
12 minute read
Electrifying The Mining Industry
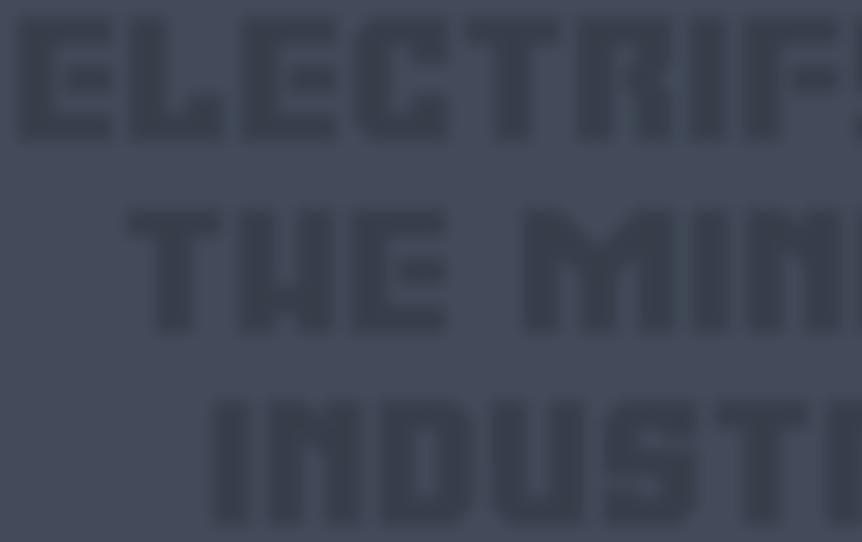
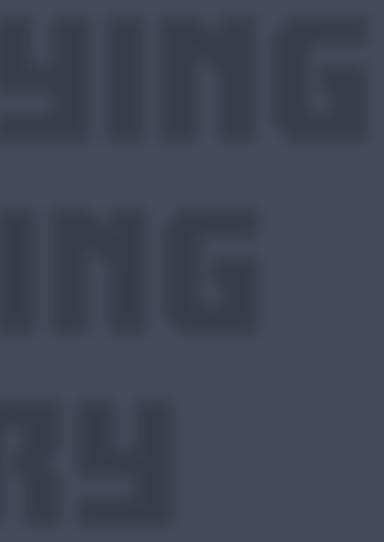
Dr Barry Flannery, Xerotech, Ireland, provides an insider’s perspective on the fundamentals of electrifying a typical piece of heavy equipment for the mining industry.
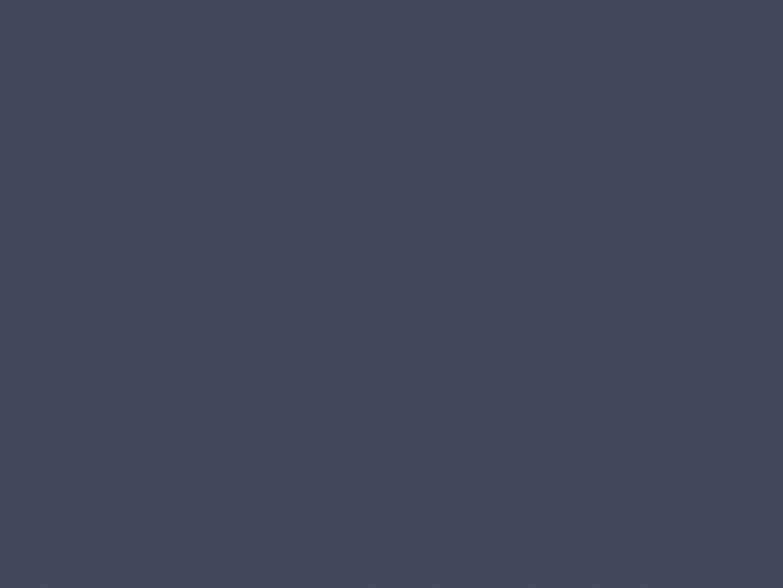
It is an open secret in the mining industry today that virtually every original equipment manufacturer (OEM) in the world has an active electrification programme. Global trends towards sustainable and zero-emission equipment are creating tremendous pressure on manufacturers to deliver battery electric or plug-in machinery. In-market research indicates that leadership at major OEMs are forecasting up to 50% mine equipment electrification by 2025, and near-full electrification by 2030.
Eliminating emissions from underground equipment can reduce mine OPEX by as much as 15 – 20% per year through ventilation system electricity savings. Furthermore, the benefits of enhanced miner safety and air quality improvements in the underground working environment cannot be understated.
Despite these obvious benefits, a new market pull has emerged in recent months with mine operators specifically mandating all-electric equipment for new mine developments. This eff ective ban on diesel equipment has caused many OEMs to scramble for solutions from a supply chain that is at best unprepared, and at worst non-existent.
This article is an insider’s guide to the fundamentals of electrifying a typical piece of heavy equipment, with particular emphasis on: the high-voltage battery,
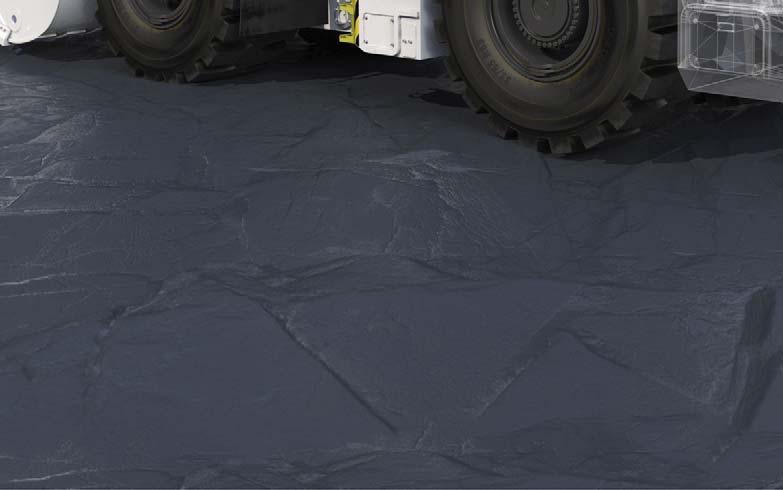
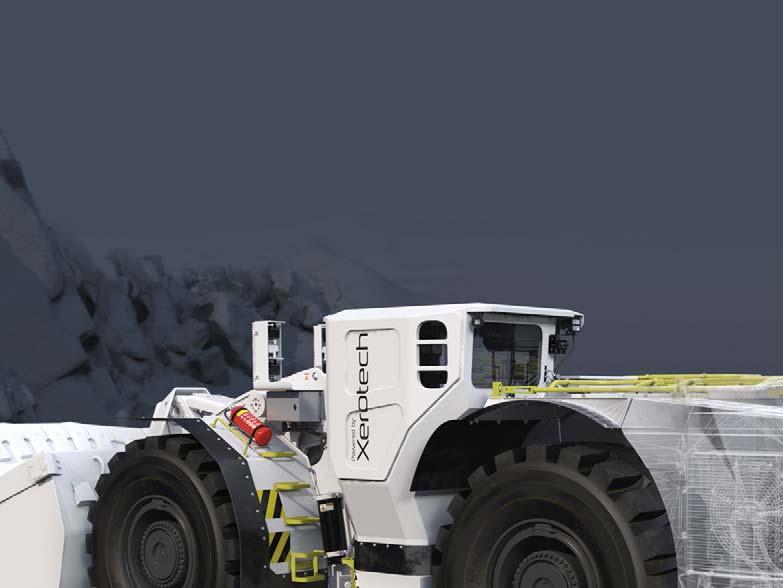
the common challenges that an OEM is likely to face, and suggestions on how to overcome them.
Ditching the diesel engine
One of the greatest challenges OEMs face on their electrification journey today is a lack of experience and familiarity with electrified power systems. Diesel engines are eff ectively a commodity product today. The only diff erentiation is usually supply chain considerations, regional support, and engine emission legislative compliance. Increasingly more stringent emission laws have typically resulted in more expensive and complex engines, but existing Tier-1 engine suppliers have generally had a solution.
The disruption that is happening today is due to the shift from stricter emissions to no emissions. This has left most of the engine supply chain (and OEMs) without a clear solution for their customers. For the past century, the mining industry has built itself on one key assumption – that a diesel engine would be available from Tier-1 engine suppliers in any size and power it needs for a given piece of mining equipment. This assumption is now being tested.
A major gap in low-volume high-diversity markets like mining is that there are no readily available platform solutions. Of course, there are battery pack manufacturers who will manufacture a system to an OEM’s specification, but this usually requires significant time, non-recurring engineering, tooling, and manufacturing charges. In layman’s terms, the battery supply chain is currently geared for high-volume automotive applications like cars, buses, and trucks, and not low volume markets like mining.
The automotive industry is fixated with cost per kWh, and unfortunately, some of this mindset has trickled into the mining industry, creating significant confusion and distorting the realities of operating in this market. It is widely reported that major automotive OEMs have battery pack costs in the region of US$100 – US$150/kWh today. A typical price in low volume markets like mining could be US$300 – US$1000/kWh depending on the configuration of the battery, but this is not the complete economic picture.
Separate to the cost per kWh, but arguably more important, is the overall programme cost. This is oft en overlooked by mining equipment OEMs who are more concerned with battery prices. Automotive has the luxury of being able to amortise multi-million and even multi-billion-dollar development programme costs over millions of units. This is fundamentally diff erent to the mining industry where even the largest OEMs only build a few thousand machines a year (all of which are usually very diverse). This can significantly add to the ‘eff ective price per kWh’ for very low volume applications. The only viable solution for low-volume markets is standardisation or platform approaches to electrification. It is not economic to develop ‘silver bullet’ solutions for every individual product line. Instead, modular and scalable architectures using standard components and high commonality across the OEM’s product portfolio is necessary. Highly scalable battery platforms are a new concept that have recently become available to the mining industry, with the aim of off ering a ‘catalogue’ of battery solutions to OEMs without significant design and development costs or lead-times. Figure 1 shows the major elements of a diesel powertrain and their corresponding electrified equivalents. It is important to note that ‘going electric’ requires far more than identifying a battery supplier. In fact, in most cases, the OEM will need to seek out multiple diff erent suppliers; a battery manufacturer, a motor drive and traction inverter manufacturer (oft en sold matched together), an onboard charger and DC-DC converter supplier, and finally a thermal system manufacturer.
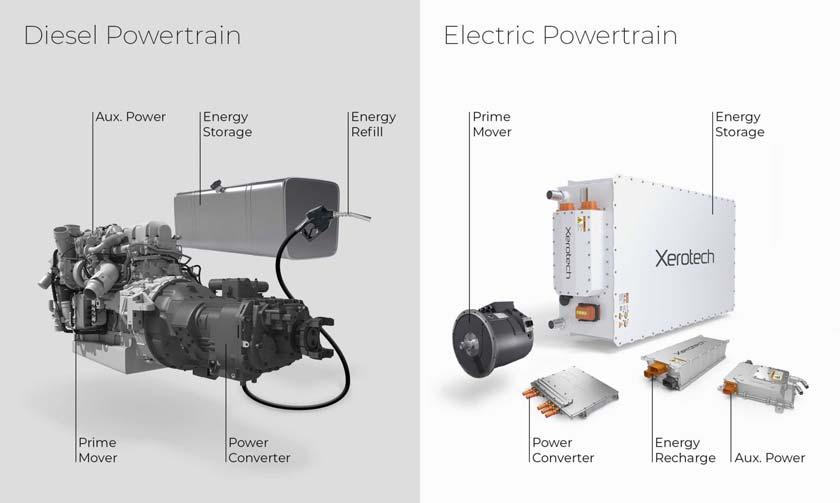
Figure 1. The major elements of a diesel powertrain and their corresponding electrified equivalents. Figure 2. Battery module featuring 2170 cylindrical cells, sidewall thermal management and passive propagation resistant safety technology.
Choosing a battery chemistry
Before even attempting to choose a battery pack manufacturer, it is important to understand the terminology. A battery pack is made up of battery modules, which in turn
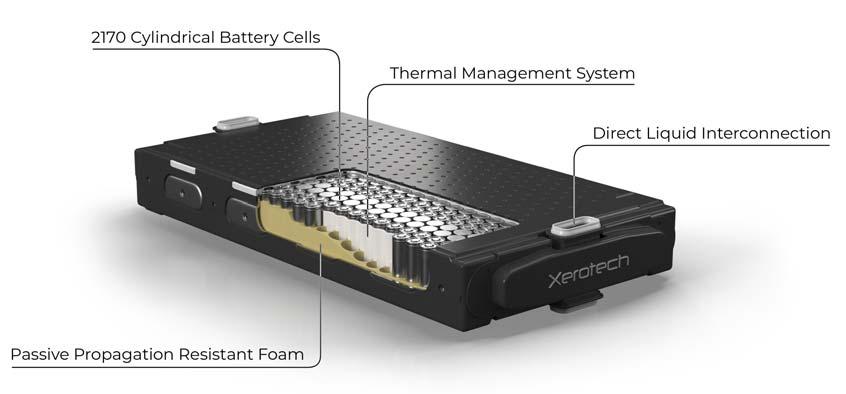
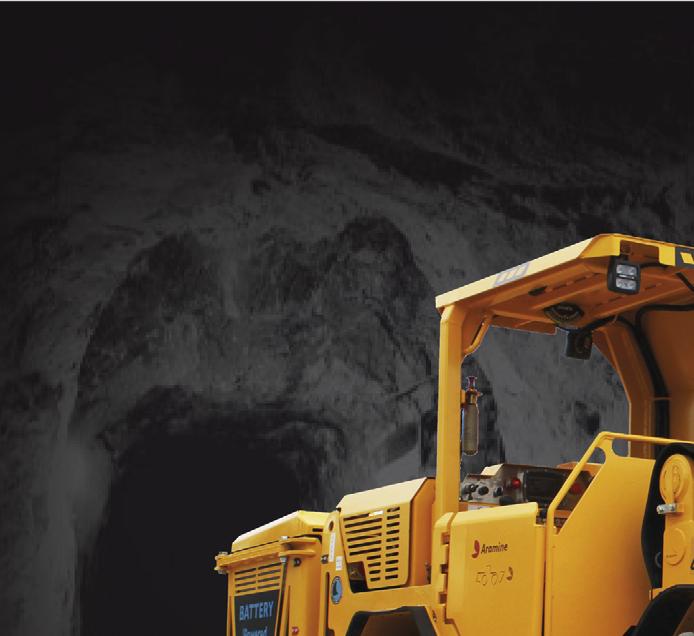
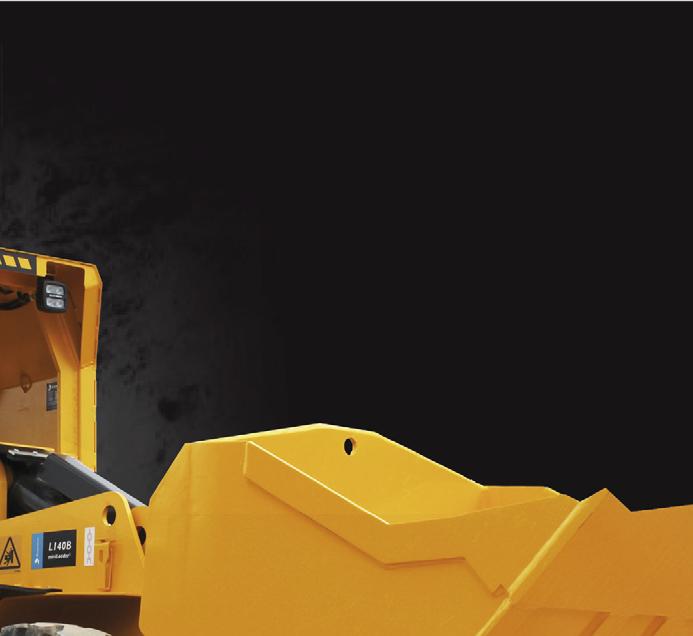

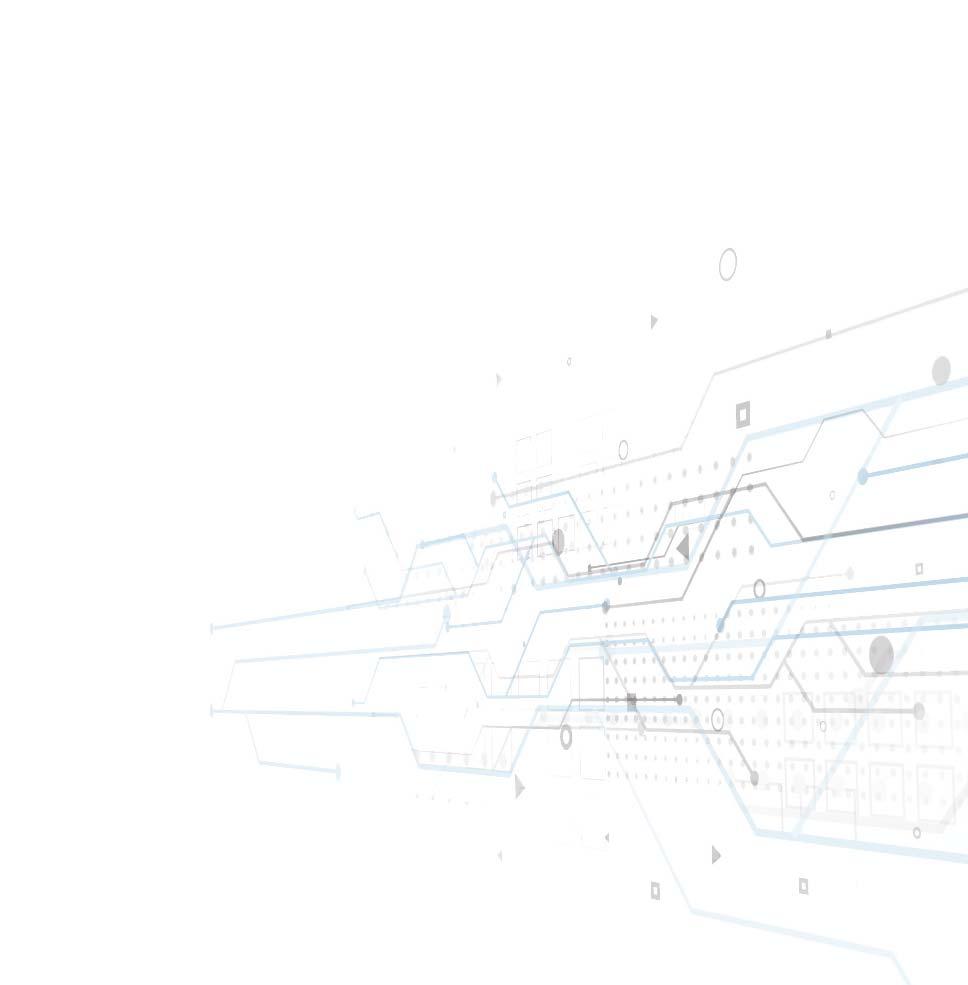
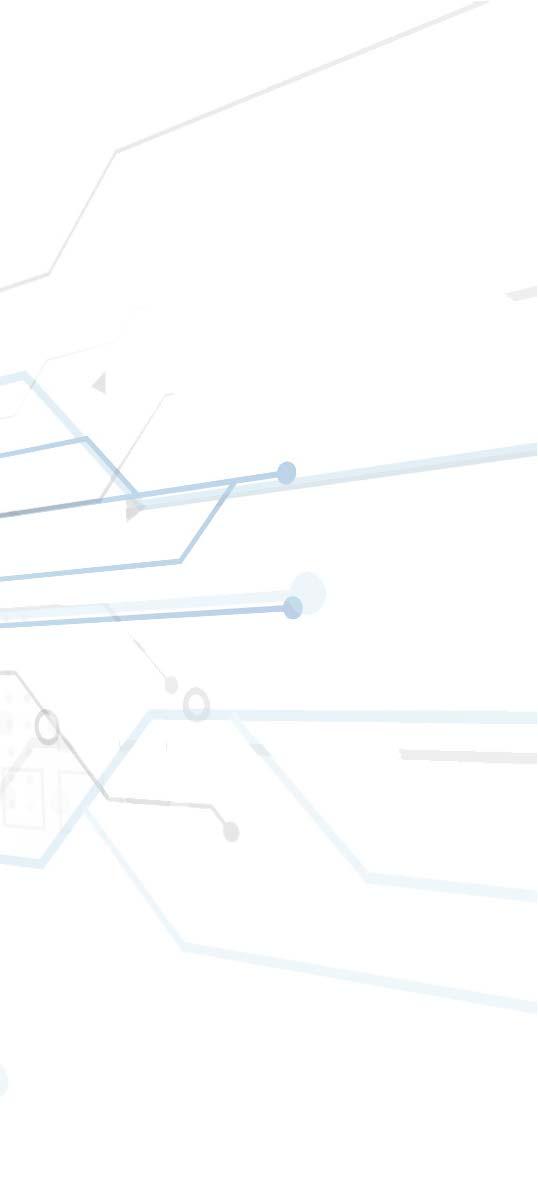
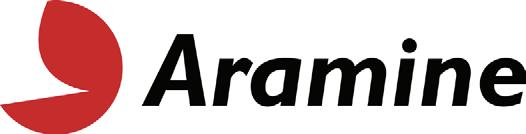
are made up of individual battery cells. A mining vehicle may have one or more battery packs comprising tens of modules and thousands of cells.
Typically, lithium-ion battery technology is used. A key point to understand is that lithium-ion is a family of technologies and not one single thing. The most popular general chemistries are defined by their cathode materials: nickel-cobalt aluminium (NCA), nickel cobalt manganese (NCM), or lithium-iron phosphate (LFP). Within these families, there are many variations (silicon anodes, high nickel, etc.). Building a lithium-ion cell is like baking a cake; there are several key ingredients and adjusting their proportions can change performance characteristics significantly (energy, power, and lifetime).
Diff erent chemistries are used for diff erent use-cases. NCA is the highest energy density and most expensive. An NCA battery will hold twice as much energy as an LFP battery for a given weight and volume. LFP has very high stability giving it very long cycle life (>3000 vs >1000 cycles for NCA) and it is also lower cost. NCM is an intermediate solution, but much closer to NCA in terms of performance and cost than to LFP.
A major recommendation to OEMs is to align technology strategies with the automotive industry. Today, there are several very exotic battery chemistries used in the mining industry, such as: sodium nickel, lithium-titanate, and others. These solutions are unlikely to be cost competitive, nor sustainable in the long-term. Automotive economies of scale will create a 1000:1 manufacturing advantage for the conventional chemistries, meaning long-term availability and a variety of high-quality suppliers.
Cylindrical, prismatic, or pouch cells?
The next major step aft er choosing a chemistry is understanding battery cell formats. Oft entimes, this will not be selectable by the OEM directly, but it is crucial to understand the implications of a battery pack manufacturer’s decision. There are three major cell formats, cylindrical (popularised by Tesla and used by most electric vehicle startups), pouch (very popular amongst European electric vehicle OEMs), and prismatic (popular in Chinese markets, particularly for LFP).
An entire article could be written on choosing battery cell formats but, for the purposes of brevity, the strongest recommendation to OEMs is to use cylindrical cells (preferably 21700 format). They are the most cost eff ective, highest energy density, and, most importantly, they are available from virtually all suppliers. Pouch cells are extremely popular with the major Korean cell manufacturers, but an OEM is eff ectively ‘locked-in’ by using this format. Major cell shortages are currently disrupting the market in 2021, and these Tier-1 battery cells suppliers are quoting ‘TBD shipping dates’ even with contracted supply agreements. It is an extremely dangerous market today to be locked-in to any supplier.
Cell supplier flexibility is paramount in today’s market, especially for the next 2 – 3 years until supply stabilises. Remember that the chemistry is simply what goes into the cell housing – each manufacturer has their own ingredients for the ‘cake’ and the cell format is just the final shape of the ‘cake’. Cylindrical cells are the closest thing to a global standard and most suppliers are off ering the latest chemistries in 21700 form factor, in order to gain access to the automotive market. Figure 2 shows a typical cylindrical cell battery module design.
Prismatic cells are a tricky topic. At first, they appear like a good solution – available in a range of large sizes, meaning much lower numbers of cells per pack/module. However, the fundamental diff iculty with prismatic cells is that they are virtually impossible to cool properly in demanding applications (fast charging, high regenerative braking, and rapid discharging typically found in mining). Basically, the bigger the cell, the longer the heat transfer path out of the cell, meaning higher internal cell temperatures.
Furthermore, with regards to safety, bigger is not always better. Large cells contain more energy meaning they are extremely diff icult to contain in the event of a thermal runaway or fire event. Very sophisticated active suppression systems are required. By contrast, smaller cells can utilise passive propagation technologies, such as intumescent encapsulation technologies, which are highly insulating when burned and are naturally fire retardant (Figure 3).
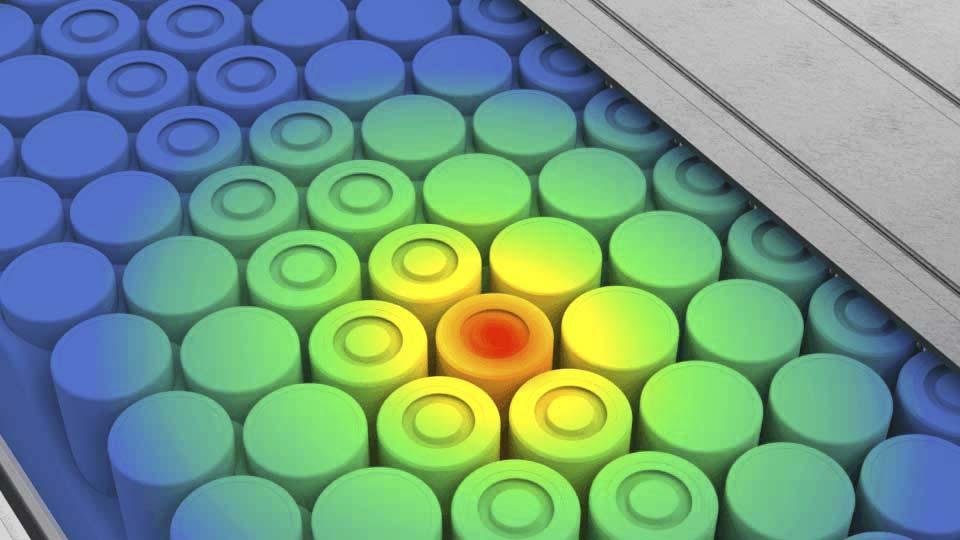
Figure 3. Containing a failed battery cell and stopping the spread of heat from causing a domino-like chain reaction failure is one of the hardest and most important aspects of battery systems engineering. Larger prismatic and pouch battery cells are extremely hard to contain in the event of failure.
Heating and cooling batteries: thermal management and the thermal system
Lithium-ion batteries do not like adverse temperatures. Most chemistries cannot be recharged below 0˚C and have a risk of thermally running away above 60˚C. Outside of these safety critical regions, there are some other major benefits for controlling the temperature of a lithium-ion battery – most notably lifetime. A general rule of thumb is that every 10˚C above 25˚C will reduce battery lifetime by half.
Cold ambient environments are uncommon in underground mining applications, but oft entimes equipment might return to the surface at end of shift or for maintenance. Heating the battery is very important in this scenario. Heating batteries is relatively straightforward and can be done using electrical heating systems, or by using more advanced technologies like heat pumps. Batteries also self-heat when used so this is typically only required for starting.
Deep underground mining is typically known for being hot with wall-rock temperatures that can exceed 60˚C. Active battery cooling is mandatory in these types of
applications to maintain safety and achieve satisfactory lifetimes.
Not all thermal management is created equal. Many battery systems today are being marketed as ‘liquid-cooled’, but there are tremendous diff erences between the type of cooling employed. Most systems today are using cold-pate technology, which is simply an extruded aluminium plate with glycol-water circulated through it. The battery cells or Figure 4. (left to right) 1: Cold plate cooling. 2: Sidewall modules sit on this plate and can be heated or cooled. More (conventional) cooling. 3: Sidewall (Xerotherm®) cooling. advanced solutions use side-wall battery cooling or 4: Immersion cooling. 5: Air cooling. immersion cooling technologies. Figure 4 shows the four main methods of battery cooling: cold plate cooling, sidewall cooling, immersion cooling, and air cooling.
Cooling large prismatic or pouch cells can be very challenging, as the internals of a battery cells are extremely poor at conducting heat. This results in the thermal management systems only heating or cooling the outer skin or region of the battery and having little eff ect on the core. This could theoretically allow thermal runaway if the core of the cell exceeds its safety threshold.
The internal aspects of battery pack heating and cooling is called thermal management. This, however, is only half of the solution, as most battery pack manufacturers today just specify a required flowrate and temperature in and out of the battery pack. The battery pack manufacturer provides a method to get the heat out of the battery, but it is left as an exercise for the OEM to get it off the vehicle.
Getting heat off the vehicle is one of the most challenging parts of electrification today. Overlooking the thermal system can easily add 6-months worth of development time to an electrification programme. Conclusions
Choosing a battery manufacturer
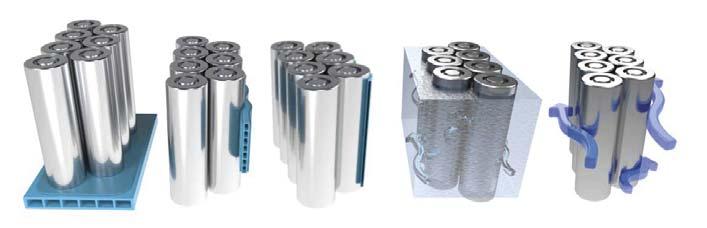
There are a variety of battery pack manufacturers supplying the mining industry today. They vary significantly in capability and product off ering – from basic assemblers of prismatic cells in sheet metal boxes, to the leading European pack suppliers with advanced manufacturing, cell characterisation, safety, and thermal management technology.
OEMs are strongly encouraged to buy a complete battery system, and not just a battery box and add a separate control box or battery management system (BMS). The integration and testing of the whole system is the bulk of the engineering challenge with battery pack design. The battery pack and the BMS go hand-in-hand, therefore they must be developed by the same company.
Some key considerations when selecting a battery pack manufacturer include: Do they offer thermal management – if so, what type of technology do they use? Do they have battery pack test capability? Do they support integration (electrical, thermal, and controls)? Can they meet the requirements of the full product portfolio? Do they manufacturer their own BMS? Do they create the software and control algorithms for the battery pack? Do they have any capability around battery cell characterisation? Can they support thermal system design and development?
Electrification is hard. A key challenge for OEMs is that electrification solutions are unlikely to become a commodity solution until closer to the end of the decade. OEMs need to quickly educate themselves and take a holistic view of their product portfolios and to make their machinery compatible with emerging battery platform solutions.
There are major capacity constraints in the market today, with most leading battery pack manufacturers overwhelmed with OEM requests and orders. It is highly recommended that OEMs engage with suppliers immediately and secure supply agreements, or risk missing the greatest disruption the mining equipment industry has seen since the invention of the steam engine.
Hexagon PPM transforms your mining operations with intelligent technology, operational excellence and improved productivity.
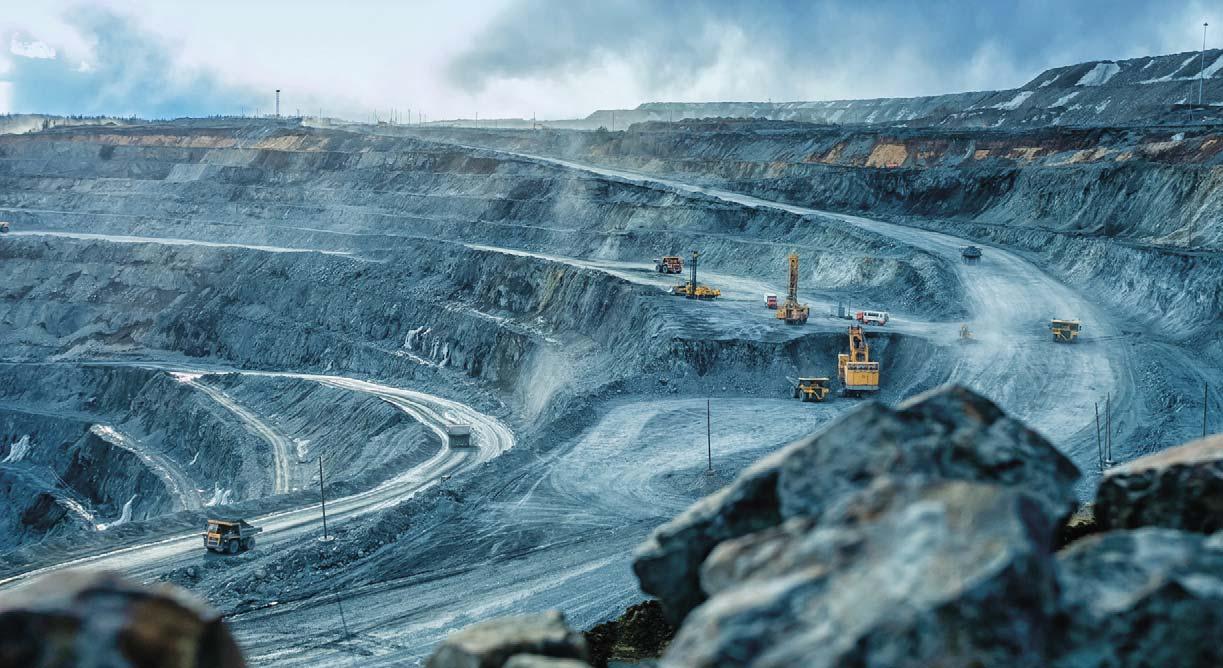
| Visit bit.ly/HexagonOpsExcelMining