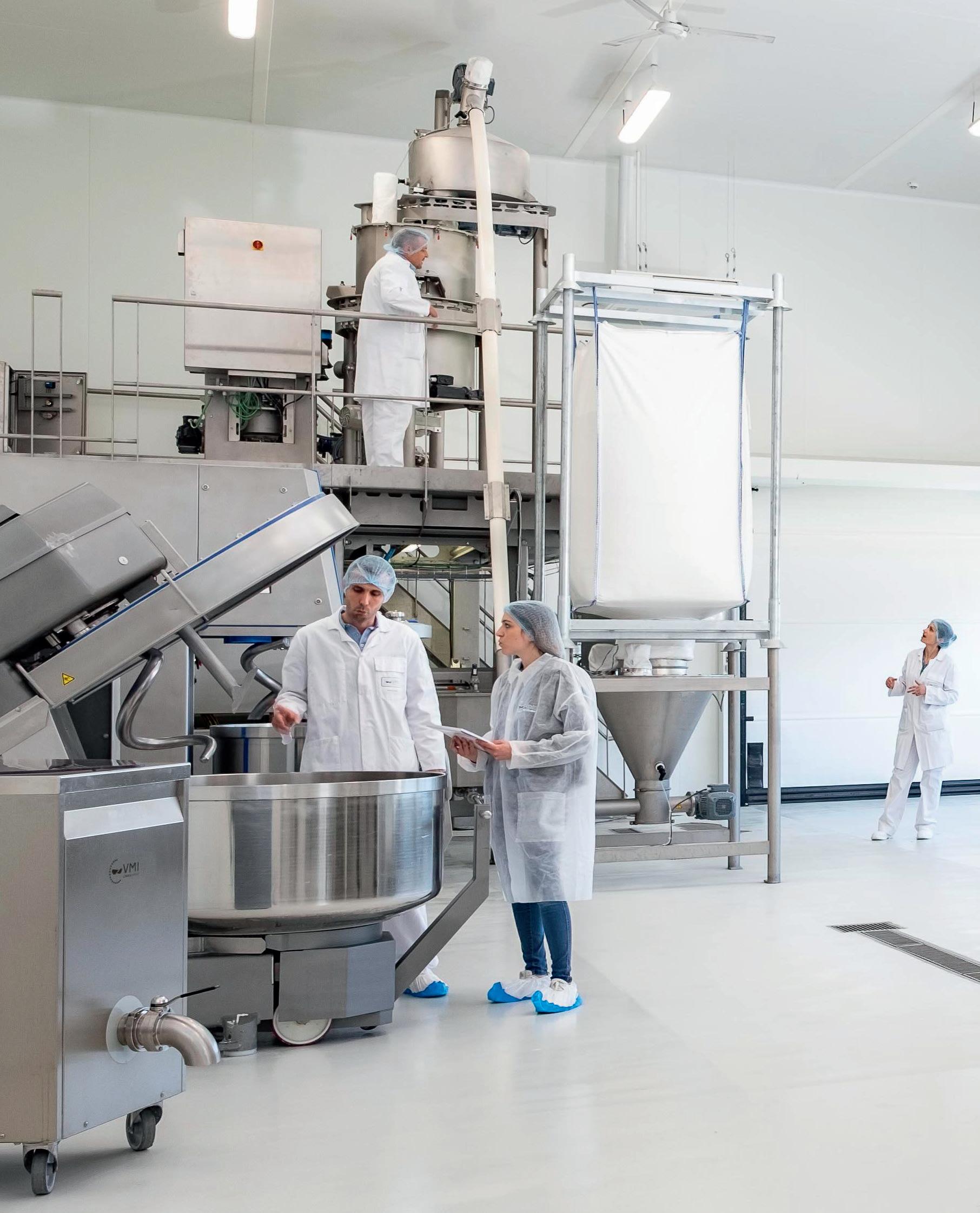
21 minute read
Mixing: Dough mixing supervision: an overview
from f2m Automation Book
by landmagd
Dough mixing supervision: an overview
The key parameters governing the mixing phase, their importance and their effects on the final products are described, as well as examples of strategies for monitoring the mixing process.
+Cereal-based foodstuffs are at the base of the food pyramid. Among them, wheat flour is one of the most important food sources in the world, particularly because of its unique property of forming a dough and developing gluten when mixed with water. The mixing process is the first step towards the final bakery product and it plays a major role in the whole breadmaking process. The quality of the dough obtained, in particular its rheological properties, will have a great impact on the behavior of the dough during shaping, fermentation and baking.
General aspects of dough mixing
Dough mixing is a process in which wheat flour, water, and other ingredients like salt, yeast, sugar, oil, enzymes and emulsifiers are blended together, leading to a series of bio-physical-chemical reactions. As the first step in the breadmaking process, it has a strong impact on the quality of the final product. The mixing operation has three main functions: + the hydration of the flour in order to prepare the formation of a viscoelastic network that will ensure the gas-holding capacity during fermentation and baking (Bloksma, 1990) + the creation of a homogeneous network forming the matrix of the bread dough (Figure 1) + the incorporation of air into the cereal matrix to form the gas cells that will later become the cells of the baked product However, hydration alone does not allow the development of the dough and a certain mechanical energy is necessary to form and to organize the gluten network (Campos et al., 1996). Thus, proper dough development depends on the quality and quantity of ingredients, the mixer geometry, the mixing duration and mixing speed. Any variation in mixing can affect the end-product quality; although it is a decisive step, it is also one which can be most controlled.
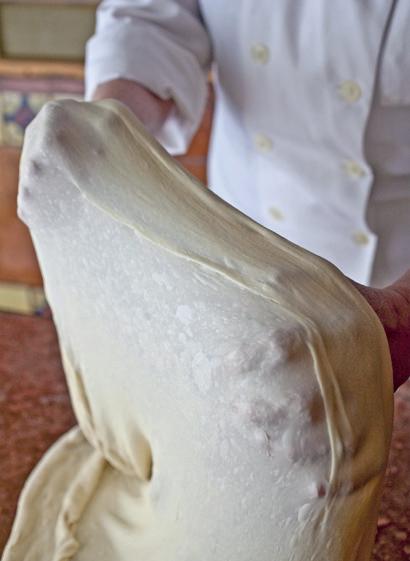
© Erb / Dufault Photo – stock.adobe.com Figure 1: The manipulation of the dough by a baker is a traditional way to assess the degree of dough mixing. Stretching the dough until reaching a thin and film is the usual criteria to decide on the end of mixing in addition to dough compression and dough stickiness assessed through manual contact
DOUGH MIXING SUPERVISION: AN OVERVIEW
Figure 2: Evolution of the power, the Specific Mechanical Energy and the temperature during mixing. The time to peak tPEAK corresponds to the maximum power consumed by the mixer. It is usually located just after the optimal dough development that is determined by an expert baker
Background
Over time, mixing technology has evolved according to the wheat grinds, the types of mixers and the breads consumed by the population. Before the 20th century, the bread consumed was very dense and made from firm dough. Mixing was done by the baker without mechanical aids; walking and pressing the dough with the feet was a common way to carry out the mixing, which was based mainly on compression and partly on shearing. The mixing trough was generally a wooden box with a rounded bottom, closed with a hinged lid. The first attempts to mechanize the mixing process were made between 1760 and 1840, but they were not democratized in bakeries, for fear that machines would replace the work of men. The first mechanical mixing machines tried to imitate man’s movement on the dough, by bringing energy by way of a wheel actuated initially by animals or a crank, which reduced the effort needed, then thereafter by an electric motor.
At the beginning of the 20th century, hygiene standards caused an increase in the number of mechanical mixers in bakeries, particularly with models with a plunging arm. In parallel, bread made from soft dough became the most consumed. Therefore, the mixing process has evolved between conventional, intensified and improved: + Conventional mixing can be defined as slow mixing, corresponding mainly to manual mixing, as practiced before mechanization. + Intensified mixing can be defined as mechanized mixing with formulations using oxidizing agents leading to voluminous breads often presenting a white crumb. + Improved mixing can be defined as a compromise between conventional mixing and intensified mixing. It aims to obtain a better balance between the development of the dough and the preservation of its texture, taste and aromas.
The mixing phases
There are as many ways of mixing bread dough as there are bakers, recipes and mixers. According to Tömösközi and Békés (2015), two conditions must be met in order to achieve the desired breadmaking properties: the formulation of the ingredients must be balanced and the ingredients within the dough must be evenly distributed. The recipe used by the bakers determines the first condition. In order to achieve the second condition, most bakers use a two-stage mixing process, starting with a slow speed and continuing with a faster speed.
Slow speed mixing
The initial phase of mixing is operated at a slow speed; its main purpose is to hydrate the solid ingredients and homogenize the dough. The water ensures the plasticization of the system, promoting molecular mobility. The low speed used and the low resistance of the dough means that the power consumed by the tool to maintain its rotation speed is not very high. The power consumption increases very little. However, this step is marked by a rapid increase in the temperature of the dough (Figure 2). At the end of this step, it is possible to adjust the consistency of the dough by adding water or flour, or depending on the recipe, ingredients such as salt or fat.
High speed mixing
The second step begins with the switch to a higher mixing speed, which is usually called kneading. This step leads to the formation of a three-dimensional developed gluten network,
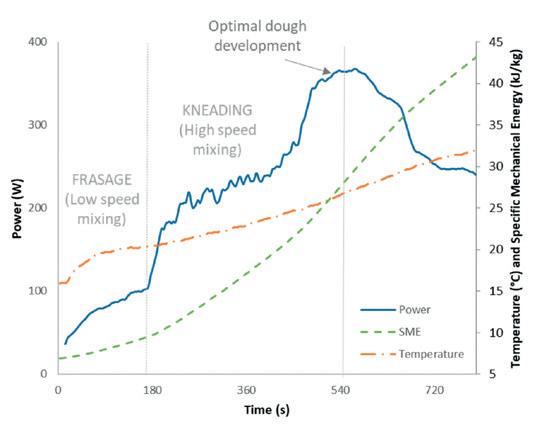
© ONIRIS-GEPEA
which gives the dough its viscoelastic properties and its gas holding capacity. Indeed, the mechanical work is more intense, with physical and biochemical consequences. Kneading imposes a stretching, shearing and extension stress on the dough, modifying to a large extent the interactions between the ingredients. Molecular interactions within the dough can be of low energy (hydrophobic, hydrogen bonds) or generate chemical reactions (formation/breakage of covalent bonds), the latter being most often catalyzed by enzymes. With the strengthening of its gluten network, there is an increase in the dough’s capacity to retain the gases incorporated by the tool during mixing.
During the kneading process, the consistency of the dough reaches a maximum (Figure 2). The time to obtain this consistency is called the ‘optimum dough development time’ or ‘time to peak’ indicated as tPEAK (Sadot et al., 2017). This time does not necessarily correspond to the kneading time to get the best rheological properties of the dough, but is usually very close to it. To successfully knead dough to its maximum level of development, a minimum critical speed must be achieved. Above this value, any speed can be used for kneading; but, it has to be kept in mind that increasing the speed of the mixer leads to an increase in the maximum torque supplied by the motor. The integration over time of the power consumed by the mixer yields the Specific Mechanical Energy (SME) in J/kg of dough, or often determined in kWh/kg dough. Keeping the SME constant is often considered in the industry to adjust the mixing time. Another approach consists in counting the number of tool revolutions. It was observed by Sadot et al. (2017) that the SME is proportional to the number of tool revolutions in a spiral mixer. Therefore, the total kneading time must be decreased if the rotation speed is increased in order to obtain the same SME and possibly the same number of tool revolutions to reach the maximum torque (Chin and Campbell, 2005). However, using too high a speed can lead to a deterioration in bread quality, especially crumb texture (Kilborn and Tipples, 1972). Beyond the optimum kneading time, the dough weakens, collapses and becomes sticky; the dough qualifies as overmixed. Dough undergoing a lamination process (sheeted dough like puff dough) is often undermixed to prevent an excess of elasticity (springiness), which may address difficulties during sheeting (lack of plasticity).
The mechanics of mixing
A distinction can be made between batch mixers, generally consisting of one or more tools rotating in a bowl, which are more suited to craft bakers and medium-size industry manufacturers, and continuous mixers, which can produce several tonnes per hour and which are rather adapted to large industry. The geometry of mixers may vary but is always based on the same principle of supplying energy to the dough being formed by the movement of the tool. The capacity to provide the necessary amount of energy in a given time is one of the criteria to be taken into account when choosing a mixer.
The different types of batch mixers
The four most popular mixers in the European craft industry are plunger arm mixers, fork mixers, vertical (spiral tool) and horizontal kneaders. For a baker, the choice of a mixer will depend on the type of dough they want to obtain.
Plunge arm mixers The plunge arm mixer is historically the most recognized but probably the least used today. In order to imitate the mixing movements of a baker’s arms, two linked arms exert a symmetrical action so that the end of each arm folds the ingredients from the center to the outside of the bowl during mixing. As the dough begins to form, this movement lifts it, stretches and folds it, then the rotation of the bowl brings it back towards the tools. Unlike other mixers, such a mixer has only one mixing speed and the mixing action is only slight against the bowl walls. The main advantages are that the cycloidal kneading movement consumes little energy, yielding in a moderate dough warm-up during mixing. The work is mainly done in extension and shearing, which induces a blowing effect that
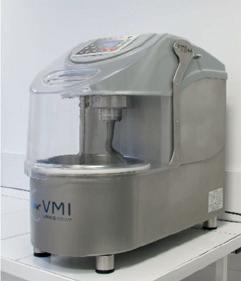
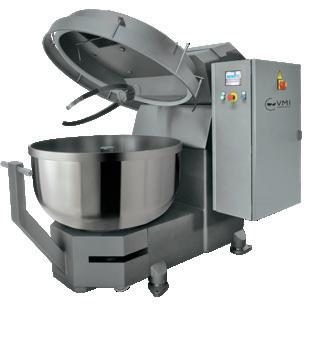
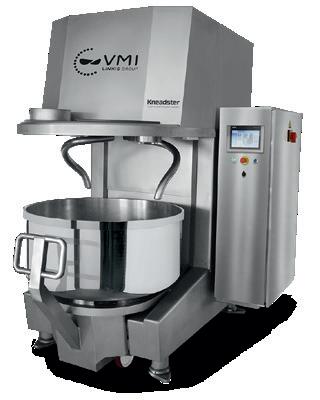
Figure 3: From left to right, Spiral mixer (5kg capacity), Fork mixer, Kneadster mixer with double tools; Bottom: Automated mixing systems with suspended shuttle for high capacity lines
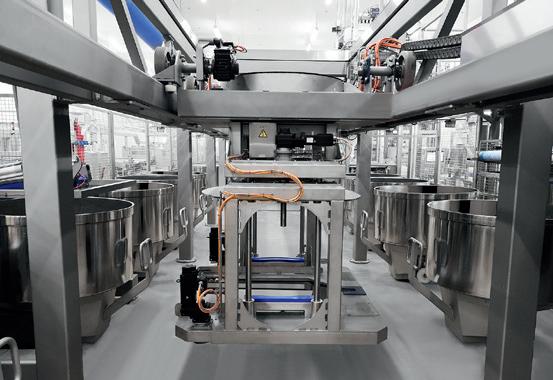
contributes to the formation of large air pockets in the dough. Such a mixer is adapted for breadmaking and panettone-type cakes. It is also an expensive and a fairly low productivity piece of equipment.
Fork mixers Fork mixers have generated the reputation of French bread throughout the world. This mixer’s tool is fork-shaped with profiled ends and is mounted at a given angle to the bowl’s axis. The center of the bowl usually has a central pivot so that the action of the tool acts between the pivot and the bowl’s wall. This mixer exerts a shearing and extension action on the dough. The initial mixing is achieved by folding the ingredients over each other and then the mixing is done by pinching the oblique axis between the bowl’s wall and the tool. The profiled ends of the tool act like a ‘plow blade’ to bring the dough between the forks of the tool into the mixing area. In this type of movement, the dough does not move continuously, but is rather pushed with each turn of the bowl.
Vertical kneaders A general definition of this type of mixer would be a mixing machine equipped with a spiralshaped mixing tool, having a rotary movement along a vertical axis against the inner circumference of a bowl, which also rotates around a vertical axis. Several geometries exist for these mixers; some manufacturers offer a very wide tool that sweeps an area larger than the radius of the bowl in order to eliminate any areas where the dough is not mixed, especially in the center of the bowl. Others use a central pivot which has an effect on dough development. A very wide center
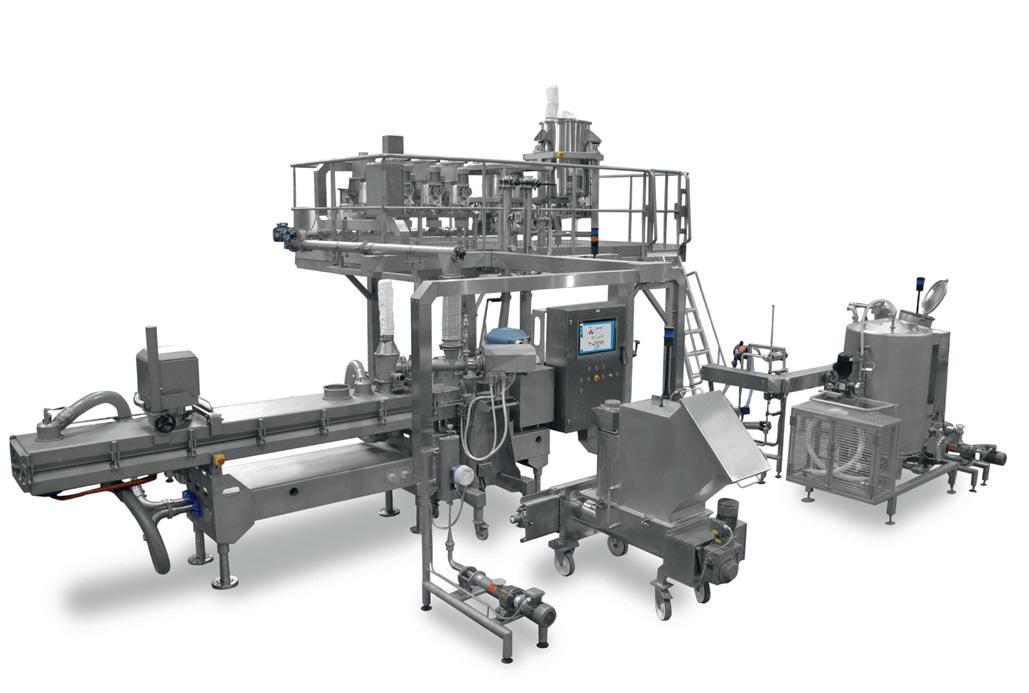
Figure 4: Continuous Mixer installation
© VMI
pivot will increase the effective kneading area, while a blade-shaped pivot can be used to create a shear zone between it and the spiral tool. A double mixing tool can also be proposed and enables slower kneading. Each geometry has advantages and disadvantages in terms of the homogeneity of the kneading, the structure of the dough, the time to achieve optimum kneading and the energy used to achieve it. This kind of mixer is the most efficient equipment for the production of bread dough, Viennese pastry, brioches and pizza with loads ranging from 80 to 900 kg.
Horizontal kneaders Horizontal mixers are widely used for doughs that need to develop a gluten network, such as cracker mixers and hamburger buns. The tools are mounted horizontally in a U-shaped bowl that can discharge the dough after kneading into a hopper. Several tool geometries are available for this type of mixer: Z, sigma or paddleshaped with scrapers at the ends (Niranjan et al., 1994).
Automated batch kneading This involves ensuring continuous dough production from mixers working in a discontinuous manner: the mixing bowls are moved automatically (linear bowl transport system, for example) from one station to another corresponding to the various stages of mixing: introduction of ingredients, mixing, breaking, delayed incorporation of ingredients, etc.
Continuous mixers
This type of installation has become particularly important with the development of large-capacity industries. In contrast to batch mixing, the dough
moves through a mixing line in which the phases of mixing take place: dosing of the powders (flour, salt, improvers), dosing of the liquids (water, leavening, poolish or other possible liquids), premixing and kneading. The dough falls from the pre-mixer into the kneading trough, the latter being designed in such a way that the dough moves from one end of the trough to the other (driven by the rotor blades) while being mixed (Figure 4). Continuous kneading allows an increase in the production rate, a reorientation of production towards products with a long shelf life, and the expansion of distribution areas.
Factors influencing mixing
Some key parameters can significantly improve the kneading process and its effect on the quality of the final product. Indeed, the SME, flour composition, dough temperature, water content, kneading time and mixing speed must be carefully controlled.
Energy
Mixing involves an intense input of mechanical work through the movement of the dough mixer arm. The force varies according to the geometry of the mixer (shape of the arm and action on the dough), the mass of dough and the properties of the dough (adhesion to the bowl and arm, viscous and elastic resistance). Only a part of the energy supplied by the moving arm is absorbed by the dough and converted to heat resulting from viscous dissipation, causing the dough to warm up. Some of this absorbed energy is stored via the formation of molecular structures (Belton, 2005), while some is converted into thermal energy by viscous dissipation. The thermal energy returned to the dough by viscous dissipation is measured from its temperature rise during kneading (Amjid et al., 2013; Contamine et al., 1995). Some of this energy is exchanged with the mixer bowl and is evacuated to the outside. The same SME input will not necessarily give the same effective work for different materials (type of deformation applied), different conditions of use (speed, duration) or different doughs (consistency, composition). The more resistant a dough is, the greater the force required to deform it, and the greater the power consumed by the mixer. Therefore, the mixing time will be shortened.
Flour composition
The composition of the flour and particularly its protein content is a significant factor determining the final quality of the bread. The higher the protein content, the greater the dough’s ability to trap and hold carbon dioxide and the greater the volume of the bread can be. The quality of the protein and the amount of damaged starch also have an impact on the quality of the dough. Indeed, damaged starch granules absorb more water than undamaged ones. It was found that a strong flour, containing more gluten, takes longer to reach the peak consistency (Frazier et al., 1975).
Water
Water is a mobility enhancer. As the water content in the dough increases, the elastic properties and viscosity of the dough are reduced. A moisture content below 44% does not allow for optimal gluten formation. A moisture content between 44 and 50% does not modify the structure of the dough but has a plasticization effect. The addition of electrolytes can also change the nature and intensity of hydrophobic interactions between the gluten proteins. For example, the addition of salt increases ionic strength and reduces the ability of the proteins to retain water.
Temperature
Temperature is a process condition that affects physical properties. Dough temperature depends on the temperature of the raw materials, the mechanical energy absorbed during mixing, and the energy exchanged with the mixer bowl. The solubilization and swelling processes also generate heat due to the exothermic hydration reactions. Controlling dough temperature is perhaps the most important parameter that can affect the final consistency of the dough during mixing, regardless of the mixing process. In general, doughs processed at lower temperatures give better dough properties and final bread quality than those
processed at higher temperatures. A final temperature of about 30°C is generally recommended to obtain doughs with optimal rheological properties for further processing. Such a temperature also matches with the range of temperature used for fermentation.
Kneading speed and kneading time
Increasing the kneading speed results in a decrease in the time required to achieve optimal dough development (Auger, 2008; Muchová and Žitný, 2010). According to Kilborn and Tipples (1972), mixing is more efficient at higher speeds, as more work is delivered to the dough for each tool rotation. However, working above a maximum mixing time causes overdevelopment and disruption of the dough structure. On the contrary, there is a critical minimum kneading speed below which the dough consistency does not change during mixing. The viscoelastic properties of the dough do not develop, and the final product obtained from such doughs is inconsistent and has a low volume. Below this critical speed, increasing the energy input, i.e. increasing the kneading time, improves the qualities of the product, but without ever reaching those of a product obtained from doughs kneaded above this critical speed.
Mixing monitoring
Real-time detection techniques that do not require manual sampling are of particular interest because of their automatic data acquisition capability. They can therefore be used in control systems to automate processes and improve product quality by monitoring certain critical parameters.
Power monitoring
Power and torque measurements are simple and inexpensive techniques used to determine in real-time the force required to drive the tool (i.e., spiral in a spiral mixer). The power input is simpler to measure because no equipment needs to be installed inside the bowl. The power input is measured based on the electrical consumption of the motor driving the mixer. During mixing, the power required to maintain a constant tool speed increases to a maximum (Figure 2). The mixing must therefore be stopped at a fixed energy input, chosen according to a particular rheological state of the dough.
Temperature monitoring
Mixers are usually equipped with a conventional thermocouple or possibly touchless infrared temperature sensors. When the temperature of the dough is measured in real-time and the energy loss of the mixer is the same for each mixing batch, the temperature at the end of mixing can be used as a control mechanism.
Spectroscopic monitoring
Just as in milling, where the application of NearInfrared Spectroscopy (NIRS) is widespread for monitoring the composition of raw materials and finished products, the trend of using the benefits of spectroscopic analysis techniques is also increasing in the baking industry, particularly for the continuous monitoring of the dough development process in the mixer. Spectroscopic techniques are based on the light/matter interactions and thus have the potential to provide a non-invasive means of probing the chemical changes that occur during dough development. As the wavelengths of the absorbed and emitted radiation depend on the chemical composition and the intensity depends on the concentration,
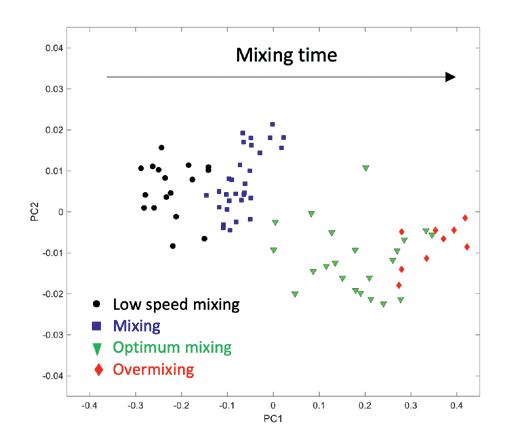
Figure 5: PCA analysis of NIR spectra obtained during mixing
© ONIRIS-GEPEA
they allow for both qualitative and quantitative multi-component chemical identification (water, starch, protein and fat). The measuring probes are generally in a reflectance mode and are placed directly in contact with the dough or possibly in front of a viewing window on the bowl. Noncontact probes exist but are very expensive. NIRS is particularly sensitive to O-H, C-H and N-H bonds, making this technique ideally suited to low moisture content measurements. But it has broad absorption bands that are quite difficult to interpret. The processing of spectra requires the help of chemometric analysis (Aït Kaddour and Cuq, 2011). Chemometrics is the combination of statistics and chemical knowledge to obtain information from chemical systems. Common chemometric methods include Principal Component Analysis (PCA) and Partial Least Squares (PLS) regression. PCA applied to the NIR spectra during dough mixing makes it possible to follow the evolution of hydrogen bonds and the gluten network development (Figure 5).
Acoustic monitoring
The use of ultrasonic techniques has also raised some interest in assessing the rheological properties of dough. The speed of sound can be measured by the time of flight of the sound wave, which depends on the density and compressibility of the material. The attenuation of the sound wave is the loss of energy as it travels through the medium due to viscous losses. As the speed of ultrasound is highly sensitive to the presence of air, it can be used to measure the aeration of the dough during mixing. Depending on the frequency used, it is possible to study the distribution of bubbles within the dough during mixing (Leroy et al., 2015) or the porosity of the dough (Petrauskas, 2010).
Conclusions
This article aimed to provide a general understanding of the mixing process and its relevance in the breadmaking process, the different stages of dough mixing, the main pieces of equipment
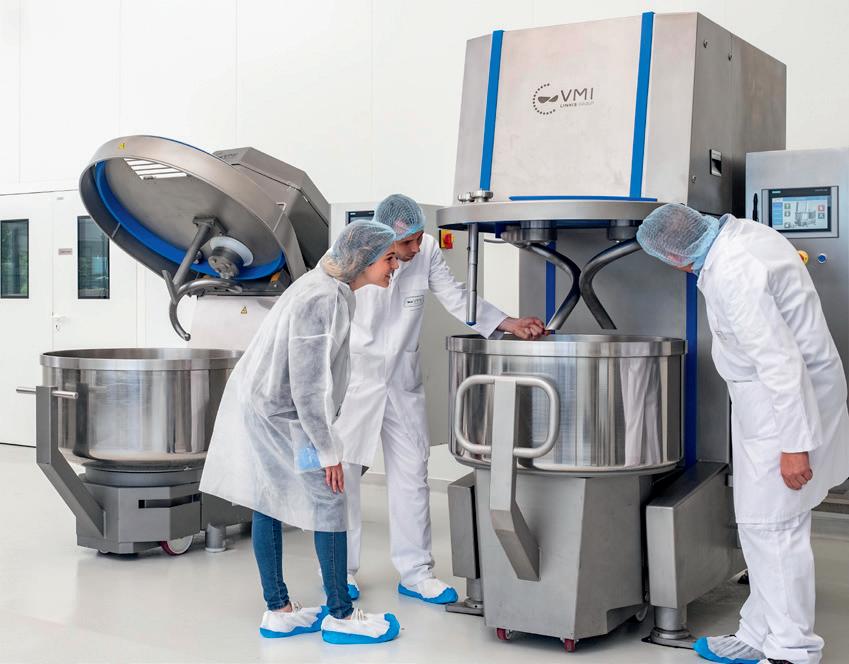
and their applications, the key parameters influencing the process and how real-time monitoring can help to achieve the best quality and determine the end of the process. Indeed, a good knowledge of these key parameters and the choice of the best kneading machine can improve the process and the quality of the final product. +++
Acknowledgments This project was funded by the ANR project “MIXILAB” (n°15-LCV3-0006-01) and by ONIRIS-GEPEA.
Authors
Eloïse Lancelota, Dominique Della-Vallea , Joran Fontainea,b, Adrien Rebillarda,b , Anthony OGEa, José Cheiob, Alain Le-Baila
aONIRIS, UMR CNRS GEPEA 6144, 44300 Nantes France bVMI, 85224 St Hilaire de Loulay, France
Declaration of Competing Interest The authors declare that they have no known competing financial interests or personal relationships that could have influenced the work reported in this paper.
References
Aït Kaddour, A., Cuq, B., 2011. Dynamic NIR spectroscopy to monitor wheat product processing: A short review.
Am. J. Food Technol. https://doi.org/10.3923/ ajft.2011.186.196 Amjid, M.R., Shehzad, A., Hussain, S., Shabbir, M.A., Khan, M.R.,
Shoaib, M., 2013. A comprehensive review on wheat flour dough rheology.
Pakistan J. Food Sci. 23, 105–123. https://doi.org/10.1051/ epjconf/20135605004 Auger, F., 2008. Etude des mécanismes d’ agglomération du gluten au cours du pétrissage de suspensions farine-eau. Belton, P.S., 2005. New approaches to study the molecular basis of the mechanical properties of gluten.
J. Cereal Sci. 41, 203–211. https://doi.org/10.1016/j. jcs.2004.06.003 Bloksma, A.H., 1990. Rheology of the Breadmaking Process.
Am. Assoc. Cereal Chem. 35, 228–236. Campos, D.T., Steffe, J.F., Ng, P.K.W., 1996. Mixing wheat flour and ice to form undeveloped dough.
Cereal Chem. 73, 105–107. Chin, N.L., Campbell, G.M., 2005. Dough aeration and rheology :
Part 1 . Effects of mixing speed and headspace pressure on mechanical development. J. Sci. Food Agric. 2184–2193. https://doi.org/10.1002/ jsfa.2236 Contamine, a. S., Abecassis, J., Morel, M.-H., Vergnes, B., Verel, a., 1995. Effect of mixing conditions on the quality of dough and biscuits.
Cereal Chem. Frazier, P.J., Daniels, N.W.R., Russell Eggitt, P.W., 1975. Rheology and the continuous breadmaking process.
Cereal Chem. 52. Kilborn, R.H., Tipples, K.H., 1972. Factors affecting mechanical dough development. I. Effect of mixing intensity and work input.
Cereal Chem. 49, 34–47. Leroy, V., Fan, Y., Strybulevych, A.L., Bellido, G.G., Page, J.H.,
Scanlon, M.G., Melorose, J., Perroy, R., Careas, S., 2015.
Investigating the bubble size distribution in dough using ultrasound. Statew. Agric. L. Use Baseline 2015 1, 1–11. https://doi.org/10.1017/CBO9781107415324.004 Muchová, Z., Žitný, B., 2010. New approach to the study of dough mixing processes.
Czech J. Food Sci. 28, 94–107. https://doi. org/10.17221/91/2009-cjfs Niranjan, K., Smith, D.L.O., Rielly, C.D., Lindley, J.A., Phillips,
V.R., 1994. Mixing Processes for Agricultural and Food
Materials: Part 5, Review of Mixer Types. J. Agric. Eng. Res. https://doi.org/10.1006/jaer.1994.1072 Petrauskas, A., 2010. The application of the ultrasonic method for evaluating the porosity of bread.
Ultragarsas (Ultrasound) 65, 20–27. Sadot, M., Cheio, J., Le-Bail, A., 2017. Impact on dough aeration of pressure change during mixing.
J. Food Eng. 195, 150–157. https://doi.org/10.1016/j.jfoodeng.2016.09.008 Tömösközi, S., Békés, F., 2015. Bread: Dough Mixing and Testing
Operations.
Encycl. Food Heal. 490–499. https://doi.org/10.1016/B9780-12-384947-2.00086-6