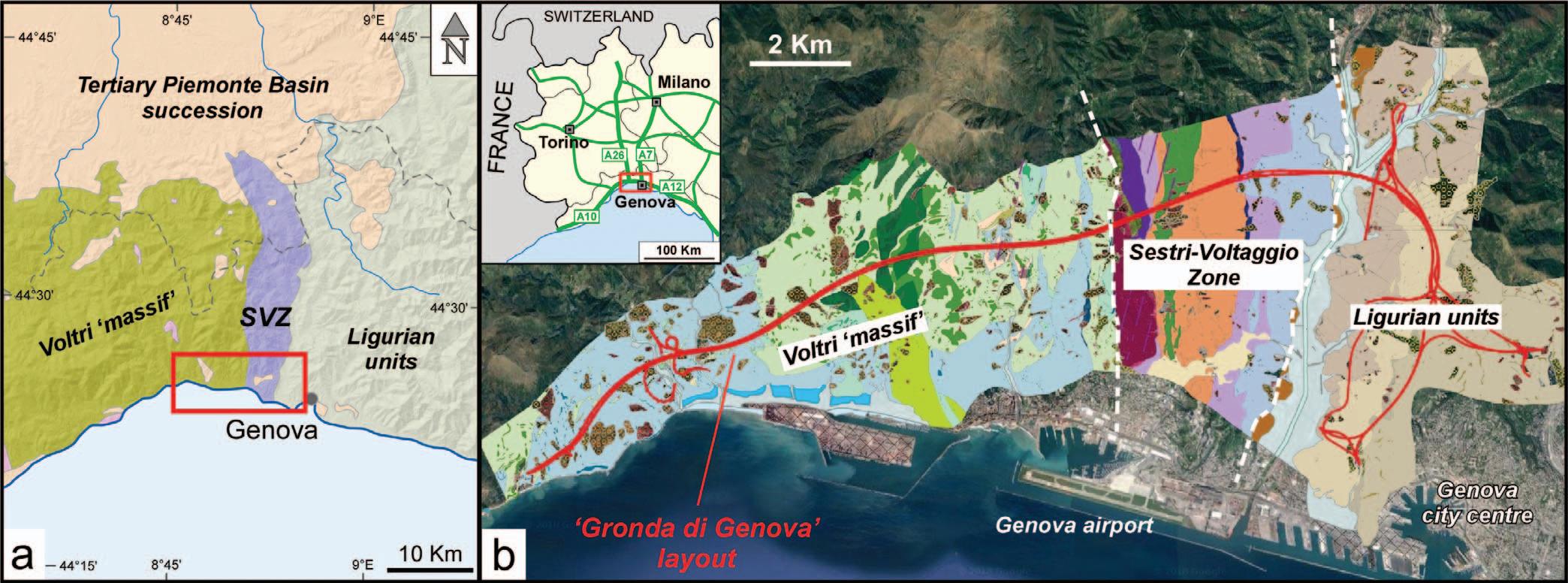
15 minute read
Geological Model for Naturally Occurring Asbestos Content Prediction in the Rock Excavation of a Long Tunnel (Gronda di Genova Project, NW Italy
from EEG Journal - February 2020 Vol. XXVI, No. I (2)
by Association of Environmental & Engineering Geologists (AEG)
Geological Model for Naturally Occurring Asbestos Content Prediction in the Rock Excavation of a Long Tunnel (Gronda di Genova Project, NW Italy)
LUCA BARALE FABRIZIO PIANA* SERGIO TALLONE Institute of Geosciences and Earth Resources, National Research Council of Italy, Via Valperga Caluso 35, 10125 Torino, Italy, and “G. Scansetti” Interdepartmental Centre for Studies on Asbestos and Other Toxic Particulates, University of Torino, Via Pietro Giuria 7, 10125 Torino, Italy
ROBERTO COMPAGNONI Department of Earth Sciences, University of Torino, and “G. Scansetti” Interdepartmental Centre for Studies on Asbestos and Other Toxic Particulates, University of Torino, Via Valperga Caluso 35, 10125 Torino, Italy
CHIARA AVATANEO SERENA BOTTA IGOR MARCELLI Gi-RES srl, Via Gottardo 223, 10154 Torino, Italy
ANDREA IRACE Institute of Geosciences and Earth Resources, National Research Council of Italy, University of Torino, Via Valperga Caluso 35, 10125 Torino, Italy
PIETRO MOSCA Institute of Geosciences and Earth Resources, National Research Council of Italy, Via Valperga Caluso 35, 10125 Torino, Italy
ROBERTO COSSIO Department of Earth Sciences, University of Torino, Via Valperga Caluso 35, 10125 Torino, Italy
FRANCESCO TURCI Department of Chemistry, University of Torino, and “G. Scansetti” Interdepartmental Centre for Studies on Asbestos and Other Toxic Particulates, University of Torino, Via Pietro Giuria 7, 10125 Torino, Italy
Key Terms: NOA, Asbestos, Rock Tunneling, Geological Model, Meta-Ophiolites
ABSTRACT
For a reliable evaluation of the geo-environmental risk due to naturally occurring asbestos (NOA) during rock excavation of large infrastructure projects, a proper procedure is needed. First, it is necessary to
*Corresponding author email: fabrizio.piana@cnr.it
provide a detailed geological model tailored to the NOArelated issues that should drive the rock sampling procedures in order to obtain a representative sampling. The sampling procedures should take into account lithological variability and relative spatial distributions of the rock units. The geological model for NOA should be thus constrained by the main NOA petrofacies occurring in a given geotectonic context, which take into consideration both the mineralogical and structural features, and the identification of NOA homogeneous zones in which the NOA petrofacies are distributed. In this paper, some geo-environmental problems faced during the
excavation, in meta-ophiolites, of a long highway tunnel are described. The geological model of the complex setting of the tunnel area (northern Italy, Alps-Apennines junction) is described, focusing on how the NOA-related problems were addressed to allow reliable and detailed estimations of NOA contents for each NOA homogeneous zone and the relevant tunnel layout segment.
INTRODUCTION
The Gronda di Genova project concerns the realization of a highway bypass around the city of Genoa in NW Italy, aiming at relieving the traffic from the existing highway system, which connects the port and the city of Genoa with the rest of Italy and adjoining countries (Figure 1). The project, presently in the working plan phase, proposes 72 km of new highway, including 54 km of tunnels; of these, about half will be excavated in potentially asbestos-bearing rocks (meta-ophiolitic units).
The construction of large infrastructure in rocks with naturally occurring asbestos (NOA) poses several geo-environmental issues, such as adequate preparation of the construction site, workers’ safety, and spoil management. The estimated total amount of spoils that will derive from the Gronda di Genova tunnel excavation is about 11,000,000 m 3 (ASPI, 2011). Some of these spoils are expected to contain NOA above the concentration threshold fixed by the Italian regulation (NOA limit, in the following); this limit corresponds to a concentration of asbestos in the rock of 1,000 mg/kg (or ppm; Italian regulation Decreto Legislativo 152/06 (Repubblica Italiana, 2006). Spoils below the NOA limit or without NOA will be mainly re-used to fill the tunnel invert and for filling work in the stilling channel of the Genoa airport to enlarge the runway strip. The latter use will allow disposal of about 8,000,000 m 3 of spoils (ASPI, 2011). Conversely, spoils above the NOA limit represent, by law, a hazardous waste and have to be disposed of in special dumps, thus resulting in high disposal costs. For this reason, a preliminary evaluation of the expected amount of asbestiferous spoils was deemed necessary in the first phases of project development (results of early studies were reported in Giacomini et al., 2010; Turci et al., 2015). This task required a multi-disciplinary team of researchers and professionals with varied expertise including structural geology, petrography, mineralogy, and analytical chemistry.
The first step was the creation of an ad hoc geological model tailored to NOA issues (cf. Labagnara et al., 2013; Vignaroli et al., 2014) to guide representative sampling of NOA-bearing rocks. The whole set of information derived from field observations, drill core analysis, and petrographic studies, together with the data on NOA content provided by scanning electron microscopy–energy dispersive X-ray spectroscopy (SEM-EDS) quantitative analyses, allowed definition of several NOA-targeted petrostructural facies (Botta et al., 2019), categorized into four NOA-content classes (very high, high, low, nil). The distribution of NOA petrofacies was then presented in detailed geological sections along the project layout, thus allowing prediction of the total volume of NOA-bearing rocks along the tunnels.
Figure 1. (a) Simplified geological scheme of the Genoa area; SVZ = Sestri-Voltaggio Zone. (b) Excerpt of the geological map produced for the present study, with indication of the main geological units crossed by the Gronda di Genova project layout. In the inset, the position of the Genoa area within the highway system of NW Italy is shown (existing highways are indicated by green lines).
The Genoa area is located in the Alps-Apennines “interference zone” (sensu Piana et al., 2017), made up of several geological units that shared, at least in part, the evolution of both orogens. From west to east, the geological units crossed by the Gronda di Genova layout are (Capponi and Crispini, 2008; Capponi et al., 2016, with references therein; Figure 1): the Voltri “massif,” made up of meta-ophiolites and relevant meta-sedimentary cover; in the study area, the Voltri “massif” is composed of two tectono-metamorphic units, the Voltri and PalmaroCaffarella units, which experienced, respectively, eclogitic- and blueschist-facies peak metamorphism during the Alpine orogeny; the Sestri-Voltaggio Zone, a several-kilometer-large tectonic slice zone containing both meta-ophiolitic and non-ophiolitic rock sequences, which recorded blueschist- to prehnite-pumpellyite–facies Alpine metamorphism; and the Ligurian units, corresponding to a very lowgrade to non-metamorphic hemipelagic sedimentary succession of Cretaceous age.
These units underwent a polyphase tectonometamorphic evolution, including early phases of isoclinal to tight folding (D1 and D2 phases), which generated composite, transposed regional foliation, and later phases (D3 and D4) responsible for the generation of meter- to kilometer-scale gentle to open folds, associated with reverse faults and shear zones (Capponi and Crispini, 2008, with references).
The Voltri massif and the Sestri-Voltaggio Zone units are presently juxtaposed along a N-S–striking deformation zone, known in the literature as “Sestri-Voltaggio line, which acted as a zone of preferential shear concentration at different crustal levels during the polyphase evolution of the AlpsApennine system (Crispini et al., 2009; Piana et al., 2017, with references).
NOA minerals (chrysotile and actinolite-tremolite amphiboles) are widespread in the meta-ophiolitic sequences of the Voltri massif and of the SestriVoltaggio Zone, whereas they are absent in the (meta-)sedimentary Ligurian units.
METHODS
NOA-Oriented Geological Model and Sampling Strategy
The construction of the NOA-oriented geological model primarily relied on detailed geological mapping at 1:5,000 scale along a 3- to 5-km-large belt around the tunnel layout (Figure 1b). This was accompanied by detailed structural analysis to achieve a thorough view of mesoscale structural features of NOA-bearing lithotypes and subdivision of the study area into homogeneous structural domains. Field surveys were integrated using the analysis of a large amount of available drill cores and logs (about 300 drillings were produced for the Gronda di Genova project, and data from hundreds others drilled in the area were obtained from public and private agencies). Integration of field and drilling data allowed the production of 1:5,000 scale geological sections along both tubes of the main tunnels and underground access ramps.
In parallel, a detail petrographic analysis was conducted, focusing on potentially NOA-bearing lithotypes (Botta et al., 2019). Particular attention was dedicated to the description of veins, with respect to their composition, thickness, and presence of fibrous minerals (either regulated or non-regulated) and elongated/acicular minerals. All of this information provided an important support to subsequent SEM observations made for quantitative analysis. Optical microscopy has always been coupled with microRaman spectroscopy, which has been useful both in the univocal distinction of serpentine polymorphs (e.g., Rinaudo et al., 2003; Groppo et al., 2006), and in the determination of fine-grained minerals or mineral aggregates.
At this point, after considering all the acquired geological and petrographic information, a proper strategy for representative sampling could be planned. Sampling was guided by two criteria: the lithological and the statistical representativeness. The first criterion guaranteed that all the lithotypes and lithological varieties identified in the study area were represented by at least one sample (logically, more abundant lithotypes were granted a higher number of samples than the rarest ones). The statistical representativeness was intended to have samples covering all the structural domains, independently of their lithology, evenly distributed along the project layout. Each sample was divided into two sub-samples, with one utilized for thin section preparation and the other (around 1 kg) prepared for quantitative analysis.
Quantitative Evaluation of NOA Content
SEM-EDS quantitative analyses represented the central part of the work and were made on 190 selected rock samples (106 from outcrops and 84 from drill cores). Twenty-one soil samples were also analyzed to evaluate the asbestos content in the surficial cover at the main open-air construction sites (e.g., tunnel entrances). The broad range of analytical cases encountered during this project, together with the previous
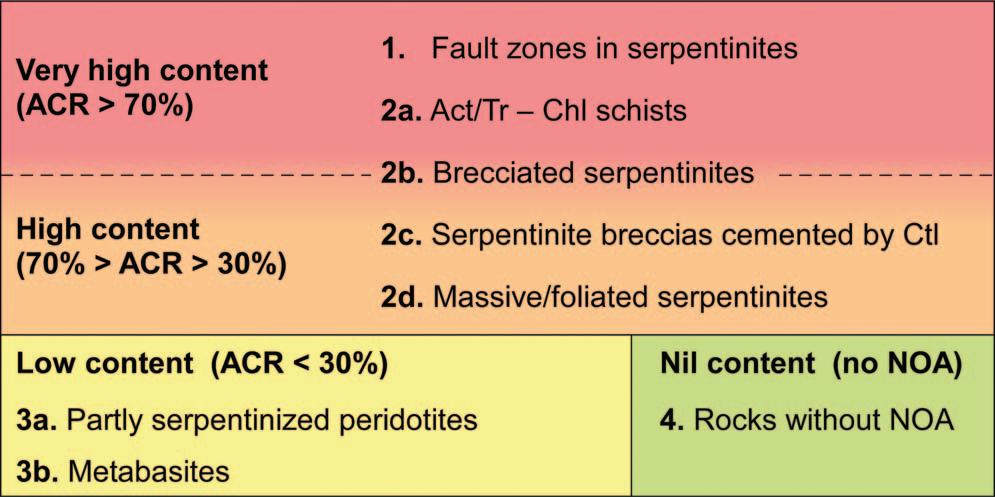
Figure 2. NOA petrofacies defined for the Gronda di Genova project, and their subdivision in very high, high, low, and nil content classes.
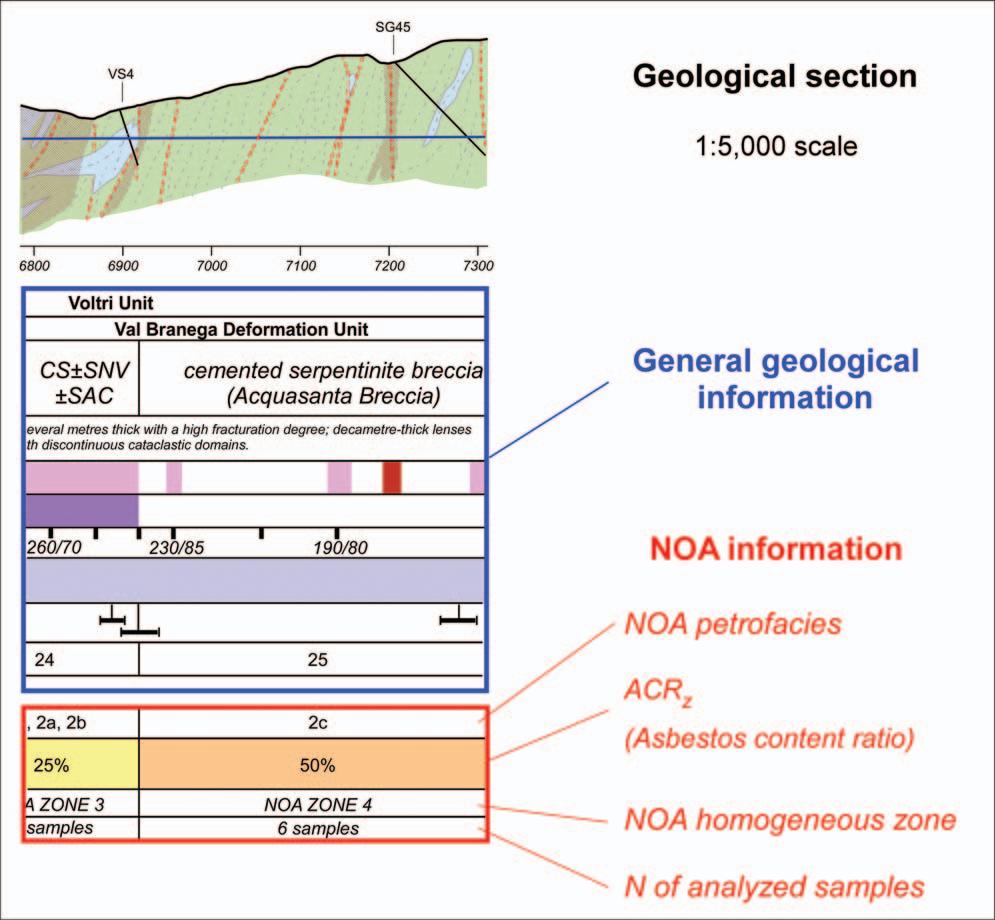
knowledge acquired in analogous studies, allowed our group to address important issues regarding the analytical procedure, including the definition of guidelines and best practices for sample preparation and analysis (see Turci et al., in press).
The results of quantitative analyses were integrated with the data derived from petrography and structural analysis to define several NOA petro-structural facies (NOA petrofacies). NOA petrofacies are classes of rocks that share common lithological-structural features and NOA content. NOA petrofacies were subdivided into four NOA content classes (Figure 2) according to their asbestos content ratio (ACR), i.e., the ratio between the number of samples in which NOA content is higher than 1,000 mg/kg and the total number of analyzed samples.
Very-high-content petrofacies and high-content petrofacies contain asbestos commonly above 1,000 mg/kg (ACR > 30 percent); these include most types of serpentinites and serpentinite breccias (petrofacies 2b, 2c, 2d), as well as actinolite/tremolitechlorite schists (SAC, petrofacies 2a) and fault rocks developed at the expense of serpentinites (petrofacies 1). Low-content petrofacies are those that do contain NOA, but commonly below 1,000 mg/kg (ACR < 30 percent); they comprise partly serpentinized peridotites (petrofacies 3a), and metabasites (petrofacies 3b). The nil content group corresponds to NOA petrofacies 4; this petrofacies groups all lithotypes that do not contain NOA, for which further distinction was useless for the aims of the present work (Botta et al., 2019).
Following the identification of the NOA petrofacies present in the area, the tunnels were subdivided along their entire length into NOA homogeneous zones, i.e., tunnel segments characterized by the presence of a distinct NOA petrofacies or an association of a few NOA petrofacies. For each NOA homogeneous zone, the ACR z was defined, representing the ratio between
Figure 3. Excerpt of the geological section along the main tunnel of the Gronda di Genova project. The geological section is accompanied by a table identifying the main geological information, including NOA information in the last four rows.
the rock volume above the NOA limit and the total rock volume:
ACR z = rock volume above NOA limit total rock volume .
Each NOA zone had its own ACR z based on the type and relative proportions of NOA petrofacies. The ACR z was calculated as the sum of the ACR of all the petrofacies within a given NOA zone, each one multiplied by a correction factor (ranging between 0 and 1), which represented the relative volume of each NOA petrofacies in that NOA zone. The obtained ACR z values were reported on the complete geological sections of the tunnels (Figure 3), together with general geological data (e.g., structural domain, lithology, presence and type of faults and fault rocks) and other NOA information (homogeneous zone, petrofacies, NOA content class, and number of analyzed samples). The ACR z was then utilized to calculate, for each homogeneous zone, the volume of rocks above 1,000 mg/kg, by multiplying the zone length by the tunnel section area and by the ACR z itself:
Volume above 1, 000 mg/kg = homogeneous zone length × tunnel section area × ACR z .
By adding the volumes obtained for each NOA homogeneous zone, the total volume of rocks above NOA limit (1,000 mg/kg) for the Gronda di Genova project was estimated as 978,000 m 3 of in situ rock. This
corresponds to about 1,270,000 m 3 of spoils, considering a bulking factor of 1.3.
The “Frac_Asbestos” Test
In parallel with the above described work, a test on semi-quantitative prediction of asbestos content in a given rock volume was conducted based on mathematical modeling of fracture systems (“Frac_Asbestos”; Piana et al., in press).
The test was made on an outcrop of intensely fractured lizardite serpentinites with lizardite and chrysotile veins. On this outcrop, the main fracture systems were identified and characterized both geometrically (orientation, spacing, persistence) and petrographically (occurrence and type of vein minerals). This characterization was followed by a semi-automatic extraction of fracture traces on a three-dimensional laser scanner image; discrete fracture network modeling was then completed to obtain the “fracture intensity” (i.e., the total amount of fracture surface per unit volume). The obtained fracture intensity was utilized to calculate the NOA weight per unit volume, by multiplying by a series of factors obtained from outcrop observation and thin section study.
To validate the test, the calculated NOA concentration was compared with the results of quantitative analyses made on samples from three cores drilled on the same outcrop in roughly perpendicular directions and intended to be representative of the whole rock mass.
CONCLUSIONS
The evaluation of NOA content in the Gronda di Genova highway project highlighted the importance of: a geological model tailored to NOA issues, to define structural domains and to constrain representative sampling; definition of NOA petro-structural facies by comparing data from structural analysis, petrography, and quantitative NOA analyses, beginning with first stages of sampling; and different approaches, including NOA petrostructural facies or fracture network modeling to calculate NOA-bearing rock volumes.
ACKNOWLEDGMENTS
This work was developed in the frame of the geoenvironmental model for the “Gronda di Genova” working plan (highway bypass of Genoa city, Italy, designed by Autostrade per l’Italia [ASPI], Rome), commissioned by Spea Engineering spa, Milan (Italy), to a consortium comprising the “G. Scansetti” Interdepartmental Center for Studies on Asbestos and Other Toxic Particulates of the University of Torino (Italy), the Institute of Geosciences and Earth Resources of the National Research Council of Italy, Torino, and Gi-RES srl (a Consiglio Nazionale delle Ricerche [CNR] spin-off company), Torino, Italy.
The authors are indebted to Girolamo Belardi, Daniele Passeri, and Francesca Trapasso (Institute of Environmental Geology and Geoengineering [CNRIGAG], Rome) for designing and performing comminution of the samples. A research paper on this last complex and unexplored subject is currently being prepared. Francesco Cipolli (Spea Engineering, Genoa), and Vittorio Boerio and Simona Polattini (Spea Engineering, Milan) are kindly acknowledged for their continuous support and feedback throughout all working stages. Mark Bailey and two anonymous reviewers are kindly acknowledged for their comments on an earlier version of the manuscript.
REFERENCES
ASPI (Autostrade per l’Italia), 2011, Opera a Mare nel Canale di Calma. Parte Descrittiva Generale. APG9030: Electronic document (in Italian), available at http://www.va.minambiente.it/ File/Documento/25374. Botta, S.; Avataneo, C.; Barale, L.; Compagnoni, R.; Cossio, R.; Marcelli, I; Piana, F.; Tallone, S.; and Turci, F., 2019, Petrofacies for the prediction of NOA content in rocks: Application to the ‘Gronda di Genova’ tunnelling project: Bulletin Engineering Geology Environment, https://doi.org/10.1007/s10064-019-01539-6. Capponi, G. and Crispini, L., 2008, Carta Geologica d’Italia alla scala 1.50.000 e Note Illustrative. Foglio 213-230 Genova: APAT, Rome, Italy. Capponi, G.; Crispini, L.; Federico, L.; and Malatesta, C., 2016, Geology of the Eastern Ligurian Alps: A review of the tectonic units: Italian Journal Geosciences, Vol. 135, No. 1, pp. 157–169. Crispini, L.; Federico, L.; Capponi, G.; and Spagnolo, C., 2009, Late orogenic transpressional tectonics in the “Ligurian Knot”: Italian Journal Geosciences, Vol. 128, pp. 433–441. Giacomini, F.; Boerio, V.; Polattini, S.; Tiepolo, M.; Tribuzio, R.; and Zanetti, A., 2010, Evaluating asbestos fibre concentration in metaophiolites: A case study from the Voltri Massif and Sestri-Voltaggio Zone (Liguria, NW Italy): Environmental Earth Sciences, Vol. 61, pp. 1621–1639. Groppo, C.; Rinaudo, S.; Cairo, D.; Gastaldi, R.; and Compagnoni, R., 2006, Micro-Raman spectroscopy for a quick and reliable identification of serpentine minerals from ultramafics: European Journal Mineralogy, Vol. 18, No. 3, pp. 319–329. Labagnara, D.; Patrucco, M.; Rossetti, P.; and Pellegrino, V., 2013, Predictive assessment of asbestos content in the Western Italian Alps: An essential tool for an effective approach to risk analysis in tunneling operations and muck reuse: Environmental Earth Sciences, Vol. 70, pp. 857–868.
Piana, F.; Barale, L.; Botta, S.; Compagnoni, R.; Fidelibus, C.; Tallone S.; Avataneo C.; Cossio, R.; and Turci, F., in press, Direct and indirect assessment of the amount of naturally occurring asbestos in fractured rocks: Boletín Geológico Minero. Piana, F.; Fioraso, G.; Irace, A.; Mosca, P.; d’Atri, A.; Barale, L.; Falletti, P.; Monegato, G.; Morelli, M.; Tallone, S.; and Vigna, G.B., 2017, Geology of Piemonte region (NW Italy, Alps-Apennines interference zone): Journal Maps, Vol. 13, No. 2, pp. 395–405. Repubblica Italiana, 2006, Decreto Legislativo 3 aprile 2006, n. 152. Gazzetta Ufficiale, Serie Generale n.88 del 14-04-2006 - Suppl. Ordinario n. 96: Electronic document (in Italian), available at http://www.gazzettaufficiale.it/eli/gu/2006/04/14/ 88/so/96/sg/pdf Rinaudo, S.; Gastaldi, D.; and Belluso, E., 2003, Characterization of chrysotile, antigorite and lizardite by FT-Raman spectroscopy: Canadian Mineralogist, Vol. 41, pp. 883–890. Turci, F.; Avataneo, C.; Botta, S.; Marcelli, I.; Barale, L.; Tomatis, M.; Cossio, R.; Tallone, S.; Piana, F.; and Compagnoni, R., in press, New tools for the evaluation of asbestosrelated risks during excavation in NOA-rich geological setting: Environmental and Engineering Geoscience (in press). Turci, F.; Compagnoni, R.; Piana, F.; Delle Piane, L.; Tomatis, M.; Fubini, B.; Tallone, S.; Fuoco, S.; and Bergamini, M., 2015, Geological and analytical procedures for the evaluation of asbestos-related risk in underground and surface rock excavation. In Lollino, G., et al. (Editors), Engineering Geology for Society and Territory, Vol. 5: Springer, Berlin, Germany, pp. 619–622. Vignaroli, G.; Ballirano, P.; Belardi, G.; and Rossetti, F., 2014, Asbestos fibre identification vs. evaluation of asbestos hazard in ophiolitic rock mélanges, a case study from the Ligurian Alps (Italy): Environmental Earth Sciences, Vol. 72, pp. 3679–3698.