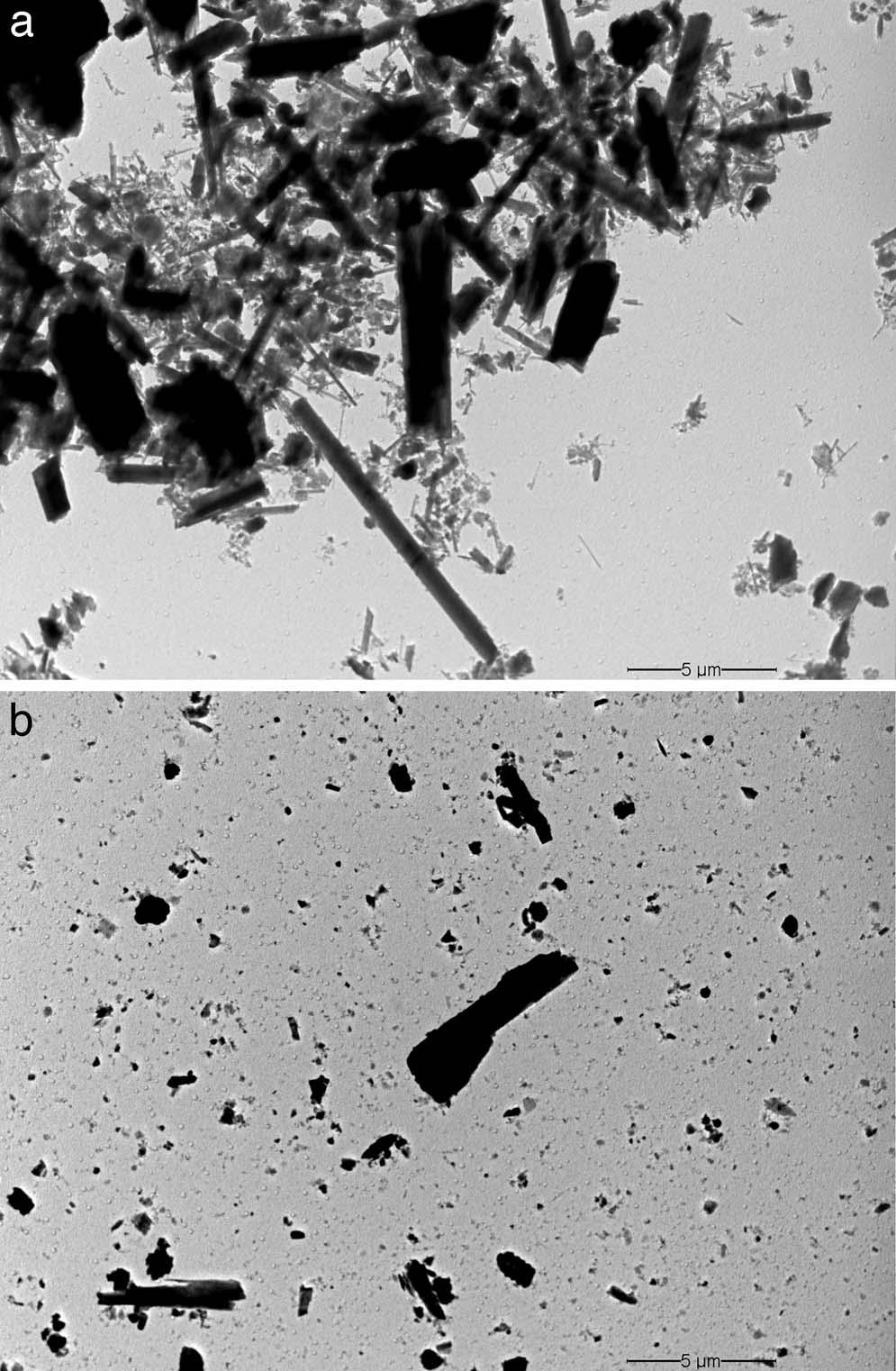
18 minute read
Discerning Erionite from Other Zeolite Minerals during Analysis
from EEG Journal - February 2020 Vol. XXVI, No. I (2)
by Association of Environmental & Engineering Geologists (AEG)
ROBYN RAY* EMSL Analytical, Inc., 200 Route 130 North, Cinnaminson, NJ 08070
Key Terms: Erionite, Transmission Electron Microscopy, Liquid Nitrogen Cryogenic Holder, Cold Stage, Offretite, Quantitative EDS, Asbestos
ABSTRACT
Erionite, a naturally occurring fibrous mineral that belongs to the zeolite group has been designated by the International Agency for Research on Cancer (IARC) as a Group 1 Carcinogen on the basis of mesothelioma, a disease also resulting from the inhalation of asbestos fibers. Significant outcrops of fibrous erionite have been reported in California, North Dakota, Nevada, Oregon, and other states. For geologists and industrial hygienists dealing with mining, construction, or various aspects of community protection, it is vital to understand the basics of detecting and handling erionite, since it is similar to asbestos and can cause similar disease. There are many fibrous zeolites, and discerning erionite from these other minerals requires modifications to current asbestos analysis methods. Without these modifications, identification and quantification are questionable and could increase the likelihood of both false negatives and false positives. There is currently no published method specific to erionite analysis; without guidance standards, each laboratory has approached erionite analysis independently. With a few small but significant changes to asbestos analysis methodologies, we developed a reproducible analytical procedure for rapid identification of erionite fibers in air, bulk, and soil samples by transmission electron microscopy (TEM). Using specialized preparation techniques, energy dispersive Spectrometry (EDS) calibrations, and a liquid nitrogen cryo-holder (cold stage), we were able to overcome the difficulties associated with erionite analysis. By incorporating these changes, commercial analytical laboratories can contribute reliable data to air-exposure studies and characterization guidelines, which may help in determining regulations and further understanding the health risks of erionite.
*Corresponding author email: rray@emsl.com
INTRODUCTION
Erionite is a member of a large group of hydrated aluminosilicate minerals called zeolites. Zeolites are found in altered volcanic tuffs, ash, and the soils derived from them. In Turkey, these volcanic tuffs are used as building stone for houses (Carbone et al., 2011). Similar to asbestos mineral fibers, erionite mineral fibers have been shown to cause to cancer (International Agency for Research on Cancer [IARC], 2012; Saracci, 2015). Internationally, there are areas in Turkey (Dogan et al. (2008)) and Mexico (Ilgren et al., 2008; Kliment et al., 2009) where malignant mesothelioma has been attributed to erionite that was found in the local environment. There are several occurrences of erionite worldwide. In the United States, it is predominately found in the intermountain west: California, North Dakota, Nevada, Oregon, and other states (Van Gosen et al., 2013, Van Gosen et al. (1996), USGS (1996)).
Erionite has not been mined for commercial use since the late 1980s (National Toxicology Program [NTP], 2004). Therefore, many commercial laboratories focusing on asbestos in bulk building material are inexperienced in handling erionite. In fact, in 2009, when erionite was provided as an unknown sample on the transmission electron microscopy (TEM) proficiency round for the National Voluntary Laboratory Accreditation Program (NVLAP), only 3 out of 76 accredited laboratories identified it as erionite (National Institute of Standards and Technology, 2009).
Erionite is not regulated as an asbestos mineral in the United States, and no exposure limits have been published. The potency of erionite has been shown in animal studies, where erionite is 500–800 times more tumorigenic than chrysotile and 200 times more tumorigenic than crocidolite (Carbone et al., 2011). Exposure to erionite may occur during mining and production of other zeolites where erionite is found as a contaminant (Rom et al., 1983). Erionite-related diseases are being studied among road construction and maintenance workers who may have been exposed to erionite containing gravel used in road surfacing (National Institute of Occupational Safety and Health [NIOSH], 2014). In 2017, the Nevada Department of Transportation (NDOT) started conducting
surveys of their right of ways, pit material, and import material for natural occurrences of asbestos and erionite (NDOT, 2018).
Evidence for non-cancerous outcomes due to erionite exposure has also been studied. Erionite has been shown to cause pleural fibrosis and produce the same auto-antibodies commonly seen in people with systemic auto-immune diseases (Zebedo et al., 2014). With this evidence of increased negative health effects, it is important to know who is most likely to be exposed to erionite, where it can be found today, and how to test it.
Erionite vs. Asbestos Preparation
With erionite’s similarities to asbestos, it is easy to expect that asbestos analysis methods would be sufficient. However, erionite and asbestos could not be more different. Erionite is very delicate and does not endure well when subjected to asbestos preparation and analysis techniques.
The majority of the erionite samples that have come into our laboratory for testing have been rock and soil, or air samples collected when rock and soil has been disturbed. Rock and soil material must be reduced to a fine powder for analysis by optical microscopy. We performed milling tests using material collected by NDOT from Pine Valley, NV. The material was milled to two nominal sizes: 250 μm and 75 μm. These two milling targets were chosen because the 250 μm target was used for the soil processing at the Libby Environmental Protection Agency (EPA) Superfund Site, and the 75 μm target is referenced in the California Air Resource Board (CARB) Method 435 for Serpentine in Aggregates (CARB, 2017). The results showed that erionite is fragile and extremely susceptible to overgrinding (Figure 1). Once over milled, bundles and fibers are destroyed and broken into non-fibrous particles, and these structures would no longer be countable by an analyst during microscopic examination. An IARC study in 1989 showed this to be the case with erionite from Oregon as well, though no milling size targets were specified (IARC, 1989).
In an effort to remove organics, soils can undergo the same gravimetric reduction as floor tiles and roofing shingles and assist the analyst in fiber identification (EPA, 1993). However, when dealing with erionite in soil, it is extremely vulnerable to this preparation procedure because it interferes with the chemistry that is being measured. When performing quantitative energy dispersive spectrometry (EDS) chemistry for identification, the elemental composition is less than it should be for identification of erionite. For a sample from Reese River, NV, the average charge balance, a measure of chemical reliability, prior to gravimet
Figure 1. Erionite is susceptible to over-grinding and can be shattered into non-fibrous particulate. (a) Erionite from Pine Valley, NV, milled to nominal 250 μm. (b) Erionite from Pine Valley, NV, milled to nominal 75 μm, the target set in the CARB 435 method (CARB, 2017).
ric reduction was 13.72 percent, whereas after gravimetric preparation, the average charge balance was 148.77 percent.
ERIONITE ANALYSIS
In the United States, TEM is the preferred electron microscopy technique for asbestos because one can quickly obtain chemistry, morphology, and diffraction of the fibers under analysis. Even so, that is only true if the sample can withstand the high energy that is being used during analysis. Asbestos, which is known for its thermal stability, can withstand this energy with limited damage, though even asbestos is not completely impervious to damage (Brindley and Zussman, 1957; Martin et al., 2016). Most zeolites are quite sensitive to the electron beam. Once the energy of the electron beam collides with these delicate fibers, they
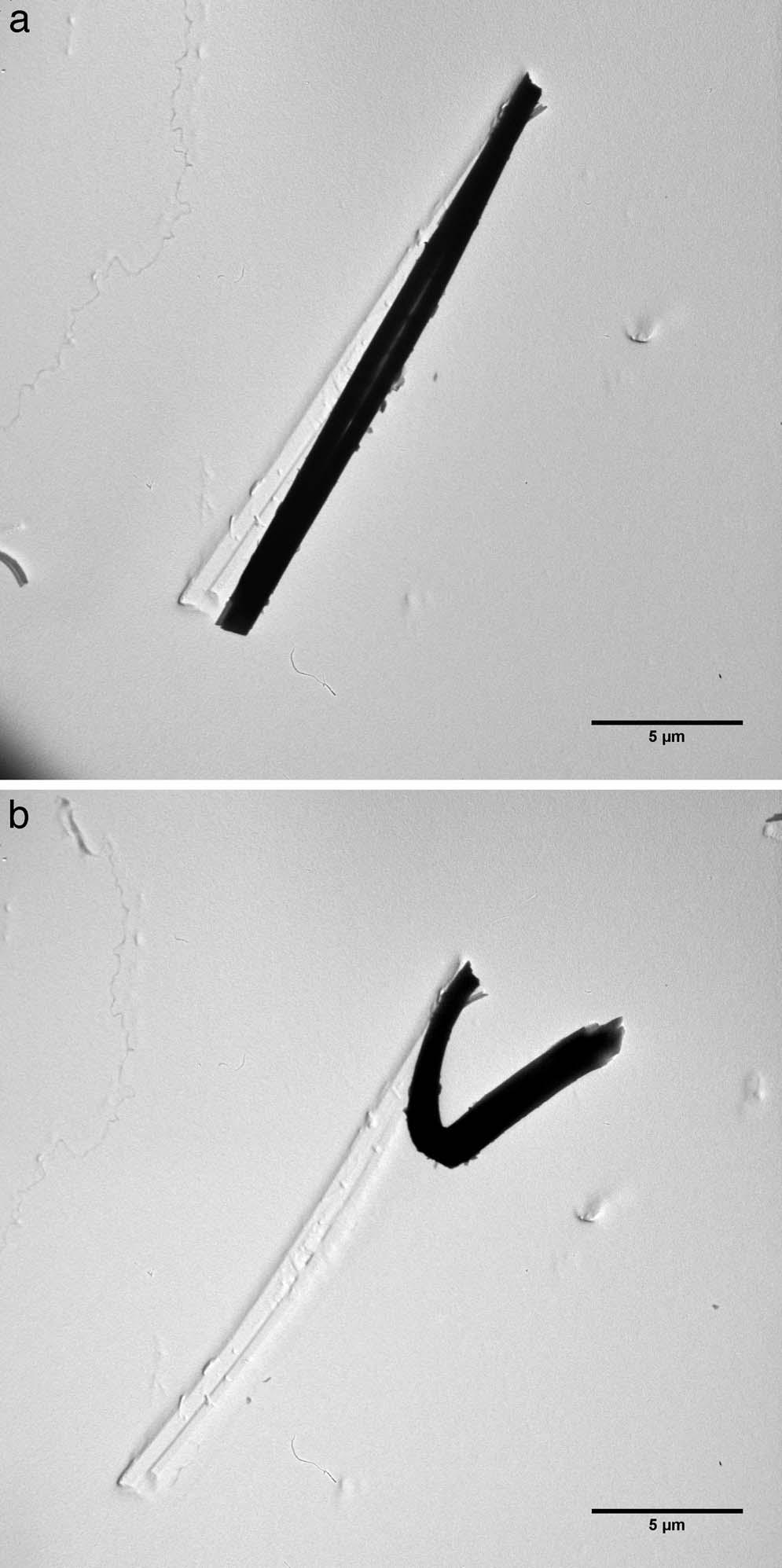
Figure 2. Erionite on a standard TEM holder. (a) The fiber prior to focused beam interaction. (b) Same fiber after focused beam interaction.
deform (Figure 2). The degradation caused by the electron beam also influences the chemistry and crystal structure. In standard TEM analyses of erionite, the selected area electron diffraction (SAED) pattern does not last long enough to be documented and measured for identification. Moreover, once these interactions start, they cannot be stopped. This problem affects quality control and re-analysis because the damaged fiber is no longer available for confirmation during the subsequent analysis.
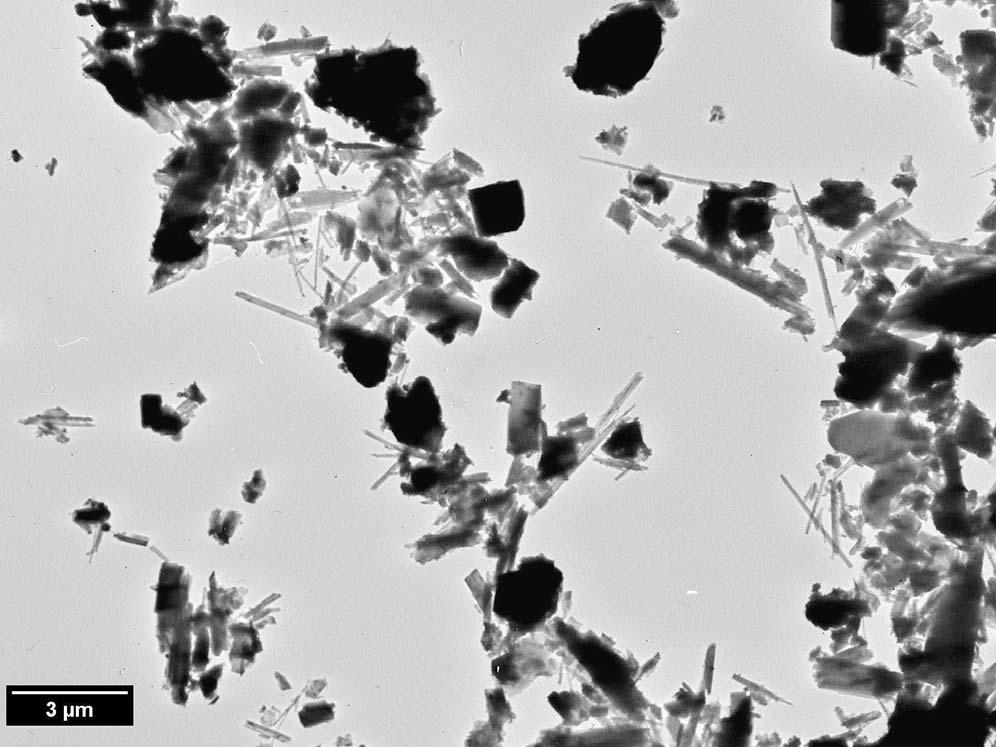
Figure 3. Erionite from Reese River, NV, showing particulate loading of a soil sample under TEM.
The majority of erionite research has focused on using chemistry alone for identification. It is typically measured by inductively coupled plasma mass spectrometry or scanning electron microscopy with an electron microprobe on large rock samples. These techniques are not suitable for individual fibers collected on air samples, so TEM equipped with an EDS system is employed. While TEM EDS is often used in asbestos analysis, it is a technique that can be heavily influenced by particulate and matrix that has also collected on the filter. Due to the hydrous nature of zeolites, when running quantitative elemental chemistry, it is important to verify the reliability of the analysis through the use of the equation for charge balance (E-balance) as described in Passaglia et al. (1998) and Dogan and Dogan (2008):
Charge balance = Al + Fe 3+ − Na + K + 2 × ( Ca + Mg + Sr + Ba ) Na + K + 2 × ( Ca + Mg + Sr + Ba ) ×100. (1)
This test assesses the Al³ + substitution in the T-site and compares it to Al³ + calculated from the measured ionic cations. Ideally, these numbers should balance each other to keep the crystal at a neutral charge. However, the weakness in this equation for TEM EDS is that the equation assumes all the Al measured in the spectrum is substituting into the T-site and is not a contribution from another source, such as a surface particulate. Indeed, Gualtieri et al. (2016) showed that Fe found in some erionite analyses was actually coming from ironbearing nano-particles on the surface of the erionite fibers. On TEM samples, one cannot always overcome high particulate loadings, which can compromise EDS collection (Figure 3).
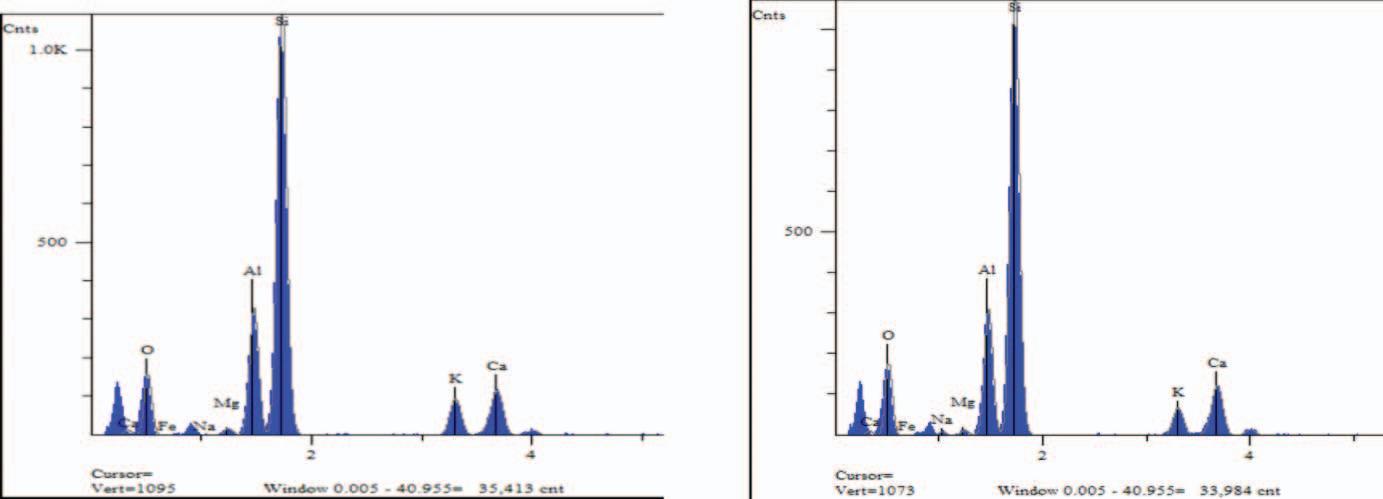
Figure 4. (Left) EDS spectra of offretite. (Right) EDS spectra of erionite from the same sample (AZ).
Another difficulty with EDS arises in the fact that distinguishing between the different zeolites on a fiber by fiber basis is challenging. In Figure 4, we show the EDS spectrum of two different zeolites from the same sample: offretite and erionite. The mineral identification was determined by diffraction; however, one can see the similarity between the two spectra are—they are almost indistinguishable. During EDS analysis, the energy of the beam displaces elements from the structure. A study in 2011 (Carbone et al., 2011), showed just how the beam affected chemistry. In this study, they found that the K counts were reduced by one third during 15 seconds of low-energy beam interaction. Under the much higher energy of TEM, we are “losing” the chemical elements we are trying to measure quantitatively to confirm erionite.
As described above, the problems related to TEM analysis of erionite stem from its interaction with the electron beam, and the key to overcoming these problems is stabilization. Cryogenic electron microscopy holders (referred to as a cold stage) have been shown to help stabilize zeolite fibers in the TEM (Gualtieri et al., 1998). The cryogenic holder is a simple addition to the laboratory protocol; cooling by liquid nitrogen (LN2), it protects the sample from the energy of the electron beam. After employing the cold stage, and applying Cliff Lorimer quantitative calibrations, we saw improved chemistry as demonstrated in the charge balance test. Table 1 shows how, with this technique, more particles pass the chemical reliability (charge balance) test of 20 percent as used by the USGS (USGS, 2010). In a comparison using both a standard (non-cooled) TEM holder and the cold stage, we estimated that on an erionite sample from Rome, OR, we were able to reduce the rate of Na loss from 41 percent to 12 percent. The cold stage cannot overcome all of the limitations of TEM EDS, and the user should still expect that interference from particulate and some cation loss will still be present. Consequently, identification by chemistry alone will remain inconclusive on some fibers.
For our samples, all TEM analysis was conducted on a JEOL 1200 EX II analytical TEM operating at 100 kV. This microscope was outfitted with an AMT Digital camera, IXRF Iridium Ultra Software, and Gatan single-tilt cryo-holder, cooled with liquid nitrogen. The samples were milled to ∼250 μm particles size in a puck mill. To calibrate the EDS system, we used ground Icelandic basaltic glass (BIR-1G) purchased
Table 1. Reese River quantitative chemistry and SAED with cryogenic holder.
Criteria Reference Value 1 2 3 4 5 6 7 8 9 10
Si + Al 36 36.03 36.3 35.77 36.04 35.91 36.06 36.17 36.11 36.43 35.87 TSi 0.68–0.79 0.77 0.77 0.76 0.78 0.77 0.77 0.77 0.77 0.77 0.78 Mg/(Ca + Na) <0.15 0.01 0.03 0.17 0.05 0.02 0.01 0.02 0.02 0.01 0.02 Mg (72 O) <0.8 0.03 0.09 0.36 0.16 0.06 0.03 0.05 0.06 0.03 0.06 Si/Al 2.85–3.6 3.34 3.31 3.25 3.48 3.38 3.43 3.39 3.34 3.36 3.48 Abundant cation Ca, Na, K KKKKKKKKKK Charge balance (%) 2.56 19.3 28.59 9.56 2.92 13.17 11.07 14.55 26.76 8.73 SAED inter-row 15.07 15.1 15.2 15.5 15.3 15.2 15.4 15.3 14.9 15.4 15.4
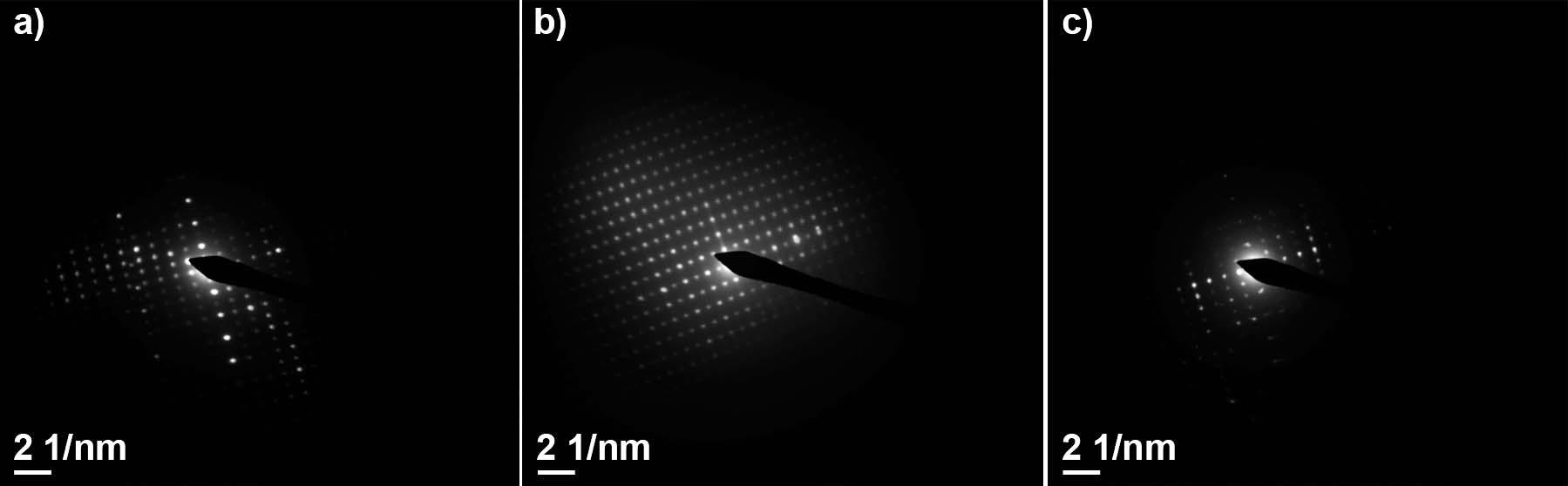
Figure 5. SAED patterns of erionite from different locations showing the ∼15.0 Å layer line spacing taken on a single-tilt cold stage. (a) Killdeer, ND. (b) Rome, OR. (c) Reese River, NV.
from the USGS and Microcline NMNH 143966 from the Smithsonian Institute, which were analyzed under the same conditions used for erionite analysis (tilt of 30 degrees and spot size of ∼160 nm on the Gatan cryoholder). The reference values obtained were used to create calibration files for the IXRF system. These calibration files were then applied to the unknown spectra and analyzed using the Foil Quantitative routine in the software. For erionite, all EDS results were based on 72 oxygen atoms. The camera constant used for indexing diffraction patterns was calibrated from the Au grid from Ted Pella.
Utilizing the cryogenic holder, the biggest difference was seen in the successful recording of diffraction patterns, with most lasting longer than 5 minutes (Figure 5). Unlike analysis with the routine holder, even when chemistry and diffraction analysis had previously been performed, the diffraction results were repeatable during quality-control analysis. This is a worthwhile alternative to having to rely solely on EDS chemistry, since it is easily compromised by surrounding particulates. Electron diffraction (ED) can now also be used to differentiate erionite from other zeolites because the 15 Å layer line spacing of erionite is unique amongst the fibrous zeolites. Therefore, when one is trying to differentiate between offretite, mordenite, and erionite, it can be easily done with ED, because offretite has half of the layer line spacing that erionite does. This is not to say EDS chemistry is not valuable—it is, but having multiple ways to confirm a mineral is necessary for defensible erionite identification (Harper et al., 2017).
We also tested other zeolites from various localities, including erionite from Arizona; and chabazite, offretite, and mordenite from Italy. Using the cold stage, we examined each mineral by chemistry and diffraction and compared them to published reference values. In compiling the data, trends were noted which showed that when the chemistry did not balance, diffraction patterns could still confirm the analysis.
Recommended Approach When Seeking Erionite Analysis
With samples such as soil and stone, it is important to ensure that a representative subsample is collected. Incremental sampling may help in this regard (Interstate Technology and Regulatory Council [ITRC], 2012). When looking for a laboratory to analyze erionite, it is important to use a laboratory that has cryogenic TEM experience and that has demonstrated competency with quantitative EDS analysis.
For analysis, request TEM EPA 600/R-93/116 with the following modifications: Do not gravimetrically reduce the sample or treat with acid. Samples are to be milled to a nominal 250 μm size. Samples are to be analyzed on the TEM cryo-holder or similar cold stage. Fibers confirmed as erionite must meet the following criteria:
1) Diffraction is ∼15 Å, and qualitative chemistry is consistent with erionite. 2) If no diffraction pattern can be achieved, then quantitative chemistry can be used provided the following conditions are met: a. The charge balance formula, as described in Passaglia et al. (1998) is modified to 20 percent as used in USGS (2010). b. Calibrations for Cliff Lorimer K-factors include K. c. Si + Al ∼ 36. d. Mg < 0.8.
An abundance of care and prior experience is needed when sampling, preparing, and analyzing erionite samples. During preparation, we demonstrated how over-milling an erionite sample could lead to false negatives, since erionite loses its elongated shape. Erionite is also sensitive to gravimetric reduction, which can affect quantitative chemistry. Since many zeolites look similar by qualitative chemistry, quantitative chemistry should also be performed. Laboratories will need to acquire additional standards to determine Cliff Lorimer K factors (k-factors) for erionite analysis. Regardless of the sample matrix, cryogenic TEM is the best technique to acquire reproducible and defensible data on erionite.
REFERENCES
Brindley, G. W. and Zussman, J., 1957, A structural study of the thermal transformation of serpentine minerals to forsterite: American Mineralogist, Vol. 42, pp. 461–474. California Air Resources Board (CARB), 2017, Implementation Guidance Document Air Resources Board Test Method 435 Determination of Asbestos Content of Serpentine Aggregate Field Sampling and Laboratory Practices: Electronic document available at https://www.arb.ca.gov/toxics/asbestos/ tm435/workshops/m435-asbestosguidance-2017.pdf Carbone, M.; Baris, Y. I.; Bertino, P.; Brass, B.; Comertpay, S.; Dogan, A. U.; Gaudino, G.; Jube, S.; Kanodia, S.; Partridge, C. R.; Pass, H. I.; Rivera, Z. S.; Steele, I.; Tuncer, M.; Way, S.; Yang, H.; and Miller, A., 2011. Erionite exposure in North Dakota and Turkish villages with mesothelioma: Proceedings of the National Academy of Sciences of the United States of America, Vol. 108, No. 33, pp. 13618–13623. Dogan, A. U. and Dogan, M., 2008, Re-evaluation and reclassification of erionite series minerals: Environmental Geochemistry and Health, Vol. 30, No. 4, pp. 355–366. Dogan, A. U.; Dogan, M.; and Hoskins, J., 2008, Erionite series minerals: Mineralogical and carcinogenic properties: Environmental Geochemistry and Health, Vol. 30, No. 4, pp. 367–381. Environmental Protection Agency (EPA), 1993, Test Method for the Determination of Asbestos in Bulk Building Materials: Environmental Protection Agency Report EPA/600/ R-93/116. Gualtieri, A.; Artioli, G.; Passaglia, E.; Bigi, S.; Viani, A.; and Hanson, J. C., 1998, Crystal structure–crystal chemistry relationships in the zeolites erionite and offretite: American Mineralogist, Vol. 83, No. 5–6, pp. 590–606. doi:10.2138/am-1998- 5-619. Gualtieri, A. F.; Gandolfi, N. B.; Pollastri, S.; Pollok, K.; and Langenhorst, F., 2016, Where is iron in erionite? A multidisciplinary study on fibrous erionite-Na from Jersey (Nevada, USA): Scientific Reports, Vol. 6, pp. 37981. doi:10.1038/srep37981. Harper, M.; Dozier, A.; Chouinard, J.; and Ray, R., 2017, Analysis of erionites from volcaniclastic sedimentary rocks and possible implications for toxicological research: American Mineralogist, Vol. 102, pp. 1718–1726. doi:10.2138/am-2017- 6069. Ilgren, E. B.; Pooley, F. D.; Larragoitia, J. C.; Talamantes, M.; Navarrete, G. L.; Krauss, E.; and Breña, A. F., 2008, First confirmed erionite related mesothelioma in North America: Indoor and Built Environment, Vol. 17, pp. 567–568. International Agency for Research on Cancer (IARC), 1989, Modification of fibrous Oregon erionite and its effects on in vitro activity. In Bignon, J.; Peto, J.; and Saracci, R. (Editors), Non-Occupational Exposure to Mineral Fibers: Scientific Publication 90, IARC, Lyon, France, pp. 74–80. International Agency for Research on Cancer (IARC), 2012, IARC Monograph on the Evaluation of Carcinogenic Risks to Humans. Volume 100C: Arsenic, Metals, Fibres and Dusts: IARC, Lyon, France, 311 p. Interstate Technology & Regulatory Council (ITRC), 2012, Incremental Sampling Methodology: Electronic document, available at https://www.itrcweb.org/ism-1/pdfs/ISM1_021512_Final.pdf Kliment, C.; Clemens, K.; and Oury, T., 2009, North American erionite-associated mesothelioma with pleural plaques and pulmonary fibrosis: A case report: International Journal of Clinical and Experimental Pathology, Vol. 2, No. 4, pp. 407–410. Martin, J.; Beauparlant, M.; Sauvé, S.; and Espérance, G., 2016, On the threshold conditions for electron beam damage of asbestos amosite fibers in the transmission electron microscope (TEM): Journal of Occupational and Environmental Hygiene, Vol. 12, pp. 924–935. National Institute for Occupational Safety and Health (NIOSH), 2014, Health Hazard Evaluation Report: Evaluation of Erionite and Silica Exposure During Dirt Road Maintenance: NIOSH HHE Report No. 2012-0141-3220, U.S. Department of Health and Human Services, Centers for Disease Control and Prevention, National Institute for Occupational Safety and Health, Cincinnati, OH: Electronic document, available at http://www.cdc.gov/niosh/hhe/reports/pdfs/2012-0141- 3220.pdf National Institute of Standards and Technology, 2009, NVLAP TEM Proficiency Test 2009-1 Summary Report: National Institute of Standards and Technology, Boulder, CO. National Toxicology Program (NTP). 2004, Erionite. In Report on Carcinogens, 11th ed.: NTP, Durham, NC, pp. III114–III115. Nevada Department of Transportation (NDOT), 2018, Documenting Naturally Occurring Asbestos and Erionite in Import Material for Nevada Department of Transportation Projects: State of Nevada Department of Transportation Environmental Services Division, Carson City, NV. Passaglia, E.; Artioli, G.; and Gualtieri, A., 1998, Crystal chemistry of the zeolites erionite and offretite: American Mineralogist, Vol. 83, No. 5–6, pp. 577–589. Rom, W.; Casey, K.; Parry, W.; Mjaatvedt, C.; and Moatamed F., 1983, Health implications of natural fibrous zeolites in the Intermountain West: Environmental Research, Vol. 30, No. 1, pp. 1–8. Saracci, R., 2015, Erionite and cancer in a Mexican village: Occupational and Environmental Medicine, Vol. 72, pp. 163–164. U.S. Geological Survey (USGS), 1996, Occurrences of Erionite in Sedimentary Rocks of the Western United States: U.S. Department of the Interior, Denver, Colorado: Electronic document, available at http://pubs.usgs.gov/of/1996/ 0018/report.pdf U.S. Geological Survey (USGS), 2010, Chemical and Morphological Comparison of Erionite from Oregon, North Dakota, and Turkey: U.S. Geological Survey Open-File Report 2010-1286, 8 p.
Van Gosen, B. S.; Blitz, T. A.; Plumlee, G. S.; Meeker, G. P.; and Pierson, M. P., 2013, Geologic occurrences of erionite in the United States: An emerging national public health concern for respiratory disease: Environmental Geochemistry and Health, Vol. 35, No. 4, pp. 419–430. doi:10.1007/s10653-012-9504-9. Zebedeo, C. N.; Davis, C.; Peña, C.; Ng, K. W.; and Pfau, J. C., 2014,. Erionite induces production of autoantibodies and IL-17 in C57BL/6 mice: Toxicology and Applied Pharmacology, Vol. 275, No. 3, pp. 257–264.