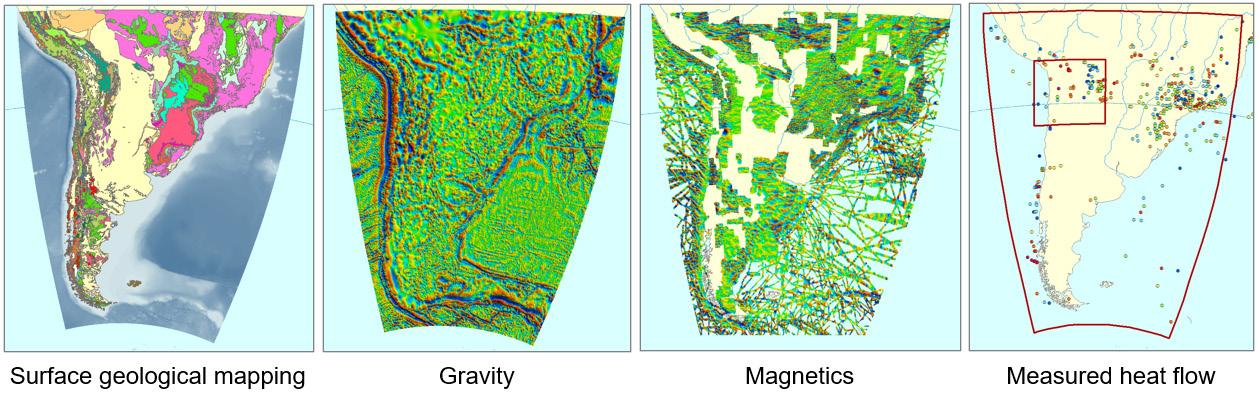
11 minute read
Iceland fires up
heating since 1969, today supplying geothermal heat to 250 000 households via 50 heating networks.8
Risk sharing
While it can provide a consistent renewable energy source, there are certain risks associated with geothermal energy relative to other complementary low-carbon energy solutions, such as wind or solar. Accessing a new geothermal resource involves not only upfront drilling expense but also geological and operational risks, which could make investment more challenging to attract.
Yet the large, long-lived, low land-footprint, baseload and flexible energy supply that can be delivered in a success case is very attractive, both from a cost and environmental perspective.
While solar and wind energy models are well understood, geothermal projects are highly specific to their local context. This requires a special kind of expertise. Detailed upfront planning, geological evaluations, and overall technical and commercial strategies to reduce risk are critical to the success of these projects.
Heat is not easily transported, unless the temperature is high enough to turn it into electricity. Therefore, even a large geothermal resource is not necessarily viable – it also may need to be in reasonable proximity to where the heat is needed. This makes geothermal prospecting very different to mineral or hydrocarbon exploration where a resource, once discovered and developed, can be potentially shipped anywhere in the world.
Most deep geothermal exploration aims to identify areas with higher temperatures (high geothermal gradients) where drilling is shallower and thus less expensive. Geothermal developments have sensitive profit margins and, if used for heating rather than electricity generation, they need a local market so early integration with the right information is critical to make projects viable.
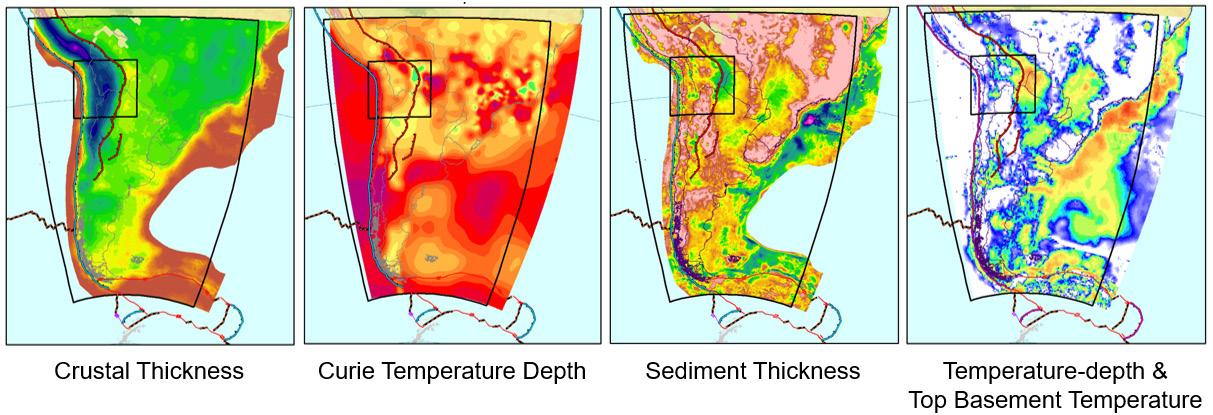
Figure 1. Example of subsurface input parameters to help identify geothermal sweet spots in South America.
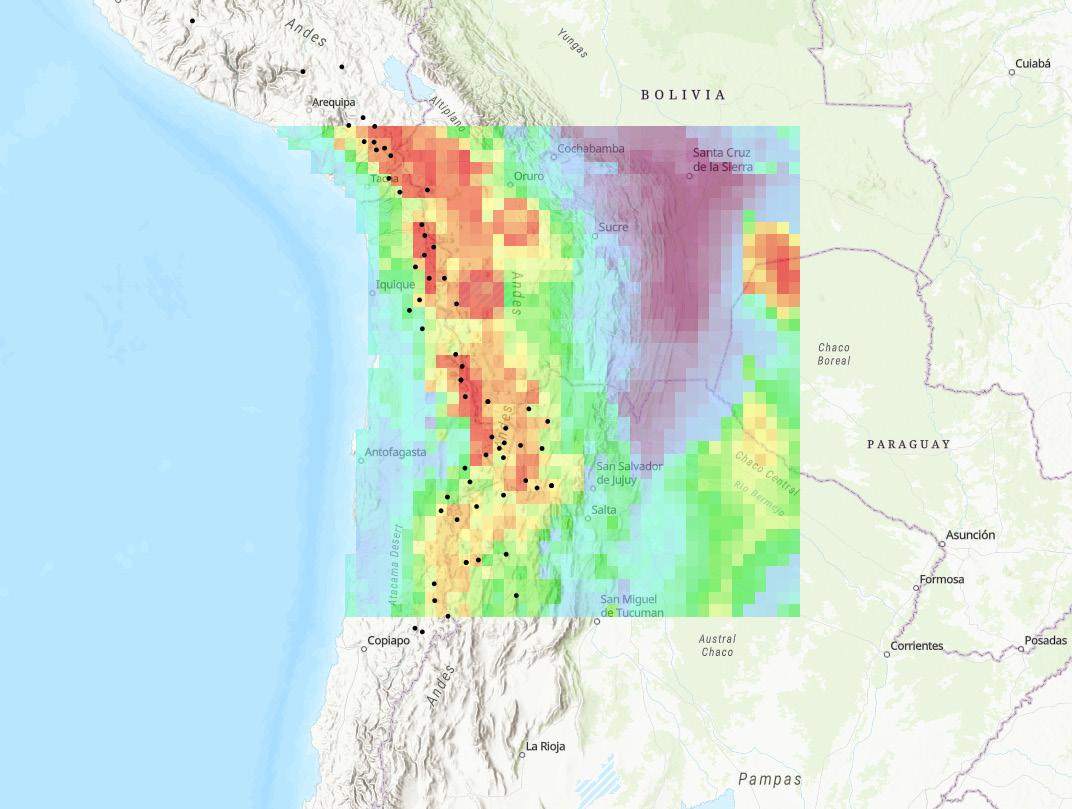
Figure 2. Machine learning derived geothermal heat predictions compared to existing and planned geothermal developments.
Identifying the best areas for geothermal
Getech brings together its geoscience data, geospatial software products, and skills in geothermal energy to enable rapid identification of sites highly prospective for this type of energy – lowering risk, increasing profit-margins, and ultimately reducing payback times and returns in geothermal projects.
The company’s approach is to apply these unique data, products, and skills to upscale the geothermal opportunity through its Heat Seeker solution, mapping geothermal favourability by combining geoscience and market factors with geospatial machine learning analytics.
Heat Seeker incorporates proprietary data as well as public domain sources, using data sets to frame the modelling of temperature, crustal structure, heat flow, and geothermal system boundary conditions (Figure 1). Some of these are derived from gravity or magnetic data using established methodologies, such as the multi-geophysical inversion method for estimation of radiogenic heat production in the crust presented by Hokstad et al., (2017).9
In a recent project, Heat Seeker was used to pinpoint favourable locations for geothermal energy developments in South America.
In order to create a surface heat flow prediction map, a variety of machine learning algorithms were tested. This was based on a number of input data sets, such as deep earth structure and temperature constraints, volcanism, and geothermal sites.
The model was trained on data sets from the US, a country that has tectonic similarities with the area of interest and already has comprehensive heat flow data useful for crustal scale modelling. Once the accuracy of the algorithm was proven, it was then applied to an area of interest in South America bordering Chile, Bolivia, Paraguay, and Argentina.
The resulting machine learning output highly correlates with known volcanic geothermal projects in the area (black dots on the map in Figure 2), modified from Pesce et al., (2014)10 and Bona and Coviello (2016).11
There are some interesting results worthy of further investigation, including high heat flows in the shared
convective basin system of North Western Paraguay and South Eastern Bolivia, which might hold further opportunities.
The success of a geothermal project depends on a lot more than geological heat flow. There are many infrastructure, economical, and social factors that influence geothermal favourability and, ultimately, viability.
To map potential sweet spots, Heat Seeker employs a method that combines multiple favourability elements as stacked layers which are then merged to highlight areas of greatest favourability for resource accumulations.
The example in Figure 3 looks at the basic drivers of supply and demand. Top basement temperature (a) and the proximity of Holocene volcanoes (c) are indicators of a potential geothermal resource, whilst population density (b) is a simple proxy of heat and energy demand. By numerically combining these factors into a single favourability map (d), there is a correlation with existing geothermal projects (e) – indicating that this approach has merit.
Next, the results are refined using further favourability criteria and more detailed data collected in Argentina (Figure 4). In this map, the output from the previous analysis is used as one of the inputs (d), combining it with: F Locations of high carbon emitting power plants (f).
F Non-industrial large heat users as proxies for single project customers (g).
The resulting favourability map (h) shows potential sweet spots where geothermal demand and supply are present, and where geothermal energy could help decarbonise the existing energy used.
This data can be refined further using additional criteria, such as potential competition from other renewables – i.e., wind (i) or solar (j).
This example of a full Heat Seeker analysis involved additional factors such as the location of historical mines, deep well data, proximity to power grids, fault slip tendency, location of existing geothermal projects, and the machine learning output described previously. The workflows are tailored to the commercial and technical questions in each area and are limited only by the imagination of the analyst and the data sets available.
Conclusion
Geothermal energy is a low-carbon resource that is globally abundant, but still vastly underexploited. Geospatial analysis and machine learning, coupled with comprehensive data sets covering potential supply and demand, can provide unique insights and understanding for policy makers, investors, operators, and stakeholders.
Developing the geothermal sector could increase domestic energy security, provide considerable economic stimulus, and contribute to job generation. This includes redeployment of both technologies and workers from the oil and gas industry who have transferable skills and experiences in risk assessment and mitigation, deep drilling, and reservoir development.
However, significant upfront capital costs and the geological risks (for example, not achieving the required temperatures) present a major barrier to the development of geothermal heat and power projects in the UK. This is where thorough upfront geoscientific analysis is needed to mitigate risk for geothermal projects.
Getech’s Heat Seeker solution considers a wide range of factors that combine to make a geothermal resource attractive, including not just technical geoscience considerations but also commercial and social factors that drive energy supply and demand.
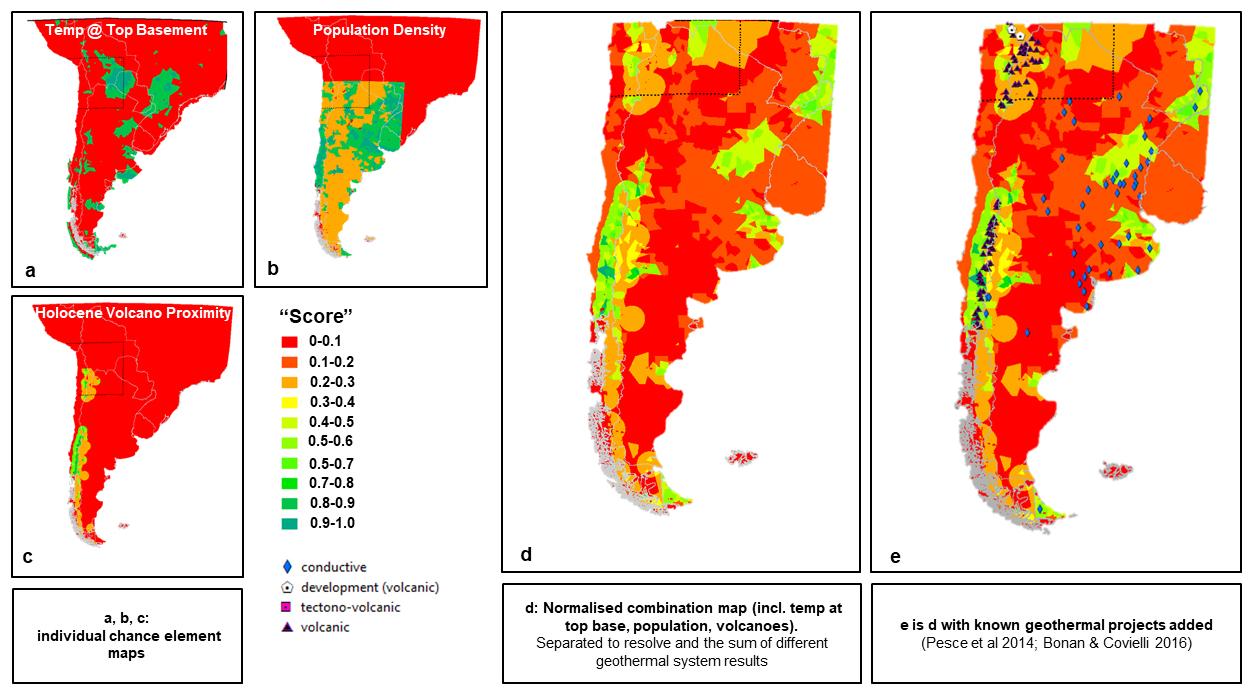
Figure 3. Initial geothermal favourability map, based on integrating supply and demand parameters.
Figure 4. Enhanced geothermal energy favourability mapping.
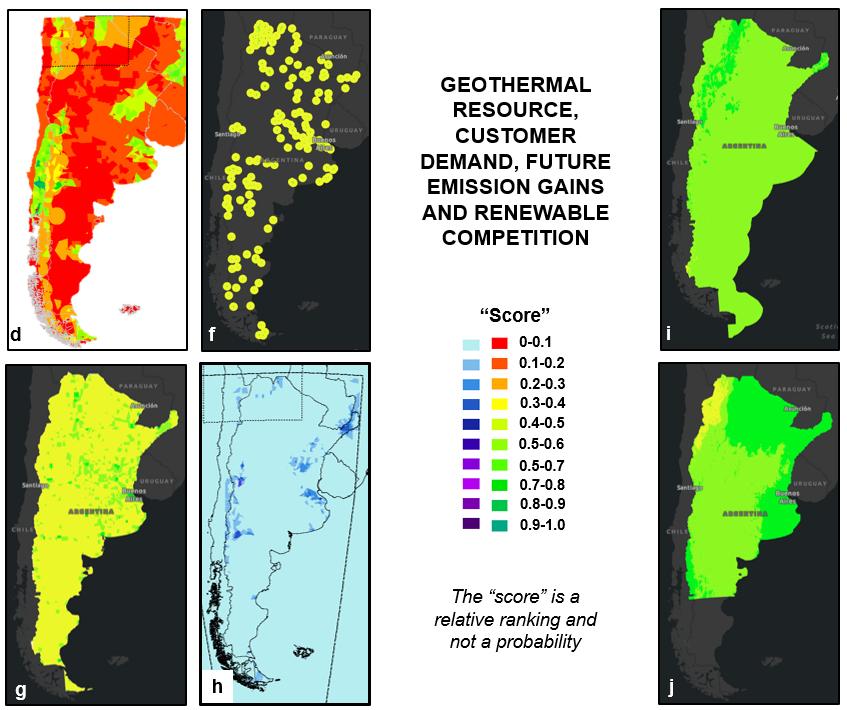
References
1. LUND, J., and TOTH, A., ‘Direct Utilisation of Geothermal Energy 2020 Worldwide Review’, February 2021. 2. https://www.egec.org 3. European Commission, ‘Heating and cooling’, Energy efficiency, 2022. 4. ABESSER, DR C., and WALKER DR A., ‘Geothermal energy’, UK Parliament POST, 27 April 2022. 5. UK Parliament POST, ‘Heat networks’, PostNote Number 632, September 2020. 6. Houses of Parliament Parliamentary Office of Science and Technology, ‘Developments in Wind Power’, PostNote Number 602, May 2019. 7. The Association for Renewable Energy and Clean Technology, ‘Government urged to help deliver a ‘world leading’ deep geothermal sector to secure the UK’s ‘green recovery’’, 13 May 2021. 8. RICHTER, A., ‘Geothermal – Greater Paris area making better and better use of enormous potential’, Think Geoenergy, 29 July 2020. 9. HOKSTAD, K., TAŠÁROVÁ, Z. A., CLARK, S. A., KYRKJEBØ, R., DUFFAUT, C., FICHLER, C., and WIIK, T., ‘Radiogenic heat production in the crust from inversion of gravity and magnetic data’, Norwegian Journal of Geology, vol. 97, no. 3 (2017), pp. 241-254. 10. PESCE, A., COIRA, B., and CASELLI, A. T., ‘Geotermia en Argentina desarrollo actual y potencial’, Geothermal Energy Workshop Salta, December (2014), pp. 44. 11. BONA, P., and COVIELLO, M. F., ‘Valoración y gobernanza de los proyectos geotérmicos en América del Sur’, CEPAL Report (2016), pp. 178.
Figure 1. In 2019, approximately 83% of Iceland’s primary energy supply came from indigenous renewable sources, of which 65% was geothermal.
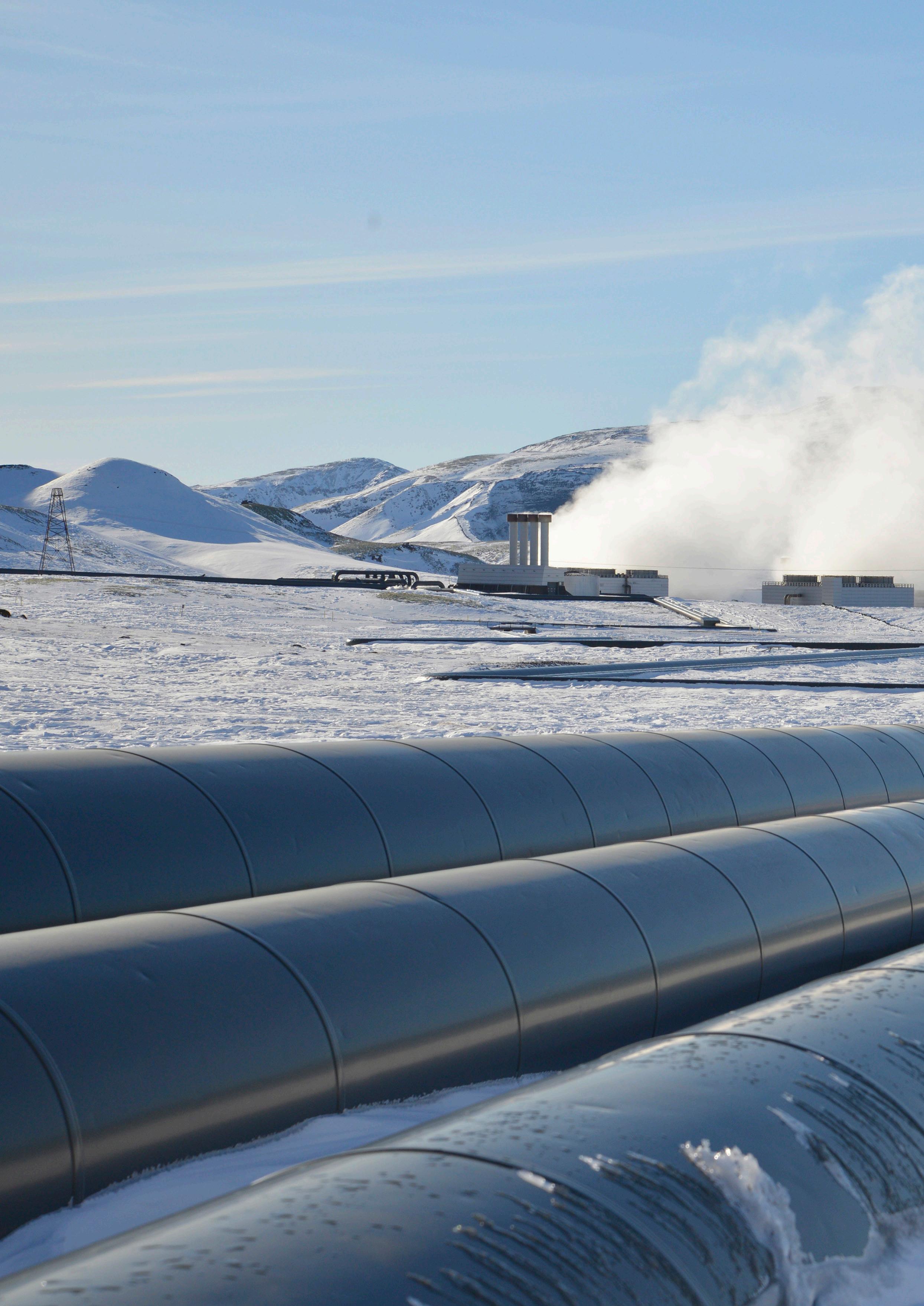
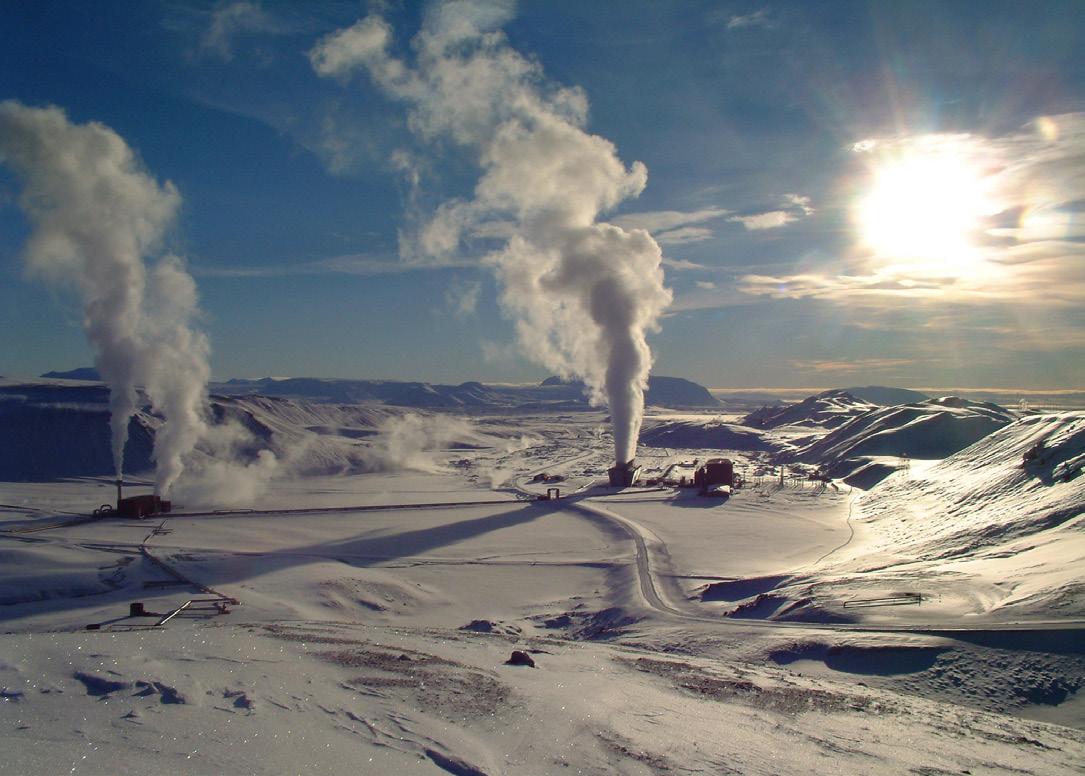
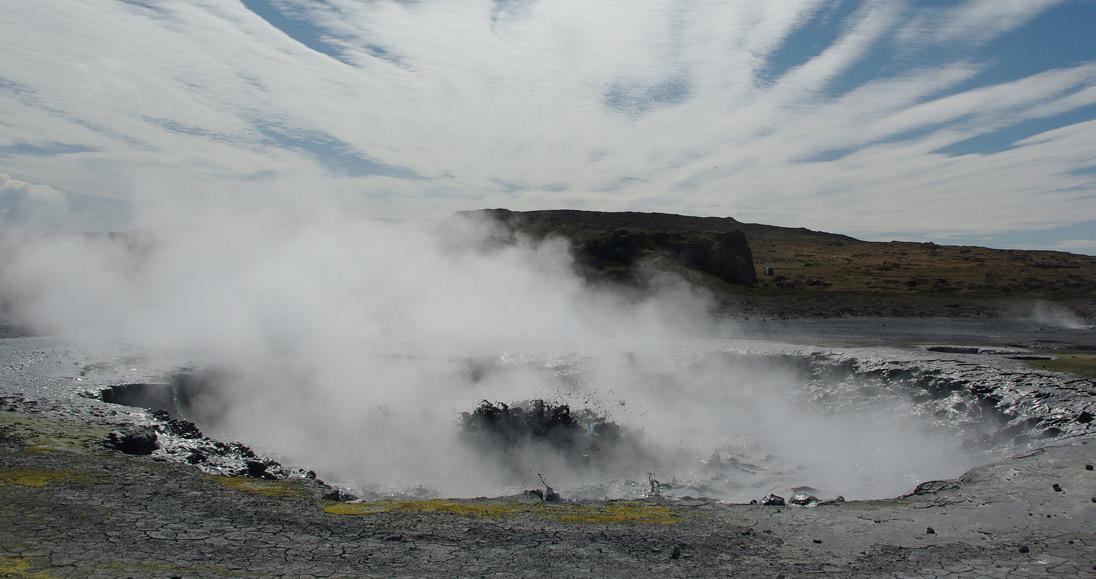
Árni Magnússon, Bjarni Richter, and
Arni Ragnarsson, ÍSOR, Iceland, explains the current landscape of geothermal research and utilisation in Iceland, outlining the ways in which this renewable resource has positively impacted the country.
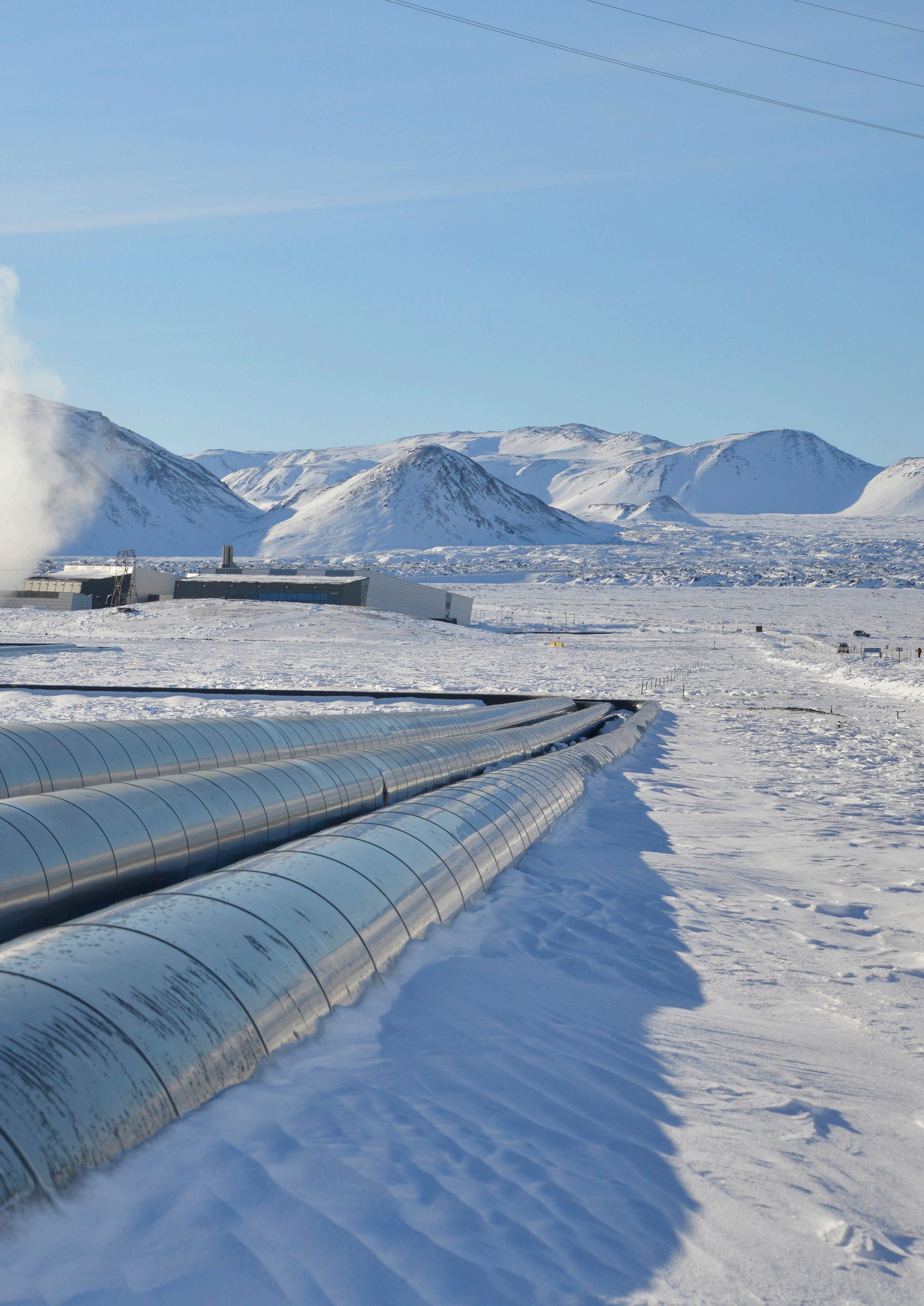
Iceland has a huge geothermal potential (Figure 2) based on the location of the country on a hot spot on the Mid-Atlantic Ridge. The country is mountainous and volcanic, with much precipitation, making hydropower resources also abundant. The population of Iceland is approximately 375 000, of which almost two-thirds live in the Reykjavik capital area. During the course of the 20th century, Iceland went from being one of Europe’s poorest countries, dependent on peat, dung, and imported coal for its energy, to a country with a high standard of living where practically all stationary energy, and approximately 83% of the primary energy supply, comes from indigenous renewable sources (65% geothermal and 18% hydropower in 2019). The rest comes mainly from imported fossil fuel used for the transport sector and fishing fleet. Iceland’s energy use per capita is among the highest in the world and the proportion provided by renewable energy sources exceeds most other countries. The electricity generation per capita in Iceland is by far the highest in the world, mainly due the energy intensive industry that uses over 75% of the electricity generated in the country.
History of geothermal research
Utilisation of the renewable energy sources started on a small scale early in the 20th century. Systematic geothermal research was initiated by the Icelandic government in the 1940s with the aim to acquire general knowledge about geothermal resources and make the utilisation of this resource profitable for the national economy. The country built up a group of skilled geothermal scientists
and researchers, especially regarding the development of geothermal district heating systems for heating of houses. A turning point in geothermal development in Iceland occurred in the early 1970s when the sharp rise in oil prices led to increased heating costs at a time when over half of the houses in Iceland were heated by oil. The government decided to reduce the use of oil as much as possible and further develop the domestic renewable energy sources (hydropower and geothermal), especially by exploring for new geothermal resources and building a new geothermal district heating system across the country. This development was partly supported by a special Energy Fund that was established by the government in 1967 to increase the use of geothermal resources. This fund has granted numerous loans for geothermal exploration and drilling. Where drilling failed to yield the expected results, loans were converted to grants.
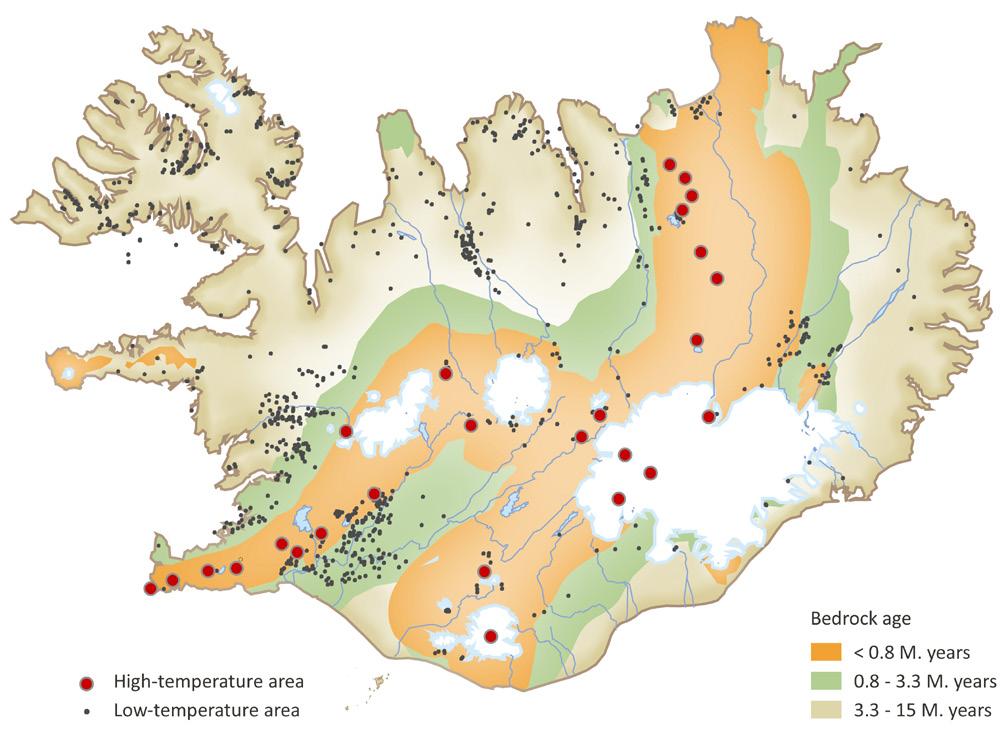
Figure 2. Overview of the volcanic zones and geothermal fields in Iceland.
Figure 3. The total power generation from geothermal in Iceland in 2020 was 5961 GWh e, which was 31% of the electricity produced in the country that year.
Figure 4. Geothermal supplies over 90% of heat for all houses in Iceland.
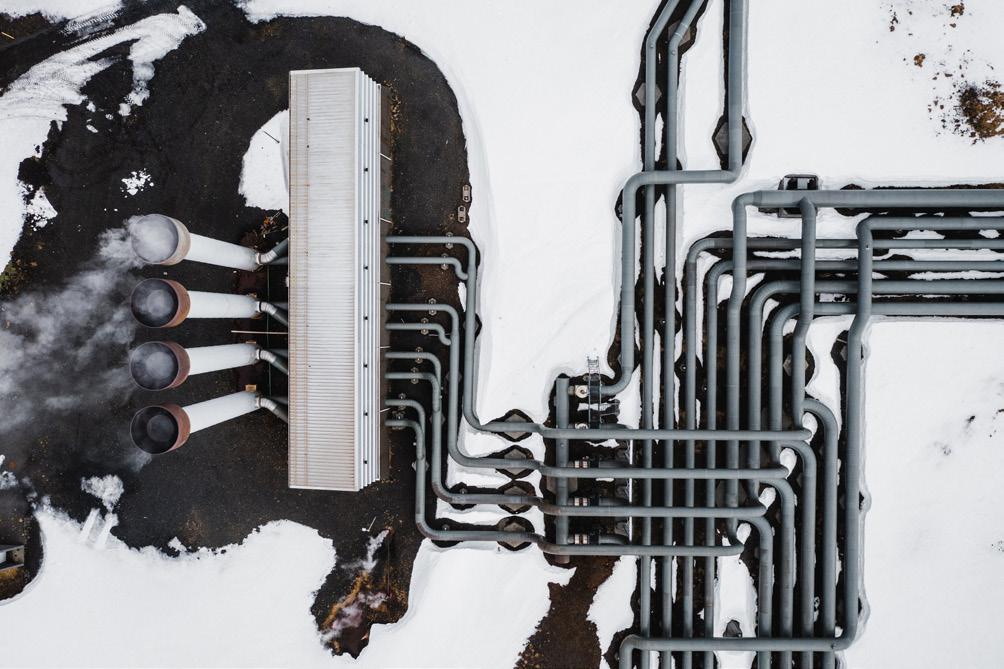
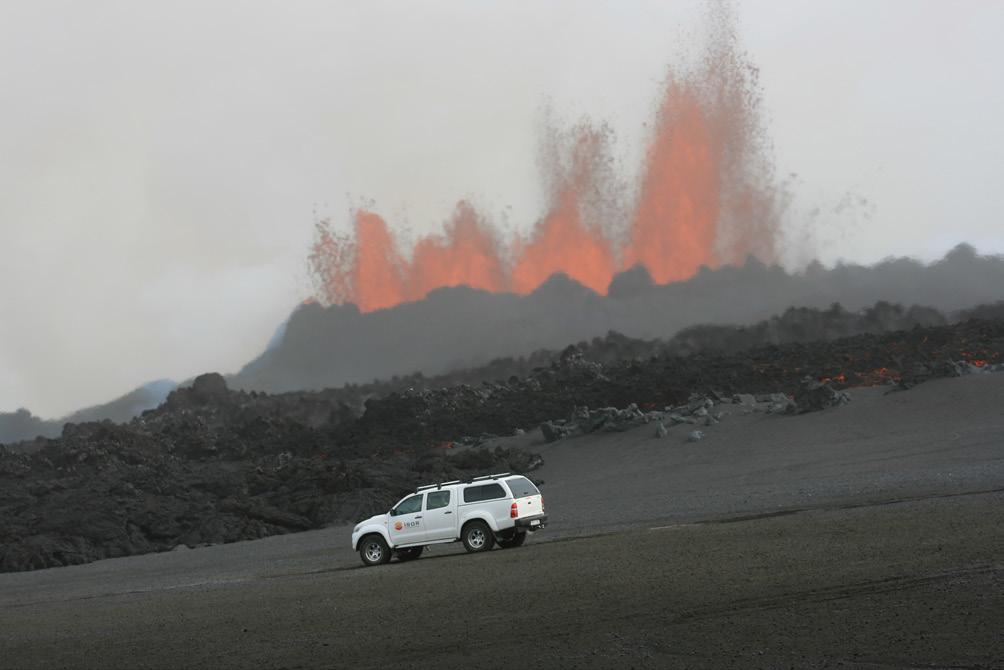
Geothermal power plants
The late 1990s were characterised by increasing interest in geothermal power plants and associated research. Significant progress was achieved in areas such as geothermal exploration and reservoir engineering. The practice of reinjection of the geothermal fluid back into the reservoir is getting more common, especially in high-temperature fields but also lowtemperature fields supplying hot water for district heating. This has improved the sustainability of the production considerably.
The early years of this millennia were characterised by construction of new geothermal power plants in high-temperature fields, mainly due to increased demand in the energy intensive industry. In total, seven such plants are now in operation in the country. Four of these plants are in the southwest of Iceland including the largest one, Hellisheidi power plant, producing 303 MW and 133 MW the for district heating. Three of the plants are in the northeast of Iceland. Additionally, one small geothermal power plant utilising the intermediate temperature resource is located in the south of Iceland. This kind of application is expected to increase in the future. The total power generation from geothermal in 2020 was 5961 GWhe, which was 31% of the electricity produced in the country that year.
Direct uses of geothermal
Direct uses of geothermal energy for different heating applications play a major role in the energy supply of Iceland. Long before power generation by geothermal started in 1969, hot geothermal water had been used for different applications. In terms of energy use as well as economic importance, the main use is house heating. By supplying heat for over 90% of all houses in the country, geothermal has had a huge positive influence on the economic development of the country and at the same time benefited the environment considerably through reduced emission of greenhouse gases (GHGs) compared to using fossil fuels for heating. The remaining 10% of house heating in Iceland is almost solely by electricity.
Other important direct use sectors of geothermal energy are the heating of swimming pools, the heating of greenhouses, industrial processes such as the drying of seaweed and fish, aquaculture farming, and snow melting.
How is geothermal used today?
The total direct use of geothermal energy in 2020 is estimated to have been 9737 GWh th (35 052 TJ). In some cases, the brine