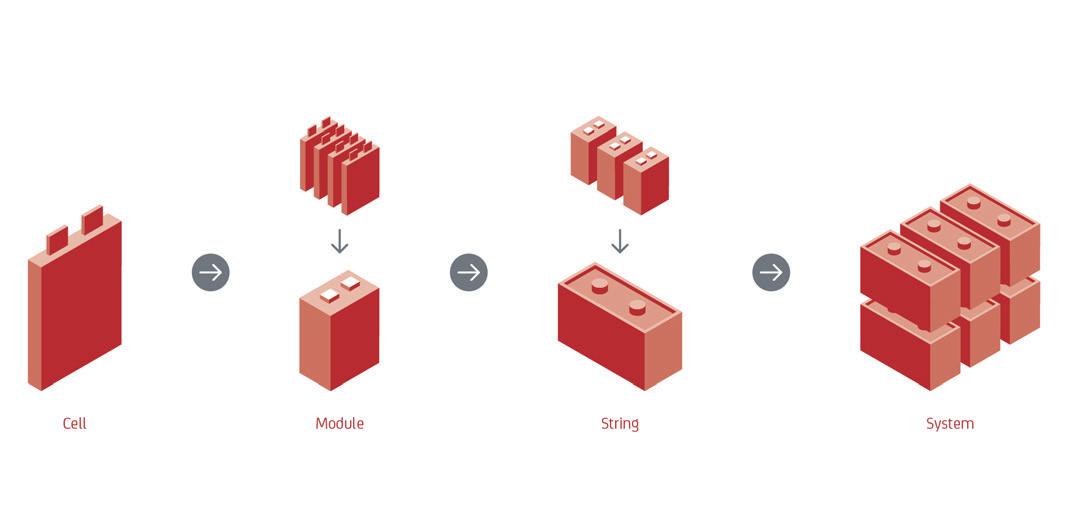
Figure 2. Lithium-ion battery (LIB) cells integration process and key components of a battery system.
Figure 3. Traditional configuration of a liquid electrolytes redox flow BESS.
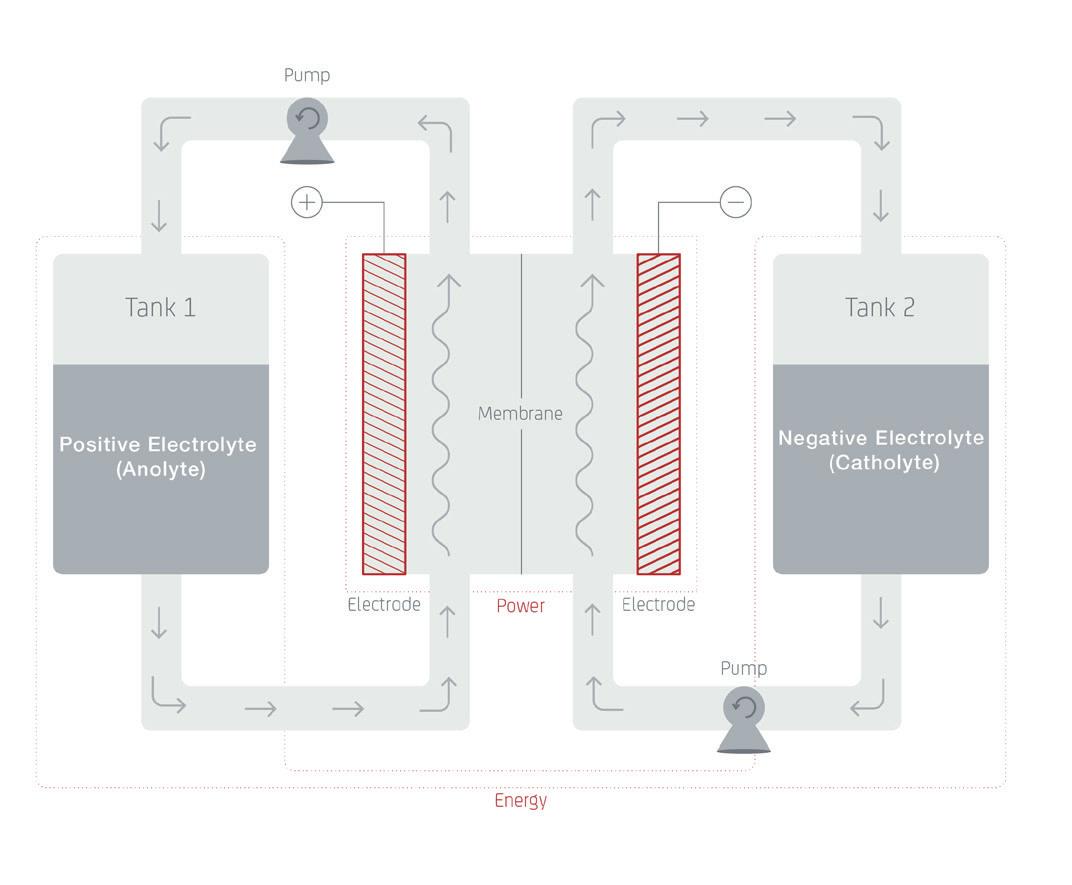
Electrochemical batteries
Electrochemical batteries are classified into two main groups: F Rechargeable cell-based batteries – composed of one or more electrochemical cells, which are produced in different shapes and sizes depending on their intended application. Common chemistries of rechargeable batteries include lead-acid and lithium-ion. Currently, the most popular chemistries for stationary applications are lithium iron phosphate (LFP) and lithium nickel manganese cobalt (NMC).
F Flow or RFBs – rechargeable batteries that use liquid electrolytes (usually two, one positively and one negatively charged) for storing energy. The electrolytes are stored in separate tanks and are pumped into the battery’s cell-stack when charging or discharging.
Which battery technology types work best?
There are currently two key battery technology options that are commercially proven and can operate at scale to best suit key project requirements and site-specific conditions and limitations.
Lithium-ion batteries
LIBs are a mature and proven technology and by far the most widely used type of battery nowadays. In this battery type, lithium ions move from the negative electrode through an electrolyte to the positive electrode of each battery cell during discharge and back when charging. Key properties of this battery technology are its high energy density, tiny memory effect, and low self-discharge.
The basic and smallest building blocks of a LIB system are the battery cells, which store the chemical energy. Cells are aggregated together, i.e. connected in series and in parallel to form a module, pack, or tray, which is normally the smallest interchangeable part of a BESS. Several modules are then aggregated to form a rack or string. Several racks are then connected to get the levels of power and energy capacity required for the BESS.
Lithium-ion is the technology that enabled the portable electronics revolution. Today it is the dominant battery technology for powering portable electronics and is increasingly being used to integrate renewables into power systems worldwide. It is also core to the rapidly growing
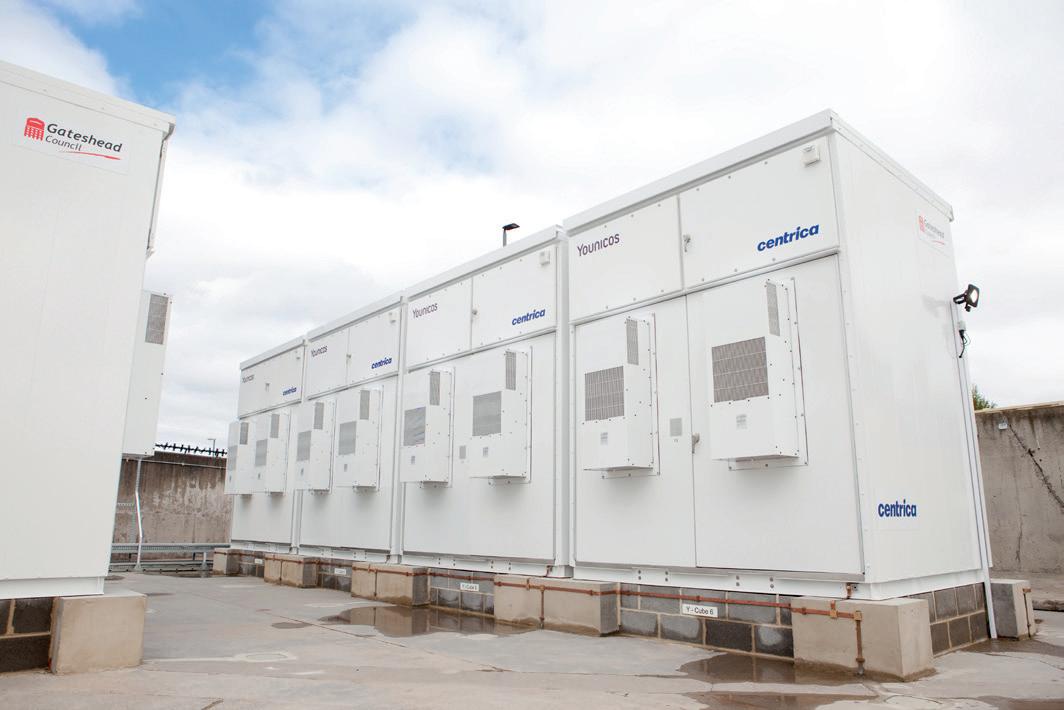
Figure 4. External view of the BESS containers of the Gateshead Council 3 MW/3.33 MWh lithium-ion BESS in the UK.
Figure 5. LIB modules and racks inside one of the BESS containers of the Gateshead Council 3 MW/3.33 MWh lithium-ion BESS.
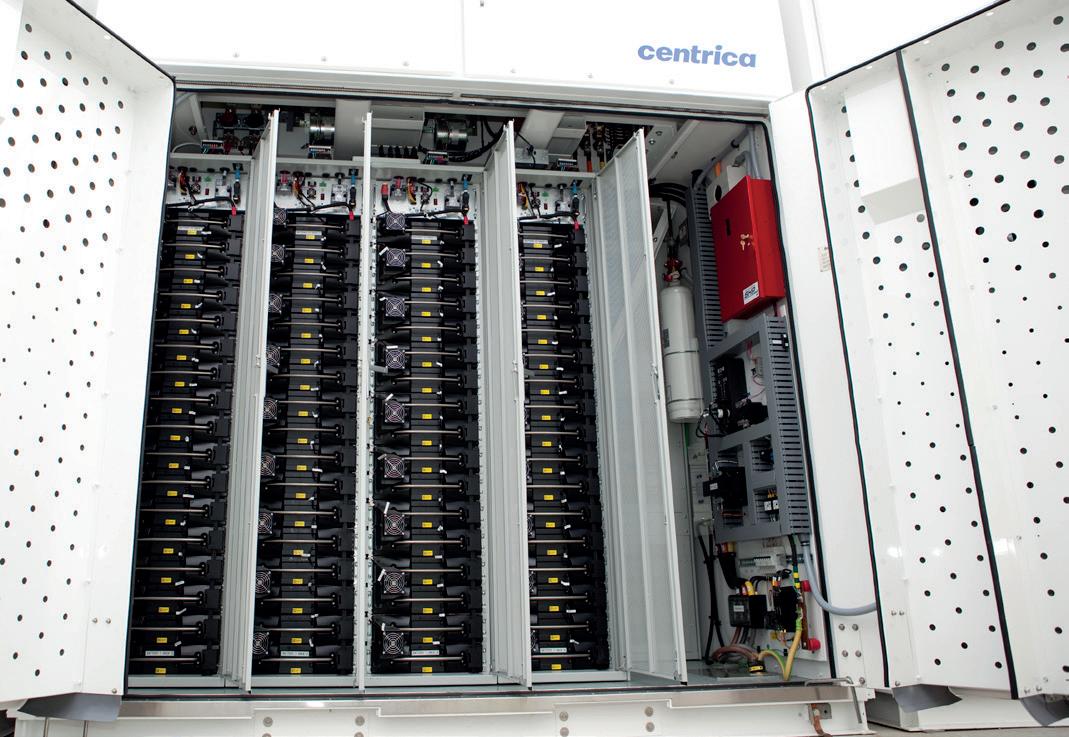
electric vehicles industry. Due to increasing demand from the automotive sector, high manufacturing volumes and continued innovation have translated into significant battery cost decline since 2010, while energy density and battery cycling lifespan continue to improve.
One of the drawbacks of lithium-ion technology is that power and energy are tied together in a cell. Therefore, it is not possible to increase one without increasing the other at the same time.
Flow batteries
RFBs are a mature energy storage technology developed by NASA in the 1970s and are quickly gaining presence in the market for long-duration applications. This rechargeable battery type separates out its positively and negatively charged liquid electrolyte energy carriers using an ionselective membrane at the cell level (where the electrical energy and chemical energy conversion happens). Charging and discharging conditions allow selected ions to pass through the cell membrane and complete chemical reactions. The electrolytes are stored in separate tanks and they are pumped into the battery cell when charging and discharging.
The storage capacity of flow batteries can be increased by simply utilising larger storage tanks for the electrolytes. Several chemistries are possible for this type of battery, but vanadium/vanadium is the most popular nowadays.
RFB technology offers a similar response time to LIB. It is quickly gaining market presence due to its suitability for long-duration applications, i.e. where energy charging/ discharging is required for more than a couple of hours, involving larger depth-of-discharge cycles. Currently, systems with more than three hours duration are a cost-competitive alternative to LIB systems and offer a much higher level of endurance. As the market share for RFB increases and accelerates, economies of scale are expected to make this technology cost-competitive for applications below three hours duration in the coming years.
Compared to LIB, RFB has three essential building blocks at the battery system device level, i.e. the electrolyte and electrolyte tanks, pumps and pipes, and the cell-stack or stack. This is due to the separation of power and energy in this type of battery technology.
The electrolyte tanks are the containers in which the positively and negatively charged electrolytes are stored. The volume of electrolytes stored in the tanks defines the energy capacity of the battery. The pumps and pipes are used for pumping the electrolytes into the stack, where the electrochemical reaction takes place. The stack is a collection of cells electrically connected, through which the electrolytes are pumped. Each cell consists of two half-cells (anode and cathode), which are divided by a separator or membrane that chemically and electrically isolates the two sides of the cell. The separator/membrane allows ionic transfer to support the electrochemical reaction on both sides of the cell.
The power of an RFB system is defined by the surface area of the electrodes located in the anode and cathode of each cell, which allows the power of the system to be increased without changing its energy capacity.
A key characteristic and advantage of RFB technology over LIB is that power and energy are not tied together and can be increased independently. Additionally, the cycling-degradation performance of RFB is much superior to that of popular LIB chemistries, and the electrolytes can be fully re-used at the end of the operational life of the battery, which is typically 20 years for this battery technology. However, RFB has a lower energy density, which translates into systems that are heavier and require a larger footprint.
How do you navigate the complexity around battery types?
Choosing the best battery storage solution is complex, but there are three key characteristics that define a battery’s operating capabilities and influence specification. These are: F Battery chemistry – refers to the technology used for storing energy in the battery. This determines the power and energy density of the battery, and also the operational characteristics and limitations, as well as cycling lifespan.
F Power – typically measured in kW or MW, refers to the maximum level of electricity that can be discharged at any one moment.
F Energy capacity – typically measured in kWh or MWh – refers to the volume of energy the battery can store, thereby determining the duration of discharged power.
Battery chemistry, power, and energy capacity determine the main flexibility features of the battery for charging and discharging energy and should be carefully assessed when deciding the most appropriate BESS for an organisation’s needs. The selection process is also dependent on many other factors, such as: > Cycling operating regime(s), degradation, and lifespan performance. > System roundtrip efficiency. > System response time and ramp rates. > Active and reactive power requirements. > Available space and battery footprint. > Warranties and guarantees. > Site connection capacity and restrictions. > Operation and maintenance. > Type and capacity of on-site energy infrastructure.
A careful and adequate sizing and specification of the BESS is key for ensuring the battery can be monetised in all relevant energy and grid support markets, and that risks are minimised and return on investment maximised.
Battery storage in action
Centrica Business Solutions has extensive experience of designing, building, and operating LIB and RFB projects, including: F Centrica Business Solutions is using lithium-ion technology in one of the UK’s largest commercial storage facilities of 49 MW/24.5 MWh at the former Roosecote power station in Barrow, UK.
F A behind-the-meter 3 MW/3.33 MWh LIB system Centrica
Business Solutions supplied to Gateshead Council in the
UK, which is capable of powering up to 3000 homes for an hour. This system has been commercialised in the dynamic Firm Frequency Response (dFFR) and capacity markets.
F Centrica Business Solutions is operating a series of
LIB assets in the UK and across Europe as part of virtual power plants, using its FlexPondTM optimisation technology. Various consumption and generation assets are aggregated and multiple grid support markets can be accessed, such as fast frequency response services.
F Centrica Business Solutions partnered with redT (today
Invinity Energy Systems) to combine a vanadium/ vanadium RFB with solar photovoltaic at a project in
Cornwall, UK. This project, which is part of the Cornwall
Local Energy Market Project, is increasing solar utilisation and reducing peak-time electricity costs.
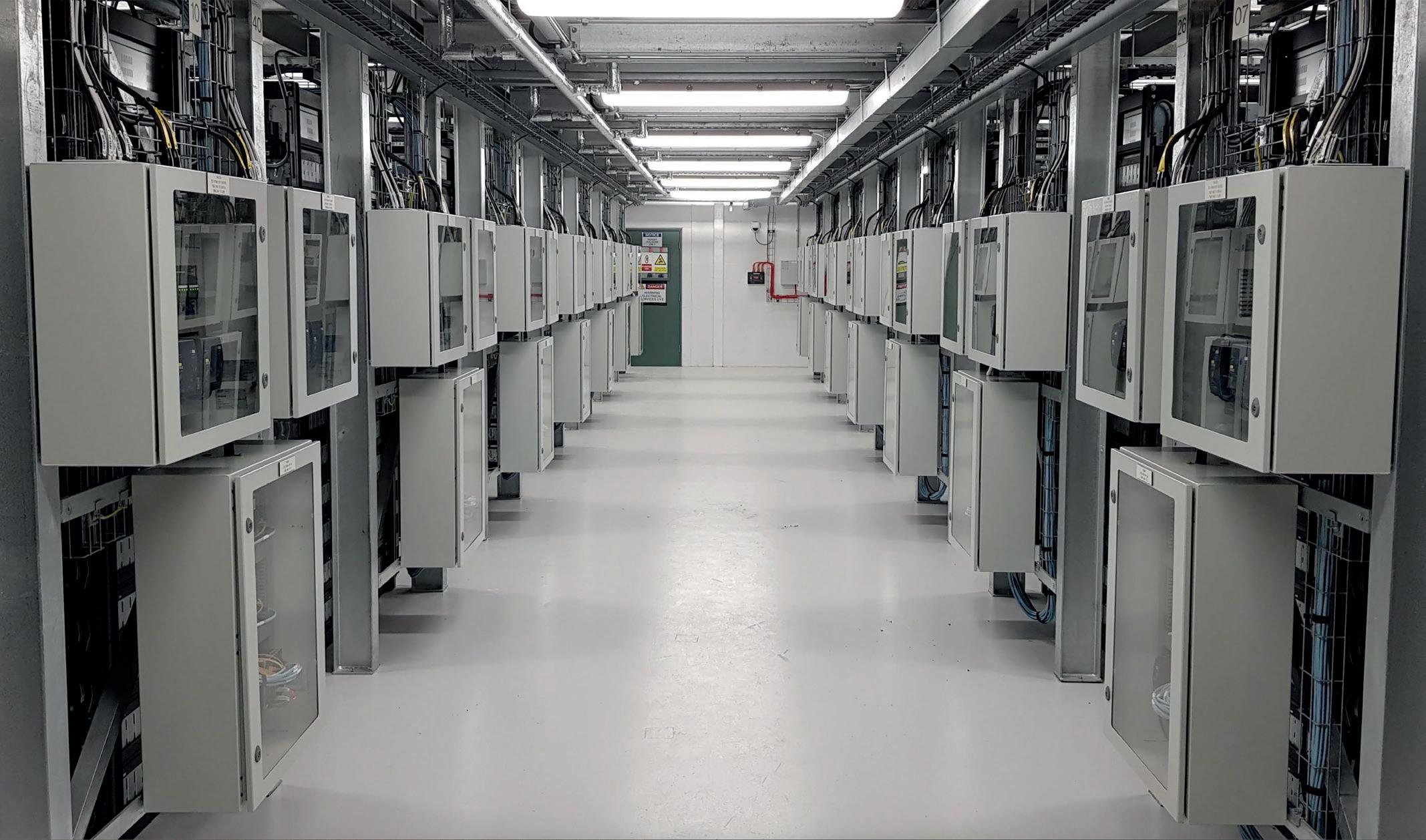
Figure 6. Internal view of the Roosecote 49 MW/24.5 MWh lithium-ion BESS, showing the PLCs that control the charging and discharging of the 63 battery arrays.