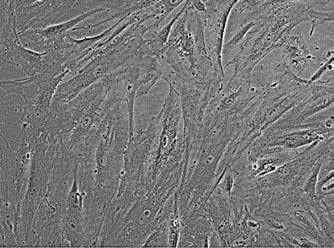
11 minute read
Adventitial extracellular matrix from aneurysmal aorta fails to promote pericyte contractility
Kaitlyn Wintruba a , Bryant Fisher b , Jennifer C. Hill b , Tara D. Richards b , Marie Billaud b-d , Amadeus Stern d , Thomas G. Gleason b-d , Julie A. Phillippi b-d a Department of Chemical Engineering, b Department of Cardiothoracic Surgery, c Department of Bioengineering, d McGowan Institute for Regenerative Medicine University of Pittsburgh, Pittsburgh, PA, USA
Kaitlyn Wintruba
Kaitlyn Wintruba is in her second year at the Swanson School of Engineering where she studies Chemical Engineering. After graduating, Kaitlyn plans to pursue her interest in regenerative medicine and biomedical research in graduate school.
Dr. Julie Phillippi is Associate Professor with Tenure in the Department of Cardiothoracic Surgery, School of Medicine with a secondary appointment in the Department of Bioengineering Swanson School of Engineering. Dr. Phillippi’s research is focused on the role of perivascular progenitor cells, adventitial biology, and vasa vasorum function in human aortic disease. Her work is currently funded by the National Heart, Lung and Blood Institute under award # R01HL131632. Dr. Julie Phillippi
Significance Statement
Ascending thoracic aortic aneurysm (TAA) is a lifethreatening condition, for which there are no strategies for early intervention. Our work supports the use of an extracellular matrix hydrogel as a potential regenerative biomaterial in the setting of TAA and translation towards microvascular regeneration in clinical applications.
Category: Experimental research Keywords: Pericyte, Extracellular Matrix Hydrogel, Adventitia, Aortic Aneurysm Abbreviations: thoracic aortic aneurysm (TAA), extracellular matrix (ECM), aortic adventitia (Adv), porcine aortic adventitia (pAdv), human aortic adventitia (hAdv), fibroblast growth factor 2 (FGF2), platelet derived growth factor (PDGF), vascular endothelial growth factor A (VEGF-A), transforming growth factor beta 1 (TGFβ1), dimethyl sulfoxide (DMSO)
Abstract
Ascending thoracic aortic aneurysm (TAA) is a life-threatening condition lacking adequate diagnostics and risk adjudication for aortic dissection or rupture. Studying the adventitia, the outer most layer of the aorta, our group previously uncovered downregulation of several pro-angiogenic factors and reduced density of the microvascular network of vasa vasorum in aneurysmal aortic specimens. Knowledge of how adventitial extracellular matrix (ECM) influences the function of vasa vasorum-associated cells could potentially lead to developing a better diagnostics and therapies for TAA or aortic dissection. We hypothesized that adventitial ECM from normal aorta enhances pericyte function through a growth factor-mediated mechanism deficient in aneurysm-derived aortic adventitia. To test this hypothesis, we quantified pericyte contractility within a 3D hydrogel tissue culture scaffold as a measure of pericyte function. Human aortic adventitia (Adv)-derived pericytes were cultured within 2mg/mL bovine Type I collagen gels in the presence or absence of lyophilized human or porcine Adv ECMs. Addition of Adv ECMs to collagen accelerated gelation at 37˚C as evidenced by a higher optical density (p<0.05). Addition of normal human Adv ECM increased pericyte contractility when compared with aneurysm-derived Adv ECM (p<0.001). Inhibition of TGF -1R decreased porcine Adv ECM-induced contractility when compared with cells treated with vehicle and porcine Adv ECM (p<0.001). This work supports the use of porcine-derived ECM hydrogels to improve function of vasa vasorum-associated cells as a potential therapeutic biomaterial for microvascular regeneration in human aortic disease.
1. Introduction
Ascending thoracic aortic aneurysms (TAAs) are often asymptomatic, and the only treatment to prevent aortic dissection or rupture is elective replacement of the ascending aorta. Aortic dissection occurs in 5 to 30 cases per million of the population annually [1] with the mortality rates of aortic dissection worldwide being 1% per hour without surgical intervention [2]. Interactions between the cellular and extracellular matrix (ECM) components of the intimal, medial, and adventitial layers of the aorta mediate blood flow throughout the body. Currently, the complex cellular molecular mechanisms inciting TAA and driving disease progression remain incompletely understood. Improved understanding of these mechanisms could help develop less invasive treatment options.
Prior work in the Thoracic Aortic Disease Research Laboratory identified involvement of the adventitial microenvironment in the pathogenesis of TAA. Growth factors within adventitial ECM such as fibroblast growth factor 2 (FGF2), platelet derived growth factor (PDGF), vascular endothelial growth factor A (VEGF-A) can influence vasa vasorum-associated cells. These pro-angiogenic growth factors involved with vasculogenic function were found to be downregulated in human aneurysmal aortic specimens and porcine-derived adventitial (pAdv) ECM exhibited FGF2-mediated angiogenic activity in vitro and in vivo [3]. Transforming growth factor beta (TGFβ) is involved in various vasculogenic functions
and induced a contractile phenotype in aortic smooth muscle cells in vitro [4]. Within the adventitia, TGFβ serves as a pro-angiogenic growth factor influencing perivascular progenitor cell function. The vasa vasorum exhibited microvascular remodeling and lower density suggestive of reduced neovascularization in aortic specimens from TAA patients [5].
Furthermore, several populations of vasa vasorum-associated progenitor cells reside within the adventitia using analytical flow cytometry and immunohistochemistry to identify pericytes with the surface marker profile of CD146 + /CD34 ± /CD31 - [6]. Pericytes serve as perivascular progenitor cells within capillaries and micro vessels and in ex vivo culture exhibited unique spheroid formation and sprouting on Matrigel substrates [6], two behaviors that mimic vasculogenic function. Pericytes cultured in the presence of TGFβ1 and PDGF developed a spindle-shape morphology and increased expression of markers for smooth muscle lineages [6]. In the present study, we hypothesized that adventitial ECM from normal aorta promotes pericyte function by increasing pericyte contractility through a growth factor-mediated mechanism deficient in aneurysm-derived aortic adventitia. To test this hypothesis, we quantified pericyte contractility within a 3D hydrogel tissue culture scaffold as a measure of pericyte function.
2. Methods
Human ascending aortic adventitial tissue specimens were collected from patients undergoing ascending aortic and/or aortic valve replacement, or heart transplantation with Institutional Review Board approval and using an informed consent process. Pericytes (Figure 1) were isolated from the adventitia of non-aneurysmal human aorta and then immortalized using a lentiviral vector to deliver HPV-E6/E7 to avoid senescence and phenotypic changes at high passage. A surface marker profile of CD146 + /CD31 - indicative of pericytes was routinely enriched with magnetic bead separation, during which biomolecules are attached to magnetic beads of specific affinity and filtered through a magnetic separation column such that only positive marker expression is captured. Adventitial ECMs were prepared from decellularized and lyophilized adventitial specimens from human and porcine aorta. Human aortic tissue specimens were collected from patients with ages ranging from 17 to 82 years. The Adv ECMs were then finely ground to produce an ECM particulate. These ECMs were previously shown to contain minimal DNA content while retaining numerous bioactive growth factors [3].
Figure 1: CD146 + / CD31 - Pericytes. Representative phase contrast image of pericytes with uniform elongated cell morphology.
Pericytes were cultured within 2mg/mL bovine Type I collagen gels in the presence or absence of normal or aneurysmal human adventitial (hAdv) ECMs (250µg/mL) within the collagen gel. Parallel experiments cultured pericyte-embedded collagen gels in the presence or absence of pAdv ECM (250µg/mL) or with TGFβ-1 receptor inhibitor SB431542 (100nM) added to the culture medium or DMSO as the vehicle control. Optical absorbance readings (405nm) over 3h of dry heat incubation (37˚C) were obtained to calculate gelation kinetics of collagen blended with hAdv and pAdv ECMs. Normalized absorbance was calculated at two-minute intervals using the following equation:

(1)
where A represents the absorbance reading at a particular time point, A max represents maximum absorbance, and A 0 represents the initial absorbance. Pericyte-embedded collagen gels were imaged with bright-field microscopy (Nikon SMZ25, 1X SHR Plan Apo Objective), and cell contraction was quantified by measuring the initial gel area and after 48 hours of culture (Nikon Elements AR 4.60). All experiments were performed with four assay replicates and cells obtained from two different patients (n=4). Statistical analysis was carried out utilizing one-way ANOVA with Tukey’s post-hoc test (SigmaPlot 12.5) and a p<0.05 was considered statistically significant.
3. Results 3.1 Adventitial ECM Promotes Pericyte Contractility
All ECM treatments increased pericyte contractility as evidenced by decreased gel area when compared with pericyteembedded collagen gels lacking ECM treatment (p<0.001). Addition of normal hAdv ECM doubled the degree of pericyte contractility when compared with gels lacking any ECM treatment and aneurysm-derived hAdv ECM (Figure 2). Aneurysm-derived hAdv ECM failed to induce contractility above baseline (Control). Inhibition of TGFβ-1 receptor decreased pAdv ECM-induced contractility when compared with cells treated with vehicle (±DMSO) and pAdv ECM (Figure 3).
Figure 2: Pericyte Contractility of Human Adv ECMs. Contractility of collagen gels without pericytes (No Cell), with pericytes (Control), and with pericytes treated with 250µg/mL hAdv ECMs, respectively. Bars represent the mean contractility of four assay replicates ± standard error of the mean. * indicates p<0.001.
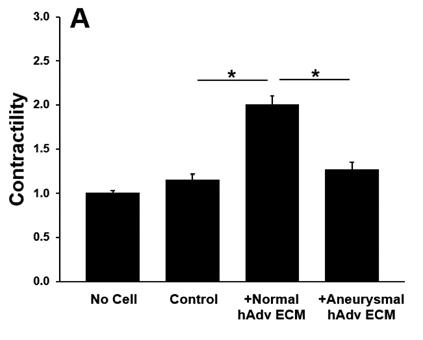
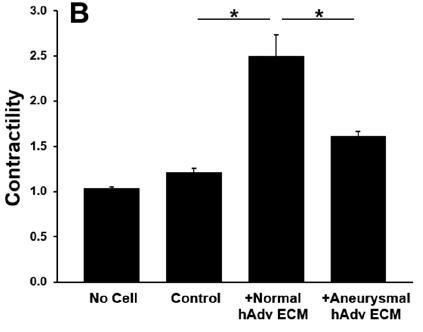
Figure 3: pAdv ECM-induced Pericyte Contractility ± TGFβ-1 Inhibitor. Contractility of 250µg/mL pAdv ECM treated pericyte-embedded collagen gels with TGFβ-1 inhibitor (+SB431542) or vehicle (+DMSO) and respective acellular and cell controls. Bars represent the mean contractility of four assay replicates ± standard error of the mean. * indicates p<0.001.
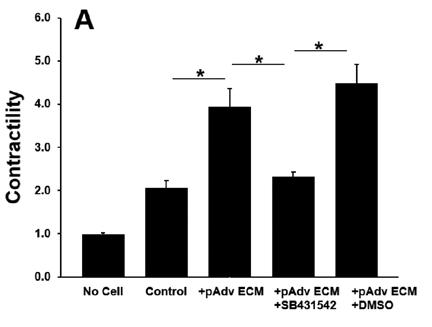
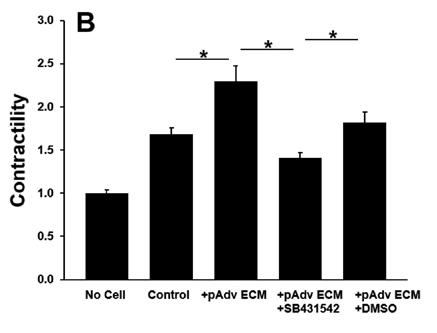
3.2 hAdv ECM Accelerates Collagen Gelation
Calculation of normalized absorbance during gelation revealed peak gelation was reached within 100 minutes for acellular hydrogels, and in the presence of cells and Adv ECMs (Figure 4). Addition of hAdv ECM to Type I collagen accelerated gelation as evidenced by a higher optical density (Figure 4) and decreased time to 50% gelation (Table 1) (p<0.05). Conversely, addition of pAdv ECM decreased the gelation rate compared to collagen alone. Furthermore, the gelation rate was greater with the addition of aneurysmal hAdv ECM compared to normal hAdv ECM.
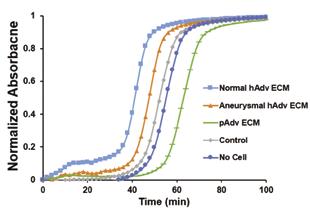
108 Undergraduate Research at the Swanson School of Engineering Figure 4: Collagen Gelation ± Adv ECMs. Lines depict normalized absorbance readings of pericyte-embedded collagen (2mg/mL) blended with normal and aneurysmal hAdv and pAdv ECMs (250µg/mL) over 100 minutes.
No Cell Control Normal hAdv ECM Aneurysmal hAdv ECM pAdv ECM Rate (OD/min)
0.0659 ± 0.0041 0.0616 ± 0.0024 0.0656 ± 0.0035 0.0675 ± 0.0039 0.0563 ± 0.0029 T 1/2 (min)
54.76 ± 1.11 50.84 ± 1.37 47.11 ± 2.16 *41.10 ± 0.78 63.14 ± 1.20
Table 1: Gelation Kinetics of Collagen Blended with Adv ECMs. Gelation rate (optical density divided by time) and time required for 50% gelation (T 1/2 ) of pericyte-embedded collagen blended with Adv ECMs. Data represents mean of four assay replicates ± standard deviation. * indicates p<0.05 compared to acellular collagen gel.
4. Discussion
Less pericyte contractility with aneurysm-derived hAdv ECM compared with normal hAdv ECM treatment demonstrates adverse effects in pericyte function may be caused by growth factor deficiencies in adventitia from TAA [3]. Porcine Adv ECMinduced pericyte contractility and SB431542-mediated inhibition of contractility indicates that Adv ECM influences pericyte function via a TGFβ1-mediated mechanism. TGFβ1-mediated pericyte contractility supports the hypothesis that normal hAdv ECMinduced increase in pericyte function is growth factor dependent. Notably, the validity of these results could be improved with the testing of additional cell lines. Wrinkler et al. showed pericytes wrap capillaries and express contractile proteins to potentially regulate capillary diameter by constricting or relaxing [7]. However, it remains unclear whether this is true in vivo. Another study has shown functional cell behavior in response to ECM hydrogels to be comparable to collagen substrate for cardiac applications [8]. Our work expands previous studies by examining functional effects of aortic adventitial pericyte-ECM interaction in a 3D microenvironment.
ECM hydrogels represent a candidate biomaterial for less invasive treatment in the setting of TAA due to their potential to replenish growth factors and restore a pro-angiogenic adventitial microenvironment. Pericytes comprise a subpopulation of cells within the adventitia that localize to the vasa vasorum and serve as progenitor cells with vasculogenic behavior. The therapeutic potential of an ECM hydrogel likely lies, at least in part, in its ability to promote pericyte function. Future experiments should determine baseline and pAdv ECM-induced contractility of pericytes isolated from adventitia of aneurysmal human aorta. Furthermore, additional studies will seek to explore how adventitial ECM affects other pericyte functions including sprouting, migration, and chemotaxis.
5. Conclusions
Knowledge that adventitial ECM promotes pericyte function by significantly increasing pericyte contractility and accelerating hydrogel gelation might assist in developing a novel therapeutic intervention for patients at risk for TAA or aortic dissection. The pAdv-induced and TGFβ1-mediated increase in pericyte contractility supports the use of porcine-derived ECM hydrogels to improve function of vasa vasorum-associated cells as a potential therapeutic biomaterial as part of a microvascular regeneration strategy to treat human aortic disease.
6. Acknowledgements
This study was supported by the National Institutes of Health under award #HL131632 (JAP), #HL127214 (JAP) and #HL109132 (TGG), UPMC Health System Competitive Medicine Research Fund (JAP) and the McGowan Institute of Regenerative Medicine Summer School Program (KLW).
7. References
[1] Davies RR, Goldstein LJ, Coady MA, et al. Yearly rupture or dissection rates for thoracic aortic aneurysms: simple prediction based on size. Annals of Thoracic Surg. 73 (2002) 17-28.
[2] Masuda Y, Yamada Z, Morooka N, et al. Prognosis of patients with medically treated aortic dissections. Circulation. 84 (1991) 117-113.
[3] Fercana GR, Yerneni S, Billaud M, et al. Perivascular extracellular matrix hydrogels mimic native matrix microarchitecture and promote angiogenesis via basic fibroblast growth factor. Biomaterials. 123 (2017) 142-154. [4] Crosas-Molist E, Meirelles T, López Luque J, et al. Vascular smooth muscle cell phenotypic changes in patients with Marfan syndrome. Arteriosclerosis, Thrombosis, and Vascular Biology. 35 (2015) 960-972.
[5] Billaud M, Hill JC, Richards TD, et al. Medial hypoxia and adventitial vasa vasorum remodeling in human ascending aortic aneurysm. Front. Cardiovasc. Med. 5 (2018) 124.
[6] Billaud M, Donnenberg VS, Ellis BW, et al. Classification and functional characterization of vasa vasorum-associated perivascular progenitor cells in human aorta. Stem Cell Reports. 9 (2017) 292-303.
[7] Winkler EA, Rutledge WC, Kalani MYS, et al. Pericytes regulate cerebral blood flow and neuronal health at a capillary level. Neurosurgery. 81:5 (2017) N37-N38.
[8] DeQuach JA, Mezzano V, Miglani A, et al. Simple and high yielding method for preparing tissue specific extracellular matrix coatings for cell culture. PLoS One. 5 (2010) 130-139.