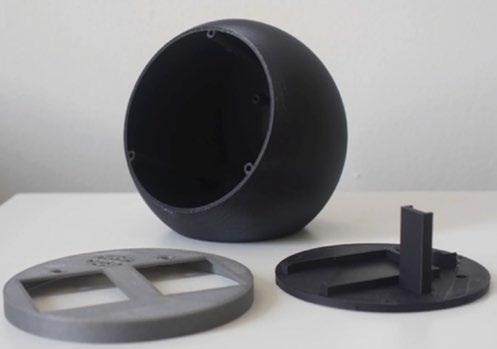
12 minute read
Department of Bioengineering, Department of Electrical Engineering, Department of Mechanical Engineering, Innovation, Product Design, and Entrepreneurship Program
Sophie Shapiroa,d, Stuart McCutchenb,d, Zeve Cohenc,d, Elizabeth Gilmanb,d, William Clarkb,d
aDepartment of Bioengineering, bDepartment of Electrical Engineering, cDepartment of Mechanical Engineering, dInnovation, Product Design, and Entrepreneurship Program
Sophie Shapiro Sophie Shapiro is a bioengineering major and mechanical engineering minor at the Swanson School of Engineering. Her interests include the solid and fluid biomechanics of pathology and treatment, product design, and entrepreneurship. She intends to use her interdisciplinary training to deliver innovative regenerative and restorative therapies to cardiovascular medicine.
Elizabeth Gilman Elizabeth Gilman has always had a passion for engineering and working with others. In the future she hopes to design and build devices that can improve people’s lives and the world around them.
Stuart McCutchen Stuart McCutchen is a junior electrical engineering student. He is passionate about product design and development, particularly in the tech industry. His interests consist of digital electronics, IoT, signal processing, and machine learning. He currently plans to pursue his masters in electrical engineering.
Zeve Cohen is a senior Mechanical Engineering student from Philadelphia. Driven by his innate creativity, Zeve is passionate about innovative design. His involvement in Pitt’s Makerspace has help fuel his love for design and fabrication. Zeve plans to work at the intersection of mechanical engineering Zeve Cohen and industrial design with a focus on product ideation and rapid prototyping. His broad interests could lead him to numerous industries, but his primary goal is to create products that help others. Professor William Clark joined the Mechanical Engineering and Material Science Department in 1992 and has an active research and teaching program in dynamic systems and controls. He participated in an SSOE faculty effort to create the Innovation, Product Design, and Entrepreneurship certificate proWilliam Clark, Ph.D. gram starting in 2014, which lead to the development of the Makerspaces in 2016 as well as subsequent student innovation opportunities. He currently directs the program, which invites students to participate in intensive design and innovation experiences in the summer. The paper presents the results of one of those teams.
Significance Statement
Preventing the spread of SARS-CoV-2 has resulted in research activity being significantly diminished at several institutions. To help mitigate exposure risks with in-person work, three individual product designs were developed with the cumulative goal of creating a safer environment as educational and research efforts adapt.
Category: Device Design
Key Words: SARS-CoV-2, engineering education, product design, prototyping
†Editors’ Choice
Abstract
The onset of the severe acute respiratory syndrome coronavirus 2 (SARS-CoV-2) pandemic significantly disrupted the engineering research community, as academia reluctantly transitioned to an all-remote learning environment. With many research and development endeavors being time-sensitive, the University of Pittsburgh looks to slowly restart and ramp-up research operations while establishing effective safety measures. Working within Pitt’s plan to reopen facilities, we propose three isolated product designs that will jointly reduce the risk of exposure. By utilizing new advances in additive manufacturing and software development, our product designs are aimed at helping researchers mitigate risk as essential in-person work resumes. Following several design iterations, the projects resulted in the following, fully functional prototypes: RFID-enhanced contact tracing system, mass customization facepiece workflow, and an open-sourced LED Interactive wall. The current state of our projects has collectively worked to manage the immediate risk of SARS-CoV-2 in a scalable and compliant-driven manner, while providing a foundation for future opportunities for these designs to grow.
1. Introduction
The novel virus, severe acute respiratory syndrome coronavirus 2 (SARS-CoV-2), emerged out of Wuhan, China in December 2019. As of June 2020, there have been over 10 million reported cases of SARS-CoV-2, and its infectious nature paired with a lack of curative solutions has led to a worldwide pandemic [1]. Due to the apparent danger, 107 countries had implemented national school closures [2]. The University of Pittsburgh was amongst many other higher educational institutions that were obligated to shut down and switch to an all-remote learning environment for the safety of students and faculty. These operating constraints significantly disrupted the engineering research community. Laboratories, specifically ones handling living organisms, could not transform to a virtual platform as quickly or successfully as their academic counterparts; many finding it difficult to ramp down their time-sensitive research to reduced personnel and essential work.
With campus activity initially restricted to essential work, the University of Pittsburgh’s Research Restart Working Group crafted policies and protocols intended to help boost research operations via implementing effective safety precautions [3]. Adjunctively, groups such as the Veser Laboratory and a joint effort by Pitt Engineering and Carnegie Mellon University helped to make 75% alcohol content hand-sanitizers and donate unused personal protective equipment (PPE) to essential workers, respectively [4]. As result of the combined response across Pitt’s and neighboring universities’ campuses, reopening has substantially increased with newly established safety measures. Working within Pitt’s plan to restart facilities, we propose three isolated product designs that will jointly contribute to the mitigation of exposure risks. The product designs help to improve the effectiveness of current, in-person safety measures. Our RFID-enhanced contact tracing system, mass customization facepiece workflow, and LED Interactive wall will collectively contribute to the ongoing effort to manage the risks of SARSCoV-2 in a scalable and compliant-driven manner, while providing a foundation for future iterations.
2. Methods
2.1 Contact tracing
2.1.1 Hardware design
The urgency to contact trace more effectively in Pitt research facilities led to an early-stage device design equipped to track and store the following user-specific information: personal identification, date and time of entry/ exit, and location. Working to increase usability through transparency and ease, the project was created as an open-source, easy to manufacture and assemble device. The multi-part device was modeled in Fusion 360 (2020, Autodesk, San Rafeal, California) and designed for additive manufacturing. The device requires users to scan their Pitt identification (ID) card to which it can then retrieve the necessary information about the user. Utilizing infrared light (IR) sensors, the device differentiates between the entry and exit of users via the placement of the user’s ID card. To reinforce this, the device’s front panel is marked with an “in” and “out” section, corresponding to entry or exit. For user feedback, two Adafruit LEDs (New York City, New York, Cat # 302) and an Adafruit Piezo Buzzer (New York City, New York, Cat # 160) inside the device display green or red and sound a two-note rising or falling note interval, respectively, dependent on entrance or exit; the complete feedback loop takes no more than five seconds.
2.1.2 RFID and software development
Within the actual device is an Adafruit Feather HUZZAH ESP8266 (New York City, New York) which processes data, controls outputs, and sends data over WIFI to a remote database. Its small build, WIFI capabilities, low price, and easy sourcing made it an effective and scalable solution.
The remote database is a host on a shared network with the contact tracing devices via a Raspberry Pi 4b. The database is in MariaDB (2019, MariaDB Corporation Ab, Espoo, Finland), and the database interface is phpMyAdmin (2020, phpMyAdmin Project, Brownsville, Texas). The database records the date and time of which the data was received, what room it is from, the Pitt ID, and whether they were entering or exiting the room. Data older than 28 days automatically deletes, eliminating the need for manual database updates. 2.2 Mass customization facepiece workflow
2.2.1 Facemask design
Following a user-discovery phase with medical personnel, the concept to create customized facemasks was pursued to ensure extended user comfort and a reliable seal. Within Fusion 360 software, a 3-D modeled
half-mask respirator was designed to accurately conform to any facial structure. The mask was optimized for efficient airflow while minimizing required material to avoid a cumbersome facemask.
2.2.2 Simulation-based deformities
In the 3-D procedural generation software, Houdini (2019, Side Effects Software, Toronto, Ontario), the mask body’s seal was controllably deformed to various facial 3-D models using a built-in gravity simulator. This allowed the mask’s seal to conform to the test subject’s unique facial features and facial landmark locations. Mesh parameters of the modeled mask were revised multiple times until negligible breaches between the user-to-seal interface and symmetric positioning of the mask body was attained. Breaches were distances less than 0.5 millimeters between the seal and face, and positioning was with respect to the midsagittal plane of the face.
2.2.3 Manufacturing and post-processing
Post deformation, the now customized mask body is fabricated using stereolithography (SLA) (Formlabs, Somerville, Massachusetts). 50A Elastic Resin (Formlabs, Somerville, Massachusetts, Cat # RS-F2-ELCL-01) was chosen for the first physical prototypes for its Shore durometer ranking. A universally-sized filter housing was attached to the mask’s universal interface via mechanical mechanisms. 2.3 Open-source LED Interactive wall
2.3.1 Software Development
The LED Wall is a 1200 RGB LED display made for the basement of Benedum Hall at the University of Pittsburgh. It involved the use of Python, primary data processor, and Java, primary graphics, to transfer data from a camera to the LEDs. Python (Version 3.9.0) was preferred for image processing due to the OpenCV library. To transfer image processing data to Java, a WebSocket was used to convert Python to a data package recognizable by Java.
A silhouette mirroring interaction was created using image differencing which drew a point wherever the camera detected a person’s location. The camera would start with an initial frame to identify the changes from the current frame and convert into coordinate points for the Java program. The program would then draw a shape based on the coordinates sent to it and generate an outline of a person.
The software created preview graphics that reflected what the actual pixelization of the silhouette would be if the program was physically being ran on the wall. This makes software development easier for future designers to develop and test code remotely.
2.3.3 Electrical Design Requirements
Two power supplies were needed to meet the power demand and maintain a reliable source of 5-volt power. The rewiring would allow each power supply to control half of the LEDs so providing more power to fewer LEDs. This decreased flickering and incorrect color changes in the LEDs. It also powered the peripheral devices such as the camera and Jetson-Nano (NVIDIA, Santa Clara, California).
3. Results
3.1 RFID-enhanced contact tracing
Figure 1: Contact tracing housing. 3D-Printed contact tracing housing consisting of three pieces and intended to enclose all necessary hardware.
The contact tracing electronics enclosure is a threepiece, screw-shut housing. Via additive manufacturing, it can be fabricated in 23 hours using low-cost Fused Deposition Modeling 3-D printers (Creality, Shenzhen, China). The database is accessed via phpMyAdmin and is password-protected for security purposes. As more labs utilize this system, its value will grow exponentially as more data is collected and used to isolate and track exposure on campus. 3.2 Mass customization facepiece workflow
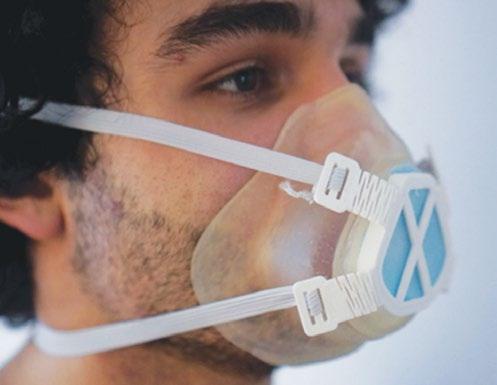
Figure 2: Customized facemask. Negligible breaches between the user-seal interface.
The semi-automated workflow allowed for customization for 6 users in one day. The utilization of SLA printing enabled rapid manufacturing times, giving a complete timeline for fabrication of approximately 9.5 hours. The filter housing includes an area for strap connections and
can accommodate a range of different filter materials. Further material selection and manufacturing methods are still being explored for both the mask body and filter housing, to increase mass customization. 3.3 Open-source LED Interactive wall
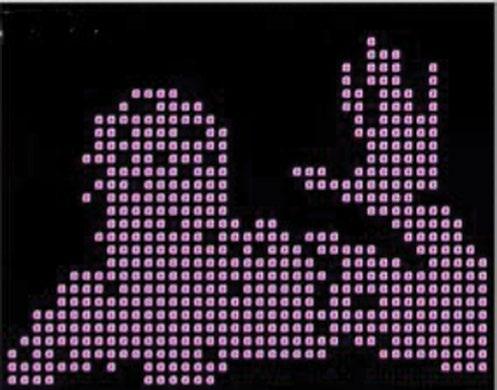
Figure 3: LED Interactive wall. Image differencing showing the sillhoute of someone in the camera’s view.
The LED Interactive wall is made up of 1200 LEDs. The camera and software involved enable graphics utilizing computer vision. The interaction demonstrated in Figure 3 shows the wall’s ability to mirror the silhouettes of passing pedestrians. Interactions such as these are the building blocks to developing even better computer vision programs, such as social-distancing and mask-wearing detection for students and faculty.
4. Discussion
The three functioning prototypes serve to promote the recommended safety precautions and catalyze the reopening of research facilities, all while providing a baseline for further design refinement and optimization. Both the contact tracing system and facepiece workflow significantly reduce the university’s dependency on third party compliance-related needs. Our contact tracing system provides an accessible and customizable alternative for universities to better streamline the tracking of SARS-CoV-2 amongst their campus. Currently, it is being tested at reopening research facilities at the University of Pittsburgh. The future entails reiterating on user feedback and scaling up the system to be successful in more labs and possibly, classrooms.
The facepiece workflow works jointly with our contact tracing system to encourage compliance within the workplace. An increase in individuals wearing PPE has the power to significantly reduce and manage the risk of exposure as campus activity increases. Future direction entails further streamlining the mask body design and fully automating the workflow to increase facepiece output. Having the ability to output predictable face fittings of masks both, digitally and remotely, has been an exciting breakthrough for enhancing the user-to-seal interface and subsequentially, student and faculty health.
In parallel with the mentioned endeavors, our LED Interactive wall reached the first of many breakthroughs in regard to helping manage the SARS-CoV-2 exposure risk. In achieving full body detection and tracking, it has paved a path for further expansion on this technology. With refinement and customization, various modules can be added to help drive compliance, such as social distancing and mask-wearing recognition programs. The LED Interactive wall not only serves as its own artform, but as a tool for reinforcing the precautions necessary for keeping in-person workers safe. Additionally, the wall was designed to be open-source and encourages further collaboration to achieve these next compliance-driven goals.
5. Conclusion
With little promise of an imminent eradication of SARS-CoV-2, it is imperative for institutions to adapt quickly. Student and faculty safety during this dangerous and uncertain era is of utmost importance to universities. Hence, in order to continue educating, researching, and fully operating, it is crucial to mitigate health risks. The three functional prototypes introduced in this paper present in-house tools that Pitt and other institutions can adopt to help manage those risks. The contact tracing system allows universities to localize the data that can help contain the spread of the virus on their campus. The facepiece workflow delivers a fast and personalized solution that addresses a fundamental challenge of increasing compliance with PPE. The LED Interactive Wall acts as an impartial advocator of compliance, accountability, and awareness. These are all scalable and customizable approaches that universities can take to safely and effectively reopen facilities in the face of a pandemic.
6. Acknowledgments
We thank all of our mentors for their outstanding support. We thank the Swanson School of Engineering for funding our endeavors.
7. References
[1] Viner RM, Russell SJ, Croker H, et al. School closure and management practices during coronavirus outbreaks including COVID-19: a rapid systematic review. Lancet Child Adolesc Health. 2020;4(5):397-404. doi:10.1016/S2352-4642(20)30095-X [2] Wiersinga WJ, Rhodes A, Cheng AC, Peacock SJ, Prescott HC. Pathophysiology, Transmission, Diagnosis, and Treatment of Coronavirus Disease 2019 (COVID-19): A Review. JAMA. 2020;324(8):782-793. doi:10.1001/ jama.2020.12839 [3] Swanson School of Engineering. 2020. Managing—Not Avoiding—Risks. [online] Available at: <https:// www.engineering.pitt.edu/News/2020/Research-Restart/> [Accessed November 2020]. [4] University Times. 2020. How the University community is helping out its neighbors. [online] Available at: <https://www.utimes.pitt.edu/news/how-university-community> [Accessed December 2020].